- 1Department of Neurosurgery, University of Colorado Anschutz Medical Campus, Aurora, CO, United States
- 2Department of Neurosurgery, Medical University of South Carolina, Charleston, SC, United States
- 3Department of Psychiatry and Behavioral Sciences, Medical University of South Carolina, Charleston, SC, United States
Non-invasive brain stimulation is designed to target accessible brain regions that underlie many psychiatric disorders. One such method, transcranial magnetic stimulation (TMS), is commonly used in patients with treatment-resistant depression (TRD). However, for non-responders, the choice of an alternative therapy is unclear and often decided empirically without detailed knowledge of precise circuit dysfunction. This is also true of invasive therapies, such as deep brain stimulation (DBS), in which responses in TRD patients are linked to circuit activity that varies in each individual. If the functional networks affected by these approaches were better understood, a theoretical basis for selection of interventions could be developed to guide psychiatric treatment pathways. The mechanistic understanding of TMS is that it promotes long-term potentiation of cortical targets, such as dorsolateral prefrontal cortex (DLPFC), which are attenuated in depression. DLPFC is highly interconnected with other networks related to mood and cognition, thus TMS likely alters activity remote from DLPFC, such as in the central executive, salience and default mode networks. When deeper structures such as subcallosal cingulate cortex (SCC) are targeted using DBS for TRD, response efficacy has depended on proximity to white matter pathways that similarly engage emotion regulation and reward. Many have begun to question whether these networks, targeted by different modalities, overlap or are, in fact, the same. A major goal of current functional and structural imaging in patients with TRD is to elucidate neuromodulatory effects on the aforementioned networks so that treatment of intractable psychiatric conditions may become more predictable and targeted using the optimal technique with fewer iterations. Here, we describe several therapeutic approaches to TRD and review clinical studies of functional imaging and tractography that identify the diverse loci of modulation. We discuss differentiating factors associated with responders and non-responders to these stimulation modalities, with a focus on mechanisms of action for non-invasive and intracranial stimulation modalities. We advance the hypothesis that non-invasive and invasive neuromodulation approaches for TRD are likely impacting shared networks and critical nodes important for alleviating symptoms associated with this disorder. We close by describing a therapeutic framework that leverages personalized connectome-guided target identification for a stepwise neuromodulation paradigm.
Introduction
Treatment-resistant depression (TRD) is defined as major depressive disorder (MDD) unresponsive to one or more conscripted treatments (Souery et al., 2006). Estimated failure rates of MDD treatment are as high as 10–30%, however, the type and number of treatments as well as the length of administration required for TRD diagnosis are inconsistently defined in the literature (Al-Harbi, 2012). Only 30% of TRD patients ever achieve remission using other means, and another 30% attempt suicide at least once in their lifetime (Bergfeld et al., 2018; Cole et al., 2022).
Measuring connectivity of brain networks for psychiatric disorders has become an increasingly used analytical technique, because it has the potential to illuminate neuromodulation targets for both non-invasive, e.g., TMS and invasive, e.g., DBS, approaches (Hollunder et al., 2022). This is especially critical since both modalities have considerable drawbacks. TMS for TRD requires up to 6 weeks of daily treatment sessions, and DBS may not show consistent efficacy in some patients until a year or more after implantation. Thus, an algorithm that helps clinicians identify and define TRD subtypes and suggests patient-specific treatments is needed.
Though many neural networks exhibit dysfunction in the setting of TRD, a close reading of the psychiatric literature consistently correlates default mode, salience, and central executive network dysfunction with TRD presentation and may suggest etiology (Hamilton et al., 2013). In contrast, a close reading of the neurosurgical literature for TRD highlights cortico-basal ganglia limbic circuits as well as cognitive control, reward and other networks that might be targeted based on specific symptom domains, including affect and attention (Williams et al., 2021). Compounding the problem of separate taxonomies in the literature is the fact that there is not enough crosstalk between the two disciplines, threatening to extend the semantic confusion surrounding these topics. Fortunately, the overall impression from examining these two bodies of literature is that the structures and networks described are very similar, if not the same, being targeted using these different approaches. As an example, functional connectivity studies show that DLPFC and subcallosal cingulate cortex (SCC), the two most common targets of TMS and DBS, respectively, are co-activated during effective treatment of TRD patients (Anderson et al., 2016). The implication is that a convergence of network anatomy and connectivity relationships among various neuromodulation treatments for TRD are needed to highlight similarities between them and advance care of TRD patients in a meaningful way.
In this review, we discuss the evidence accumulated in favor of pursuing a neuromodulatory approach for TRD. Putative mechanisms for both TMS and DBS are reviewed as well as an alternative procedure, epidural cortical stimulation, that likely engages similar circuitry. We conclude with a synthesis of network topology and argue that this may form the basis for developing a stepwise interventional approach to TRD, increasing in invasiveness only when a less invasive approach fails. In summary, much more work is needed to define clinical outcomes associated with a network-centric approach to treating TRD, however, this paper lays the important groundwork for consolidating the concepts and terminology for future trans-disciplinary discourse and action.
Neurobiology of transcranial magnetic stimulation
TMS is a non-invasive modality that has been FDA-approved in the United States for the management of treatment-resistant depression, smoking cessation and obsessive compulsive disorder, and is being investigated for numerous other psychiatric disorders such as addiction, chronic pain, anxiety, panic, and post-traumatic stress disorder (Cohen et al., 2022). Delivery of therapy is accomplished using a pulsatile electromagnetic field induced in a coil held at the surface of the scalp that stimulates underlying cortex (Barker et al., 1985). Electrophysiological simulations and patch-clamp studies suggest TMS-induced action potentials occur at or near somata rather than the axon hillock, the axon itself, or dendrites (Pashut et al., 2011). Computational modeling has shown that fibers running parallel to the surface of the scalp are more likely to be stimulated compared to oblique fibers, and vertical fibers are most resistant to stimulation (Tofts, 1990). Somatic depolarization drives both orthodromic and antidromic action potential propagation. Orthodromic propagation causes action potentials and activity-dependent plasticity in an anterograde manner. Antidromic propagation promotes dendritic spine growth of stimulated neurons through a local Ca2+ spike, leading to increased presynaptic connectivity (Pashut et al., 2011). White matter architecture changes also occur over time via synaptic strengthening and pruning (Anderson et al., 2016).
Evolution of clinical experience with TMS in treatment-resistant depression
George et al. (2000) were the first to publish results of a double-blind, sham-controlled study showing the antidepressant potential of TMS over left DLPFC in randomized subjects (George et al., 2000). TMS had been introduced years earlier by Pascual-Leone for motor cortical stimulation (Pascual-Leone et al., 1994). In the George study, 30 medication-free patients with major depressive and bipolar disorder were treated with TMS daily for 2 weeks. Twenty patients were assigned to the active TMS group, whereas 10 were assigned to the sham group. TMS sessions lasted for 20 min and occurred each weekday. All patients underwent cerebral blood flow single photon emission computed tomography (SPECT) at the start (1 day), middle (5 day), and 3 days after the end of the study. The authors concluded that daily TMS over left DLPFC produced a significant antidepressant response, defined as greater than 50% improvement in baseline Hamilton Rating Scale for Depression (HRSD) scores. In the same year, Berman et al. also assessed the efficacy of TMS in unmedicated TRD patients using a randomized, double-blind design. TRD patients enrolled in the trial were assigned to either active TMS (N = 10) or sham (N = 10) treatment. The active TMS group received 20 2 s trains of 20 Hz with 58 s intervals daily over a 2 week course (Berman et al., 2000). As in George’s study, results showed statistically significant reductions in depressive symptoms compared to the sham group. These two important papers instigated a groundswell of interest in TMS for depression and many other psychiatric disorders.
