- 1Department of Psychiatry, The Third People’s Hospital of Huai’an, Huaian, Jiangsu, China
- 2Department of Geriatric Psychiatry, The Affiliated Brain Hospital of Nanjing Medical University, Nanjing, China
- 3Department of Hepatology, The Second Hospital of Nanjing, Nanjing University of Chinese Medicine, Nanjing, China
- 4Department of Neurology, Affiliated Zhongda Hospital, School of Medicine Southeast University, Nanjing, China
- 5Institute of Neuropsychiatry, The Affiliated Brain Hospital of Nanjing Medical University, Nanjing, China
- 6The Affiliated Xuzhou Oriental Hospital of Xuzhou Medical University, Xuzhou, Jiangsu, China
Objective: To investigate brain structural and functional characteristics of three brain functional networks including default mode network (DMN), central executive network (CEN), and salience network (SN) in persistent negative symptoms (PNS) patients.
Methods: We performed an activation likelihood estimation (ALE) meta-analysis of functional connectivity (FC) studies and voxel-based morphometry (VBM) studies to detect specific structural and functional alterations of brain networks between PNS patients and healthy controls.
Results: Seventeen VBM studies and twenty FC studies were included. In the DMN, PNS patients showed decreased gray matter in the bilateral medial frontal gyrus and left anterior cingulate gyrus and a significant reduction of FC in the right precuneus. Also, PNS patients had a decrease of gray matter in the left inferior parietal lobules and medial frontal gyrus, and a significant reduction of FC in the bilateral superior frontal gyrus in the CEN. In comparison with healthy controls, PNS patients exhibited reduced gray matter in the bilateral insula, anterior cingulate gyrus, left precentral gyrus and right claustrum and lower FC in these brain areas in the SN, including the left insula, claustrum, inferior frontal gyrus and extra-nuclear.
Conclusion: This meta-analysis reveals brain structural and functional imaging alterations in the three networks and the interaction among these networks in PNS patients, which provides neuroscientific evidence for more personalized treatment.
Systematic Review RegistrationThe PROSPERO (https://www.crd.york.ac.uk/PROSPERO/, registration number: CRD42022335962).
1. Introduction
Negative symptoms are related to severe impairment in social function and have a negative impact on the treatment and prognosis of schizophrenia (Galderisi et al., 2018). Negative symptoms include blunted affect, anhedonia, alogia, avolition, and a sociality (Kirkpatrick et al., 2006; Buchanan, 2007). Negative symptoms recently have been mainly divided into the following two subtypes (Szendi et al., 2017). One is deficit syndrome, which refers to primary or idiopathic persistent negative symptoms. Another is the concept of Persistent negative symptoms (PNS), described as primary or secondary negative symptoms of moderate or worse severity lasting more than 6 months after the first episode of psychosis (Carpenter et al., 1988; Castellon et al., 1994), and demonstrating defined threshold levels of positive symptoms, depression, and extrapyramidal side effects during the stable phase of schizophrenia (Buchanan, 2007). The PNS is considered as a broader concept than deficit syndrome and is not specific for diagnosis and therefore more suitable for clinical trials. Previous studies have shown that the duration of untreated psychosis is associated with the development of PNS and can be considered a risk factor (Galderisi et al., 2013; González-Valderrama et al., 2017) and it clinically represents an unmet therapeutic need in many cases (Kirkpatrick et al., 2006). Therefore, effective diagnosis and appropriate intervention for PNS patients are essential.
Previous studies have revealed anomalous connectivity in several functional networks, including the default mode network (DMN) (Yang et al., 2019; Fan et al., 2020), central executive network (CEN) (Chen et al., 2016), and salience network (SN) (Huang et al., 2020), which have been considered as potential neural network foundation for psychopathology and abnormal cognition and emotion (Menon, 2011). The DMN includes the medial prefrontal cortex, posterior cingulate cortex, precuneus, medial and inferior temporal lobes, and bilateral inferior parietal lobules (IPL) (Buckner et al., 2008, 2011; Yeo et al., 2011). This network is usually active when participants are not working on any specific task and inactive during effortful cognitive tasks (Raichle et al., 2001; Greicius et al., 2009). It is also manages the monitoring associated with internal generative processes, including autobiographical memory recollection, self-monitoring, and internal and external cognition (Buckner et al., 2008). The CEN includes the superior frontal gyrus (SFG), dorsolateral prefrontal cortex and posterior parietal cortices, and is involved in goal-directed/externally oriented tasks and the working memory process. The SN, anchored on the insula and anterior cingulate gyrus (ACG), plays an important role in cognitive control by focusing on motivational salience stimuli and employing suitable functional brain-behavior networks to regulate actions (Menon, 2011; Peters et al., 2016). Exploring structural and functional changes within these three brain networks in patients with PNS will help provide a neuroimaging basis for designing targeted and effective interventions.
Recent advances in neuroimaging technology have accumulated substantial evidence regarding functional and structural alterations in these brain networks in schizophrenia. Voxel-based morphometry (VBM) has been broadly accepted to detect structural alterations in brain networks, and it can provide an unbiased method for estimating regional gray matter volume (GMV) (Ashburner and Friston, 2000). Independent component analysis and seed-based resting state functional connectivity (rs-FC) can reflect connectivity between different brain regions or networks (Cole et al., 2010). These two imaging techniques are better comparable in independent study settings and populations, and are not affected by task paradigms (Shehzad et al., 2009; Liu et al., 2020), so this study conducted a meta-analysis of structural (volumetric) and resting-state functional magnetic resonance imaging (rs-MRI) findings. Results of previous VBM studies showed reduced GMV within the DMN, CEN, and SN in schizophrenia (Honea et al., 2008; Fornito et al., 2009; Schuster et al., 2012; Kim et al., 2017). Meanwhile, the accumulated evidence has suggested that brain activity of the three networks is disrupted in schizophrenia, but the results are inconsistent (Chen et al., 2016; Hare et al., 2019; Yang et al., 2019; Fan et al., 2020; Huang et al., 2020). Few studies have been conducted on PNS patients and the findings are inconsistently arising from the use of different acquisition, processing, analysis techniques and different sample characteristics (Benoit et al., 2012; İnce and Üçok, 2018). It is obvious that more studies with larger sample sizes are needed in the future to investigate brain structural and functional alterations in PNS patients.
The main findings of VBM and FC studies were summarized in some systematic reviews and meta-analyses, most of which investigated a widespread tendency of reduced GMV and large-scale brain network connectivity in schizophrenia (Ellison-Wright et al., 2008; Pettersson-Yeo et al., 2011; Haijma et al., 2013; Kambeitz et al., 2016; Dong et al., 2018). Furthermore, several studies (Brady et al., 2019; Gao et al., 2020), and meta-analyses (Li et al., 2018; Chee et al., 2020) investigating the neuroanatomy and neurological relevance of psychiatric symptoms have shown that demonstrating that GMV and FC aberrations in schizophrenia are associated with negative symptoms severity. Several articles have revealed structural and functional alterations in these core networks in PNS patients (Benoit et al., 2012; Bodnar et al., 2014; İnce and Üçok, 2018). However, only one meta-analysis has found reduced GMV in the brain regions of the reward network, especially the left caudate nucleus in patients with PNS compared with HC (Li et al., 2018). Hence, it is necessary to conduct a meta-analysis of whole-brain VBM and FC studies to assess certain structural and functional changes in DMN, CEN and SN in PNS patients.
2. Methods
2.1. Literature search
The meta-analysis was preregistered on the PROSPERO (https://www.crd.york.ac.uk/PROSPERO/, registration number: CRD42022335962) and performed in accordance with the PRISMA statement (Moher et al., 2009). Structural and functional imaging studies related to DMN, SN and CEN were retrieved from the PubMed, Web of Science and EMBASE databases up to July 2023. The search keywords were “schizophrenia” and “default mode network/central executive network/salience network” and “gray matter” and “voxel-based morphometry”; “schizophrenia” and “default mode network/central executive network/salience network” and “functional connectivity” and “Functional Magnetic Resonance Imaging/functional MRI/fMRI.” Figure 1 shows the selection procedure for the inclusion of empirical studies.
2.2. Eligible criteria
The studies included in this meta-analysis were required to meet the following requirements: (1) Negative symptoms of patients must be at least mild or moderate in severity, and assessed by a proven negative symptom scale such as the Positive and Negative Syndrome Scale (PANSS), Scale for the Assessment of Negative Symptoms (SANS), Negative Symptoms Assessment, and Brief Negative Symptom Scale (Kay et al., 1987; Andreasen, 1989; Kirkpatrick et al., 2011). (2) The duration of illness is at least 6 months. (3) The included studies need to use the whole-brain analysis method (VBM or FC) to investigate the brain imaging differences between schizophrenia and healthy subjects and use the Montreal Neurologic Institute (MNI) or Talairach system to report the results. (4) All studies compared PNS patients with healthy controls (HCs), and we adopted the following exclusion criteria formulated by Li et al. (2018) to identify the relevant studies: (1) The patient’s mean PANSS negative score was less than 19, and the mean PANSS positive subscale score was greater than 21. (2) The patient’s mean SANS total score was less than 20, and the mean Scale for the Assessment of Positive Symptoms total score was greater than 20. (3) The patient’s mean positive symptom scores was greater than negative symptom scores.
