- 1Department of Medicine and Surgery, Unit of Neurology, Neurophysiology, Neurobiology and Psychiatry, Università Campus Bio-Medico di Roma, Rome, Italy
- 2Neurological Clinic, Department of Experimental and Clinical Medicine (DIMSC), Marche Polytechnic University, Ancona, Italy
- 3Fondazione Policlinico Universitario Campus Bio-Medico, Rome, Italy
The semantic variant of primary progressive aphasia (svPPA), known also as “semantic dementia (SD),” is a neurodegenerative disorder that pertains to the frontotemporal lobar degeneration clinical syndromes. There is currently no approved pharmacological therapy for all frontotemporal dementia variants. Transcranial direct current stimulation (tDCS) is a promising non-invasive brain stimulation technique capable of modulating cortical excitability through a sub-threshold shift in neuronal resting potential. This technique has previously been applied as adjunct treatment in Alzheimer’s disease, while data for frontotemporal dementia are controversial. In this scoped review, we summarize and critically appraise the currently available evidence regarding the use of tDCS for improving performance in naming and/or matching tasks in patients with svPPA. Clinical trials addressing this topic were identified through MEDLINE (accessed by PubMed) and Web of Science, as of November 2022, week 3. Clinical trials have been unable to show a significant benefit of tDCS in enhancing semantic performance in svPPA patients. The heterogeneity of the studies available in the literature might be a possible explanation. Nevertheless, the results of these studies are promising and may offer valuable insights into methodological differences and overlaps, raising interest among researchers in identifying new non-pharmacological strategies for treating svPPA patients. Further studies are therefore warranted to investigate the potential therapeutic role of tDCS in svPPA.
1. Introduction
In the 1980s, the recognition of clinical entities distinct from Alzheimer’s disease led to the identification of primary progressive aphasias (PPAs) (Mesulam, 1987), neurodegenerative disorders characterized by early and prominent speech or language impairment with relative sparing—at least in the mild stage—of other cognitive domains, such as memory or behavior. Although other symptoms may present in the later stages of PPA, language impairment remains the most prominent feature (Gorno-Tempini et al., 2011).
Three variants of PPA are recognized. While the logopenic variant is largely considered as an atypical variant of Alzheimer’s disease, the nonfluent/agrammatic variant and the semantic variant (svPPA) are classified as frontotemporal dementia (FTD) syndromes because of their association with frontotemporal lobar degeneration pathology (Mendez, 2019). Differentiating between PPA subvariants can be challenging due to overlapping clinical features. However, an accurate diagnosis is crucial for optimal clinical management and targeted interventions.
According to the current diagnostic criteria (Pick, 1892; Gorno-Tempini et al., 2011), the first diagnostic step requires proving that the primary clinical feature is language impairment, without other clinical-neuropsychological features that may indicate an alternative diagnosis (Table 1). The current criteria also include a set of mandatory core features and supporting features that allow clinically accurate identification of the three main PPA variants (Table 2).
After Pick’s 19th-century description of a progressive language disorder associated with frontotemporal atrophy (Pick, 1892), and Warrington’s, 1975 paper about the selective impairment of semantic memory in three patients with anomia (Warrington, 1975), in 1989 Snowden proposed the term “semantic dementia” (SD) (Snowden et al., 1989), defining for the first time a condition that has continued to fascinate behavioral neurologists in the 21st century. Despite some authors defining svPPA as the purely linguistic form of SD (Adlam et al., 2006), using the term “semantic dementia” for indicating the progressive impairment of multi-modal semantic representations (Hodges et al., 1992; Mesulam, 2001, 2003), in this paper the two terms are used interchangeably (Gorno-Tempini et al., 2011).
Although research on SD prevalence is lacking, it is expected to account for one-fourth to one-third of FTD cases (Hodges and Patterson, 2007; Landin-Romero et al., 2016), i.e., 2.5–7.3 per 100,000 people (Onyike and Diehl-Schmid, 2013; Landin-Romero et al., 2016). SD usually manifests before the age of 65, but one-fourth of cases may occur after the age of 70 (Hodges et al., 2010). It severely impairs patients’ communication abilities, having a significant influence on their familial and socio-professional life (Hodges et al., 2010). The median survival is on the order of 10 to 13 years, which is longer than is usually associated with FTD (Hodges et al., 2010).
From a pathological standpoint, the regions primarily affected by SD-related neurodegeneration are the temporal pole and the anterior temporal lobe (ATL) of both hemispheres (Gorno-Tempini et al., 2011; Mesulam et al., 2014), with approximately 70% of patients presenting with major left-sided involvement and 30% presenting with predominant right-sided involvement (Hodges et al., 2010; Kumfor et al., 2016).
Overall, SD patients experience a progressive and severe loss of conceptual knowledge, with the main deficit involving word meaning (Gorno-Tempini et al., 2011; Mesulam et al., 2014). This results in anomia, impaired word comprehension and fluent but content-empty speech (Davies et al., 2005), and with preserved grammar and speech articulation (Mesulam et al., 2014). Furthermore, SD can affect face recognition (Snowden et al., 2004; Luzzi et al., 2017), object feature attribution (Garrard and Carroll, 2006), sound-picture matching (Bozeat et al., 2000), object-use matching (Corbett et al., 2009), and arithmetic knowledge (Luzzi et al., 2013). The clinical presentation varies according to the side of the brain that is mainly affected by the degenerative process. The most noticeable symptom of left-sided SD is the involvement of language, with anomia and loss of single-word meaning. In right-sided SD, the prominent impairment is associative prosopagnosia (i.e., the inability to recognize familiar people’s faces), together with the inability to recognize animals and items.
Previous studies have found a link between ATL gray matter loss and impairment in a variety of semantic tasks [as picture naming (Rosen et al., 2002; McMillan et al., 2004) and word-picture association (Mummery et al., 2000)]. As a result of ATL degeneration, functional connectivity between brain areas in the frontal, temporal, parietal, and occipital lobes, including visual and auditory association cortices, is compromised (Guo et al., 2013).
The loss of connectivity has been evaluated structurally as a decrease of white matter volume in the left temporal lobe, periventricular white matter, and the corpus callosum (Good et al., 2002), as well as damage to white matter tracts such as the inferior longitudinal fasciculus and the uncinate fasciculus (Rohrer et al., 2010). Furthermore, even if the Papez circuit is likely implicated in PPA pathogenesis, the mammillary bodies and the body and tail of the hippocampus are frequently spared, and this might explain why the episodic memory is spared (Tan et al., 2014). Cortical hypometabolism of the ATL cortex—and occasionally of the subgenual area and the right anterior cingulate cortex –is a valuable imaging hallmark useful for the diagnosis (La Joie et al., 2014).
There is no approved treatment for svPPA and the other PPA variants because speech therapy protocols have not been validated and pharmaceutical treatments have not demonstrated significant results (Vercelletto et al., 2011; Boxer et al., 2013; Monti et al., 2013). Non-pharmacological approaches focused on non-invasive brain stimulation (NIBS) might then have a role in disease treatment and diagnosis (Di Lazzaro et al., 2021). Indeed, it is known that neurodegenerative conditions of different etiologies have their own “neurophysiological signature” in terms of selective involvement of neural circuits. This represents the rationale for the therapeutical application of NIBS techniques such as repetitive transcranial magnetic stimulation (Lambon Ralph, 2014).
