- 1Developmental Cognitive Neuroscience Lab, School of Medicine, Pontifical Catholic University of Rio Grande do Sul (PUCRS), Porto Alegre, Brazil
- 2Center for Medical Sciences, Federal University of Pernambuco, Recife, Brazil
Introduction: Electroconvulsive therapy (ECT) is one of the most effective strategies for treating resistant major depression. Although the mechanism of action is not fully understood and studies are limited, epigenetics is a promising area for the development of biomarkers associated with ECT treatment response.
Aim: We reviewed studies available in the literature that explored the epigenetics of ECT in peripheral samples from patients with major depressive disorder (MDD).
Methods: A systematic review was performed following The PRISMA guidelines. The search was performed in seven electronic databases: Scopus, Web of Science, Medline, PsycINFO, Embase, Cochrane, and Cinahl.
Results: Nine studies were included. Seven assessed DNA methylation and three investigated microRNAs (miR). Overall, most studies were exploratory, with small sample sizes, and we found high heterogeneity between the study’s design, ECT protocols, molecular biology methods, and epigenetic findings. Investigated candidates with some evidence of association with ECT treatment response were BDNF, S100A10, RNF213M, TNKS, FKBP5, miR-126, miR-106a, and miR-24.
Conclusion: The present findings seem to support previous preclinical research, suggesting that epigenetic mechanisms play an important role in the molecular mechanism underlying ECT effects.
1. Introduction
Electroconvulsive therapy (ECT) is one of the most effective options for the treatment of pharmacoresistant major depressive disorder (MDD), bipolar disorder that has not responded to other treatments, suicidal behavior, severe agitation, and to manage catatonia and clozapine-resistant schizophrenia (Tor et al., 2021). MDD is one of the most prevalent mental disorders across the world, potentially life-threatening when left untreated. Currently, MDD affects more than 322 million individuals, imposing a considerable economic cost on society (Gutiérrez-Rojas et al., 2020). In addition to the mortality associated with suicidality, the chronic nature of depression contributes substantially to the development of cardiovascular and metabolic illness, increasing the global burden of disease and disability (Sousa et al., 2022).
Deciphering the pathophysiology of depression is a complex phenomenon. Depressive syndromes are not homogeneous, are multidimensional and have multiple etiologies. Treatment with antidepressant drugs is ineffective for 30–50% of these patients and, when effective, it has a delayed onset and considerable side effects (Gaynes et al., 2018). The efficacy of ECT is on average three times greater than pharmacotherapy, offering up to 60% remission of symptoms among patients with MDD, and the therapeutic response is usually faster than existing psychotropic medications (Rhee et al., 2022) However, its therapeutic mechanism of action has not yet been fully elucidated.
Currently, the main findings referring to the mechanism and neurobiological aspects are related to structural, functional and compositional changes in the brain. Based on neuroimaging data, the brain networks involved with ECT include the default-mode network, saliency network, dorsal-attention network, and central-executive network (Wang et al., 2018). These brain networks appear to be valuable biomarkers for assessing the underlying neurobiology of the therapeutic effects of ECT. In addition, decades of research in animal models suggest that ECT exerts its effects through biochemical and molecular changes, including changes in the expression of genes that encode for various transcription factors, neurotrophins and structural proteins, as well as the alteration in cerebral blood flow, blood-brain barrier, neurotransmitters and neuroinflammatory signaling (Réus et al., 2019). This suggests that peripheral molecular markers also possess the potential to elucidate the mechanisms underlying ECT.
Epigenetic modifications such as DNA methylation, histone alterations, and the functional role of non-coding RNAs are potentially reversible molecular processes that can induce lasting changes in gene expression (Bredy et al., 2010). This is particularly relevant considering ECT effects, once epigenetic mechanisms might be rapid and long-lasting, providing fine-tune regulation of experience-induced gene expression (Dudley et al., 2011; Baker-Andresen et al., 2013). However, the current evidence explaining the possible role of ECT in modifying epigenetic mechanisms with therapeutic effects is mostly restricted to preclinical studies (Jiang et al., 2017). Furthermore, little is known about the implications of ECT on the epigenetic machinery in patients with MDD. Identifying reliable molecular biomarkers that could predict response to ECT would significantly reduce costs and identify patients eligible for first-line ECT treatment. Hence, we performed a systematic mini-review of the literature regarding ECT and epigenetics, focusing entirely on human studies.