Today, TMS is practiced globally, and TRD remains the primary indication. The procedure is well tolerated; individuals who receive TMS report a > 50% decrease in symptom severity (Lisanby et al., 2009; George et al., 2010; O’Reardon et al., 2010). Despite this, one obstacle that has remained in scaling its deployment even further is the burdensome requirement for multiple treatments. For example, an initial treatment regimen consists of 40–60 min sessions of active treatment, 5 days per week for 3–6 weeks (Holtzheimer et al., 2010). Because of this, innumerable attempts have been made in the two decades since George’s study to modify the TMS protocol with the goal of shortening the amount of therapy needed. In 2018, the US Food and Drug Administration (FDA) approved intermittent theta-burst stimulation (iTBS) as a new variant of TMS for the treatment of TRD (Mendlowitz et al., 2019). FDA approval was contingent on several new studies of theta-burst stimulation, including a multi-center clinical trial by Blumberger et al. (2018) that compared the efficacy of iTBS to conventional TMS in patients with TRD (Blumberger et al., 2018). In their study, TRD patients were randomized to iTBS (N = 209) or 10 Hz TMS (N = 205). Patients were treated with the modality they were randomized to for 5 days a week for 4–6 weeks. The 10 Hz TMS sessions lasted 37 min and consisted of 3,000 pulses per session. The iTBS session consisted of triplet 50 Hz pulses repeated at 5 Hz for 600 pulses over only 3 min. The HRSD was administered after each of the sessions and 1-, 4-, and 12 weeks after treatment. They observed that scores significantly improved in both iTBS and TMS groups (overall reduction in HRSD-17 scores was 10.1 points in the iTBS group and 9.9 points in the 10 Hz TMS group) at baseline and 1 week after treatment. This trial became the formative study establishing iTBS as a safe, tolerable, and effective treatment for people with TRD. Furthermore, iTBS sessions last only a few min and are less costly than conventional TMS.
Cole and colleagues addressed another well-known challenge with TMS protocols: overall treatment duration. In the SAINT study, patients with TRD were enrolled (N = 22) and received 5 days of iTBS (Cole et al., 2020). Each day consisted of ten daily sessions, for a total of 18,000 pulses per day. Functional MRI identified individualized targets for iTBS in each patient - the region of left DLPFC most anticorrelated with SCC activity. Intent-to-treat analysis revealed 86.4% (N = 19) of patients met remission criteria, without negative cognitive effects. In the follow-up double-blind RCT SNT study (2022), patients with TRD were enrolled and randomly assigned to one of two groups: sham (N = 15) or iTBS stimulation (N = 14) (Cole et al., 2022). At baseline and 4 weeks following treatment, patients completed the Montgomery-Asberg Depression Scale (MADRS). Patients in the stimulation group experienced a 52% reduction in depression scores. Compared to conventional TMS, the discovery of iTBS and new accelerated protocols may result in substantial clinical responses in a shorter time frame.
Structural and functional connectivity of neural networks in depression: Salience as a key switching mechanism
Diffusion-weighted imaging (DWI) is a structural MR technique that derives several important physical tissue properties within the brain. These imaging markers can be used to extract brain network profiles and regions important for TRD pathophysiology. Fractional anisotropy and mean diffusivity, two of the most frequently reported metrics, provide information on microstructural architecture and integrity of white matter. Both metrics are obtained using diffusion tensor imaging, the analytical method forming the basis of tractography (Basser and Pierpaoli, 1996). Another variant of diffusion-weighted imaging is diffusion kurtosis imaging which quantifies the non-Gaussian quality of water diffusion (Jensen et al., 2005). Diffusion imaging has been used extensively to investigate how structural connectivity differs in TRD patients. Peng and colleagues conducted a double-blind, randomized study aimed at ascertaining whether white matter abnormalities cause network dysfunction in TRD patients (Peng et al., 2012). This group enrolled 30 TRD patients and randomized subjects to sham or active TMS treatment. The investigators acquired diffusion imaging and found significant reductions in FA in the left middle frontal gyrus not observed in the sham group. Korgaonkar et al. (2014) analyzed 102 MDD patients and found that the anterior cingulate cortex (ACC) limbic white matter is a useful predictor of antidepressant treatment outcome (Korgaonkar et al., 2014). Thus, based on DWI, FA could provide a glimpse into which patients with depression might be responsive to treatment.
Measurement of gray and white matter thickness using voxel-based morphometry (VBM) as well as connectivity between brain regions, inferred as specific white matter tracts or networks, can be extracted using a combination of structural and diffusion-weighted imaging. For example, the default mode network (DMN), which is distributed over many cortical regions, and the frontoparietal central executive network (CEN), have been shown to be dysregulated in patients with depression (Liston et al., 2014). DLPFC is in fact a node within the CEN. However, tractography studies were inconclusive as to whether DLPFC modulates connectivity between the two networks. Liston et al. (2014) sought to address this knowledge gap utilizing resting-state functional MRI (Liston et al., 2014). They enrolled 17 patients with depression and 35 healthy controls. The patients received 25 sessions of TMS over a 5 week period. Clinical scales assessing depression were completed at baseline and 1–3 days after completing the treatment period. Structural and diffusion-weighted scans were acquired before and after treatment. Functional connectivity maps were generated between the CEN, and DMN, using DLPFC as a seeding region. The authors observed that TMS of the DLPFC leads to connectivity changes in the DMN. This supports the idea that structures remote from the DLPFC such as DMN are also affected by TMS, suggesting a model based on knowledge of structural connectomics. However, very few studies have combined tractography and connectivity analyses in the same cohort with DWI and fMRI to determine the correlation between the two methods, particularly with respect to DLPFC and TMS.
The salience network (SN) is also dysfunctional in TRD, though it appears to have certain predictive characteristics for TMS response not seen in DMN or CEN. Fan et al. (2019) enrolled subjects with MDD and twenty healthy controls (Fan et al., 2019). Twenty real and sham sessions of TMS were administered to the DLPFC 5 days per week. Resting state fMRI sessions were performed before and after the TMS sessions, while clinical scales measuring depression were administered weekly. Segregation analyses were performed to index connections between and within networks. Using these analyses, the authors observed that segregation of the SN predicts symptom improvement after TMS, which adds to our understanding of the pathophysiology of depressive symptoms. Several other authors have hypothesized that SN performs a switching mechanism during effective treatment of depression, since it appears to refocus maladaptive ruminative behavior driven by DMN towards purposeful executive function and planning behavior driven by CEN (Sridharan et al., 2008; Menon, 2011; Goulden et al., 2014; Anderson et al., 2016). Tables 1, 2 summarize the changes in DMN, CEN, and SN associated with TRD.