2.3. Data extraction and quality assessment
All data were extracted separately by two authors, with a third author resolving any inconsistencies before the final analysis. First, we chose DMN, CEN, and SN related articles. Second, all aberrant brain areas retrieved from the articles were grouped as one of three brain networks (DMN, CEN, or SN). Third, we extracted the coordinates that we needed from whole-brain VBM/FC studies. Finally, we extracted the general characteristics of each study, including the first author, year of publication, sample size, sex of subjects, illness duration, and assessment scale were extracted as the basic data. We used a 12-point checklist to assess the methodological quality of the included studies (Brambilla et al., 2003), and case studies with scores above 6.0 were included in our meta-analysis.
2.4. Activation likelihood estimation
We used Ginger activation likelihood estimation (ALE) version 2.3.61 to run the ALE algorithm and perform a voxel-based meta-analysis of neuroimaging data (Eickhoff et al., 2017). Structural and functional studies were analyzed separately, and we extracted coordinates of increased FC in schizophrenia compared with healthy subjects and decreased FC in schizophrenia compared with healthy subjects in the included study for meta-analysis, and divided the former into FC increasing group and the latter into FC decreasing group. First, we turned the activation coordinates reported in the study in Talairach space into MNI space. Then, we collected the coordinates of these three specific networks containing brain regions and followed the software guide to obtain the final cluster. The present study performed family-wise error (FWE) correction at p < 0.05 for cluster-level inference, and threshold at voxel level p < 0.01 for cluster-forming, with 1,000 permutations. Lastly, we present the ALE maps through the DPABI software (Yan et al., 2016).2
3. Results
3.1. Search results
According to the search formula, 2,832 VBM articles and 4,405 FC articles were retrieved from the three databases, of which 559 and 2,466 duplicate articles were retrieved, respectively. After rigorous screening, a total of 17 VBM studies and 20 FC studies were selected. More detailed information on each of the included studies is shown in Tables 1, 2. For the VBM-meta-analysis, we identified 15 experiments with the DMN (Paillère-Martinot et al., 2001; Sigmundsson et al., 2001; Salgado-Pineda et al., 2004; Jayakumar et al., 2005; Bassitt et al., 2007; Koutsouleris et al., 2008; Meisenzahl et al., 2008; Herold et al., 2009; Whitford et al., 2009; Huang et al., 2015; Poletti et al., 2016; Kuroki et al., 2017; Szendi et al., 2017; Spalthoff et al., 2018; Neugebauer et al., 2019), 5 experiments with the CEN (Jayakumar et al., 2005; Bassitt et al., 2007; Koutsouleris et al., 2008; Meisenzahl et al., 2008; Herold et al., 2009), and 15 experiments with the SN (Paillère-Martinot et al., 2001; Sigmundsson et al., 2001; Salgado-Pineda et al., 2004; Jayakumar et al., 2005; Bassitt et al., 2007; Koutsouleris et al., 2008; Meisenzahl et al., 2008; Herold et al., 2009; Anderson et al., 2015; Poletti et al., 2016; Kim et al., 2017; Kuroki et al., 2017; Szendi et al., 2017; Spalthoff et al., 2018; Neugebauer et al., 2019). For the FC-meta-analysis, we identified 17 experiments with the DMN (Bluhm et al., 2007; Wolf et al., 2011; Fan et al., 2013; Chang et al., 2014; Manoliu et al., 2014; Zhuo et al., 2014, 2017; Alonso-Solís et al., 2015; Xu et al., 2015; Zhou et al., 2015; Chen et al., 2016; Penner et al., 2016, 2018a,b; Peters et al., 2017; Sharma et al., 2018; Dong et al., 2019), 7 experiments with the CEN (Chang et al., 2014; Manoliu et al., 2014; Zhuo et al., 2014; Penner et al., 2016, 2018a,b; Peters et al., 2017), and 10 experiments with the SN (Wolf et al., 2011; Manoliu et al., 2014; Wang et al., 2015; Xu et al., 2015; Zhou et al., 2015; Chen et al., 2016; Penner et al., 2016, 2018a,b; Peters et al., 2017) reporting decreased FC in the PNS group relative to the HC group. In addition, 6 experiments with the DMN (Wolf et al., 2011; Chang et al., 2014; Alonso-Solís et al., 2015; Wang et al., 2015, 2016; Liu et al., 2018), 3 experiments with the CEN (Wolf et al., 2011; Manoliu et al., 2014; Penner et al., 2018a), and 2 experiments with the SN (Manoliu et al., 2014; Chen et al., 2016) reported that FC was increased in the PNS patients. The quality assessment of the included studies ranged from low scores of 10.5 and moderate scores of 11–11.5 to high scores of 12. The result of the quality assessment and the areas involved in core brain networks in each study are detailed in Supplementary material.
3.2. Meta-analysis results
In the DMN, PNS patients showed decreased gray matter in the bilateral medial frontal gyrus (MFG) and left ACG and a significant reduction of FC in the right precuneus (Table 3 and Figure 2). Also, PNS patients had a decrease of gray matter in the left IPL and MFG and a significant reduction of FC in the bilateral SFG in the CEN (Table 3 and Figure 3). In comparison with HCs, PNS patients exhibited reduced gray matter in the bilateral insula, ACG, left precentral gyrus (PreCG) and right claustrum and lower FC in these brain areas in the SN, including the left insula, claustrum, inferior frontal gyrus (IFG) and extra-nuclear (Table 3 and Figure 4). No significantly increased FC was found in the patients in the three networks.
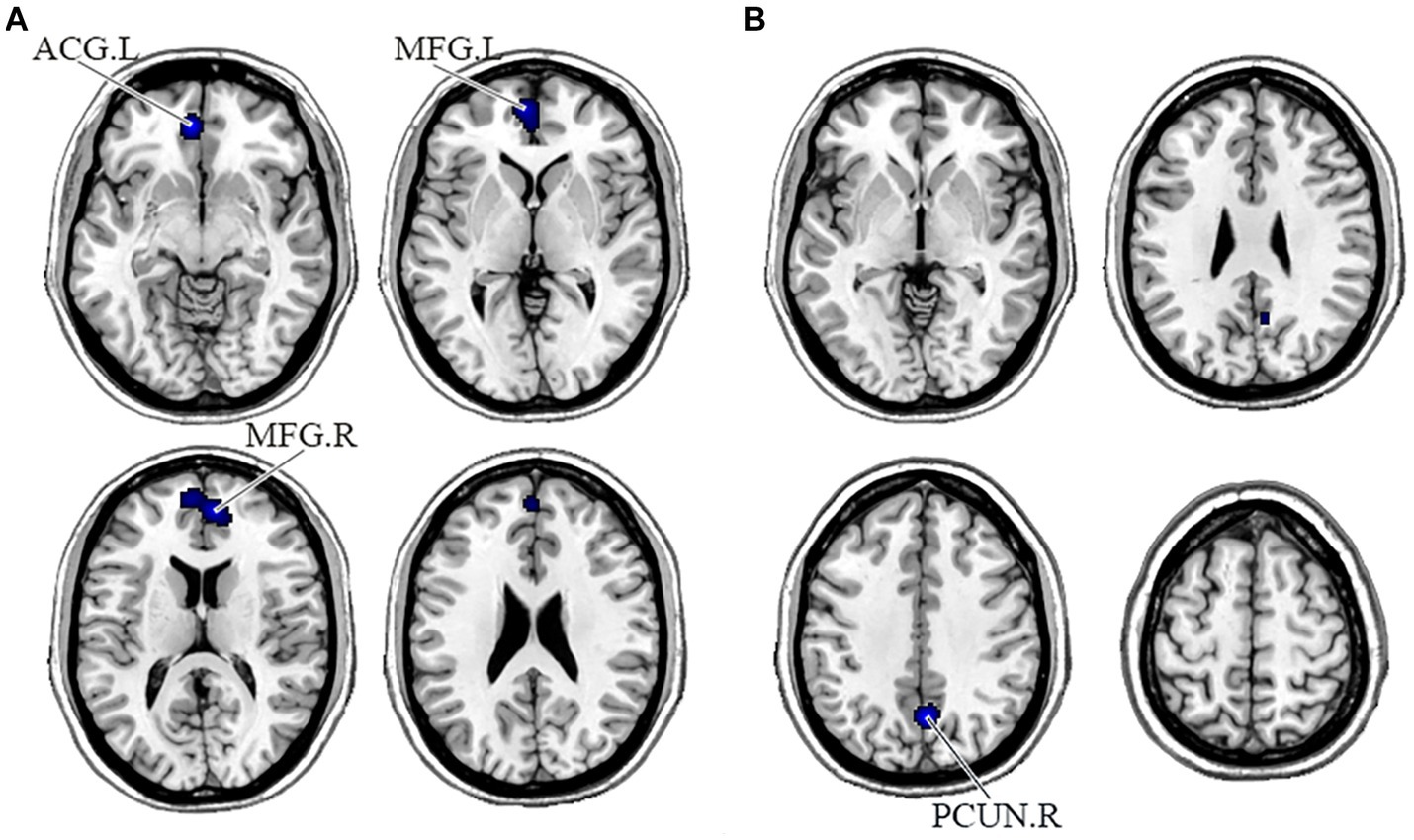
Figure 2. Displayed are significant results from the meta-analysis of the DMN studies. (A) Gray matter reduction in the DMN in PNS patients relative to HCs. (B) Areas showing lower FC in the DMN in PNS patients relative to HCs. FC, functional connectivity; PNS, Persistent negative symptoms; HCs, healthy controls; DMN, default mode network; ACG, anterior cingulate gyrus; MFG, medial frontal gyrus; PCUN, precuneus; R, right; L, left.