2. Materials and methods
This review was performed following The PRISMA guidelines (Moher et al., 2009). The research question was: Is electroconvulsive therapy associated with changes in epigenetic markers? For this, on 17 December 2020, we used the following descriptors (MiRNAs OR microRNAs OR epigenetic OR epigenomic OR epigenomics OR DNA methylation OR 5 mC OR DNA hydroxymethylation OR 5 hmC OR histone acetylation OR histone deacetylation OR histone methylation OR long non-coding RNA OR lincRNA OR epigenetic process) AND (electroconvulsive OR ECT OR electroconvulsive therapy) to search in seven electronic databases: Scopus, Web of Science, Medline, PsycINFO, Embase, Cochrane, and Cinahl.
After duplicate removal, three independent researchers screened search records by title and abstract, using the Rayyan QCRI software (Ouzzani et al., 2016). The inclusion criteria were: studies with samples of men/women between 18 and 65 years old, with MDD and psychiatric indication for treatment with ECT; studies who had any epigenetic investigation associated with ECT. Observational studies randomized controlled trials (RCT), cross-sectional, and case-control studies were considered; while qualitative studies, animal models, summaries, book chapters, literature reviews, or studies that did not present any epigenetic investigation were excluded. Occasional divergence in selection was resolved by consensus with the help of our mentor.
Full-text studies were evaluated in detail in terms of the objective, hypothesis, and study design. From a methodological perspective, samples were characterized (sample size, psychiatric diagnosis, etc.) and the clinical scales used to diagnose patients were also evaluated. Mechanical and functional scrutiny of the ECT protocol was carried out, seeking to specify the duration (number of sessions), frequency (number of weekly or monthly sessions), equipment used (type of device), applied anesthesia and electrical stimulation, unilateral or bilateral, lower/higher dosages. Regarding epigenetic outcomes, we focused mainly on molecular biology methods (analyses and extraction of DNA or RNA) and on which epigenetic markers were evaluated (DNA modifications, non-coding RNA, etc.).
3. Results
3.1. Characteristics of studies
The nine reviewed studies combined for a sample of 358 participants with MDD (mean clinical sample size = 39). Figure 1 displays the flowchart of the screening and eligibility process. Characteristics of studies are presented in Table 1. Studies were published between 2014 and 2022.
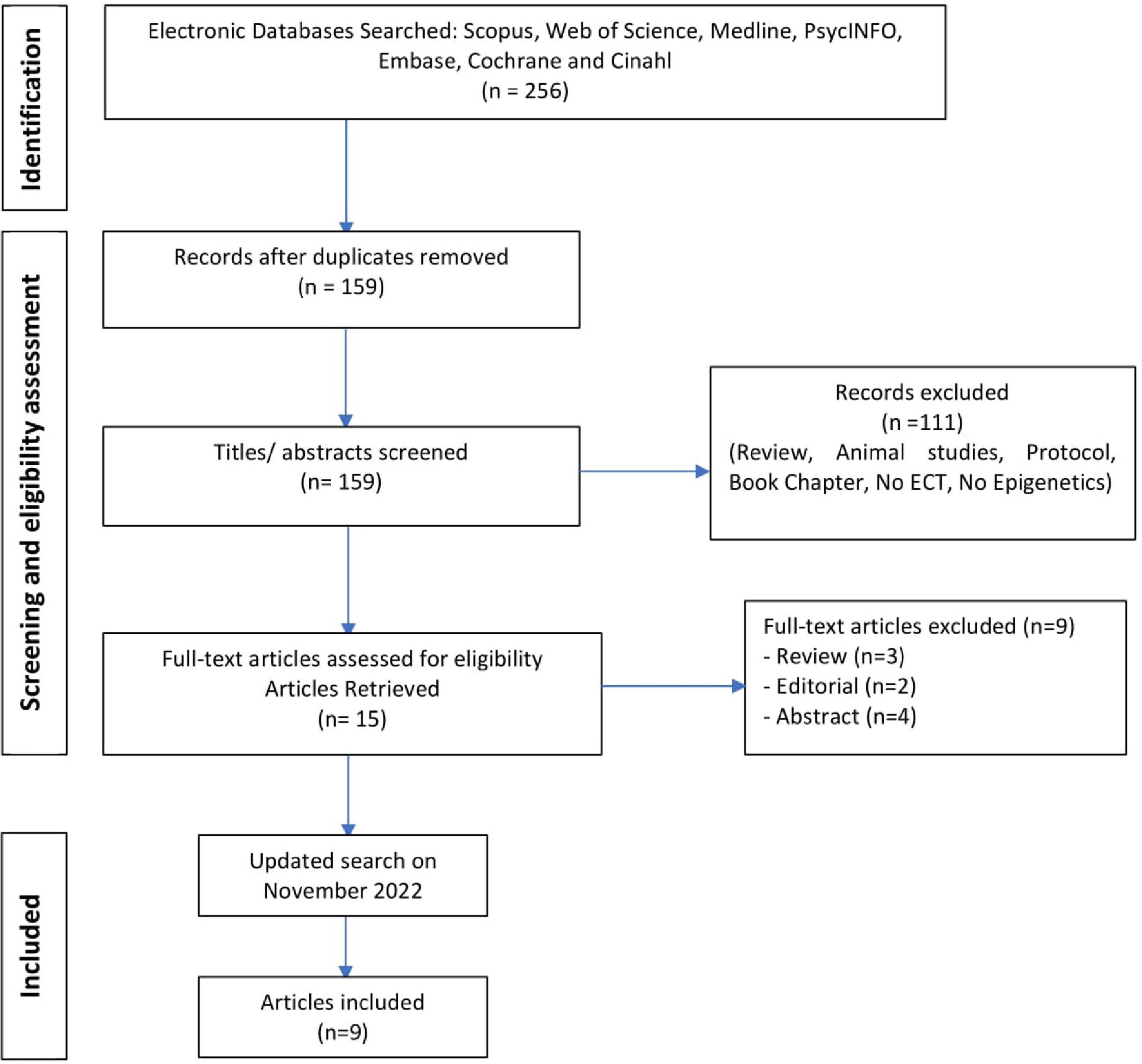