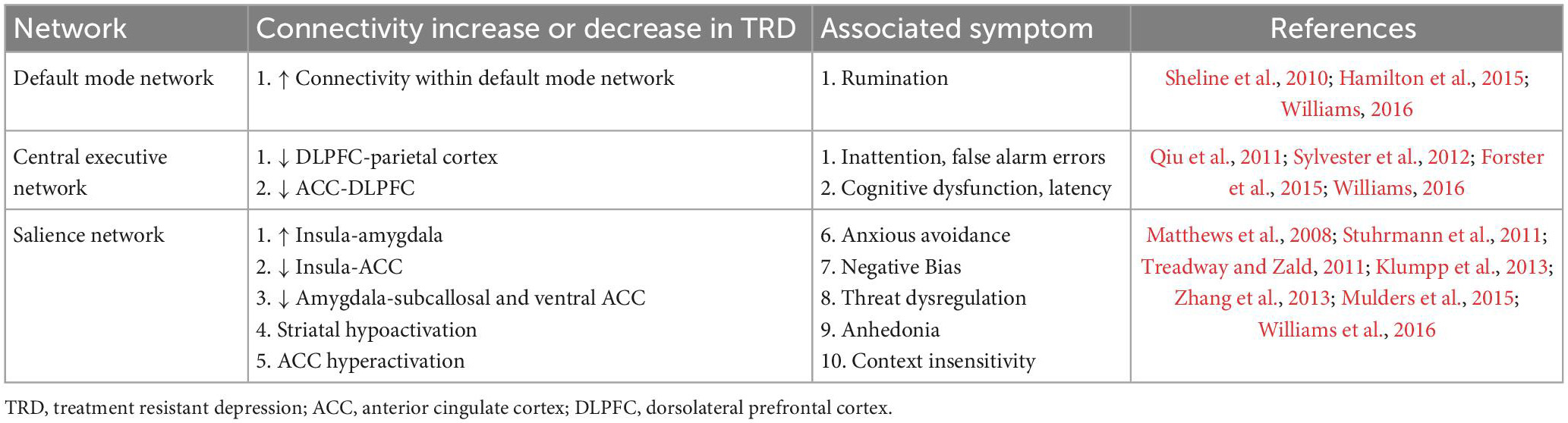
Table 2. Network connectivity changes in treatment-resistant depression (TRD) and resultant symptoms.
Epidural cortical stimulation for treatment-resistant depression: Which is more effective – unilateral or bilateral stimulation of DLPFC?
Given the success and US FDA approval of TMS for MDD in 2008, epidural cortical stimulation (EpCS) followed as an expansion of neuromodulation tools explored for the treatment of TRD. In an open-label study of EpCS as an adjunctive therapy for TRD, 5 patients were enrolled who had previously failed electroconvulsive therapy (ECT), TMS, and vagus nerve stimulation (VNS). Each patient underwent implantation of bilateral paddle leads positioned epidurally over Brodmann’s areas 10 (frontopolar prefrontal cortex) and 46 (dorsolateral prefrontal cortex) (Nahas et al., 2010). After 7 months of follow-up, patients experienced significant improvement in depressive symptoms, compared to the pre-implantation baseline—with over 50% mean improvement in metrics of depression severity and 80% of the cohort achieving remission (Nahas et al., 2010; Williams et al., 2016, 2018). Remission was sustained at the 3 and 5 year follow-up timepoints, highlighting the potential of EpCS as an adjunctive therapy for TRD, following the failure of other modalities of neuromodulation.
Similar to TMS, EpCS generates peak electric fields at the gyral crown, subjacent to the stimulation site (Wongsarnpigoon and Grill, 2008). This suggests that the antidepressive effects may depend on direct activation of gray matter, recruitment of deeper white matter and subcortical structures or both. In a meta-analysis of resting state functional imaging studies, patients with depression displayed hypoactivation of the pregenual cingulate cortex (pgCC), dorsal anterior cingulate cortex (dACC), and insula (Fitzgerald et al., 2008). Interestingly, hypoactivity was significant for bilateral dorsolateral prefrontal cortices (DLPFC) in that study (Fitzgerald et al., 2008). In addition, Salomons et al. targeted bilateral prefrontal cortices and observed changes in connectivity between SN, CEN, and DMN networks following 20 sessions of 10 Hz TMS (Salomons et al., 2014; Anderson et al., 2016). These findings support the Nahas and Williams trials which resulted in up to 80% sustained remission (Nahas et al., 2010; Williams et al., 2016, 2018) while, in a separate study, unilateral (left-sided) conventional DLPFC stimulation produced an average of 30% remission (Kopell et al., 2011). This also highlights the potential to utilize EpCS as an alternative approach in TRD patients non-responsive to TMS, perhaps drawing on similar key mechanisms (Fitzgerald et al., 2008; Grimm et al., 2008).
In addition to direct modulation of hypoactivated DLPFC, EpCS of DLPFC could improve symptoms of TRD by altering connectivity with the subcallosal cingulate cortex (SCC), similar to TMS (Fox et al., 2012) or by indirectly modulating SN. As mentioned, the SN is hypothesized to act as a “switch” that serves to transition between other core functional networks (including CEN and DMN) recruited during emotion regulation and social cognition. Nodes in the SN include dACC and bilateral insular cortices. These regions have been designated “common core” regions with aberrant activation in many psychiatric disorders, including obsessive-compulsive and post-traumatic stress disorders (Downar et al., 2016). These relationships suggest a functional interaction between DLPFC and the SN that is possibly exploited via TMS (Table 3) and EpCS for the modulation of TRD. DLPFC may act as a superficial access point to drive modulation of the deeper salience network, functioning to improve mood regulation by normalizing the activation balance and promoting transitions between intrinsic and task-evoked circuits.
Deep brain stimulation for treatment-resistant depression: Novel ideas emerge from clinical trials
Deep brain stimulation (DBS) is an invasive modality applied via stereotactic insertion of one or more intracranial leads. Its initial utility was investigated in the context of movement disorders, inspired by evidence that high frequency stimulation (100–200 Hz) of the ventral intermediate thalamic nucleus could alleviate tremor (Albe Fessard et al., 1963). In 1996, thalamic DBS received FDA approval in the US for essential and Parkinsonian tremors. Approvals for subthalamic nucleus (STN) and globus pallidus internus (GPi) DBS followed in 2003 for Parkinson’s Disease (Miocinovic et al., 2013) and anterior thalamic nucleus (ATN) DBS for epilepsy in 2018 (Salanova et al., 2015; Kim et al., 2017). Evidence-based application of DBS for TRD has proven complex given the promising outcomes in open-label investigations that have not been reproduced in randomized clinical trials (RCT) (Morishita et al., 2014). In cohort studies of nucleus accumbens DBS, 40–45% of the TRD cohort responded with > 50% reduction in depressive symptoms. For ventral capsule/ventral striatum (VC/VS) DBS cohort studies, response rates were 53% at 12 months, and 71% by the long-term endpoint (mean of 37 months) (Malone et al., 2009; Malone, 2010). Despite these positive results, the multicenter RCT of VC/VS DBS failed to meet its primary endpoint (Dougherty et al., 2015). Similarly, positive effects of subcallosal cingulate cortex (SCC) DBS were reported in multiple case reports (Neimat et al., 2008; Guinjoan et al., 2010; Holtzheimer and Mayberg, 2010; Hamani et al., 2012) and cohort studies demonstrating > 60% responder rate (Mayberg et al., 2005; Kennedy et al., 2011). A subsequent multicenter trial showed a 29% responder rate at 12 months (Lozano et al., 2012), while a single-blinded study by Holtzheimer et al. reported 91% response rate and 58% remission rate (Holtzheimer et al., 2012). Of note the later study utilized higher intensity stimulation (6–10 mA) than the former (5.2 mA). These led to the BROADEN trial, which was conducted as a multicenter, randomized controlled trial for SCC DBS. Subjects underwent bilateral SCC implantation and were randomized to active or sham DBS for 6 months. Following the first endpoint of 6 months, no group differences were found, and the study was discontinued following a futility analysis. Nevertheless, two novel findings emerged from this study. First, a retrospective tractography study observed that SCC DBS responders were more likely to have the active contacts located at the convergence of four white matter pathways: forceps minor, uncinate fasciculus, cingulum bundle, and fronto-striatal fibers (Riva-Posse et al., 2014). Second, when subjects were followed longitudinally (2–8 years), the response and remission rates rose to 81 and 54%, respectively (Crowell et al., 2019). These follow-up studies provide encouragement for the therapeutic potential of DBS for TRD, as increasing evidence suggests that heterogeneity of psychiatric disorders may warrant an individualized approach, including connectomics and tractography, for patient-specific target identification (Riva-Posse et al., 2018; Allawala et al., 2021; Hollunder et al., 2022).