Clinically, svPPA is primarily characterized by a significant impairment in a particular cognitive function, specifically semantic knowledge. This feature is accompanied, uniquely in this variant and notably in the initial stages of the disease, by a corresponding “neuroanatomical signature,” denoting the engagement of discrete brain regions (mainly the ATL and the temporal pole). This SD uniqueness underscores the significance of a comprehensive study, as it holds promising implications for unraveling the underlying neural mechanisms associated with language processing and semantic memory (Deakin et al., 2004).
Transcranial direct current stimulation (tDCS) is one of the most widely employed NIBS techniques, characterized by numerous advantages (e.g., cost, portability, comfort, possibility of delivering during rehabilitation). It consists of a weak electric current (with an average intensity of 1–2 mA) delivered between two electrodes placed on separate areas of the scalp (Elder and Taylor, 2014). The electric current produces a polarization gradient between electrodes that modulates cortical excitability through a sub-threshold alteration of the resting membrane potential (Ulm et al., 2015). Although tDCS has been used for several years in neurological, otorhinolaryngological, and psychiatric research, a 2017 comprehensive review highlighted that we currently have a sufficient level of evidence to provide recommendations for employing tDCS in just a few disorders (i.e., chronic neuropathic pain due to spinal cord lesion, fibromyalgia, tinnitus, depression, and addiction/craving syndromes) (Lefaucheur et al., 2017). According to that review, for the rest of the conditions on which it has been tried, tDCS cannot yet be the subject of therapeutic recommendations because of the often-inconsistent trial results. This discrepancy would likely be due to differences in the protocols used, such as in current intensity, number and timing of stimulation sessions, placement, shape and size of electrodes (Lefaucheur et al., 2017).
The goal of this study is to describe and critically evaluate the current available evidence on tDCS-based therapy for improving language in patients with svPPA and suggest implications for the future research.
2. Methods
This scoped review adopted a systematic approach in the processes of research question selection, search and selection of literature.
Clinical trials published up to November 2022 (week 3) and addressing the topic were identified using Web of Science and MEDLINE accessed by PubMed. We developed subject-specific search strategies for each academic database, as follows.
Web of Science search strategy: [TS = (semantic dementia)] OR [TS = (svPPA)] OR [TS = (semantic variant PPA)] OR [TS = (semantic variant primary progressive aphasia)] AND [TS = (tDCS)] OR [TS = (transcranial direct current stimulation)].
MEDLINE search strategy: [semantic dementia (Title/Abstract)] OR [svPPA (Title/Abstract)] OR [semantic variant primary progressive aphasia (Title/Abstract)] OR [frontotemporal dementia (Title/Abstract)] AND [tDCS (Title/Abstract)] OR [electrical brain stimulation (Title/Abstract)] OR [transcranial direct current stimulation (Title/Abstract)].
The literature selection and exclusion were conducted by a single researcher, based on the PICOS (Patient Intervention Comparison Outcome Study design) of the PRISMA protocol (Moher et al., 2009). The present review included: (1) studies conducted on svPPA patients, (2) studies evaluating the clinical effects of tDCS, (3) studies with an experimental design, and (4) studies written in English. The exclusion criteria were: qualitative studies, case reports, unpublished papers and articles written in other languages. Duplicated publications were excluded by comparing titles and abstracts. Moreover, publications not related to the study topic were excluded.
Although the methodological footprint employed by the present study resembles that of a systematic review, it endeavors to address a highly specific question, necessitating the inclusion of articles that require qualitative analysis of figures and raw data. Furthermore, this study was not prospectively registered in any of the register for systematic reviews. For these two reasons, the study has been conducted as a scoped review.
As a result, the final six publications were reviewed.
Supplementary Figure S1 shows the flowchart of the study selection process, according to the PRISMA protocol.
3. Results
Table 3 summarizes the main features of published data on tDCS in svPPA patients, as described in this review.
So far, only one trial specifically addressed the efficacy of tDCS in improving semantic performance (evaluated as picture-to-picture and word-to-word matching tests) in svPPA patients (Teichmann et al., 2016) while the other available data originate from trials designed to investigate the effects of tDCS in different degenerative speech diseases, including svPPA (Hung et al., 2017; Roncero et al., 2017; Ficek et al., 2018; Tsapkini et al., 2018). For the purpose of the present review, we used the specific subgroup analysis about the semantic variant reported in one study (Tsapkini et al., 2018), while for the others (Hung et al., 2017; Roncero et al., 2017; Ficek et al., 2018) we addressed the raw data explicitly related to svPPA patient, or plots for distinct subgroups, as reported by the authors.
Teichmann et al. (2016) conducted a monocentric, randomized, sham-controlled, crossover trial for evaluating the efficacy of tDCS in improving svPPA patients’ performance in semantic word-to-word and picture-to-picture matching. SvPPA diagnosis was based on neuropsychological testing, Gorno-Tempini’s criteria, and metabolic imaging-supported evidence (i.e., PET-FDG). The authors found that a single 20 min session of tDCS (1.59 mA) enhanced performance in a “find the intruder”-type task when compared to sham stimulation (Table 3; Teichmann et al., 2016). Twelve participants were enrolled and underwent three stimulation sessions each: left excitatory tDCS (with the anode over the left temporal pole and the cathode over the contralateral supra-orbital region), right inhibitory tDCS (with the cathode over the right temporal pole and the anode over the contralateral supraorbital region), and sham. The study cohort’s average age was 66.8 years, and 33% were female. Cognitive assessments were carried out at baseline and immediately after the tDCS session, with no further follow-up or language training. The primary efficacy outcome of improved performance in the word-to-word matching task occurred in both left-excitatory tDCS (baseline 33% ± 29 correct, post-tDCS 48% ± 23 correct; F = 5.5, p = 0.029) and right-inhibitory tDCS (baseline 28% ± 26 correct, post-tDCS 43% ± 32 correct; F = 6.5, p = 0.027), whereas sham stimulation had no effect on performance (baseline 34% ± 27 correct, post-sham 31% ± 24 correct; F < 1). Moreover, analyses on reaction times revealed that right-inhibitory tDCS speeded post-stimulation reactions for the “living” category of presented items [baseline (3,575 ms ± 749, post-tDCS 3,269 ms ± 650, F = 11.0, p = 0.007)] (Teichmann et al., 2016).