Figure 1. Preferred Reporting Items for Systematic Reviews (PRISMA) Flow Diagram. The detailed process for selecting articles evaluating the association between epigenetic processes and electroconvulsive therapy (ECT) in humans.
All were performed with patients with MDD (Kolshus et al., 2017; Neyazi et al., 2018; Moschny et al., 2020a), while six studies referred to cases of treatment-resistant depression (TRD) (Kleimann et al., 2015; Gururajan et al., 2016; Moschny et al., 2020b; Israel-Elgali et al., 2021; Sirignano et al., 2021; Schurgers et al., 2022). They were all observational studies of a cohort of patients enrolled in a clinical trial with ECT, and with the evaluation of longitudinal biomarkers. Two studies also evaluated epigenetic changes induced by ketamine in addition to ECT (Gururajan et al., 2016; Sirignano et al., 2021). Some studies compared patients who improved from depression after ECT with those who did not improve (Kleimann et al., 2015; Kolshus et al., 2017; Moschny et al., 2020b; Sirignano et al., 2021), while some studies compared longitudinal changes before and after ECT treatment (Gururajan et al., 2016; Neyazi et al., 2018; Moschny et al., 2020a; Israel-Elgali et al., 2021; Schurgers et al., 2022).
Heterogeneity was found regarding ECT protocols. Treatment lasted from 2 to 6 weeks. The frequency was one to three times a week. Regarding the ECT method, brief-pulse was the most used method. The devices used were Spectrum Mecta® 5000 (Kolshus et al., 2017; Moschny et al., 2020a), and Thymatron® System IV (Kleimann et al., 2015; Moschny et al., 2020b; Israel-Elgali et al., 2021; Schurgers et al., 2022). Most studies reported the usage of short-acting anesthetics. The application of the electrical stimulus was unilateral (Moschny et al., 2020b) or bitemporal (Gururajan et al., 2016; Schurgers et al., 2022). Psychiatric pharmacotherapy is presented in detail in Table 1.
3.2. Effects of ECT on microRNAs
Epigenetic findings of studies are summarized in Table 1. MicroRNAs (miRNAs) are a class of non-coding RNAs, averaging 22 nucleotides in length, that play important roles in regulating gene expression (Jonas and Izaurralde, 2015). These small molecules accomplish essential post-transcriptional regulatory steps of gene expression through either the degradation of an mRNA transcript or the inhibition of translation (He and Hannon, 2004). Both in the peripheral and central nervous systems, miRNAs are considered potential biomarkers in the diagnosis of MDD and in monitoring therapeutic responses (Šalamon Arèan et al., 2022). They regulate key processes in the central nervous system including neuronal development, neurogenesis, and synaptic plasticity, while in the periphery they are implicated with immune and endocrine regulation, for instance.