DBS antidepressive mechanisms
An advantage of SCC DBS is that it directly targets the white matter tracts underlying the subgenual cingulate cortex and thus gains access to functional networks involving the cingulum bundle. In contrast, the stimulus area accessed by ECT, TMS and EpCS is less focused. Notwithstanding, Tsolaki and colleagues demonstrated differences in responsivity to ECT based on SCC connectivity, again supporting the idea that non-invasive and invasive modalities share network coupling dynamics (Tsolaki et al., 2021). DBS contacts apply a three-dimensional electric field to surrounding tissue, resulting in depolarization or hyperpolarization of neighboring dendrites and axons (McIntyre and Foutz, 2013). The therapeutic effects are generated via high frequency stimulation (∼100–130 Hz) of a target selected for its ability to modulate an aspect of neurologic dysfunction such as ATN for epilepsy and STN for Parkinson’s disease. Initial functional studies in patients with depression demonstrated increased glucose metabolism in the SCC and pregenual cingulate cortex (pgCC) (Sacher et al., 2012). Mayberg and colleagues observed that hypermetabolism in SCC was attenuated with pharmacologic antidepressants (Mayberg et al., 1997, 2000), identifying SCC as a potential target for modulation of TRD with DBS. As suggested by tractography studies (Riva-Posse et al., 2014; Tsolaki et al., 2017), SCC DBS likely activates white matter tracts in close proximity to the electrode contacts, such as the cingulum bundle and uncinate fasciculus. The cingulum is a large white matter tract superficial to the corpus callosum and involved in executive control, emotion, pain, and memory (Bubb et al., 2018). Axons from the subgenual and anterior cingulate subsections have terminations in the anterior cingulate cortex (ACC), prefrontal cortex (dorsolateral, medial and orbital), amygdala, insula and superior temporal cortex (Bubb et al., 2018). Each of these structures have been strongly implicated in the SN and serve as potential sites of modulation for emotion dysregulation. Moreover, in a study of error rate in an emotional empathy task after ischemic stroke, Oishi et al. showed that right uncinate fasciculus lesions were associated with greater error rate. This demonstrated the role of the uncinate fasciculus, a tract with connections to orbitofrontal cortex, anterior insula, temporal pole, and amygdala, in emotional empathy (Oishi et al., 2015). SCC DBS at the intersection of these and other tracts may provide a locus for modulation of the SN given apparent overlap with key nodes: dorsal anterior cingulate cortex, anterior insula, temporal pole, and the amygdala (Friston, 2017). Direct access to the SN may provide a useful clue as to the potential mechanism for DMN and CEN activation via SCC DBS. Table 4 summarizes key clinical evidence of SN modulation with DBS.
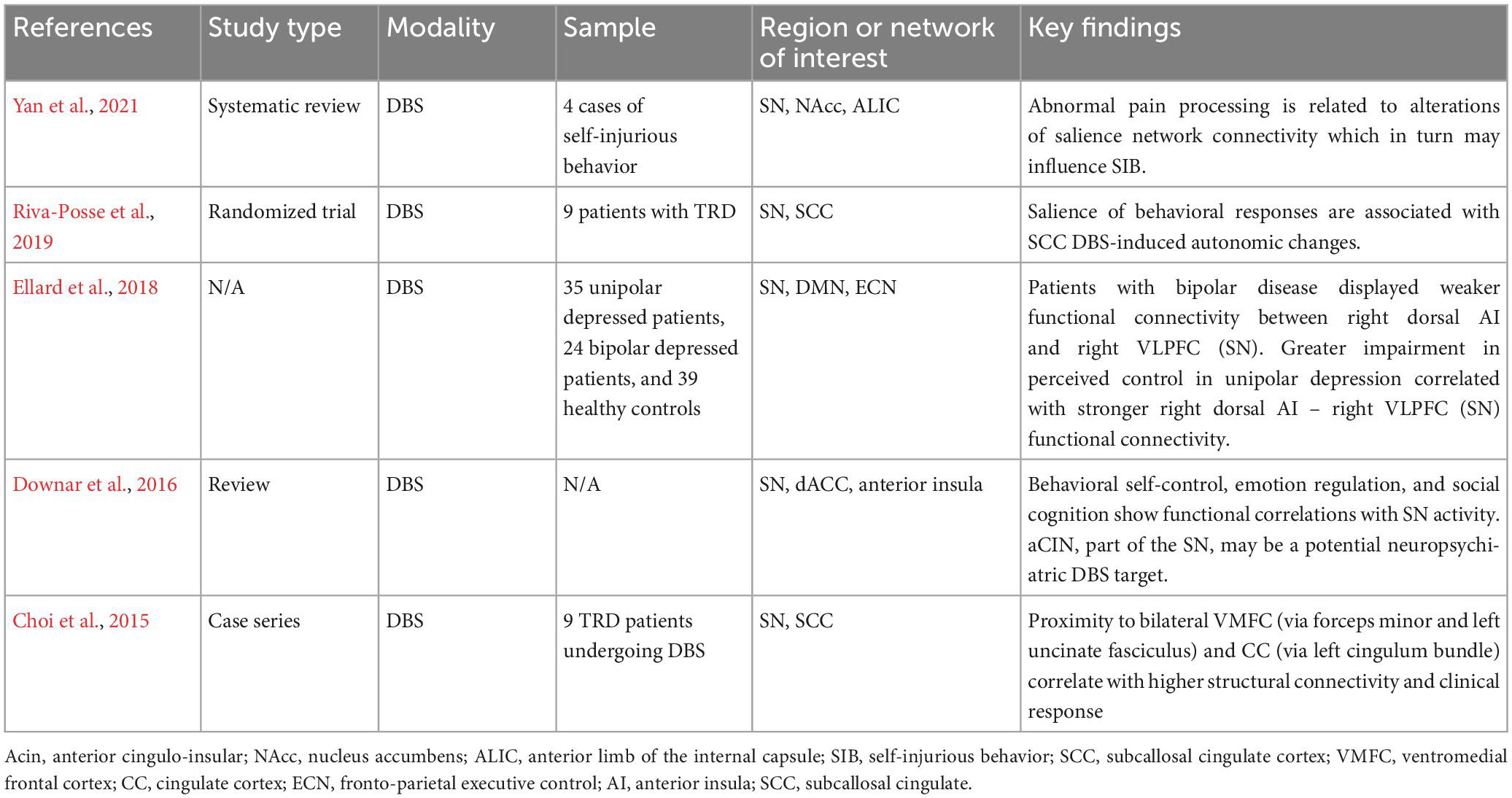
Table 4. Deep brain stimulation (DBS) for treatment-resistant depression (TRD) focusing on salience network.