Hung et al. (2017) designed a simple clinical trial without a control group that included both pre- and post-tDCS assessments employing an oral naming task. Diagnoses were based on clinical criteria. In addition to a semantic training strategy based on Reilly et al. (2016) research, they administered 10 sessions of 30 min tDCS (1.5 mA) over 2 weeks, with the anode over the left inferior temporoparietal area and the cathode centered on the forehead. A 6 months follow-up visit with a new cognitive testing was also reported. A total of 5 individuals with semantic or logopenic PPA or Alzheimer’s disease were recruited in the research. The svPPA cohort contained three patients with an average age of 67.3 years of which one was female. This paper does not expressly disclose results in the subset of svPPA individuals, but it does contain an image reporting raw individual data that appears to demonstrate a significant effect of tDCS on naming performance. Furthermore, in the discussion session, the authors note that svPPA patients were most likely to experience a sustained benefit from tDCS (Hung et al., 2017).
Roncero et al. (2017) presented a randomized, crossover-design, sham controlled clinical trial designed to study the efficacy of tDCS in patients with Alzheimer’s disease or PPA. Diagnoses were made according to neuropsychological and language testing, clinical criteria, and PET-FDG findings. In this study, the anode was placed over the left inferior temporoparietal region, while the cathode was placed over the right fronto-orbital region. The experimental phase consisted of ten 30 min tDCS (2 mA) treatments for 18 days in combination with a picture-naming training, compared with sham stimulation plus the same training. Two svPPA patients were enrolled among a total of 10 participants. The svPPA-mean group’s age was 63.5 years, and both patients were female. Two figures in the text showing raw individual data seem to demonstrate a measurable impact of tDCS on naming of trained and untrained items, although no explicit results for patients with svPPA are mentioned in the manuscript (Roncero et al., 2017).
Tsapkini et al. (2018) conducted a randomized, within-subject, crossover trial on patients with primary progressive aphasia (all variants), comparing the effect of 15 sessions of 20 min tDCS (2 mA, with the anode over the left inferior frontal gyrus and the cathode on the right cheek) in addition to speech therapy, with sham stimulation and speech therapy. Diagnoses were based on Gorno-Tempini criteria. A total of 36 patients were enrolled; among them, 10 suffered from svPPA, with a mean age of 68.6 years (five females). The cognitive evaluations were performed at baseline, immediately after the tDCS sessions, after 2 weeks, and after 2 months from the last session, and focused especially on written naming and spelling accuracy. In the subgroup analysis conducted on svPPA patients, they did not find any significant effect of tDCS as add-on to language training, at each timepoint (trained items effect size = 0.07, 95% CI 0.70–20.56, p = 0.793; untrained items effect size = 0.36, 95% CI 2.66–21.94, p = 0.466) (Tsapkini et al., 2018).
In 2018, the same research group published another paper presenting a subgroup analysis of their trial. In this study, they employed the same stimulation paradigm (15 sessions of 20 min anodal 2 mA tDCS over the left inferior frontal gyrus versus sham stimulation, in addition to language therapy) (Ficek et al., 2018), but the patient’s accurate diagnosis was determined not only through neuropsychological/language testing and clinical criteria but also using structural brain imaging, namely magnetic resonance imaging (MRI). The semantic variant of PPA’s cohort included 8 individuals (descriptive characteristics of subgroups, such as average age and sex, are not reported in the publication). Although results for patients with svPPA are neither explicitly nor systematically presented, a figure published within the paper seems to demonstrate a significant impact of tDCS on writing accuracy following 15 stimulations (Ficek et al., 2018).
Roncero et al. (2019) published another paper in 2019 focusing on individuals with all-variant PPA. The study was designed as a randomized, within-subject sham-controlled crossover trial, assessing the effect of 10 sessions of 30 min tDCS at 2 mA, coupled with language training for a picture-naming task. A formal diagnosis of PPA was established through neuroimaging scans (e.g., FDG PET, MRI) and neuropsychological testing. The efficacy of sham tDCS was compared to two active tDCS configurations: parieto-temporal anodal stimulation, with the anode positioned over TP9 and cathode on the right fronto-orbital area; and dorsolateral prefrontal cortex (DLPFC) stimulation, with the anode placed over left DLPFC (F3) and the cathode over the right deltoid muscle. Follow-up assessments were conducted at 2 weeks and 2 months after the final tDCS session. Although no formal analysis was performed on the four sv-PPA patients (mean age 61.8 years, one female) included out a total of 27 enrolled individuals, the authors observed an immediate improvement in naming accuracy of untrained items only in one svPPA participant following the 10 sessions of parieto-temporal tDCS (Roncero et al., 2019).
4. Discussion
To date, few data have been published about the effects of tDCS on cognitive functions in svPPA patients. These data are characterized by significant methodological heterogeneity as detailed below.
4.1. Anodal vs. cathodal tDCS
TDCS can deliver electric stimulations in two different ways: anodal and cathodal stimulation. Anodal stimulation (i.e., placing the anode over the targeted area) is supposed to shift the membrane resting potential of nearby neurons, increasing the neural excitability of the stimulated area (excitatory effects). Cathodal stimulation (with the cathode positioned over the targeted region) lowers the membrane potential away from the firing threshold, reducing regional excitability (inhibitory effects) (Sanches et al., 2019).
Several studies have demonstrated the effects of NIBS techniques in patients with post-stroke aphasia and ischemic lesions sited in the left hemisphere. Specifically, tDCS has been applied to improve functional recovery in aphasic stroke patients, through the promotion of neural plasticity. Language-related networks, which may be modulated using NIBS, have nodes in both hemispheres, because transcallosal connections (Park et al., 2008; Kaplan et al., 2010) connect the left and right homotopic areas, according to the notion of inter-hemispheric communication (Ferbert et al., 1992). Non-invasive brain stimulation has been used to treat left hemisphere stroke aphasia in three ways (Fregni and Pascual-Leone, 2007; Hamilton et al., 2011): (A) excitatory stimulation (anodal tDCS) on left hemisphere language areas, to activate perilesional regions and reactivate language processes; (B) inhibitory stimulation (cathodal tDCS) on right hemisphere, to reduce trans-callosal inhibition; (C) excitatory stimulation (anodal tDCS) on right hemisphere, to activate potential language networks sited there. Recent evidence suggests that tDCS approaches should be chosen depending on the structural reserve of the injured hemisphere. If such reserve is inadequate, the unaffected hemisphere will try to compensate for the functional deficit, and it should need support (stimulating tDCS). If, on the other hand, the stroke hemisphere has a sufficient amount of structural reserve, the unaffected hemisphere would exert a disproportionate inhibition on the contralateral, and should be suppressed to promote recovery [bimodal balance recovery model; for a comprehensive review (see Di Pino et al., 2014; Di Pino and Di Lazzaro, 2020)].
The anodal tDCS on left hemisphere and the cathodal tDCS on right hemisphere proved to be more promising in determining clinical outcomes on language disabilities (Monti et al., 2008; Baker et al., 2010; Fiori et al., 2011). Researchers observed transient beneficial effects with single tDCS-session, as well as longer-lasting effects (>6 months) with periodic stimulations, which most likely promoted neuroplasticity (Naeser et al., 2005, 2011).