Three studies assessed miRNAs in peripheral blood or peripheral blood mononuclear cells (PBMCs). Gururajan et al. (2016) investigated by microarray the expression of several blood miRNAs at 2 different time points, before and after ECT. In addition, they assessed miRNAs in healthy controls. Pre-ECT expression of let-7b and let-7c was lower in TRD patients compared to controls. However, pre- and post-treatment levels were similar, and baseline expression of these miRNAs could not predict ECT treatment response. These results suggest that let-7b and let-7c miRNAs may be useful as diagnostic biomarkers of TRD, but not of ECT. Kolshus et al. (2017) performed a transcriptomic analysis through RNA-sequencing of miRNAs in the blood of patients after ECT, finding miR-130a-3p/5p, miR-126-3p/5p, miR-106a-5p, and miR-942-3p as potential targets associated with ECT response, but these findings were not confirmed in the validation study in a larger sample. Interestingly, in the validation study, they found that miR-126-3p and miR-106a-5p were significantly elevated in patients with psychotic depression in baseline when compared to healthy controls. However, after ECT the levels of these miRNAs normalized. Finally, Israel-Elgali et al. (2021) analyzed RNA samples extracted from PBMCs from patients with TRD submitted to ECT. By using NanoString technology and assessing hundreds of miRNAs, they found no statistically significant changes in miRNAs as a result of ECT.
3.3. Effects of ECT on DNA methylation
DNA methylation is a major epigenetic mechanism that regulates gene expression by recruiting proteins involved in gene repression or by inhibiting the binding of transcription factors to DNA. This epigenetic mechanism involves the transfer of a methyl group onto the C5 position of the cytosine to form 5-methylcytosine, frequently occurring on cytosines followed by a guanine nucleotide, known as CpG sites (Moore et al., 2013). The methylation of nucleobases can change the functionality of a segment of DNA without altering the sequence. Studies have shown altered DNA methylation patterns associated with MDD and antidepressants (Webb et al., 2020; Šalamon Arèan et al., 2022).
Six studies evaluated the relationship between DNA methylation and the effects of ECT (Kleimann et al., 2015, Neyazi et al., 2018; Moschny et al., 2020a,b; Sirignano et al., 2021; Schurgers et al., 2022). DNA samples were extracted from peripheral blood in all studies. Kleimann et al. (2015) investigated the methylation levels of the BDNF gene promoter in 11 TRD patients before and during ECT sessions. Responders had lower methylation rates, especially in the exon I promoter of BDNF. In addition, Schurgers et al. (2022) found that the gene expression of BDNF, ERK1, and NR3C1, in blood samples of TRD patients, significantly increased over time during ECT treatment. For these 3 genes changes, expression levels were highly correlated with changes in DNA methylation levels for several CpG sites, including negative correlations between mRNA levels of BDNF and methylation (i.e., hypomethylation), particularly in 3 CpGs (cg15462887, cg27351358, cg23426002). This suggests some overlap between the findings of Schurgers and Kleimann’s studies, at least regarding BDNF demethylation throughout ECT treatment. Neyazi et al. (2018) assessed DNA methylation of the p11 promoter, also known as the S100A10 gene. The protein coded is multifunctional, and previous evidence suggests a link between depressive states, and response to antidepressant treatment, with peripheral p11 levels (Chen et al., 2022; Gałecka et al., 2022). Interestingly, they found higher S100A10 promoter DNA methylation in responders to ECT. Moschny et al. (2020b), on the other hand, investigated DNA methylation of the promoter regions of the genes PLAT and SERPINE1, finding no significant differences between baseline and post-ECT DNA methylation levels after controlling for potential confounders.
Different from the aforementioned studies that used candidate gene approaches, Moschny et al. (2020a) identified 5 genes (RNF175, RNF213, TBC1D14, TMC5, and WSCD1) and 3 non-coding RNAs (AC018685.2, AC098617.1, and CLCN3P1), differentially methylated, using a genome-wide approach in samples of TRD patients undergoing ECT. The highest effect sizes were found in four CpG sites located in the RNF213 gene in the group that responded to ECT. Finally, Sirignano et al. (2021), examined treatment-associated changes in DNA methylation levels in 34 patients across the epigenome. Among the 20 genes with a significant effect, TNKS and FKBP5 were the only differentially methylated genes with a p-value below 10–7, highlighting their potential for future investigations.