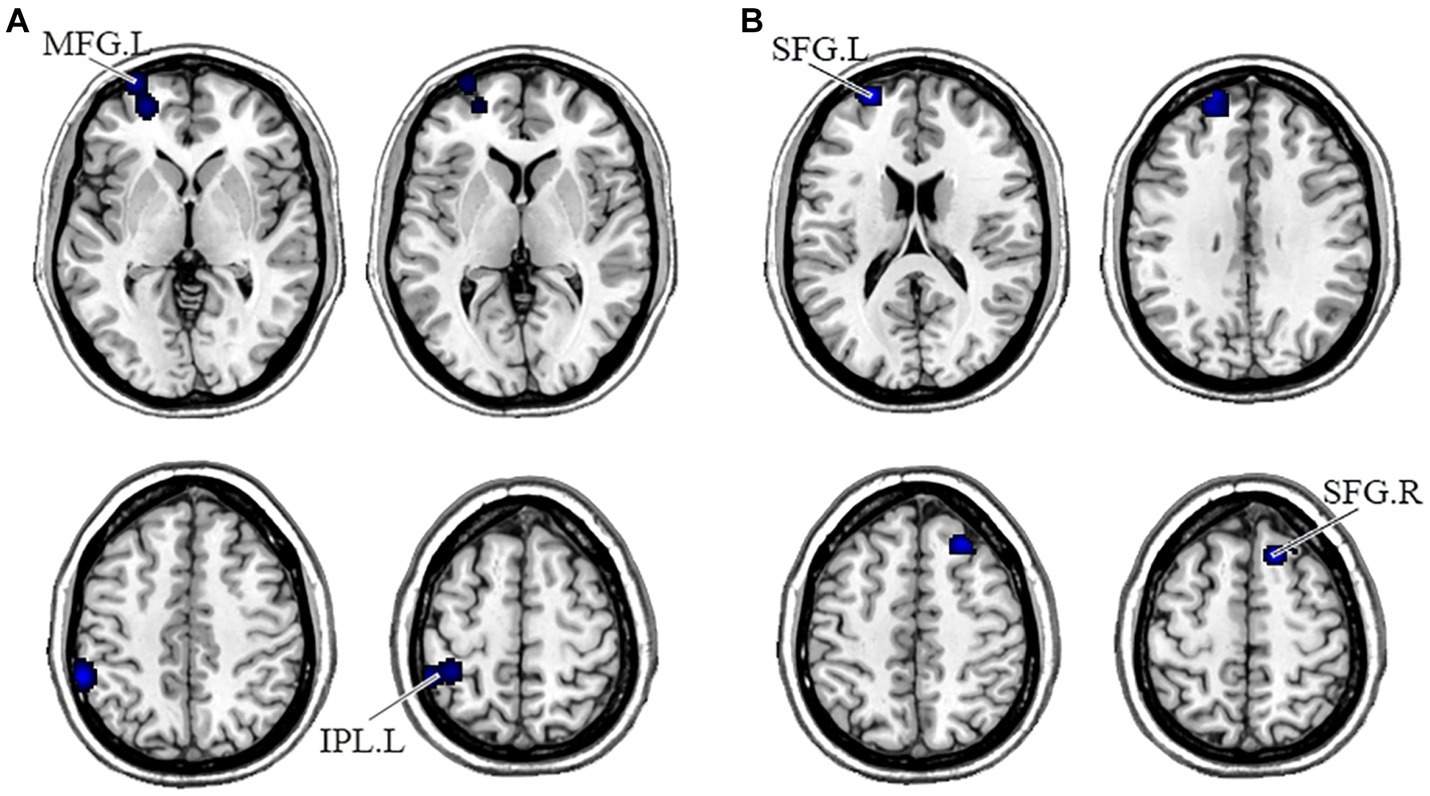
Figure 3. Displayed are significant results from the meta-analysis of the CEN studies. (A) Gray matter reduction in the CEN in PNS patients relative to HCs. (B) Areas showing lower FC in the CEN in PNS patients relative to HCs. FC, functional connectivity; PNS, Persistent negative symptoms; HCs, healthy controls; CEN, central executive network; MFG, medial frontal gyrus; IPL, inferior parietal lobule; SFG, superior frontal gyrus; R, right; L, left.
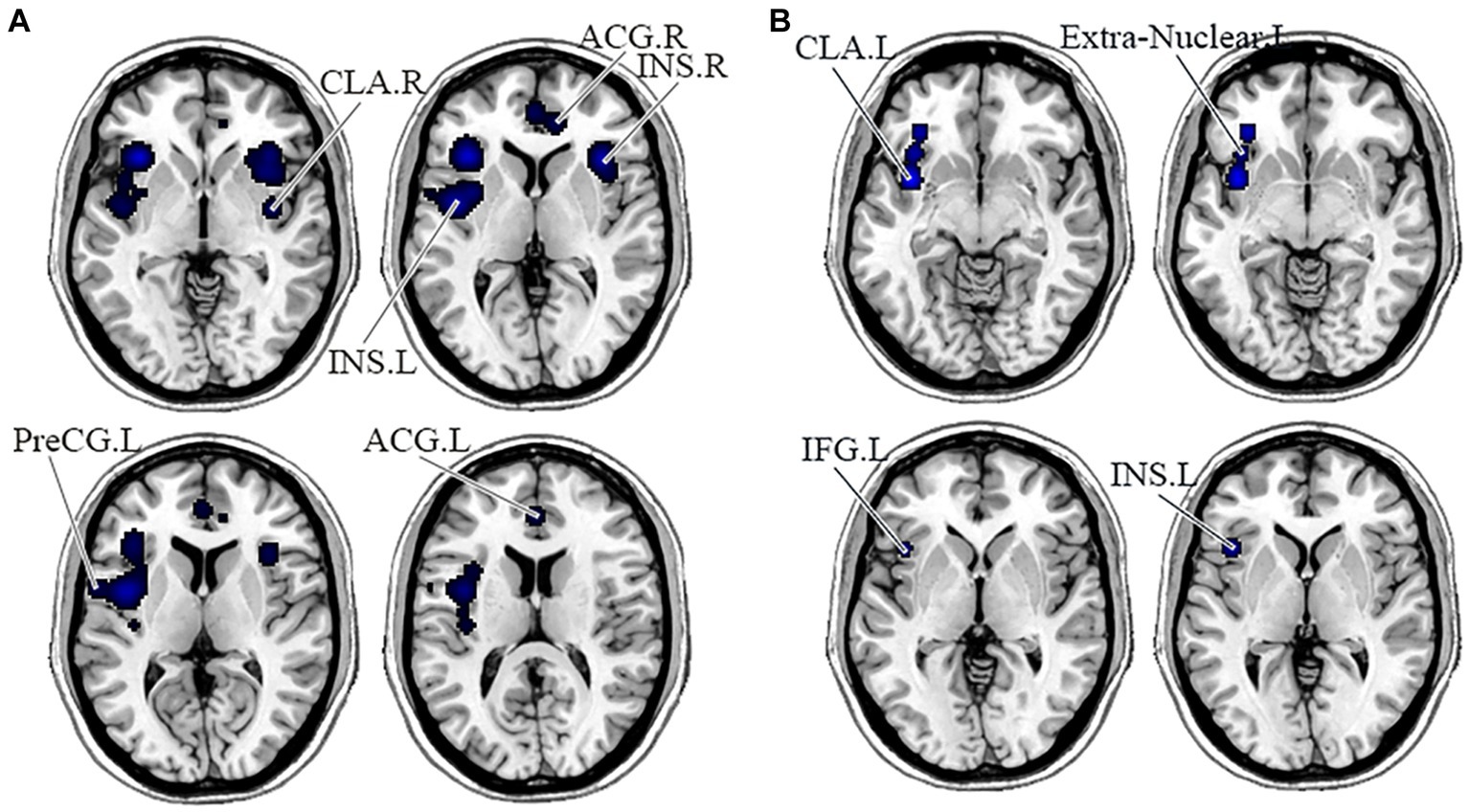
Figure 4. Displayed are significant results from the meta-analysis of the SN studies. (A) Gray matter reduction in the SN in PNS patients relative to HCs. (B) Areas showing lower FC in the SN in PNS patients relative to HCs. FC, functional connectivity; PNS, Persistent negative symptoms; HCs, healthy controls; SN, salience network; CLA, claustrum; ACG, anterior cingulate gyrus; INS, insula; PreCG, precentral gyrus; IFG, inferior frontal gyrus; R, right; L, left.