Multi-modality neuromodulation for TRD: A network-centric clinical algorithm
Given the complexity and heterogeneity associated with TRD, it would be advantageous to create a systematic therapeutic approach. We propose an heuristic clinical pathway that utilizes available neuromodulation technologies, tiered by invasiveness, to find the optimal, personalized treatment plan for individuals with TRD. This stepwise neuromodulation clinical pathway, paired with individualized target identification via tractography and functional connectomics, could result in more consistent patient outcomes.
In this pathway, patients that have failed three or more antidepressants and ECT will be treated with Level 1A neuromodulation—conventional DLPFC TMS protocol (6 weeks). If symptoms persist (<50% improvement in symptoms), patients may undergo additional testing including functional connectivity imaging, tractography, behavioral and cognitive testing for characterization of patient-specific TRD neurobiology contributing to depressive symptoms. Unique TRD profiles in certain patients have been suggested by several authors proposing that specific depressive symptoms map onto distinct functional networks (Williams, 2016; Hollunder et al., 2022). Imaging data would be used to identify personalized targets for subsequent neuromodulation. The next step, Level 1B neuromodulation, consists of accelerated TMS such as the 5 day SAINT protocol, using iTBS. If this fails, evidence of abnormal activation in DMN (hyperactivation) or CEN (hypoactivation) would be an indicator for Level 2 neuromodulation—bilateral DLPFC and frontopolar PFC EpCS for 6 months. If imaging identifies hypoactivation of SN, Level 3 neuromodulation—SCC DBS, would be recommended. VNS, which has been implicated in DLPFC activation via connections to the locus coeruleus, is an alternative if intracranial surgery is contraindicated (Conway et al., 2006, 2018; Dorr and Debonnel, 2006; Roosevelt et al., 2006; Kamel et al., 2022). Broader network dysfunction in DMN, CEN, and SN or severe, refractory cases would be an indication for Level 4 neuromodulation—stereotactic electroencephalography (sEEG)-informed adaptive DBS. For this level, sEEG would assist with identifying symptom-specific biomarkers and targets for personalized closed-loop DBS. Scangos and colleagues used this approach and reported favorable outcomes with VC/VS stimulation for modulation of symptom-associated gamma power in the amygdala of one patient with TRD (Scangos et al., 2021). In that study, machine learning (ML) algorithms were used to analyze biomarkers in one structure while stimulating in a remote site. While specific white matter pathways were not considered in that study, it is interesting that the ML algorithms modeling the data identified a target other than SCC as most effective in ameliorating the patient’s symptoms. The findings support the idea that not only may TRD manifest differently in each individual, structures outside the traditional DMN, SN or CEN networks, such as the amygdala, may provide useful biomarker correlations. A schematic of the proposed clinical pathway is depicted in Figure 1.
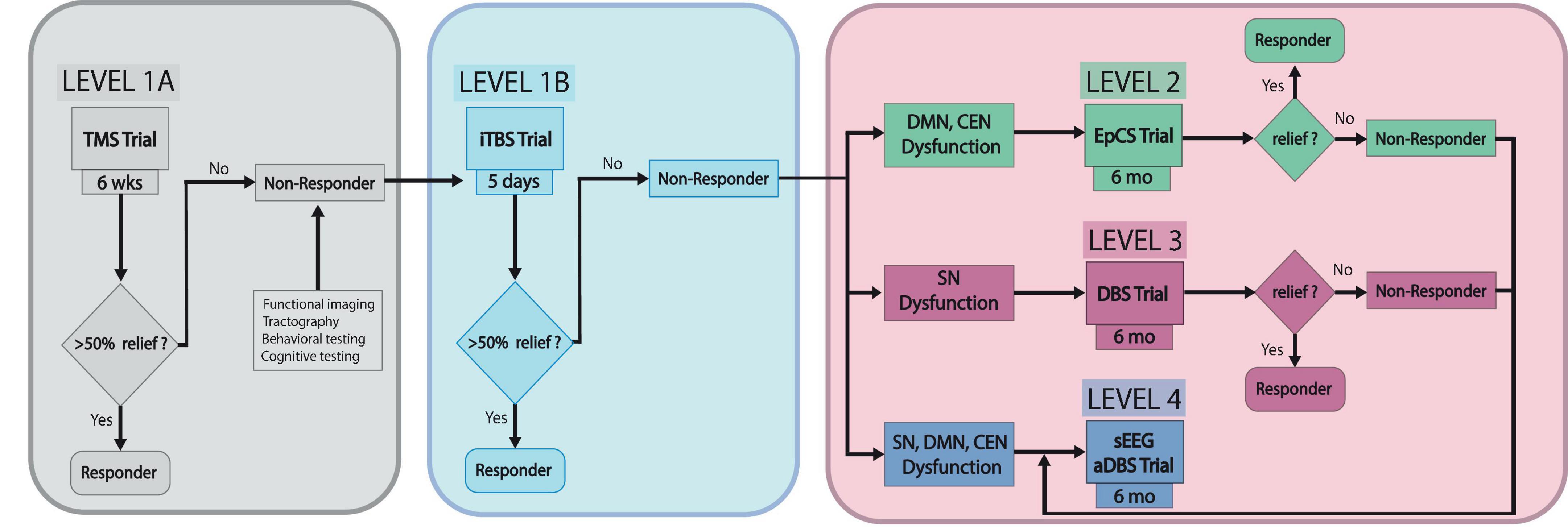
Figure 1. TRD neuromodulation clinical pathway. This schematic depicts the proposed stepwise pathway for optimizing individualized management of treatment-resistant depression sEEG, stereotactic electroencephalography; aDBS, adaptive deep brain stimulation.
As previously described, depression is associated with hyperactivation of the DMN which may contribute to symptoms of rumination and pessimism (Anderson et al., 2016). This may or may not be cosynchronous with SN and CEN hypoactivation, resulting in aberrant responses to salient stimuli, memory deficits, and attentional dysfunction (Anderson et al., 2016). The neuromodulation clinical pathway we have proposed enables a multifaceted approach for treating depression by targeting nodes within each of these networks. This provides a systematic approach for implementing neuromodulation to find the target and therapy that works optimally for each patient.