Regarding svPPA patients, two studies were conducted on anodal stimulation of the left inferior frontal lobe, with the anode placed on F7 (according to the international EEG 10–20 system) and the cathode on the right cheek (Ficek et al., 2018; Tsapkini et al., 2018), and two studies carried out the excitatory tDCS of the left temporoparietal region, with the anode placed on P3 (according to the international EEG 10–20 system) and cathode centered on forehead (Hung et al., 2017) or sited on the right fronto-orbital area (Roncero et al., 2017).
Teichmann et al. (2016) investigated the impact of more than one tDCS modality in svPPA patients. For this reason, their protocol was designed to compare the anodal excitatory stimulation of left ATL with the cathodal inhibition of right ATL, finding no differences in the primary endpoint. The main limitation of that study is that it failed to take into account an intrinsic feature of the semantic variant of PPA, namely that 30% of patients might have predominant right-ATL involvement (Hodges et al., 2010; Kumfor et al., 2016), that might exacerbate symptoms with a right-inhibitory tDCS.
4.2. Stimulation sites
Table 4 provides a brief overview of the hypothetical objectives linked to each tDCS setup employed in the reviewed studies. Figure 1 shows the different tDCS configurations.
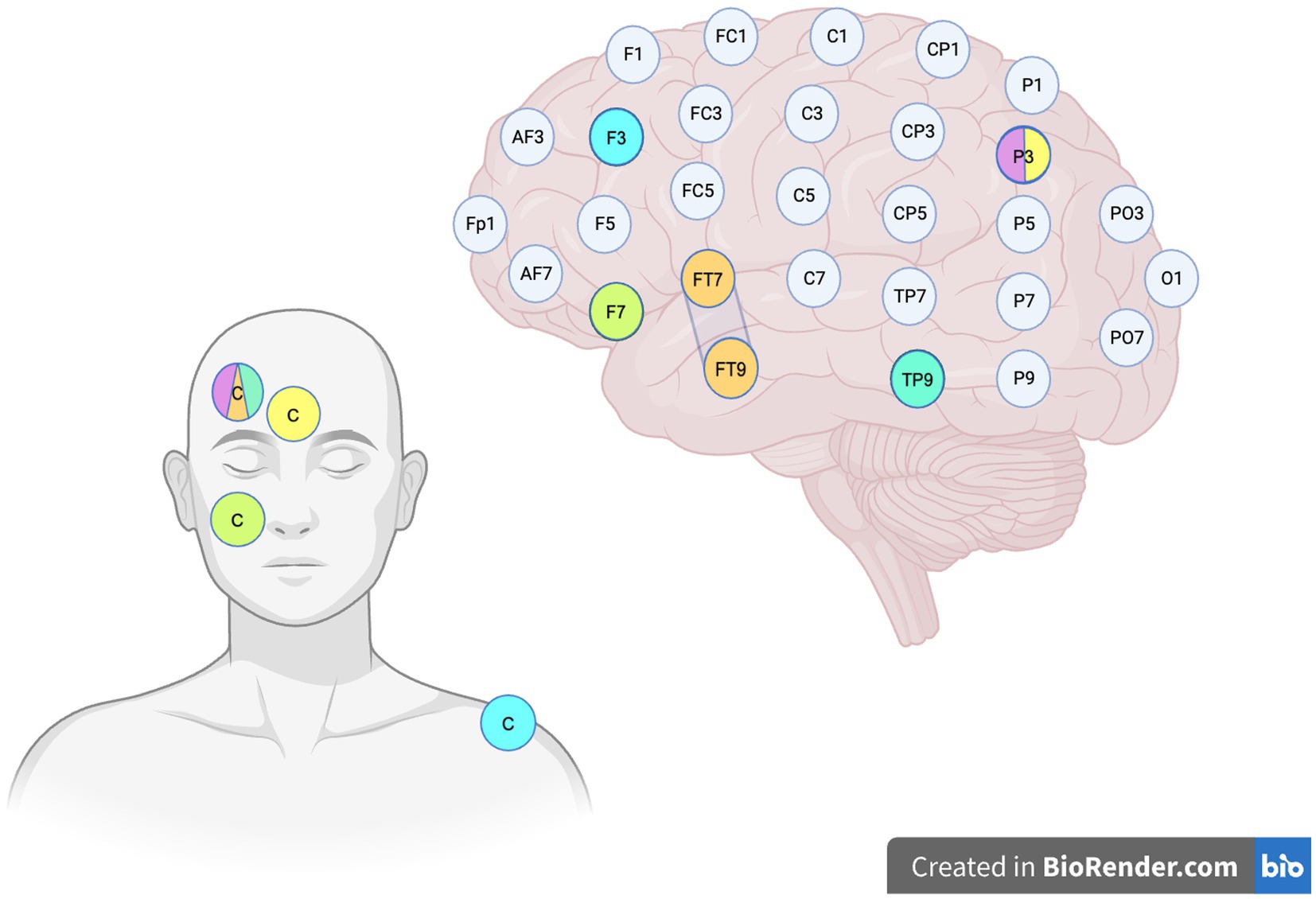
Figure 1. Stimulation sites. The image illustrates the various tDCS configurations employed in the reviewed studies, based on the EEG International 10-10 system. The color legend is set as follows: Orange: Teichmann et al. (2016) left anodal configuration, where the anode is placed over the left temporal pole and the cathode (C) is positioned on the contralateral supraorbital region. The second condition, which is not depicted in the figure, is designed with cathode placed over the right FT8–FT10 and the anode on the left supraorbital region. Green: configuration employed by Tsapkini et al. (2018) and Ficek et al. (2018), with the anode over the left inferior frontal lobe and the cathode (C) on the right cheek. Yellow: tDCS montage used by Hung et al. (2017), with the anode positioned over the left parieto-temporal region and the cathode (C) centered on the forehead. Lavender: configuration utilized by Roncero et al. (2017), with the anode over the left parieto-temporal region and the cathode placed on the right fronto-orbital region. Aquamarine: Roncero et al. (2019) inferior parieto-temporal anodal stimulation, with the anode positioned over TP9 and the cathode on the right fronto-orbital region. Cyan: Roncero et al. (2019) DLPFC stimulation, with the anode over F3 and the cathode sited over the right deltoid muscle.
One of the Teichmann et al. (2016) study’s strengths is that it targeted the left or right temporal pole, which is the more clearly involved area in svPPA/SD pathogenesis (Gorno-Tempini et al., 2011; Mesulam et al., 2014). However, we have to acknowledge that PPA is often diagnosed at late stage, where cortical atrophy due to aging might impair the effectiveness of neuromodulatory techniques.
By contrast, both Hung et al. (2017) and Roncero et al. (2017) trials aimed to stimulate the left inferior temporoparietal region, that has been previously described as a critical hub region for semantic control processing, particularly for naming (Koenigs et al., 2009; Price et al., 2016; Reilly et al., 2016).
In their paper, Roncero et al. (2019) employed two distinct active tDCS configurations, one targeting the inferior left parietotemporal area (TP9) and the other stimulating the left DLPFC. Recent research has identified the white matter of the left parietotemporal region, which encompasses the superior temporal gyrus, the angular gyrus, and parietal areas, as an additional network that plays a significant role in observed fact retrieval and arithmetic fact retrieval (Smaczny et al., 2023). In NIBS clinical trials on patients with mild cognitive impairment or dementia due to Alzheimer’s disease, the left DLPFC is frequently selected as the cortical target due to its crucial role in cognitive functions such as attention, executive functions, and working memory (Lambon Ralph, 2014; Lara and Wallis, 2015).