4. Discussion
This was the first meta-analysis to evaluate the functional and structural integrity of three brain networks in patients with PNS. Compared with the HCs, the PNS group had reduced GMV in bilateral MFG and left ACG, reduced FC in the right precuneus in the DMN; decreased GMV in the left IPL and MFG, and decreased FC in the bilateral SFG in the CEN; reduced GMV in the bilateral insula, ACG, left PreCG and right claustrum, and lower FC in the left insula, claustrum, IFG and extra-nuclear these in the SN. Similar to our results, an early report indicated that schizophrenia exhibited brain surface area contractions compared with normal controls in the three networks at the same time (Palaniyappan et al., 2011). Chronic and first-episode schizophrenia also had a significant reduction in the volume of gray matter (Chan et al., 2011; Koelkebeck et al., 2019; Sun et al., 2020), which relates to the severity of negative symptoms (Lei et al., 2019; Li et al., 2020). In addition, previous studies have shown altered FC of key regions in the DMN, CEN (Whitford et al., 2009; Repovs et al., 2011; Littow et al., 2015), and SN (Manoliu et al., 2013; Orliac et al., 2013; Wotruba et al., 2014). Overall, intra-network comparisons are useful to focus on recognizing brain areas with aberrant anatomical or functional alterations and therefore may be useful in detecting imaging features of PNS disease.
In our study, PNS patients showed structural and functional alterations in the DMN, including reduced GMV in bilateral MFG and left ACG, and reduced FC in the right precuneus. The MFG is located at the medial part of the prefrontal gyrus (Frascarelli et al., 2015), which is responsible for regulating emotional behavior and self-reference processes through the frontal-limbic circuit (Etkin et al., 2011; Chen et al., 2012; Müller et al., 2020). In many imaging studies, there is a consistent correlation between gray matter reduction in the prefrontal gyrus and the severity of negative symptoms (Cascella et al., 2010; Benoit et al., 2012; Jiang et al., 2019). The ACG, as a part of the ventromedial brain circuit, plays a critical role in evaluating the salience of emotional and motivational information and regulating emotional responses (Bush et al., 2000). Earlier findings indicated this region to be structurally altered and associated with negative symptoms (Sigmundsson et al., 2001; Baiano et al., 2007; Fornito et al., 2009; Berge et al., 2011), where the patients with the highest negative symptom levels show more gray matter loss (Asami et al., 2013). The precuneus modulates negative emotional responses primarily by triggering an attention shift in emotion regulation strategies (Ferri et al., 2016). Resting-state metabolic activities in the precuneus, dorsolateral prefrontal cortex and supplementary motor region had a negative correlation with physiological anhedonia in schizophrenia (Park et al., 2009). The pattern of functional interaction alterations in the DMN of schizophrenia patients has been universally correlated with negative symptoms (Park et al., 2009; Yang et al., 2019), indicating a key role for DMN in the etiology of negative symptoms and particularly in the field of apathy (Forlim et al., 2020). The primary function of the DMN includes self-referential processing (Buckner et al., 2008), so its abnormalities may result in disruptions in self-reflection and self-awareness, which may be the cause of anhedonia and apathy in schizophrenia.
Similarly, our study reported structural and functional alterations in the CEN, including decreased GMV in the left IPL and MFG, and reduced FC in the bilateral SFG. The gray matter loss in the IPL endorses the conception that IPL is engaged in the progression of schizophrenia with PNS (Sigmundsson et al., 2001). This may relate to the function of mirror neurons in the IPL in social cognition, especially empathy and understanding the behavior and intentions of others (Gallese et al., 2004; Rizzolatti and Sinigaglia, 2010). The prefrontal cortex, as a prototypical center of higher-order cognitive processing, is broadly connected to various brain regions (Meyer-Lindenberg et al., 2002; Barch and Dowd, 2010), and the interaction between synaptic plasticity and dopamine transmission in the prefrontal cortex has a prominent effect on psychotic symptoms in schizophrenia, particularly negative symptoms (Seamans and Yang, 2004). Numerous studies have reported the association between the prefrontal cortex and negative symptom severity, and rTMS treatment of the prefrontal cortex can reduce negative symptoms in schizophrenia (Brady et al., 2019; Kumar et al., 2020). The function of this region in negative symptoms requires further studies to elucidate.
Our study also reported reduced gray matter and lower FC in these brain areas in the SN, including the insula, PreCG, claustrum and extra-nuclear. Reduced GMV in the insula seems to be particularly associated with schizophrenia with PNS (Cascella et al., 2010; Li et al., 2018). The claustrum is a thin layer of neurons that appears to derive from migrating neuroblasts in the overlying cortex and lies below the insula and the temporal lobe (Galeno et al., 2004). Previous studies demonstrated a link between left insula dysfunction in schizophrenia and negative symptoms such as anhedonia and diminished social interactions (Manoliu et al., 2013), which might be related to impaired responses on pleasant stimuli caused by insula dysfunction. Despite few studies reporting the association between PreCG volume and negative symptoms, a prior meta-analysis found reduced GMV in the PreCG in schizophrenia with PNS (Li et al., 2018). The extra-nuclear region is located in the ventral emotional processing system and contains the main fibers connecting the striatum to the frontoparietal cortex, therefore structural or functional abnormalities of this pathway may affect emotional processing (Kring and Barch, 2014). Previous studies have reported that dysfunctioning of the cortico-striatal system has some connection with amotivation and anhedonia (Kring et al., 2013; Barnes et al., 2014). Early studies also indicated that in the triple-network model, SN-centered patients with low connectivity often had more severe and persistent negative symptoms than the subgroup of patients with high connectivity (Liang et al., 2021). However, we did not find that PNS patients had increased FC in the SN, which may be due to differences in patient samples, statistical methods and disease states. By summarizing these reports, our results confirm that PNS patients show more prominent GMV and FC decreases in these core networks than HCs, which may be the reason why patients with PNS exhibit a greater clinical symptom burden and poorer therapeutic outcomes.
In our study, the key region where DMN and CEN overlap is MFG, and the main region where DMN and SN overlap is ACG, suggesting that these networks interact in PNS patients. It is well known that the MFG and ACG play a key role in regulating emotional responses (Bush et al., 2000; Chen et al., 2012), and structural defects in these regions may be related to apathy in PNS patients. Moreover, self-rated avolition and anhedonia in schizophrenia are related to increased FC between the caudate and posterior DMN/CEN (Brakowski et al., 2020). It has been reported that connectivity between different subnetworks of CEN and DMN correlated with the severity of different clinical symptoms in schizophrenia (Xi et al., 2021). Taken together, one core network impairment affects other networks and its clinical aspects may surpass the initial disability. Significant impaired structure and function in the three networks signifies that schizophrenia with PNS involves multiple networks and the discovery of abnormalities in network connectivity has vital significance in searching for the network endophenotype of neuropsychiatric disease.
5. Limitations
This analysis has some limitations that should be considered. First, though we ascertained related studies by defining strict criteria for PNS, we were unable to ensure that negative symptoms persisted for at least 6 months and that all subjects included in the study met the PNS criteria, which may led to the heterogeneity of results. Second, the brain region of the three networks selected for the included studies may be influenced by the a priori hypotheses of the authors of each study. The clusters detected in our meta-analysis were facilitated by minority studies and require a greater sample size in the future. Third, the ALE software could not investigate the effect size differences between networks, and it failed to provide any solving approach to analyze the confidence interval to increase the robustness of our findings. Fourth, the study was limited by heterogeneity, including different data sources, different negative symptom assessment scales, preprocessing protocols, smoothing kernel size, slice thickness, and statistical threshold, which may have influenced our results in this study. Lastly, there is a great deal of heterogeneity among schizophrenia patients, including age of onset and dose of antipsychotic medications.
6. Conclusion and perspectives
This meta-analysis illuminates within-network comparisons to assist in the key identification of brain areas with abnormal anatomical or functional alterations within the DMN, CEN, and SN. The meaningful overlap of brain regions between three networks opens up new ideas for selecting specific brain regions as therapeutic targets for rTMS in the future. These current findings extend our understanding of patients with PNS through a brain network-level perspective and provide a starting point for designing targeted and effective interventions. However, the study of imaging markers in PNS patients is still in the preliminary stage, and the limitations of insufficient study samples and single study methods lead to the lack of consistent conclusions. In the future, neuroimaging studies should combine molecular biology, genomics, epigenetics, etc., to explore the formation mechanism of PNS at multiple levels, use deep learning and other methods to explore stable and reliable imaging markers of PNS patients and combine multi-modal biological indicators to explore biological targets conducive to diagnosis and curative effect prediction.
Author contributions
TZ guided by XZ and JC designed the study. ZeW, WW, and YL performed the meta-analysis and drafted the manuscript. ZiW, CZ, XF, CH, and CX helped in literature extraction and data analyses. All authors contributed to and approved the final text.
Funding
This work was supported by the National Key Research and Development Program of China (No. 2018YFC1314300), the National Natural Science Foundation of China (Nos. 81971255 and 82101572), the Social Development Foundation of Jiangsu Province, China (No. BE2019610), the Jiangsu Provincial Medical Talent Project of China (No. ZDRCA2016075), the Special Project of Basic Research on Frontier Leading Technology of Jiangsu Province, China (No. BK20192004D), the Key Project supported by Medical Science and Technology Development Foundation, Nanjing Department of Health (No. YKK20090), the National Natural Science Foundation of China (No. 81701675), the Key Project supported by Medical Science and technology development Foundation, Nanjing Department of Health (No. JQX18005), the Key Research and Development Plan (Social Development) Project of Jiangsu Province (No. BE2018608), the Top-Notch Talent Program of the Jiangsu Province High-Level Healthcare Talent “Six-Ones” Project (No. LGY2020058), and the Science and Technology Development Program of Nanjing Medical University (No. NMUB2019107).
Conflict of interest
The authors declare that the research was conducted in the absence of any commercial or financial relationships that could be construed as a potential conflict of interest.