In summary, the imbalance between network activity may prime individuals to preferentially respond to a particular stimulation locus and modality. TMS at the DLPFC directly modulates the node of the central executive network. But this region also has functional connections with nodes of the SN (ACC and anterior insula) and the DMN (ventromedial prefrontal cortex (VMPFC), posterior cingulate cortex (PCC) and lateral parietal cortex) (Figures 2A–C). Patients with pathologic hypoactivation of DLPFC as the primary insult may be responders to TMS. Bilateral DLPFC and frontopolar stimulation with EpCS likely alters activity of the CEN and DMN but also has downstream effects on the salience network. DBS at the intersection of the SCC, cingulum, and uncinate fasciculus likely modulates the SN via dACC and anterior insula (Figures 2C, D). This is probably paired with effects on the CEN and DMN through functional connections with DLPFC and VMPFC to PCC, respectively. Patients with hypoactivation of the salience network may preferentially respond to SCC DBS. A network-centric clinical pathway brings together multiple disciplines and proposes a new common language with the aim of enhancing care for treatment-resistant depression.
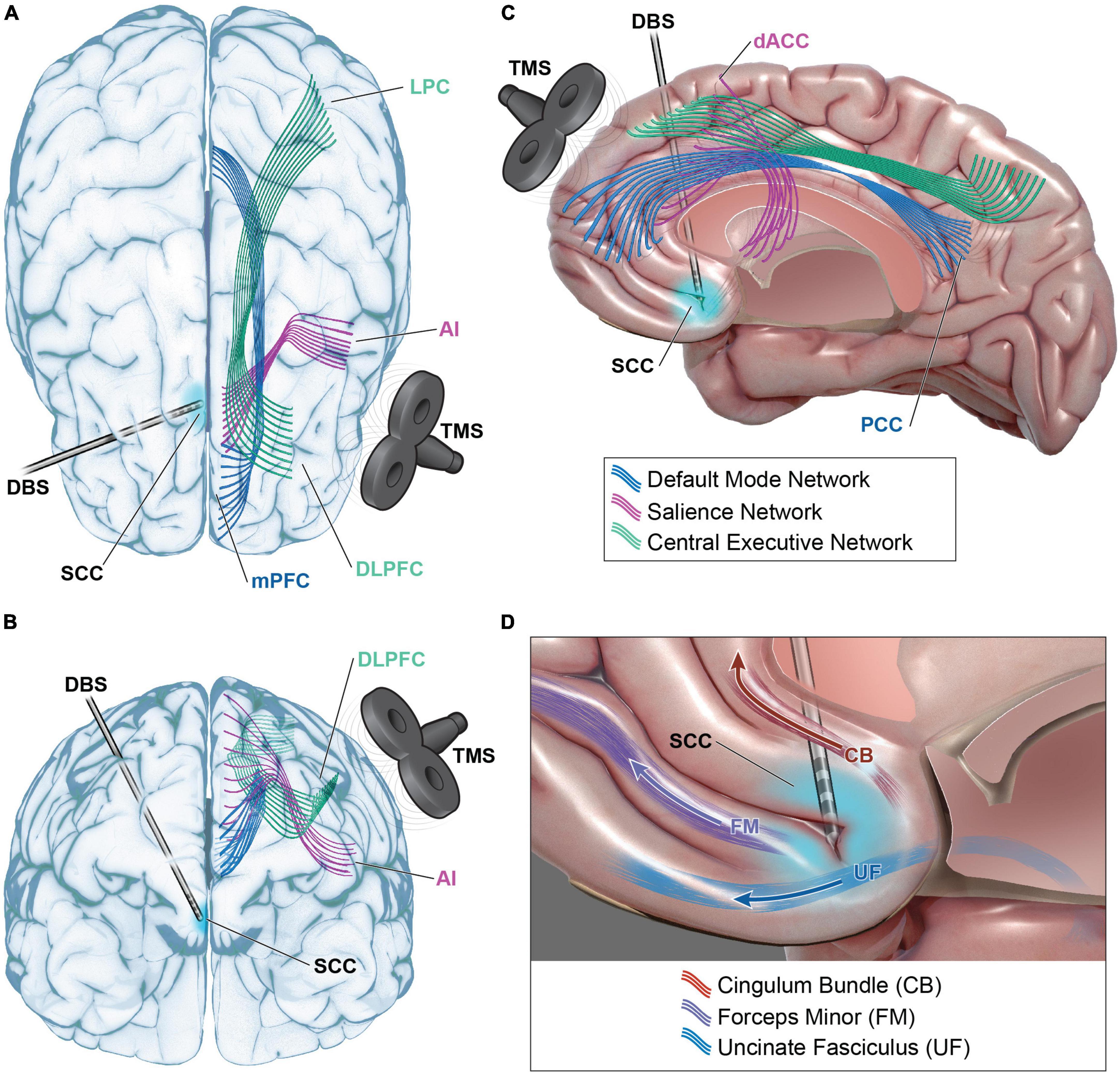
Figure 2. Functional network modulation in TRD. Default mode network (DMN – blue), salience network (SN – purple), and central executive network (CEN – green) are accessible for modulation with transcranial magnetic stimulation (TMS) and deep brain stimulation (DBS). Dorsal lateral prefrontal cortex (DLPFC) TMS may modulate CEN via direct modulation of DLPFC and its projections to lateral parietal cortex (LPC) (A–C). DLPFC TMS could indirectly modulate SN via functional connections with anterior cingulate cortex (ACC), anterior insula (AI), or DMN via functional connections with medial prefrontal cortex (mPFC) and posterior cingulate cortex (PCC). DBS at the intersection of subcallosal cingulate cortex (SCC), the cingulum bundle (CB), uncinate fasciculus (UF), and forceps minor (FM) may modulate SN via projections to dorsal anterior cingulate cortex (dACC) and AI (C,D). Functional connections to DLPFC, mPFC, and PCC also present avenues for modulation of CEN and DMN. Please see Table 1 for full listing of network structures.
Author contributions
NR and ES helped to frame to scope of the manuscript and provided the editorial support. SI-A, CS, MB, and NR composed the manuscript, figures, and tables. All authors contributed to the article and approved the submitted version.
Conflict of interest
The authors declare that the research was conducted in the absence of any commercial or financial relationships that could be construed as a potential conflict of interest.
Publisher’s note
All claims expressed in this article are solely those of the authors and do not necessarily represent those of their affiliated organizations, or those of the publisher, the editors and the reviewers. Any product that may be evaluated in this article, or claim that may be made by its manufacturer, is not guaranteed or endorsed by the publisher.
Abbreviations
TMS, transcranial magnetic stimulation; TRD, treatment-resistant depression; DBS, deep brain stimulation; DLPFC, dorsal lateral prefrontal cortex; SCC, subcallosal cingulate cortex; MDD, major depression; BDNF, brain-derived neurotrophic factor; GABA, gamma-aminobutyric acid; NMDA, N-Nitrosodimethylamine; HRSD, Hamilton Rating Scale for Depression; iTBS, intermittent theta-burst stimulation; MADRS, Montgomery-Asberg Depression Scale; RCT, randomized control trial; DWI, diffusion weighted imaging; ACC, anterior cingulate cortex; CEN, central executive network; DMN, default mode network; SN, salience network; EpCS, epidural cortical stimulation; ECT, electroconvulsive therapy; sEEG, stereotactic electroencephalogram; MRI, magnetic resonance imaging; dACC, dorsal anterior cingulate cortex; pgCC, pregenual cingulate cortex; VC/VS, ventral capsule/ventral striatum; STN, subthalamic nucleus; ATN, anterior thalamic nucleus; GPi, globus pallidus internus; VMPFC, ventromedial prefrontal cortex; PCC, posterior cingulate cortex.