4. Discussion
ECT was introduced more than 80 years ago and was one of the early biological treatments for mental disorders. Epigenetics is one of the areas that can help to better understand the molecular processes associated with ECT action. Genes are regulated through the activity of epigenetic mechanisms, serving to turn up or turn down levels of gene expression in response to rapidly changing stimuli, such as ECT. In this review, we included nine studies that investigated either miRNAs or DNA methylation patterns related to ECT in peripheral samples of MDD patients. Overall, most studies were exploratory, with small sample sizes, and we found high heterogeneity between the study’s design, ECT protocols, molecular biology methods, and epigenetic findings.
DNA methylation was interrogated in 6 studies. Four of them had a candidate gene approach (Kleimann et al., 2015; Neyazi et al., 2018; Moschny et al., 2020b; Schurgers et al., 2022), focusing on genes that have been frequently associated with the molecular pathophysiology of MDD, such as BDNF and NR3C1. Although preliminary and without overlap regarding the assessed CpGs on DNA sequence, Kleimann et al. (2015) and Schurgers et al. (2022) presented evidence suggesting that ECT response is associated with a demethylation pattern of BDNF (e.g., exon 1 promoter). If considering that demethylation might be associated with higher gene expression, such as partially showed by Schurgers et al. (2022), these findings are promising. Particularly, enhancement of the BDNF signaling through epigenetic regulation and increased gene expression has been suggested to be a molecular mechanism of action of conventional antidepressants (Cubillos et al., 2022). However, it should be noted that the 2 methylome studies included in our review (Moschny et al., 2020a; Sirignano et al., 2021), did not find BDNF as a significantly differentially methylated gene regarding ECT. Similarly, a hypermethylation pattern of the S100A10 promoter in responders to ECT was found at the level of targeted genes by Neyazi et al. (2018), but this was not confirmed by the methylome studies. Hence, when analyzing the findings of the 2 genome-wide DNA methylation studies included in our review, several promising hits were discovered. By applying the Illumina Infinium Methylation EPIC Array, or the Methyl Capture EPIC Next-generation sequencing, these studies analyzed thousands of CpG sites across the genome. Therefore, Moschny et al. (2020a) identified non-coding RNAs, in addition to protein-coding genes, as potential genomic regions with DNA methylation patterns associated with ECT treatment response. In addition, Sirignano et al. (2021) found FKBP5 as one out of two differentially methylated genes with a stringent statistical significance threshold associated with ECT. FKBP5 is a well-known gene involved in neuroendocrine signaling associated with MDD, supported by both genetic and epigenetic evidence (Alshaya, 2022). Changes in FKBP5 gene expression in the blood are also associated with ECT treatment response (Israel-Elgali et al., 2021). Although the findings of these genome-wide studies require further validation, it seems clear that unbiased methods of epigenetic investigation are the most promising strategies for revealing ECT molecular biomarkers.
In this sense, all studies that assessed the relationship between ECT and miRNAs performed transcriptomic analyses, not focusing entirely on candidate miRNAs. Therefore, several miRNAs emerged as potential biomarkers of ECT response, requiring further validation. The most relevant considering statistical significance thresholds and longitudinal changes pre- and post-ECT treatment are miR-126, miR-106a, and miR-24. These 3 miRNAs have already been associated with depressive disorders, by evidence showing altered levels in peripheral blood samples of patients with MDD (Camkurt et al., 2020; Maffioletti et al., 2021; Roumans et al., 2021). It seems that ECT treatment response is associated with a pattern of normalization of their expression in blood samples, which are altered by MDD in the pre-ECT period. The mRNA molecules that these miRNAs target and regulate post-transcriptionally as a function of ECT still need to be investigated.
This review has limitations that should be discussed. First, we only included nine studies, showing that the relationship between ECT and epigenetics is still lacking robust evidence when investigated in humans. Second, most studies were performed with small sample sizes, and their findings need validation in larger samples. This is particularly relevant for genome-wide studies since they usually need high statistical power and larger sample sizes to deal with stringent false discovery rate, as well as with cell-type deconvolution algorithms. Importantly, cell-type-specific data is critical for the proper interpretation of epigenetic findings (Avila Cobos et al., 2020). Third, although DNA methylation and miRNA transcriptional levels were assessed mostly in whole blood samples, ECT is applied in the brain, suggesting that peripheral molecular mechanisms may not overlap with central ones. Fourth, the possible effects of anesthesia and pharmacotherapy are potential confounders, especially in severely depressed patients receiving multiple antidepressant regimens. Few studies have explored the relationship with pharmacotherapy, except for those investigating ketamine versus ECT (Gururajan et al., 2016; Sirignano et al., 2021). Finally, another important group of epigenetic modifications, and for which we found no study, is the covalent post-translational modification of histone proteins. Evidence suggesting that histone modifications are part of the epigenetic landscape associated with ECT effects is still restricted to animal studies (Pusalkar et al., 2016).