Finally, both Tsapkini et al. (2018) and Ficek et al. (2018) targeted the left IFG (inferior frontal gyrus), one of the main language hubs, involved in the writing ability, the orthographic long-term memory, and the phoneme-to-grapheme conversion (Purcell et al., 2011; Rapp and Dufor, 2011; Planton et al., 2013; DeMarco et al., 2017). The IFG is involved in all-variant PPA: in non-fluent/agrammatic variant, the left IFG is the primary site of cortical atrophy; in logopenic variant, atrophy is especially noticeable in the left supramarginal and angular gyri, which the IFG connects via the dorsal language stream (Hickok and Poeppel, 2004), the superior longitudinal fasciculus III, or the arcuate fasciculus (Catani et al., 2003); in semantic variant, the ATL is the major atrophy center, connected to the IFG through the ventral language stream, which includes the uncinate fasciculus (Hickok and Poeppel, 2004).
Furthermore, it is worth pointing out that in the studies conducted by Teichmann et al. (2016) and Roncero et al. (2019), the placement of electrodes was determined based on a brain MRI of the subjects, allowing for more precise estimation of the targeted coordinates.
4.3. TDCS intensity
As shown in Table 3, the reviewed studies used a defined current intensity of tDCS, but this differed between protocols.
While the majority used an arbitrary current intensity of 2 mA, Hung et al. (2017) used 1.5 mA (again an arbitrarily chosen value).
On the other hand, Teichmann et al. (2016) used 1.59 mA, calculated starting from a current density of 0.06 mA/cm2, as previously used in post-stroke aphasia or PPA studies with larger leads (Monti et al., 2008; Tsapkini et al., 2014). The purpose of this adjustment is to apply the same current intensity regardless of electrode shape and size.
4.4. Behavioral outcome measures
Regarding the discrepancies in the cognitive outcome chosen to measure changes in semantic tasks such as naming and matching, it is evident that the only study that has specifically addressed svPPA patients is that of Teichmann et al. (2016), with its “find the intruder”-type tasks. It consisted of a test based on the famous Pyramid and Palm Trees Test (Howard and Patterson, 1992), and participants had to select which of the two given items was related to the test item as accurately and promptly as possible. The items might be written words or pictures of either live or non-living beings.
The studies examining patients with various variants of progressive aphasia opted to evaluate production tasks, such as naming/writing accuracy. More specifically, Hung et al. (2017) and Roncero et al. (2017, 2019) used the naming of trained and untrained nouns (i.e., a verbal task), while both Tsapkini et al. (2018) and Ficek et al. (2018) measured letter accuracy of treated and untreated written words as the main outcome measure of their study. These outcomes were adopted because oral or written spelling accuracy is compromised in all degenerative forms of language impairment, even if it is not exclusive of svPPA patients.
4.5. Strengths and limitations of the present study
The main, implicit advantage of studying svPPA is the opportunity to observe a progressive aphasia that affects a well-defined brain region (the ATL). It follows that it might pave the way for the development of an experimental model of degenerative aphasias and their NIBS-based treatment.
However, as previously detailed, there are major differences among trials, regarding the study population (including different forms of dementia often without a biological diagnosis), time from disease onset (or rather, the lack of information on the progression of the disease for each patient), tDCS setups and intensities, stimulation sites, and outcome measures.
This methodological heterogeneity hinders the ability to draw definitive conclusions on the efficacy of tDCS-based treatments in svPPA patients.
Another critical limitation of this study is the significant impact of small sample sizes of svPPA patients on the cited results. Furthermore, the majority of these studies either do not specifically target svPPA patients or lack treatments tailored to the brain areas primarily affected by this disease. The insufficient sample size reduces the likelihood of obtaining statistically significant results with adequate statistical power, leading to an increased risk of false negatives and reduced result reliability. Considering the aforementioned methodological heterogeneity, this review cannot overcome the bias of inadequate sample size through meta-analysis. As a result, the conclusions that can be drawn from this study are considerably restricted.
5. Conclusion and future directions
With the exception of a single trial (Tsapkini et al., 2018) and major concerns about the methodological heterogeneity of the included papers, all of the findings of the selected studies suggest the improvement of the semantic performance of patients with svPPA following non-invasive electrical stimulation.
If the goal is to develop the most effective non-invasive treatment tailored to each patient, the aforementioned concerns need to be taken into account. To determine the most optimal tDCS arrangement, appropriate cognitive tests and neurophysiological evaluations (e.g., quantitative electroencephalography, QEEG) (Livint Popa et al., 2020) would be useful tools to identify the involved networks and differentiate right from left SD patients with high specificity. Furthermore, obtaining current intensity values from a safe and effective predefined value of current density, in combination with the selection of an appropriate stimulation site and a homogenous outcome variable, would allow for experiment standardization across centers despite the use of different equipment.
Because svPPA is a very rare disease, with huge recruitment difficulties and the added challenge of being able to intervene with NIBS only in the mild stage of the disease, most authors included svPPA patients in tDCS-studies involving subjects with other forms of frontotemporal lobar degeneration clinical syndromes and even patients with Alzheimer’s disease. Multi-center trials (or, at the at least, standardizing monocentric studies) could represent a reasonable next strategy. These would allow researchers to remain focused on a single nosological entity. A greater specificity may represent a promising way to achieve more significant and reproducible results that may be integrated in future guidelines.
Data availability statement
The original contributions presented in the study are included in the article/Supplementary material, further inquiries can be directed to the corresponding author.
Author contributions
FM, FC, and DN: conceptualization. FC and FM: methodology. DN: software, investigation, resources, data curation, visualization, and project administration. DN and SL: writing—original draft preparation. FC, FP, AM, and FM: writing—review and editing. VL, FP, FC, and SL: supervision. All authors contributed to the article and approved the submitted version.
Conflict of interest
The authors declare that the research was conducted in the absence of any commercial or financial relationships that could be construed as a potential conflict of interest.
Publisher’s note
All claims expressed in this article are solely those of the authors and do not necessarily represent those of their affiliated organizations, or those of the publisher, the editors and the reviewers. Any product that may be evaluated in this article, or claim that may be made by its manufacturer, is not guaranteed or endorsed by the publisher.