References
Albe Fessard, D., Arfel, G., Guiot, G., Derome, P., Dela, H., Korn, H., et al. (1963). Characteristic electric activities of some cerebral structures in man. Ann. Chir. 17, 1185–1214.
Alexopoulos, G., Hoptman, M., Kanellopoulos, D., Murphy, C., Lim, K., and Gunning, F. (2012). Functional connectivity in the cognitive control network and the default mode network in late-life depression. J. Affect. Disord. 139, 56–65. doi: 10.1016/j.jad.2011.12.002
Al-Harbi, K. (2012). Treatment-resistant depression: therapeutic trends, challenges, and future directions. Patient Prefer Adher. 6, 369–388. doi: 10.2147/PPA.S29716
Allawala, A., Bijanki, K., Goodman, W., Cohn, J., Viswanathan, A., Yoshor, D., et al. (2021). A novel framework for network-targeted neuropsychiatric deep brain stimulation. Neurosurgery 89, E116–E121. doi: 10.1093/neuros/nyab112
Anderson, R., Hoy, K., Daskalakis, Z., and Fitzgerald, P. (2016). Repetitive transcranial magnetic stimulation for treatment resistant depression: Re-establishing connections. Clin. Neurophysiol. 127, 3394–3405. doi: 10.1016/j.clinph.2016.08.015
Andrews-Hanna, J., Reidler, J., Sepulcre, J., Poulin, R., and Buckner, R. (2010). Functional-anatomic fractionation of the brain’s default network. Neuron 65, 550–562. doi: 10.1016/j.neuron.2010.02.005
Barker, A., Jalinous, R., and Freeston, I. (1985). Non-invasive magnetic stimulation of human motor cortex. Lancet 1, 1106–1107. doi: 10.1016/s0140-6736(85)92413-4
Basser, P., and Pierpaoli, C. (1996). Microstructural and physiological features of tissues elucidated by quantitative-diffusion-tensor MRI. J. Magn. Reson. B. 111, 209–219. doi: 10.1006/jmrb.1996.0086
Bergfeld, I., Mantione, M., Figee, M., Schuurman, P., Lok, A., and Denys, D. (2018). Treatment-resistant depression and suicidality. J. Affect. Disord. 235, 362–367. doi: 10.1016/j.jad.2018.04.016
Berman, R., Narasimhan, M., Sanacora, G., Miano, A., Hoffman, R., Hu, X., et al. (2000). A randomized clinical trial of repetitive transcranial magnetic stimulation in the treatment of major depression. Biol. Psychiatry 47, 332–337. doi: 10.1016/s0006-3223(99)00243-7
Blumberger, D., Vila-Rodriguez, F., Thorpe, K., Feffer, K., Noda, Y., Giacobbe, P., et al. (2018). Effectiveness of theta burst versus high-frequency repetitive transcranial magnetic stimulation in patients with depression (THREE-D): A randomised non-inferiority trial. Lancet 391, 1683–1692. doi: 10.1016/S0140-6736(18)30295-2
Bubb, E., Metzler-Baddeley, C., and Aggleton, J. (2018). The cingulum bundle: Anatomy, function, and dysfunction. Neurosci. Biobehav. Rev. 92, 104–127. doi: 10.1016/j.neubiorev.2018.05.008
Buckner, R., Andrews-Hanna, J., and Schacter, D. (2008). The brain’s default network: Anatomy, function, and relevance to disease. Ann. N. Y. Acad. Sci. 1124, 1–38. doi: 10.1196/annals.1440.011
Choi, K., Riva-Posse, P., Gross, R., and Mayberg, H. (2015). Mapping the “Depression Switch” During Intraoperative Testing of Subcallosal Cingulate Deep Brain Stimulation. JAMA Neurol. 72, 1252–1260. doi: 10.1001/jamaneurol.2015.2564
Cohen, S., Bikson, M., Badran, B., and George, M. S. (2022). A visual and narrative timeline of US FDA milestones for Transcranial Magnetic Stimulation (TMS) devices. Brain Stimul. 15, 73–75. doi: 10.1016/j.brs.2021.11.010
Cole, E., Phillips, A., Bentzley, B., Stimpson, K., Nejad, R., Barmak, F., et al. (2022). Stanford Neuromodulation Therapy (SNT): A double-blind randomized controlled trial. Am. J. Psychiatry 179, 132–141. doi: 10.1176/appi.ajp.2021.20101429
Cole, E., Stimpson, K., Bentzley, B., Gulser, M., Cherian, K., Tischler, C., et al. (2020). Stanford accelerated intelligent neuromodulation therapy for treatment-resistant depression. Am. J. Psychiatry 177, 716–726. doi: 10.1176/appi.ajp.2019.19070720
Conway, C., Kumar, A., Xiong, W., Bunker, M., Aaronson, S., and Rush, A. (2018). Chronic vagus nerve stimulation significantly improves quality of life in treatment-resistant major depression. J. Clin. Psychiatry 79:18m12178. doi: 10.4088/JCP.18m12178
Conway, C., Sheline, Y., Chibnall, J., George, M., Fletcher, J., and Mintun, M. (2006). Cerebral blood flow changes during vagus nerve stimulation for depression. Psychiatry Res. 146, 179–184. doi: 10.1016/j.pscychresns.2005.12.007
Crowell, A., Riva-Posse, P., Holtzheimer, P., Garlow, S., Kelley, M., Gross, R., et al. (2019). Long-Term outcomes of subcallosal cingulate deep brain stimulation for treatment-resistant depression. Am. J. Psychiatry 176, 949–956. doi: 10.1176/appi.ajp.2019.18121427
Dorr, A., and Debonnel, G. (2006). Effect of vagus nerve stimulation on serotonergic and noradrenergic transmission. J. Pharmacol. Exp. Ther. 318, 890–898. doi: 10.1124/jpet.106.104166
Dougherty, D., Rezai, A., Carpenter, L., Howland, R., Bhati, M., O’Reardon, J., et al. (2015). A Randomized Sham-Controlled Trial of Deep Brain Stimulation of the Ventral Capsule/Ventral Striatum for Chronic Treatment-Resistant Depression. Biol. Psychiatry 78, 240–248. doi: 10.1016/j.biopsych.2014.11.023
Downar, J., Blumberger, D., and Daskalakis, Z. (2016). The neural crossroads of psychiatric illness: An emerging target for brain stimulation. Trends Cogn. Sci. 20, 107–120. doi: 10.1016/j.tics.2015.10.007
Ellard, K., Zimmerman, J., Kaur, N., Van Dijk, K., Roffman, J., Nierenberg, A., et al. (2018). Functional connectivity between anterior insula and key nodes of frontoparietal executive control and salience networks distinguish bipolar depression from unipolar depression and healthy control subjects. Biol. Psychiatry Cogn. Neurosci. Neuroimaging 3, 473–484. doi: 10.1016/j.bpsc.2018.01.013
Fan, J., Tso, I., Maixner, D., Abagis, T., Hernandez-Garcia, L., and Taylor, S. (2019). Segregation of salience network predicts treatment response of depression to repetitive transcranial magnetic stimulation. Neuroimage Clin. 22:101719. doi: 10.1016/j.nicl.2019.101719
Fitzgerald, P., Laird, A., Maller, J., and Daskalakis, Z. J. (2008). A meta-analytic study of changes in brain activation in depression. Hum. Brain Mapp. 29, 683–695. doi: 10.1002/hbm.20426