Despite these limitations, the present findings seem to support previous preclinical research (Dyrvig et al., 2015), suggesting that epigenetic mechanisms play an important role in the molecular mechanism underlying ECT effects. With changes in the public perception of ECT and more funding for this area of research, these preliminary findings could be deeper explored and validated by future research.
Author contributions
SC, CB, BB, and SA performed all steps of the systematic review, identification, screening, eligibility, and data extraction. TV supervised all steps, solved any discrepancy, and reviewed the manuscript. SC was the major contributor in writing, while CB, BB, SA, and TV also assisted in writing. All authors contributed to the article and approved the submitted version.
Funding
CB has been supported by the Brazilian Coordination for the Improvement of Higher Education Personnel (CAPES), with a Ph.D. scholarship.
Conflict of interest
The authors declare that the research was conducted in the absence of any commercial or financial relationships that could be construed as a potential conflict of interest.
Publisher’s note
All claims expressed in this article are solely those of the authors and do not necessarily represent those of their affiliated organizations, or those of the publisher, the editors and the reviewers. Any product that may be evaluated in this article, or claim that may be made by its manufacturer, is not guaranteed or endorsed by the publisher.
References
Alshaya, D. S. (2022). Genetic and epigenetic factors associated with depression: an updated overview. Saudi J. Biol. Sci. 29:103311. doi: 10.1016/j.sjbs.2022.103311
Avila Cobos, F., Alquicira-Hernandez, J., Powell, J. E., Mestdagh, P., and De Preter, K. (2020). Benchmarking of cell type deconvolution pipelines for transcriptomics data. Nat. Commun. 11:5650. doi: 10.1038/s41467-020-19015-1
Baker-Andresen, D., Ratnu, V. S., and Bredy, T. W. (2013). Dynamic DNA methylation: a prime candidate for genomic metaplasticity and behavioral adaptation. Trends Neurosci. 36, 3–13. doi: 10.1016/j.tins.2012.09.003
Bredy, T. W., Sun, Y. E., and Kobor, M. S. (2010). How the epigenome contributes to the development of psychiatric disorders. Dev. Psychobiol. 52, 331–342. doi: 10.1002/dev.20424
Camkurt, M. A., Karababa, İ, Erdal, M. E., Kandemir, S. B., Fries, G. R., Bayazıt, H., et al. (2020). MicroRNA dysregulation in manic and euthymic patients with bipolar disorder. J. Affect. Disord. 261, 84–90. doi: 10.1016/j.jad.2019.09.060
Chen, M. X., Oh, Y. S., and Kim, Y. (2022). S100A10 and its binding partners in depression and antidepressant actions. Front. Mol. Neurosci. 15:953066. doi: 10.3389/fnmol.2022.953066
Cubillos, S., Engmann, O., and Brancato, A. (2022). BDNF as a mediator of antidepressant response: recent advances and lifestyle interactions. Int. J. Mol. Sci. 23:14445. doi: 10.3390/ijms232214445
Dudley, K. J., Li, X., Kobor, M. S., Kippin, T. E., and Bredy, T. W. (2011). Epigenetic mechanisms mediating vulnerability and resilience to psychiatric disorders. Neurosci. Biobehav. Rev. 35, 1544–1551. doi: 10.1016/j.neubiorev.2010.12.016
Dyrvig, M., Gøtzsche, C. R., Woldbye, D. P., and Lichota, J. (2015). Epigenetic regulation of Dnmt3a and Arc gene expression after electroconvulsive stimulation in the rat. Mol. Cell Neurosci. 67, 137–143. doi: 10.1016/j.mcn.2015.06.011
Gałecka, M., Bliźniewska-Kowalska, K., Gałecki, P., Szemraj, J., and Orzechowska, A. (2022). Expression of p11 in patients with depression. J. Clin. Med. 11:5743. doi: 10.3390/jcm11195743
Gaynes, B. N., Asher, G., Gartlehner, G., Hoffman, V., Green, J., Boland, E., et al. (2018). Definition of treatment-resistant depression in the medicare population. Rockville, MD: Agency for Healthcare Research and Quality.