Supplementary material
The Supplementary material for this article can be found online at: https://www.frontiersin.org/articles/10.3389/fnhum.2023.1219737/full#supplementary-material
References
Adlam, A.-L. R., Patterson, K., Rogers, T. T., Nestor, P. J., Salmond, C. H., Acosta-Cabronero, J., et al. (2006). Semantic dementia and fluent primary progressive aphasia: two sides of the same coin? Brain J. Neurol. 129, 3066–3080. doi: 10.1093/brain/awl285
Baker, J. M., Rorden, C., and Fridriksson, J. (2010). Using transcranial direct-current stimulation to treat stroke patients with aphasia. Stroke 41, 1229–1236. doi: 10.1161/STROKEAHA.109.576785
Boxer, A. L., Knopman, D. S., Kaufer, D. I., Grossman, M., Onyike, C., Graf-Radford, N., et al. (2013). Memantine in patients with frontotemporal lobar degeneration: a multicentre, randomised, double-blind, placebo-controlled trial. Lancet Neurol. 12, 149–156. doi: 10.1016/S1474-4422(12)70320-4
Bozeat, S., Lambon Ralph, M. A., Patterson, K., Garrard, P., and Hodges, J. R. (2000). Non-verbal semantic impairment in semantic dementia. Neuropsychologia 38, 1207–1215. doi: 10.1016/s0028-3932(00)00034-8
Catani, M., Piccirilli, M., Cherubini, A., Tarducci, R., Sciarma, T., Gobbi, G., et al. (2003). Axonal injury within language network in primary progressive aphasia. Ann. Neurol. 53, 242–247. doi: 10.1002/ana.10445
Corbett, F., Jefferies, E., Ehsan, S., and Lambon Ralph, M. A. (2009). Different impairments of semantic cognition in semantic dementia and semantic aphasia: evidence from the non-verbal domain. Brain J. Neurol. 132, 2593–2608. doi: 10.1093/brain/awp146
Davies, R. R., Hodges, J. R., Kril, J. J., Patterson, K., Halliday, G. M., and Xuereb, J. H. (2005). The pathological basis of semantic dementia. Brain J. Neurol. 128, 1984–1995. doi: 10.1093/brain/awh582
Deakin, J. B., Rahman, S., Nestor, P. J., Hodges, J. R., and Sahakian, B. J. (2004). Paroxetine does not improve symptoms and impairs cognition in frontotemporal dementia: a double-blind randomized controlled trial. Psychopharmacology 172, 400–408. doi: 10.1007/s00213-003-1686-5
DeMarco, A. T., Wilson, S. M., Rising, K., Rapcsak, S. Z., and Beeson, P. M. (2017). Neural substrates of sublexical processing for spelling. Brain Lang. 164, 118–128. doi: 10.1016/j.bandl.2016.10.001
Di Lazzaro, V., Bella, R., Benussi, A., Bologna, M., Borroni, B., Capone, F., et al. (2021). Diagnostic contribution and therapeutic perspectives of transcranial magnetic stimulation in dementia. Clin. Neurophysiol. 132, 2568–2607. doi: 10.1016/j.clinph.2021.05.035
Di Pino, G., and Di Lazzaro, V. (2020). The balance recovery bimodal model in stroke patients between evidence and speculation: do recent studies support it? Clin. Neurophysiol. 131, 2488–2490. doi: 10.1016/j.clinph.2020.07.004
Di Pino, G., Pellegrino, G., Assenza, G., Capone, F., Ferreri, F., Formica, D., et al. (2014). Modulation of brain plasticity in stroke: a novel model for neurorehabilitation. Nat. Rev. Neurol. 10, 597–608. doi: 10.1038/nrneurol.2014.162
Elder, G. J., and Taylor, J.-P. (2014). Transcranial magnetic stimulation and transcranial direct current stimulation: treatments for cognitive and neuropsychiatric symptoms in the neurodegenerative dementias? Alzheimers Res. Ther. 6:74. doi: 10.1186/s13195-014-0074-1
Ferbert, A., Priori, A., Rothwell, J. C., Day, B. L., Colebatch, J. G., and Marsden, C. D. (1992). Interhemispheric inhibition of the human motor cortex. J. Physiol. 453, 525–546. doi: 10.1113/jphysiol.1992.sp019243
Ficek, B. N., Wang, Z., Zhao, Y., Webster, K. T., Desmond, J. E., Hillis, A. E., et al. (2018). The effect of tDCS on functional connectivity in primary progressive aphasia. NeuroImage Clin. 19, 703–715. doi: 10.1016/j.nicl.2018.05.023
Fiori, V., Coccia, M., Marinelli, C. V., Vecchi, V., Bonifazi, S., Ceravolo, M. G., et al. (2011). Transcranial direct current stimulation improves word retrieval in healthy and nonfluent aphasic subjects. J. Cogn. Neurosci. 23, 2309–2323. doi: 10.1162/jocn.2010.21579
Fregni, F., and Pascual-Leone, A. (2007). Technology insight: noninvasive brain stimulation in neurology-perspectives on the therapeutic potential of rTMS and tDCS. Nat. Clin. Pract. Neurol. 3, 383–393. doi: 10.1038/ncpneuro0530
Garrard, P., and Carroll, E. (2006). Lost in semantic space: a multi-modal, non-verbal assessment of feature knowledge in semantic dementia. Brain J. Neurol. 129, 1152–1163. doi: 10.1093/brain/awl069
Good, C. D., Scahill, R. I., Fox, N. C., Ashburner, J., Friston, K. J., Chan, D., et al. (2002). Automatic differentiation of anatomical patterns in the human brain: validation with studies of degenerative dementias. NeuroImage 17, 29–46. doi: 10.1006/nimg.2002.1202
Gorno-Tempini, M. L., Hillis, A. E., Weintraub, S., Kertesz, A., Mendez, M., Cappa, S. F., et al. (2011). Classification of primary progressive aphasia and its variants. Neurology 76, 1006–1014. doi: 10.1212/WNL.0b013e31821103e6
Guo, C. C., Gorno-Tempini, M. L., Gesierich, B., Henry, M., Trujillo, A., Shany-Ur, T., et al. (2013). Anterior temporal lobe degeneration produces widespread network-driven dysfunction. Brain J. Neurol. 136, 2979–2991. doi: 10.1093/brain/awt222
Hamilton, R. H., Chrysikou, E. G., and Coslett, B. (2011). Mechanisms of aphasia recovery after stroke and the role of noninvasive brain stimulation. Brain Lang. 118, 40–50. doi: 10.1016/j.bandl.2011.02.005
Hickok, G., and Poeppel, D. (2004). Dorsal and ventral streams: a framework for understanding aspects of the functional anatomy of language. Cognition 92, 67–99. doi: 10.1016/j.cognition.2003.10.011
Hodges, J. R., Mitchell, J., Dawson, K., Spillantini, M. G., Xuereb, J. H., McMonagle, P., et al. (2010). Semantic dementia: demography, familial factors and survival in a consecutive series of 100 cases. Brain J. Neurol. 133, 300–306. doi: 10.1093/brain/awp248
Hodges, J. R., and Patterson, K. (2007). Semantic dementia: a unique clinicopathological syndrome. Lancet Neurol. 6, 1004–1014. doi: 10.1016/S1474-4422(07)70266-1
Hodges, J. R., Patterson, K., Oxbury, S., and Funnell, E. (1992). Semantic dementia. Progressive fluent aphasia with temporal lobe atrophy. Brain J. Neurol. 115, 1783–1806. doi: 10.1093/brain/115.6.1783
Howard, D., and Patterson, K. E. The pyramids and palm trees test a test of semantic access from words and pictures. Bury St Edmunds. Thames Valley Test Company (1992).