Publisher’s note
All claims expressed in this article are solely those of the authors and do not necessarily represent those of their affiliated organizations, or those of the publisher, the editors and the reviewers. Any product that may be evaluated in this article, or claim that may be made by its manufacturer, is not guaranteed or endorsed by the publisher.
Supplementary material
The Supplementary material for this article can be found online at: https://www.frontiersin.org/articles/10.3389/fnhum.2023.1204632/full#supplementary-material
Footnotes
References
Alonso-Solís, A., Vives-Gilabert, Y., Grasa, E., Portella, M. J., Rabella, M., Sauras, R. B., et al. (2015). Resting-state functional connectivity alterations in the default network of schizophrenia patients with persistent auditory verbal hallucinations. Schizophr. Res. 161, 261–268. doi: 10.1016/j.schres.2014.10.047
Anderson, V. M., Goldstein, M. E., Kydd, R. R., and Russell, B. R. (2015). Extensive gray matter volume reduction in treatment-resistant schizophrenia. Int. J. Neuropsychopharmacol. 18:pyv016. doi: 10.1093/ijnp/pyv016
Andreasen, N. C. (1989). The scale for the assessment of negative symptoms (SANS): conceptual and theoretical foundations. Br. J. Psychiatry Suppl. 155, 49–52. doi: 10.1192/S0007125000291496
Asami, T., Whitford, T. J., Bouix, S., Dickey, C. C., Niznikiewicz, M., Shenton, M. E., et al. (2013). Globally and locally reduced MRI gray matter volumes in neuroleptic-naive men with schizotypal personality disorder: association with negative symptoms. JAMA Psychiatry 70, 361–372. doi: 10.1001/jamapsychiatry.2013.665
Ashburner, J., and Friston, K. J. (2000). Voxel-based morphometry—the methods. NeuroImage 11, 805–821. doi: 10.1006/nimg.2000.0582
Baiano, M., David, A., Versace, A., Churchill, R., Balestrieri, M., and Brambilla, P. (2007). Anterior cingulate volumes in schizophrenia: a systematic review and a meta-analysis of MRI studies. Schizophr. Res. 93, 1–12. doi: 10.1016/j.schres.2007.02.012
Barch, D. M., and Dowd, E. C. (2010). Goal representations and motivational drive in schizophrenia: the role of prefrontal-striatal interactions. Schizophr. Bull. 36, 919–934. doi: 10.1093/schbul/sbq068
Barnes, S. A., Der-Avakian, A., and Markou, A. (2014). Anhedonia, avolition, and anticipatory deficits: assessments in animals with relevance to the negative symptoms of schizophrenia. Eur. Neuropsychopharmacol. 24, 744–758. doi: 10.1016/j.euroneuro.2013.10.001
Bassitt, D. P., Neto, M. R., de Castro, C. C., and Busatto, G. F. (2007). Insight and regional brain volumes in schizophrenia. Eur. Arch. Psychiatry Clin. Neurosci. 257, 58–62. doi: 10.1007/s00406-006-0685-z
Benoit, A., Bodnar, M., Malla, A. K., Joober, R., and Lepage, M. (2012). The structural neural substrates of persistent negative symptoms in first-episode of non-affective psychosis: a voxel-based morphometry study. Front. Psych. 3:42. doi: 10.3389/fpsyt.2012.00042
Berge, D., Carmona, S., Rovira, M., Bulbena, A., Salgado, P., and Vilarroya, O. (2011). Gray matter volume deficits and correlation with insight and negative symptoms in first-psychotic-episode subjects. Acta Psychiatr. Scand. 123, 431–439. doi: 10.1111/j.1600-0447.2010.01635.x
Bluhm, R. L., Miller, J., Lanius, R. A., Osuch, E. A., Boksman, K., Neufeld, R. W., et al. (2007). Spontaneous low-frequency fluctuations in the BOLD signal in schizophrenic patients: anomalies in the default network. Schizophr. Bull. 33, 1004–1012. doi: 10.1093/schbul/sbm052
Bodnar, M., Hovington, C. L., Buchy, L., Malla, A. K., Joober, R., and Lepage, M. (2014). Cortical thinning in temporo-parietal junction (TPJ) in non-affective first-episode of psychosis patients with persistent negative symptoms. PLoS One 9:e101372. doi: 10.1371/journal.pone.0101372
Brady, R. O., Gonsalvez, I., Lee, I., Öngür, D., Seidman, L. J., Schmahmann, J. D., et al. (2019). Cerebellar-prefrontal network connectivity and negative symptoms in schizophrenia. Am. J. Psychiatry 176, 512–520. doi: 10.1176/appi.ajp.2018.18040429
Brakowski, J., Manoliu, A., Homan, P., Bosch, O. G., Herdener, M., Seifritz, E., et al. (2020). Aberrant striatal coupling with default mode and central executive network relates to self-reported avolition and anhedonia in schizophrenia. J. Psychiatr. Res. 145, 263–275. doi: 10.1016/j.jpsychires.2020.10.047
Brambilla, P., Hardan, A., di Nemi, S. U., Perez, J., Soares, J. C., and Barale, F. (2003). Brain anatomy and development in autism: review of structural MRI studies. Brain Res. Bull. 61, 557–569. doi: 10.1016/j.brainresbull.2003.06.001
Buchanan, R. W. (2007). Persistent negative symptoms in schizophrenia: an overview. Schizophr. Bull. 33, 1013–1022. doi: 10.1093/schbul/sbl057
Buckner, R. L., Andrews-Hanna, J. R., and Schacter, D. L. (2008). The brain's default network: anatomy, function, and relevance to disease. Ann. N. Y. Acad. Sci. 1124, 1–38. doi: 10.1196/annals.1440.011
Buckner, R. L., Krienen, F. M., Castellanos, A., Diaz, J. C., and Yeo, B. T. (2011). The organization of the human cerebellum estimated by intrinsic functional connectivity. J. Neurophysiol. 106, 2322–2345. doi: 10.1152/jn.00339.2011
Bush, G., Luu, P., and Posner, M. I. (2000). Cognitive and emotional influences in anterior cingulate cortex. Trends Cogn. Sci. 4, 215–222. doi: 10.1016/S1364-6613(00)01483-2
Carpenter, W. T., Heinrichs, D. W., and Wagman, A. M. (1988). Deficit and nondeficit forms of schizophrenia: the concept. Am. J. Psychiatry 145, 578–583. doi: 10.1176/ajp.145.5.578
Cascella, N. G., Fieldstone, S. C., Rao, V. A., Pearlson, G. D., Sawa, A., and Schretlen, D. J. (2010). Gray-matter abnormalities in deficit schizophrenia. Schizophr. Res. 120, 63–70. doi: 10.1016/j.schres.2010.03.039
Castellon, S. A., Asarnow, R. F., Goldstein, M. J., and Marder, S. R. (1994). Persisting negative symptoms and information-processing deficits in schizophrenia: implications for subtyping. Psychiatry Res. 54, 59–69. doi: 10.1016/0165-1781(94)90065-5
Chan, R. C., Di, X., McAlonan, G. M., and Gong, Q. Y. (2011). Brain anatomical abnormalities in high-risk individuals, first-episode, and chronic schizophrenia: an activation likelihood estimation meta-analysis of illness progression. Schizophr. Bull. 37, 177–188. doi: 10.1093/schbul/sbp073
Chang, X., Shen, H., Wang, L., Liu, Z., Xin, W., Hu, D., et al. (2014). Altered default mode and fronto-parietal network subsystems in patients with schizophrenia and their unaffected siblings. Brain Res. 1562, 87–99. doi: 10.1016/j.brainres.2014.03.024
Chee, T. T., Chua, L., Morrin, H., Lim, M. F., Fam, J., and Ho, R. (2020). Neuroanatomy of patients with deficit schizophrenia: an exploratory quantitative Meta-analysis of structural neuroimaging studies. Int. J. Environ. Res. Public Health 17. doi: 10.3390/ijerph17176227
Chen, Q., Chen, X., He, X., Wang, L., Wang, K., and Qiu, B. (2016). Aberrant structural and functional connectivity in the salience network and central executive network circuit in schizophrenia. Neurosci. Lett. 627, 178–184. doi: 10.1016/j.neulet.2016.05.035
Chen, X., Duan, M., He, H., Yang, M., Klugah-Brown, B., Xu, H., et al. (2016). Functional abnormalities of the right posterior insula are related to the altered self-experience in schizophrenia. Psychiatry Res. Neuroimaging 256, 26–32. doi: 10.1016/j.pscychresns.2016.09.006
Chen, J. D., Liu, F., Xun, G. L., Chen, H. F., Hu, M. R., Guo, X. F., et al. (2012). Early and late onset, first-episode, treatment-naive depression: same clinical symptoms, different regional neural activities. J. Affect. Disord. 143, 56–63. doi: 10.1016/j.jad.2012.05.025
Cole, D. M., Smith, S. M., and Beckmann, C. F. (2010). Advances and pitfalls in the analysis and interpretation of resting-state FMRI data. Front. Syst. Neurosci. 4:8. doi: 10.3389/fnsys.2010.00008