Gururajan, A., Naughton, M. E., Scott, K. A., O’Connor, R. M., Moloney, G., Clarke, G., et al. (2016). MicroRNAs as biomarkers for major depression: a role for let-7b and let-7c. Transl. Psychiatry 6:e862. doi: 10.1038/tp.2016.131
Gutiérrez-Rojas, L., Porras-Segovia, A., Dunne, H., Andrade-González, N., and Cervilla, J. A. (2020). Prevalence and correlates of major depressive disorder: a systematic review. Braz. J. Psychiatry 42, 657–672. doi: 10.1590/1516-4446-2020-0650
He, L., and Hannon, G. J. (2004). MicroRNAs: small RNAs with a big role in gene regulation. Nat. Rev. Genet. 5, 522–531. doi: 10.1038/nrg1379
Israel-Elgali, I., Hertzberg, L., Shapira, G., Segev, A., Krieger, I., Nitzan, U., et al. (2021). Blood transcriptional response to treatment-resistant depression during electroconvulsive therapy. J. Psychiatr. Res. 141, 92–103. doi: 10.1016/j.jpsychires.2021.06.039
Jiang, J., Wang, J., and Li, C. (2017). Potential mechanisms underlying the therapeutic effects of electroconvulsive therapy. Neurosci. Bull. 33, 339–347. doi: 10.1007/s12264-016-0094-x
Jonas, S., and Izaurralde, E. (2015). Towards a molecular understanding of microRNA-mediated gene silencing. Nat. Rev. Genet. 16, 421–433. doi: 10.1038/nrg3965
Kleimann, A., Kotsiari, A., Sperling, W., Gröschl, M., Heberlein, A., Kahl, K. G., et al. (2015). BDNF serum levels and promoter methylation of BDNF exon I, IV and VI in depressed patients receiving electroconvulsive therapy. J. Neural Transm. 122, 925–928. doi: 10.1007/s00702-014-1336-6
Kolshus, E., Ryan, K. M., Blackshields, G., Smyth, P., Sheils, O., and McLoughlin, D. M. (2017). Peripheral blood microRNA and VEGFA mRNA changes following electroconvulsive therapy: implications for psychotic depression. Acta Psychiatr. Scand. 136, 594–606. doi: 10.1111/acps.12821
Maffioletti, E., Bocchio-Chiavetto, L., Perusi, G., Carvalho Silva, R., Sacco, C., Bazzanella, R., et al. (2021). Inflammation-related microRNAs are involved in stressful life events exposure and in trauma-focused psychotherapy in treatment-resistant depressed patients. Eur. J. Psychotraumatol. 12:1987655. doi: 10.1080/20008198.2021.1987655
Moher, D., Liberati, A., Tetzlaff, J., Altman, D. G., and PRISMA Group (2009). Preferred reporting items for systematic reviews and meta-analyses: the PRISMA statement. PLoS Med. 6:e1000097. doi: 10.1371/journal.pmed.1000097
Moore, L. D., Le, T., and Fan, G. (2013). DNA methylation and its basic function. Neuropsychopharmacology 38, 23–38. doi: 10.1038/npp.2012.112
Moschny, N., Jahn, K., Bajbouj, M., Maier, H. B., Ballmaier, M., Khan, A. Q., et al. (2020a). DNA methylation of the t-PA gene differs between various immune cell subtypes isolated from depressed patients receiving electroconvulsive therapy. Front. Psychiatry 11:571. doi: 10.3389/fpsyt.2020.00571
Moschny, N., Zindler, T., Jahn, K., Dorda, M., Davenport, C. F., Wiehlmann, L., et al. (2020b). Novel candidate genes for ECT response prediction-a pilot study analyzing the DNA methylome of depressed patients receiving electroconvulsive therapy. Clin. Epigenetics 12:114. doi: 10.1186/s13148-020-00891-9
Neyazi, A., Theilmann, W., Brandt, C., Rantamäki, T., Matsui, N., Rhein, M., et al. (2018). P11 promoter methylation predicts the antidepressant effect of electroconvulsive therapy. Transl. Psychiatry 8:25. doi: 10.1038/s41398-017-0077-3
Ouzzani, M., Hammady, H., Fedorowicz, Z., and Elmagarmid, A. (2016). Rayyan-a web and mobile app for systematic reviews. Syst. Rev. 5:210. doi: 10.1186/s13643-016-0384-4
Pusalkar, M., Ghosh, S., Jaggar, M., Husain, B. F., Galande, S., and Vaidya, V. A. (2016). Acute and chronic electroconvulsive seizures (ECS) differentially regulate the expression of epigenetic machinery in the adult rat hippocampus. Int. J. Neuropsychopharmacol. 19:yw040. doi: 10.1093/ijnp/pyw040