Hung, J., Bauer, A., Grossman, M., Hamilton, R. H., Coslett, H. B., and Reilly, J. (2017). Semantic feature training in combination with transcranial direct current stimulation (tDCS) for progressive anomia. Front. Hum. Neurosci. 11:253. doi: 10.3389/fnhum.2017.00253
Kaplan, E., Naeser, M. A., Martin, P. I., Ho, M., Wang, Y., Baker, E., et al. (2010). Horizontal portion of arcuate fasciculus fibers track to pars opercularis, not pars triangularis, in right and left hemispheres: a DTI study. NeuroImage 52, 436–444. doi: 10.1016/j.neuroimage.2010.04.247
Koenigs, M., Barbey, A. K., Postle, B. R., and Grafman, J. (2009). Superior parietal cortex is critical for the manipulation of information in working memory. J. Neurosci. 29, 14980–14986. doi: 10.1523/JNEUROSCI.3706-09.2009
Kumfor, F., Landin-Romero, R., Devenney, E., Hutchings, R., Grasso, R., Hodges, J. R., et al. (2016). On the right side? A longitudinal study of left- versus right-lateralized semantic dementia. Brain J. Neurol. 139, 986–998. doi: 10.1093/brain/awv387
La Joie, R., Landeau, B., Perrotin, A., Bejanin, A., Egret, S., Pélerin, A., et al. (2014). Intrinsic connectivity identifies the hippocampus as a main crossroad between Alzheimer’s and semantic dementia-targeted networks. Neuron 81, 1417–1428. doi: 10.1016/j.neuron.2014.01.026
Lambon Ralph, M. A. (2014). Neurocognitive insights on conceptual knowledge and its breakdown. Philos. Trans. R. Soc. B 369:20120392. doi: 10.1098/rstb.2012.0392
Landin-Romero, R., Tan, R., Hodges, J. R., and Kumfor, F. (2016). An update on semantic dementia: genetics, imaging, and pathology. Alzheimers Res. Ther. 8:52. doi: 10.1186/s13195-016-0219-5
Lara, A. H., and Wallis, J. D. (2015). The role of prefrontal cortex in working memory: a mini review. Front. Syst. Neurosci. 9:173. doi: 10.3389/fnsys.2015.00173
Lefaucheur, J.-P., Antal, A., Ayache, S. S., Benninger, D. H., Brunelin, J., Cogiamanian, F., et al. (2017). Evidence-based guidelines on the therapeutic use of transcranial direct current stimulation (tDCS). Clin. Neurophysiol. 128, 56–92. doi: 10.1016/j.clinph.2016.10.087
Livint Popa, L., Dragos, H., Pantelemon, C., Verisezan Rosu, O., and Strilciuc, S. (2020). The role of quantitative EEG in the diagnosis of neuropsychiatric disorders. J. Med. Life 13, 8–15. doi: 10.25122/jml-2019-0085
Luzzi, S., Baldinelli, S., Ranaldi, V., Fabi, K., Cafazzo, V., Fringuelli, F., et al. (2017). Famous faces and voices: differential profiles in early right and left semantic dementia and in Alzheimer’s disease. Neuropsychologia 94, 118–128. doi: 10.1016/j.neuropsychologia.2016.11.020
Luzzi, S., Cafazzo, V., Silvestrini, M., and Provinciali, L. (2013). Arithmetic knowledge in early semantic dementia. Neurol. Sci. 34, 1613–1619. doi: 10.1007/s10072-013-1294-z
McMillan, C., Gee, J., Moore, P., Dennis, K., DeVita, C., and Grossman, M. (2004). Confrontation naming and morphometric analyses of structural MRI in frontotemporal dementia. Dement. Geriatr. Cogn. Disord. 17, 320–323. doi: 10.1159/000077163
Mendez, M. F. (2019). Early-onset Alzheimer disease and its variants. Continuum 25, 34–51. doi: 10.1212/CON.0000000000000687
Mesulam, M. M. (1987). Primary progressive aphasia—differentiation from Alzheimer’s disease. Ann. Neurol. 22, 533–534. doi: 10.1002/ana.410220414
Mesulam, M.-M. (2003). Primary progressive aphasia—a language-based dementia. N. Engl. J. Med. 349, 1535–1542. doi: 10.1056/NEJMra022435
Mesulam, M.-M., Rogalski, E. J., Wieneke, C., Hurley, R. S., Geula, C., Bigio, E. H., et al. (2014). Primary progressive aphasia and the evolving neurology of the language network. Nat. Rev. Neurol. 10, 554–569. doi: 10.1038/nrneurol.2014.159
Moher, D., Liberati, A., Tetzlaff, J., and Altman, D. G., PRISMA Group (2009). Preferred reporting items for systematic reviews and meta-analyses: the PRISMA statement. Ann. Intern. Med. 151:W64. doi: 10.7326/0003-4819-151-4-200908180-00135
Monti, A., Cogiamanian, F., Marceglia, S., Ferrucci, R., Mameli, F., Mrakic-Sposta, S., et al. (2008). Improved naming after transcranial direct current stimulation in aphasia. J. Neurol. Neurosurg. Psychiatry 79, 451–453. doi: 10.1136/jnnp.2007.135277
Monti, A., Ferrucci, R., Fumagalli, M., Mameli, F., Cogiamanian, F., Ardolino, G., et al. (2013). Transcranial direct current stimulation (tDCS) and language. J. Neurol. Neurosurg. Psychiatry 84, 832–842. doi: 10.1136/jnnp-2012-302825
Mummery, C. J., Patterson, K., Price, C. J., Ashburner, J., Frackowiak, R. S., and Hodges, J. R. (2000). A voxel-based morphometry study of semantic dementia: relationship between temporal lobe atrophy and semantic memory. Ann. Neurol. 47, 36–45. doi: 10.1002/1531-8249(200001)47:1<36::AID-ANA8>3.0.CO;2-L
Naeser, M. A., Martin, P. I., Nicholas, M., Baker, E. H., Seekins, H., Kobayashi, M., et al. (2005). Improved picture naming in chronic aphasia after TMS to part of right Broca’s area: an open-protocol study. Brain Lang. 93, 95–105. doi: 10.1016/j.bandl.2004.08.004
Naeser, M. A., Martin, P. I., Theoret, H., Kobayashi, M., Fregni, F., Nicholas, M., et al. (2011). TMS suppression of right pars triangularis, but not pars opercularis, improves naming in aphasia. Brain Lang. 119, 206–213. doi: 10.1016/j.bandl.2011.07.005
Onyike, C. U., and Diehl-Schmid, J. (2013). The epidemiology of frontotemporal dementia. Int. Rev. Psychiatry 25, 130–137. doi: 10.3109/09540261.2013.776523
Park, H.-J., Kim, J. J., Lee, S.-K., Seok, J. H., Chun, J., Kim, D. I., et al. (2008). Corpus callosal connection mapping using cortical gray matter parcellation and DT-MRI. Hum. Brain Mapp. 29, 503–516. doi: 10.1002/hbm.20314
Pick, A. (1892). Uber die Beziehungen der senilen Hirnatrophie zur Aphasie. Prag. Med. Wochenschr. 17, 165–167.