Dong, D. B., Duan, M. J., Wang, Y. L., Zhang, X. X., Jia, X. Y., Li, Y. J., et al. (2019). Reconfiguration of dynamic functional connectivity in sensory and perceptual system in schizophrenia. Cereb. Cortex 29, 3577–3589. doi: 10.1093/cercor/bhy232
Dong, D., Wang, Y., Chang, X., Luo, C., and Yao, D. (2018). Dysfunction of large-scale brain networks in schizophrenia: a meta-analysis of resting-state functional connectivity. Schizophr. Bull. 44, 168–181. doi: 10.1093/schbul/sbx034
Eickhoff, S. B., Laird, A. R., Fox, P. M., Lancaster, J. L., and Fox, P. T. (2017). Implementation errors in the GingerALE software: description and recommendations. Hum. Brain Mapp. 38, 7–11. doi: 10.1002/hbm.23342
Ellison-Wright, I., Glahn, D. C., Laird, A. R., Thelen, S. M., and Bullmore, E. (2008). The anatomy of first-episode and chronic schizophrenia: an anatomical likelihood estimation meta-analysis. Am. J. Psychiatry 165, 1015–1023. doi: 10.1176/appi.ajp.2008.07101562
Etkin, A., Egner, T., and Kalisch, R. (2011). Emotional processing in anterior cingulate and medial prefrontal cortex. Trends Cogn. Sci. 15, 85–93. doi: 10.1016/j.tics.2010.11.004
Fan, F., Tan, S., Huang, J., Chen, S., Fan, H., Wang, Z., et al. (2020). Functional disconnection between subsystems of the default mode network in schizophrenia. Psychol. Med. 52, 2270–2280. doi: 10.1017/S003329172000416X
Fan, F. M., Tan, S. P., Yang, F. D., Tan, Y. L., Zhao, Y. L., Chen, N., et al. (2013). Ventral medial prefrontal functional connectivity and emotion regulation in chronic schizophrenia: a pilot study. Neurosci. Bull. 29, 59–74. doi: 10.1007/s12264-013-1300-8
Ferri, J., Schmidt, J., Hajcak, G., and Canli, T. (2016). Emotion regulation and amygdala-precuneus connectivity: focusing on attentional deployment. Cogn. Affect. Behav. Neurosci. 16, 991–1002. doi: 10.3758/s13415-016-0447-y
Forlim, C. G., Klock, L., Bächle, J., Stoll, L., Giemsa, P., Fuchs, M., et al. (2020). Reduced resting-state connectivity in the Precuneus is correlated with apathy in patients with schizophrenia. Sci. Rep. 10:2616. doi: 10.1038/s41598-020-59393-6
Fornito, A., Yucel, M., Dean, B., Wood, S. J., and Pantelis, C. (2009). Anatomical abnormalities of the anterior cingulate cortex in schizophrenia: bridging the gap between neuroimaging and neuropathology. Schizophr. Bull. 35, 973–993. doi: 10.1093/schbul/sbn025
Frascarelli, M., Tognin, S., Mirigliani, A., Parente, F., Buzzanca, A., Torti, M. C., et al. (2015). Medial frontal gyrus alterations in schizophrenia: relationship with duration of illness and executive dysfunction. Psychiatry Res. 231, 103–110. doi: 10.1016/j.pscychresns.2014.10.017
Galderisi, S., Mucci, A., Bitter, I., Libiger, J., Bucci, P., Fleischhacker, W. W., et al. (2013). Persistent negative symptoms in first episode patients with schizophrenia: results from the European first episode schizophrenia trial. Eur. Neuropsychopharmacol. 23, 196–204. doi: 10.1016/j.euroneuro.2012.04.019
Galderisi, S., Mucci, A., Buchanan, R. W., and Arango, C. (2018). Negative symptoms of schizophrenia: new developments and unanswered research questions. Lancet Psychiatry 5, 664–677. doi: 10.1016/S2215-0366(18)30050-6
Galeno, R., Molina, M., Guirao, M., and Isoardi, R. (2004). Severity of negative symptoms in schizophrenia correlated to hyperactivity of the left globus pallidus and the right claustrum. A PET study. World J. Biol. Psychiatry 5, 20–25. doi: 10.1080/15622970410029903
Gallese, V., Keysers, C., and Rizzolatti, G. (2004). A unifying view of the basis of social cognition. Trends Cogn. Sci. 8, 396–403. doi: 10.1016/j.tics.2004.07.002
Gao, J., Tang, X., Wang, C., Yu, M., Sha, W., Wang, X., et al. (2020). Aberrant cerebellar neural activity and cerebro-cerebellar functional connectivity involving executive dysfunction in schizophrenia with primary negative symptoms. Brain Imaging Behav. 14, 869–880. doi: 10.1007/s11682-018-0032-9
González-Valderrama, A., Castañeda, C. P., Mena, C., Undurraga, J., Mondaca, P., Yañez, M., et al. (2017). Duration of untreated psychosis and acute remission of negative symptoms in a south American first-episode psychosis cohort. Early Interv. Psychiatry 11, 77–82. doi: 10.1111/eip.12266
Greicius, M. D., Supekar, K., Menon, V., and Dougherty, R. F. (2009). Resting-state functional connectivity reflects structural connectivity in the default mode network. Cereb. Cortex 19, 72–78. doi: 10.1093/cercor/bhn059
Haijma, S. V., Van Haren, N., Cahn, W., Koolschijn, P. C., Hulshoff Pol, H. E., and Kahn, R. S. (2013). Brain volumes in schizophrenia: a meta-analysis in over 18 000 subjects. Schizophr. Bull. 39, 1129–1138. doi: 10.1093/schbul/sbs118
Hare, S. M., Ford, J. M., Mathalon, D. H., Damaraju, E., Bustillo, J., Belger, A., et al. (2019). Salience-default mode functional network connectivity linked to positive and negative symptoms of schizophrenia. Schizophr. Bull. 45, 892–901. doi: 10.1093/schbul/sby112
Herold, R., Feldmann, A., Simon, M., Tényi, T., Kövér, F., Nagy, F., et al. (2009). Regional gray matter reduction and theory of mind deficit in the early phase of schizophrenia: a voxel-based morphometric study. Acta Psychiatr. Scand. 119, 199–208. doi: 10.1111/j.1600-0447.2008.01297.x
Honea, R. A., Meyer-Lindenberg, A., Hobbs, K. B., Pezawas, L., Mattay, V. S., Egan, M. F., et al. (2008). Is gray matter volume an intermediate phenotype for schizophrenia? A voxel-based morphometry study of patients with schizophrenia and their healthy siblings. Bio. Psych. 63, 465–474. doi: 10.1016/j.biopsych.2007.05.027
Huang, H., Botao, Z., Jiang, Y., Tang, Y., Zhang, T., Tang, X., et al. (2020). Aberrant resting-state functional connectivity of salience network in first-episode schizophrenia. Brain Imaging Behav. 14, 1350–1360. doi: 10.1007/s11682-019-00040-8
Huang, P., Xi, Y., Lu, Z. L., Chen, Y., Li, X., Li, W., et al. (2015). Decreased bilateral thalamic gray matter volume in first-episode schizophrenia with prominent hallucinatory symptoms: a volumetric MRI study. Sci. Rep. 5:14505. doi: 10.1038/srep14505
İnce, E., and Üçok, A. (2018). Relationship between persistent negative symptoms and findings of Neurocognition and neuroimaging in schizophrenia. Clin. EEG Neurosci. 49, 27–35. doi: 10.1177/1550059417746213
Jayakumar, P. N., Venkatasubramanian, G., Gangadhar, B. N., Janakiramaiah, N., and Keshavan, M. S. (2005). Optimized voxel-based morphometry of gray matter volume in first-episode, antipsychotic-naive schizophrenia. Prog. Neuro-Psychopharmacol. Biol. Psychiatry 29, 587–591. doi: 10.1016/j.pnpbp.2005.01.020
Jiang, Y., Duan, M., Chen, X., Zhang, X., Gong, J., Dong, D., et al. (2019). Aberrant prefrontal-thalamic-cerebellar circuit in schizophrenia and depression: evidence from a possible causal connectivity. Int. J. Neural Syst. 29:1850032. doi: 10.1142/S0129065718500326
Kambeitz, J., Kambeitz-Ilankovic, L., Cabral, C., Dwyer, D. B., Calhoun, V. D., van den Heuvel, M. P., et al. (2016). Aberrant functional whole-brain network architecture in patients with schizophrenia: a meta-analysis. Schizophr. Bull. 42, S13–S21. doi: 10.1093/schbul/sbv174
Kay, S. R., Fiszbein, A., and Opler, L. A. (1987). The positive and negative syndrome scale (PANSS) for schizophrenia. Schizophr. Bull. 13, 261–276. doi: 10.1093/schbul/13.2.261
Kim, G. W., Kim, Y. H., and Jeong, G. W. (2017). Whole brain volume changes and its correlation with clinical symptom severity in patients with schizophrenia: a DARTEL-based VBM study. PLoS One 12:e0177251. doi: 10.1371/journal.pone.0177251
Kirkpatrick, B., Fenton, W. S., Carpenter, W. T., and Marder, S. R. (2006). The NIMH-MATRICS consensus statement on negative symptoms. Schizophr. Bull. 32, 214–219. doi: 10.1093/schbul/sbj053
Kirkpatrick, B., Strauss, G. P., Nguyen, L., Fischer, B. A., Daniel, D. G., Cienfuegos, A., et al. (2011). The brief negative symptom scale: psychometric properties. Schizophr. Bull. 37, 300–305. doi: 10.1093/schbul/sbq059