Réus, G. Z., de Moura, A. B., Borba, L. A., Abelaira, H. M., and Quevedo, J. (2019). Strategies for treatment-resistant depression: lessons learned from animal models. Mol. Neuropsychiatry 5, 178–189. doi: 10.1159/000500324
Rhee, T. G., Shim, S. R., Forester, B. P., Nierenberg, A. A., McIntyre, R. S., Papakostas, G. I., et al. (2022). Efficacy and safety of ketamine vs electroconvulsive therapy among patients with major depressive episode: a systematic review and meta-analysis. JAMA Psychiatry 79, 1162–1172. doi: 10.1001/jamapsychiatry.2022.3352
Roumans, S., Sundquist, K., Memon, A. A., Hedelius, A., Sundquist, J., and Wang, X. (2021). Association of circulating let-7b-5p with major depressive disorder: a nested case-control study. BMC Psychiatry 21:616. doi: 10.1186/s12888-021-03621-4
Šalamon Arčan, I., Kouter, K., and Videtič Paska, A. (2022). Depressive disorder and antidepressants from an epigenetic point of view. World J. Psychiatry 12, 1150–1168. doi: 10.5498/wjp.v12.i9.1150
Schurgers, G., Walter, S., Pishva, E., Guloksuz, S., Peerbooms, O., Incio, L. R., et al. (2022). Longitudinal alterations in mRNA expression of the BDNF neurotrophin signaling cascade in blood correlate with changes in depression scores in patients undergoing electroconvulsive therapy. Eur. Neuropsychopharmacol. 63, 60–70. doi: 10.1016/j.euroneuro.2022.07.183
Sirignano, L., Frank, J., Kranaster, L., Witt, S. H., Streit, F., Zillich, L., et al. (2021). Methylome-wide change associated with response to electroconvulsive therapy in depressed patients. Transl. Psychiatry 11:347. doi: 10.1038/s41398-021-01474-9
Sousa, R. D., Gouveia, M., Nunes da Silva, C., Rodrigues, A. M., Cardoso, G., Antunes, A. F., et al. (2022). Treatment-resistant depression and major depression with suicide risk-The cost of illness and burden of disease. Front. Public Health 10:898491. doi: 10.3389/fpubh.2022.898491
Tor, P. C., Tan, X. W., Martin, D., and Loo, C. (2021). Comparative outcomes in electroconvulsive therapy (ECT): A naturalistic comparison between outcomes in psychosis, mania, depression, psychotic depression and catatonia. Eur. Neuropsychopharmacol. 51, 43–54. doi: 10.1016/j.euroneuro.2021.04.023
Wang, J., Wei, Q., Wang, L., Zhang, H., Bai, T., Cheng, L., et al. (2018). Functional reorganization of intra- and internetwork connectivity in major depressive disorder after electroconvulsive therapy. Hum. Brain Mapp. 39, 1403–1411. doi: 10.1002/hbm.23928
Keywords: depression, epigenetics, biomarkers, electroconvulsive therapy (ECT), major depression (MD)
Citation: Castro SCC, Bicca C, Bicca B, Araujo S and Viola TW (2023) A systematic mini-review of epigenetic mechanisms associated with electroconvulsive therapy in humans. Front. Hum. Neurosci. 17:1143332. doi: 10.3389/fnhum.2023.1143332
Received: 12 January 2023; Accepted: 06 February 2023;
Published: 09 March 2023.
Edited by:
Ryouhei Ishii, Osaka Metropolitan University, JapanReviewed by:
Jacob Peedicayil, Christian Medical College & Hospital, IndiaEthan Bryson, Icahn School of Medicine at Mount Sinai, United States
Copyright © 2023 Castro, Bicca, Bicca, Araujo and Viola. This is an open-access article distributed under the terms of the Creative Commons Attribution License (CC BY). The use, distribution or reproduction in other forums is permitted, provided the original author(s) and the copyright owner(s) are credited and that the original publication in this journal is cited, in accordance with accepted academic practice. No use, distribution or reproduction is permitted which does not comply with these terms.
*Correspondence: Thiago Wendt Viola, dGhpYWdvLndlbmR0QHB1Y3JzLmJy