Planton, S., Jucla, M., Roux, F.-E., and Démonet, J.-F. (2013). The “handwriting brain”: a meta-analysis of neuroimaging studies of motor versus orthographic processes. Cortex 49, 2772–2787. doi: 10.1016/j.cortex.2013.05.011
Price, A. R., Peelle, J. E., Bonner, M. F., Grossman, M., and Hamilton, R. H. (2016). Causal evidence for a mechanism of semantic integration in the angular gyrus as revealed by high-definition transcranial direct current stimulation. J. Neurosci. 36, 3829–3838. doi: 10.1523/JNEUROSCI.3120-15.2016
Purcell, J. J., Turkeltaub, P. E., Eden, G. F., and Rapp, B. (2011). Examining the central and peripheral processes of written word production through meta-analysis. Front. Psychol. 2:239. doi: 10.3389/fpsyg.2011.00239
Rapp, B., and Dufor, O. (2011). The neurotopography of written word production: an fMRI investigation of the distribution of sensitivity to length and frequency. J. Cogn. Neurosci. 23, 4067–4081. doi: 10.1162/jocn_a_00109
Reilly, J., Peelle, J. E., Garcia, A., and Crutch, S. J. (2016). Linking somatic and symbolic representation in semantic memory: the dynamic multilevel reactivation framework. Psychon. Bull. Rev. 23, 1002–1014. doi: 10.3758/s13423-015-0824-5
Rohrer, J. D., Ridgway, G. R., Crutch, S. J., Hailstone, J., Goll, J. C., Clarkson, M. J., et al. (2010). Progressive logopenic/phonological aphasia: erosion of the language network. NeuroImage 49, 984–993. doi: 10.1016/j.neuroimage.2009.08.002
Roncero, C., Kniefel, H., Service E, Thiel, A., Probst, S., and Chertkow, H. (2017). Inferior parietal transcranial direct current stimulation with training improves cognition in anomic Alzheimer’s disease and frontotemporal dementia. Alzheimers Dement. 3, 247–253. doi: 10.1016/j.trci.2017.03.003
Roncero, C., Service, E., De Caro, M., Popov, A., Thiel, A., Probst, S., et al. (2019). Maximizing the treatment benefit of tDCS in neurodegenerative anomia. Front. Neurosci. 13:1231. doi: 10.3389/fnins.2019.01231
Rosen, H. J., Gorno-Tempini, M. L., Goldman, W. P., Perry, R. J., Schuff, N., Weiner, M., et al. (2002). Patterns of brain atrophy in frontotemporal dementia and semantic dementia. Neurology 58, 198–208. doi: 10.1212/wnl.58.2.198
Sanches, C., Levy, R., Benisty, S., Volpe-Gillot, L., Habert, M.-O., Kas, A., et al. (2019). Testing the therapeutic effects of transcranial direct current stimulation (tDCS) in semantic dementia: a double blind, sham controlled, randomized clinical trial. Trials 20:632. doi: 10.1186/s13063-019-3613-z
Smaczny, S., Sperber, C., Jung, S., Moeller, K., Karnath, H. O., and Klein, E. (2023). Disconnection in a left-hemispheric temporo-parietal network impairs multiplication fact retrieval. NeuroImage 268:119840. doi: 10.1016/j.neuroimage.2022.119840
Snowden, J., Goulding, P. J., and David, N. (1989). Semantic dementia: a form of circumscribed cerebral atrophy. Behav. Neurol. 2, 167–182. doi: 10.1155/1989/124043
Snowden, J. S., Thompson, J. C., and Neary, D. (2004). Knowledge of famous faces and names in semantic dementia. Brain J. Neurol. 127, 860–872. doi: 10.1093/brain/awh099
Tan, R. H., Wong, S., Kril, J. J., Piguet, O., Hornberger, M., Hodges, J. R., et al. (2014). Beyond the temporal pole: limbic memory circuit in the semantic variant of primary progressive aphasia. Brain J. Neurol. 137, 2065–2076. doi: 10.1093/brain/awu118
Teichmann, M., Lesoil, C., Godard, J., Vernet, M., Bertrand, A., Levy, R., et al. (2016). Direct current stimulation over the anterior temporal areas boosts semantic processing in primary progressive aphasia. Ann. Neurol. 80, 693–707. doi: 10.1002/ana.24766
Tsapkini, K., Frangakis, C., Gomez, Y., Davis, C., and Hillis, A. E. (2014). Augmentation of spelling therapy with transcranial direct current stimulation in primary progressive aphasia: preliminary results and challenges. Aphasiology 28, 1112–1130. doi: 10.1080/02687038.2014.930410
Tsapkini, K., Webster, K. T., Ficek, B. N., Desmond, J. E., Onyike, C. U., Rapp, B., et al. (2018). Electrical brain stimulation in different variants of primary progressive aphasia: a randomized clinical trial. Alzheimers Dement. 4, 461–472. doi: 10.1016/j.trci.2018.08.002
Ulm, L., McMahon, K., Copland, D., de Zubicaray, G. I., and Meinzer, M. (2015). Neural mechanisms underlying perilesional transcranial direct current stimulation in aphasia: a feasibility study. Front. Hum. Neurosci. 9:550. doi: 10.3389/fnhum.2015.00550
Vercelletto, M., Boutoleau-Bretonnière, C., Volteau, C., Puel, M., Auriacombe, S., Sarazin, M., et al. (2011). Memantine in behavioral variant frontotemporal dementia: negative results. J. Alzheimers Dis. 23, 749–759. doi: 10.3233/JAD-2010-101632
Keywords: progressive aphasia, neurodegenerative disorders, dementia, semantic dementia, frontotemporal lobar degeneration, tDCS, non-invasive brain stimulation, neuromodulation
Citation: Norata D, Motolese F, Magliozzi A, Pilato F, Di Lazzaro V, Luzzi S and Capone F (2023) Transcranial direct current stimulation in semantic variant of primary progressive aphasia: a state-of-the-art review. Front. Hum. Neurosci. 17:1219737. doi: 10.3389/fnhum.2023.1219737
Edited by:
Jorge Leite, Portucalense University, PortugalReviewed by:
Grigorios Nasios, University of Ioannina, GreeceZeyi Wang, University of California, Berkeley, United States
Copyright © 2023 Norata, Motolese, Magliozzi, Pilato, Di Lazzaro, Luzzi and Capone. This is an open-access article distributed under the terms of the Creative Commons Attribution License (CC BY). The use, distribution or reproduction in other forums is permitted, provided the original author(s) and the copyright owner(s) are credited and that the original publication in this journal is cited, in accordance with accepted academic practice. No use, distribution or reproduction is permitted which does not comply with these terms.
*Correspondence: Davide Norata, dav.norata@gmail.com