Koelkebeck, K., Dannlowski, U., Ohrmann, P., Suslow, T., Murai, T., Bauer, J., et al. (2019). Gray matter volume reductions in patients with schizophrenia: a replication study across two cultural backgrounds. Psychiatry Res. Neuroimaging 292, 32–40. doi: 10.1016/j.pscychresns.2019.08.008
Koutsouleris, N., Gaser, C., Jäger, M., Bottlender, R., Frodl, T., Holzinger, S., et al. (2008). Structural correlates of psychopathological symptom dimensions in schizophrenia: a voxel-based morphometric study. NeuroImage 39, 1600–1612. doi: 10.1016/j.neuroimage.2007.10.029
Kring, A. M., and Barch, D. M. (2014). The motivation and pleasure dimension of negative symptoms: neural substrates and behavioral outputs. Eur. Neuropsychopharmacol. 24, 725–736. doi: 10.1016/j.euroneuro.2013.06.007
Kring, A. M., Gur, R. E., Blanchard, J. J., Horan, W. P., and Reise, S. P. (2013). The clinical assessment interview for negative symptoms (CAINS): final development and validation. Am. J. Psychiatry 170, 165–172. doi: 10.1176/appi.ajp.2012.12010109
Kumar, N., Vishnubhatla, S., Wadhawan, A. N., Minhas, S., and Gupta, P. (2020). A randomized, double blind, sham-controlled trial of repetitive transcranial magnetic stimulation (rTMS) in the treatment of negative symptoms in schizophrenia. Brain Stimul. 13, 840–849. doi: 10.1016/j.brs.2020.02.016
Kuroki, N., Kashiwagi, H., Ota, M., Ishikawa, M., Kunugi, H., Sato, N., et al. (2017). Brain structure differences among male schizophrenic patients with history of serious violent acts: an MRI voxel-based morphometric study. BMC Psychiatry 17:105. doi: 10.1186/s12888-017-1263-9
Lei, W., Kirkpatrick, B., Wang, Q., Deng, W., Li, M., Guo, W., et al. (2019). Progressive brain structural changes after the first year of treatment in first-episode treatment-naive patients with deficit or nondeficit schizophrenia. Psychiatry Res. Neuroimaging 288, 12–20. doi: 10.1016/j.pscychresns.2019.04.009
Li, Y., Li, W. X., Xie, D. J., Wang, Y., Cheung, E. F. C., and Chan, R. C. K. (2018). Grey matter reduction in the caudate nucleus in patients with persistent negative symptoms: an ALE meta-analysis. Schizophr. Res. 192, 9–15. doi: 10.1016/j.schres.2017.04.005
Li, H., Ou, Y., Liu, F., Su, Q., Zhang, Z., Chen, J., et al. (2020). Region-specific insular volumetric decreases in drug-naive, first-episode schizophrenia and their unaffected siblings. Am. J. Med. Genet. B Neuropsychiatr. Genet. 183, 106–112. doi: 10.1002/ajmg.b.32765
Liang, S., Wang, Q., Greenshaw, A. J., Li, X., Deng, W., Ren, H., et al. (2021). Aberrant triple-network connectivity patterns discriminate biotypes of first-episode medication-naive schizophrenia in two large independent cohorts. Neuropsychopharmacology 46, 1502–1509. doi: 10.1038/s41386-020-00926-y
Littow, H., Huossa, V., Karjalainen, S., Jääskeläinen, E., Haapea, M., Miettunen, J., et al. (2015). Aberrant functional connectivity in the default mode and central executive networks in subjects with schizophrenia - a whole-brain resting-state ICA study. Front. Psych. 6:26. doi: 10.3389/fpsyt.2015.00026
Liu, Y., Chen, Y., Liang, X., Li, D., Zheng, Y., Zhang, H., et al. (2020). Altered resting-state functional connectivity of multiple networks and disrupted correlation with executive function in major depressive disorder. Front. Neurol. 11:272. doi: 10.3389/fneur.2020.00272
Liu, H., Luo, Q., Du, W., Li, X., Zhang, Z., Yu, R., et al. (2018). Cigarette smoking and schizophrenia independently and reversibly altered intrinsic brain activity. Brain Imaging Behav. 12, 1457–1465. doi: 10.1007/s11682-017-9806-8
Manoliu, A., Riedl, V., Doll, A., Bäuml, J. G., Mühlau, M., Schwerthöffer, D., et al. (2013). Insular dysfunction reflects altered between-network connectivity and severity of negative symptoms in schizophrenia during psychotic remission. Front. Hum. Neurosci. 7:216. doi: 10.3389/fnhum.2013.00216
Manoliu, A., Riedl, V., Zherdin, A., Mühlau, M., Schwerthöffer, D., Scherr, M., et al. (2014). Aberrant dependence of default mode/central executive network interactions on anterior insular salience network activity in schizophrenia. Schizophr. Bull. 40, 428–437. doi: 10.1093/schbul/sbt037
Meisenzahl, E. M., Koutsouleris, N., Bottlender, R., Scheucrecker, J., Jaeger, A., Teipel, S. J., et al. (2008). Structural brain alterations at different stages of schizophrenia: a voxel-based morphometric study. Schizophr. Res. 104, 44–60. doi: 10.1016/j.schres.2008.06.023
Menon, V. (2011). Large-scale brain networks and psychopathology: a unifying triple network model. Trends Cogn. Sci. 15, 483–506. doi: 10.1016/j.tics.2011.08.003
Meyer-Lindenberg, A., Miletich, R. S., Kohn, P. D., Esposito, G., Carson, R. E., Quarantelli, M., et al. (2002). Reduced prefrontal activity predicts exaggerated striatal dopaminergic function in schizophrenia. Nat. Neurosci. 5, 267–271. doi: 10.1038/nn804
Moher, D., Liberati, A., Tetzlaff, J., and Altman, D. G. (2009). Preferred reporting items for systematic reviews and meta-analyses: the PRISMA statement. BMJ 339:b2535. doi: 10.1136/bmj.b2535
Müller, N. C. J., Dresler, M., Janzen, G., Beckmann, C. F., Fernández, G., and Kohn, N. (2020). Medial prefrontal decoupling from the default mode network benefits memory. Neuroimage 210:116543. doi: 10.1016/j.neuroimage.2020.116543
Neugebauer, K., Hammans, C., Wensing, T., Kumar, V., Grodd, W., Mevissen, L., et al. (2019). Nerve growth factor serum levels are associated with regional gray matter volume differences in schizophrenia patients. Front. Psych. 10:275. doi: 10.3389/fpsyt.2019.00275
Orliac, F., Naveau, M., Joliot, M., Delcroix, N., Razafimandimby, A., Brazo, P., et al. (2013). Links among resting-state default-mode network, salience network, and symptomatology in schizophrenia. Schizophr. Res. 148, 74–80. doi: 10.1016/j.schres.2013.05.007
Paillère-Martinot, M., Caclin, A., Artiges, E., Poline, J. B., Joliot, M., Mallet, L., et al. (2001). Cerebral gray and white matter reductions and clinical correlates in patients with early onset schizophrenia. Schizophr. Res. 50, 19–26. doi: 10.1016/S0920-9964(00)00137-7
Palaniyappan, L., Mallikarjun, P., Joseph, V., White, T. P., and Liddle, P. F. (2011). Regional contraction of brain surface area involves three large-scale networks in schizophrenia. Schizophr. Res. 129, 163–168. doi: 10.1016/j.schres.2011.03.020
Park, I. H., Kim, J. J., Chun, J., Jung, Y. C., Seok, J. H., Park, H. J., et al. (2009). Medial prefrontal default-mode hypoactivity affecting trait physical anhedonia in schizophrenia. Psychiatry Res. 171, 155–165. doi: 10.1016/j.pscychresns.2008.03.010
Penner, J., Ford, K. A., Taylor, R., Schaefer, B., Théberge, J., Neufeld, R. W., et al. (2016). Medial prefrontal and anterior insular connectivity in early schizophrenia and major depressive disorder: a resting functional MRI evaluation of large-scale brain network models. Front. Hum. Neurosci. 10:132. doi: 10.3389/fnhum.2016.00132
Penner, J., Osuch, E. A., Schaefer, B., Théberge, J., Neufeld, R. W. J., Menon, R. S., et al. (2018a). Higher order thalamic nuclei resting network connectivity in early schizophrenia and major depressive disorder. Psychiatry Res. Neuroimaging 272, 7–16. doi: 10.1016/j.pscychresns.2017.12.002
Penner, J., Osuch, E. A., Schaefer, B., Théberge, J., Neufeld, R. W. J., Menon, R. S., et al. (2018b). Temporoparietal junction functional connectivity in early schizophrenia and major depressive disorder. Chronic Stress 2:2470547018815232. doi: 10.1177/2470547018815232
Peters, S. K., Dunlop, K., and Downar, J. (2016). Cortico-striatal-thalamic loop circuits of the salience network: a central pathway in psychiatric disease and treatment. Front. Syst. Neurosci. 10:104. doi: 10.3389/fnsys.2016.00104
Peters, H., Riedl, V., Manoliu, A., Scherr, M., Schwerthoffer, D., Zimmer, C., et al. (2017). Changes in extra-striatal functional connectivity in patients with schizophrenia in a psychotic episode. Br. J. Psychiatry 210, 75–82. doi: 10.1192/bjp.bp.114.151928
Pettersson-Yeo, W., Allen, P., Benetti, S., McGuire, P., and Mechelli, A. (2011). Dysconnectivity in schizophrenia: where are we now? Neurosci. Biobehav. Rev. 35, 1110–1124. doi: 10.1016/j.neubiorev.2010.11.004
Poletti, S., Vai, B., Smeraldi, E., Cavallaro, R., Colombo, C., and Benedetti, F. (2016). Adverse childhood experiences influence the detrimental effect of bipolar disorder and schizophrenia on cortico-limbic grey matter volumes. J. Affect. Disord. 189, 290–297. doi: 10.1016/j.jad.2015.09.049
Raichle, M. E., MacLeod, A. M., Snyder, A. Z., Powers, W. J., Gusnard, D. A., and Shulman, G. L. (2001). A default mode of brain function. Proc. Natl. Acad. Sci. U. S. A. 98, 676–682. doi: 10.1073/pnas.98.2.676
Repovs, G., Csernansky, J. G., and Barch, D. M. (2011). Brain network connectivity in individuals with schizophrenia and their siblings. Biol. Psychiatry 69, 967–973. doi: 10.1016/j.biopsych.2010.11.009
Rizzolatti, G., and Sinigaglia, C. (2010). The functional role of the parieto-frontal mirror circuit: interpretations and misinterpretations. Nat. Rev. Neurosci. 11, 264–274. doi: 10.1038/nrn2805
Salgado-Pineda, P., Junqué, C., Vendrell, P., Baeza, I., Bargalló, N., Falcón, C., et al. (2004). Decreased cerebral activation during CPT performance: structural and functional deficits in schizophrenic patients. Neuroimage 21, 840–847. doi: 10.1016/j.neuroimage.2003.10.027
Schuster, C., Schuller, A. M., Paulos, C., Namer, I., Pull, C., Danion, J. M., et al. (2012). Gray matter volume decreases in elderly patients with schizophrenia: a voxel-based morphometry study. Schizophr. Bull. 38, 796–802. doi: 10.1093/schbul/sbq150
Seamans, J. K., and Yang, C. R. (2004). The principal features and mechanisms of dopamine modulation in the prefrontal cortex. Prog. Neurobiol. 74, 1–58. doi: 10.1016/j.pneurobio.2004.05.006
Sharma, A., Kumar, A., Singh, S., Bhatia, T., Beniwal, R. P., Khushu, S., et al. (2018). Altered resting state functional connectivity in early course schizophrenia. Psychiatry Res. Neuroimaging 271, 17–23. doi: 10.1016/j.pscychresns.2017.11.013
Shehzad, Z., Kelly, A. M., Reiss, P. T., Gee, D. G., Gotimer, K., Uddin, L. Q., et al. (2009). The resting brain: unconstrained yet reliable. Cereb. Cortex 19, 2209–2229. doi: 10.1093/cercor/bhn256
Sigmundsson, T., Suckling, J., Maier, M., Williams, S., Bullmore, E., Greenwood, K., et al. (2001). Structural abnormalities in frontal, temporal, and limbic regions and interconnecting white matter tracts in schizophrenic patients with prominent negative symptoms. Am. J. Psychiatry 158, 234–243. doi: 10.1176/appi.ajp.158.2.234
Spalthoff, R., Gaser, C., and Nenadić, I. (2018). Altered gyrification in schizophrenia and its relation to other morphometric markers. Schizophr. Res. 202, 195–202. doi: 10.1016/j.schres.2018.07.014
Sun, T., Zhao, P. F., Jiang, X. W., Zhou, Y. F., Li, C., Jia, L. N., et al. (2020). Distinct associations of cognitive impairments and reduced gray matter volumes in remitted patients with schizophrenia and bipolar disorder. Neural Plast. 2020, 1–9. doi: 10.1155/2020/8859388
Szendi, I., Szabó, N., Domján, N., Kincses, Z. T., Palkó, A., Vécsei, L., et al. (2017). A new division of schizophrenia revealed expanded bilateral brain structural abnormalities of the association cortices. Front. Psych. 8:127. doi: 10.3389/fpsyt.2017.00127
Wang, H. L., Guo, W. B., Liu, F., Wang, G. D., Lyu, H. L., Wu, R. R., et al. (2016). Patients with first-episode, drug-naive schizophrenia and subjects at ultra-high risk of psychosis shared increased cerebellar-default mode network connectivity at rest. Sci. Rep. 6. doi: 10.1038/srep26124
Wang, D., Zhou, Y., Zhuo, C., Qin, W., Zhu, J., Liu, H., et al. (2015). Altered functional connectivity of the cingulate subregions in schizophrenia. Transl. Psychiatry 5:e575. doi: 10.1038/tp.2015.69
Whitford, T. J., Farrow, T. F. D., Williams, L. M., Gomes, L., Brennan, J., and Harris, A. W. F. (2009). Delusions and dorso-medial frontal cortex volume in first-episode schizophrenia: a voxel-based morphometry study. Psychiatry Res. Neuroimaging 172, 175–179. doi: 10.1016/j.pscychresns.2008.07.011
Wolf, N. D., Sambataro, F., Vasic, N., Frasch, K., Schmid, M., Schonfeldt-Lecuona, C., et al. (2011). Dysconnectivity of multiple resting-state networks in patients with schizophrenia who have persistent auditory verbal hallucinations. J. Psychiatry Neurosci. 36, 366–374. doi: 10.1503/jpn.110008
Wotruba, D., Michels, L., Buechler, R., Metzler, S., Theodoridou, A., Gerstenberg, M., et al. (2014). Aberrant coupling within and across the default mode, task-positive, and salience network in subjects at risk for psychosis. Schizophr. Bull. 40, 1095–1104. doi: 10.1093/schbul/sbt161
Xi, Y. B., Guo, F., Liu, W. M., Fu, Y. F., Li, J. M., Wang, H. N., et al. (2021). Triple network hypothesis-related disrupted connections in schizophrenia: a spectral dynamic causal modeling analysis with functional magnetic resonance imaging. Schizophr. Res. 233, 89–96. doi: 10.1016/j.schres.2021.06.024
Xu, L., Qin, W., Zhuo, C., Zhu, J., Liu, H., Liu, X., et al. (2015). Selective functional disconnection of the dorsal subregion of the temporal pole in schizophrenia. Sci. Rep. 5:11258. doi: 10.1038/srep11258
Yan, C. G., Wang, X. D., Zuo, X. N., and Zang, Y. F. (2016). DPABI: Data Processing & Analysis for (resting-state) brain imaging. Neuroinformatics 14, 339–351. doi: 10.1007/s12021-016-9299-4
Yang, Z. Y., Zhang, R. T., Li, Y., Wang, Y., Wang, Y. M., Wang, S. K., et al. (2019). Functional connectivity of the default mode network is associated with prospection in schizophrenia patients and individuals with social anhedonia. Prog. Neuropsychopharmacol. Biol. Psychiatry 92, 412–420. doi: 10.1016/j.pnpbp.2019.02.008
Yeo, B. T., Krienen, F. M., Sepulcre, J., Sabuncu, M. R., Lashkari, D., Hollinshead, M., et al. (2011). The organization of the human cerebral cortex estimated by intrinsic functional connectivity. J. Neurophysiol. 106, 1125–1165. doi: 10.1152/jn.00338.2011
Zhou, Y., Ma, X., Wang, D., Qin, W., Zhu, J., Zhuo, C., et al. (2015). The selective impairment of resting-state functional connectivity of the lateral subregion of the frontal pole in schizophrenia. PLoS One 10:e0119176. doi: 10.1371/journal.pone.0119176
Zhuo, C., Zhu, J., Qin, W., Qu, H., Ma, X., Tian, H., et al. (2014). Functional connectivity density alterations in schizophrenia. Front. Behav. Neurosci. 8:404. doi: 10.3389/fnbeh.2014.00404
Keywords: persistent negative symptoms, functional connectivity, gray matter volume, default mode network, central executive network, salience network
Citation: Zhu T, Wang Z, Wu W, Ling Y, Wang Z, Zhou C, Fang X, Huang C, Xie C, Chen J and Zhang X (2023) Altered brain functional networks in schizophrenia with persistent negative symptoms: an activation likelihood estimation meta-analysis. Front. Hum. Neurosci. 17:1204632. doi: 10.3389/fnhum.2023.1204632
Edited by:
Chuanliang Han, Chinese Academy of Sciences (CAS), ChinaReviewed by:
Anna S. Huang, Vanderbilt University Medical Center, United StatesQingxia Yang, Nanjing University of Posts and Telecommunications, China
Bao-Liang Zhong, Wuhan Mental Health Center, China
Tianhong Zhang, Shanghai Jiao Tong University, China
Copyright © 2023 Zhu, Wang, Wu, Ling, Wang, Zhou, Fang, Huang, Xie, Chen and Zhang. This is an open-access article distributed under the terms of the Creative Commons Attribution License (CC BY). The use, distribution or reproduction in other forums is permitted, provided the original author(s) and the copyright owner(s) are credited and that the original publication in this journal is cited, in accordance with accepted academic practice. No use, distribution or reproduction is permitted which does not comply with these terms.
*Correspondence: Xiangrong Zhang, ZHJ4cnpAaG90bWFpbC5jb20=; Jiu Chen, ZXJpY2NzdEBhbGl5dW4uY29t
†These authors have contributed equally to this work and share first authorship