Forster, S., Nunez Elizalde, A., Castle, E., and Bishop, S. (2015). Unraveling the anxious mind: Anxiety, worry, and frontal engagement in sustained attention versus off-task processing. Cereb. Cortex 25, 609–618. doi: 10.1093/cercor/bht248
Fox, M., Buckner, R., White, M., Greicius, M., and Pascual-Leone, A. (2012). Efficacy of transcranial magnetic stimulation targets for depression is related to intrinsic functional connectivity with the subgenual cingulate. Biol. Psychiatry 72, 595–603. doi: 10.1016/j.biopsych.2012.04.028
Fox, M., Snyder, A., Vincent, J., Corbetta, M., Van Essen, D., and Raichle, M. (2005). The human brain is intrinsically organized into dynamic, anticorrelated functional networks. Proc. Natl. Acad. Sci. U.S.A. 102, 9673–9678. doi: 10.1073/pnas.0504136102
Friston, K. (2017). Precision Psychiatry. Biol. Psychiatry Cogn. Neurosci. Neuroimaging 2, 640–643. doi: 10.1016/j.bpsc.2017.08.007
George, M., Lisanby, S., Avery, D., McDonald, W., Durkalski, V., Pavlicova, M., et al. (2010). Daily left prefrontal transcranial magnetic stimulation therapy for major depressive disorder: A sham-controlled randomized trial. Arch. Gen. Psychiatry 67, 507–516. doi: 10.1001/archgenpsychiatry.2010.46
George, M., Nahas, Z., Molloy, M., Speer, A., Oliver, N., Li, X., et al. (2000). A controlled trial of daily left prefrontal cortex TMS for treating depression. Biol. Psychiatry 48, 962–970. doi: 10.1016/s0006-3223(00)01048-9
Goulden, N., Khusnulina, A., Davis, N., Bracewell, R., Bokde, A., McNulty, J., et al. (2014). The salience network is responsible for switching between the default mode network and the central executive network: Replication from DCM. Neuroimage 99, 180–190. doi: 10.1016/j.neuroimage.2014.05.052
Greicius, M., Flores, B., Menon, V., Glover, G., Solvason, H., Kenna, H., et al. (2007). Resting-state functional connectivity in major depression: Abnormally increased contributions from subgenual cingulate cortex and thalamus. Biol. Psychiatry 62, 429–437. doi: 10.1016/j.biopsych.2006.09.020
Grimm, S., Beck, J., Schuepbach, D., Hell, D., Boesiger, P., Bermpohl, F., et al. (2008). Imbalance between left and right dorsolateral prefrontal cortex in major depression is linked to negative emotional judgment: An fMRI study in severe major depressive disorder. Biol. Psychiatry 63, 369–376. doi: 10.1016/j.biopsych.2007.05.033
Guinjoan, S., Mayberg, H., Costanzo, E., Fahrer, R., Tenca, E., Antico, J., et al. (2010). Asymmetrical contribution of brain structures to treatment-resistant depression as illustrated by effects of right subgenual cingulum stimulation. J. Neuropsychiatry Clin. Neurosci. 22, 265–277. doi: 10.1176/jnp.2010.22.3.265
Hamani, C., Giacobbe, P., Diwan, M., Balbino, E., Tong, J., Bridgman, A., et al. (2012). Monoamine oxidase inhibitors potentiate the effects of deep brain stimulation. Am. J. Psychiatry 169, 1320–1321. doi: 10.1176/appi.ajp.2012.12060754
Hamilton, J., Chen, M., and Gotlib, I. (2013). Neural systems approaches to understanding major depressive disorder: An intrinsic functional organization perspective. Neurobiol. Dis. 52, 4–11. doi: 10.1016/j.nbd.2012.01.015
Hamilton, J., Farmer, M., Fogelman, P., and Gotlib, I. (2015). Depressive rumination, the default-mode network, and the dark matter of clinical neuroscience. Biol. Psychiatry 78, 224–230. doi: 10.1016/j.biopsych.2015.02.020
Hawco, C., Voineskos, A., Steeves, J., Dickie, E., Viviano, J., Downar, J., et al. (2018). Spread of activity following TMS is related to intrinsic resting connectivity to the salience network: A concurrent TMS-fMRI study. Cortex 108, 160–172. doi: 10.1016/j.cortex.2018.07.010
Hollunder, B., Rajamani, N., Siddiqi, S., Finke, C., Kühn, A., Mayberg, H., et al. (2022). Toward personalized medicine in connectomic deep brain stimulation. Prog. Neurobiol. 210:102211. doi: 10.1016/j.pneurobio.2021.102211
Holtzheimer, P., Kelley, M., Gross, R., Filkowski, M., Garlow, S., Barrocas, A., et al. (2012). Subcallosal cingulate deep brain stimulation for treatment-resistant unipolar and bipolar depression. Arch. Gen. Psychiatry 69, 150–158. doi: 10.1001/archgenpsychiatry.2011.1456
Holtzheimer, P., and Mayberg, H. (2010). Deep brain stimulation for treatment-resistant depression. Am. J. Psychiatry 167, 1437–1444. doi: 10.1176/appi.ajp.2010.10010141
Holtzheimer, P., McDonald, W., Mufti, M., Kelley, M., Quinn, S., Corso, G., et al. (2010). Accelerated repetitive transcranial magnetic stimulation for treatment-resistant depression. Depress Anxiety 27, 960–963. doi: 10.1002/da.20731
Iwabuchi, S., Auer, D., Lankappa, S., and Palaniyappan, L. (2019). Baseline effective connectivity predicts response to repetitive transcranial magnetic stimulation in patients with treatment-resistant depression. Eur. Neuropsychopharmacol. 29, 681–690. doi: 10.1016/j.euroneuro.2019.02.012
Jensen, J., Helpern, J., Ramani, A., Lu, H., and Kaczynski, K. (2005). Diffusional kurtosis imaging: The quantification of non-gaussian water diffusion by means of magnetic resonance imaging. Magn. Reson. Med. 53, 1432–1440. doi: 10.1002/mrm.20508
Kaiser, R., Andrews-Hanna, J., Wager, T., and Pizzagalli, D. (2015). Large-scale network dysfunction in major depressive disorder: A meta-analysis of resting-state functional connectivity. JAMA Psychiatry 72, 603–611. doi: 10.1001/jamapsychiatry.2015.0071
Kamel, L., Xiong, W., Gott, B., Kumar, A., and Conway, C. (2022). Vagus nerve stimulation: An update on a novel treatment for treatment-resistant depression. J. Neurol. Sci. 434:120171. doi: 10.1016/j.jns.2022.120171
Kennedy, S., Giacobbe, P., Rizvi, S., Placenza, F., Nishikawa, Y., Mayberg, H., et al. (2011). Deep brain stimulation for treatment-resistant depression: Follow-up after 3 to 6 years. Am. J. Psychiatry 168, 502–510. doi: 10.1176/appi.ajp.2010.10081187
Kim, S., Lim, S., Kim, J., Son, B., Lee, K., and Shon, Y. (2017). Long-term follow-up of anterior thalamic deep brain stimulation in epilepsy: A 11-year, single center experience. Seizure 52, 154–161. doi: 10.1016/j.seizure.2017.10.009
Klumpp, H., Post, D., Angstadt, M., Fitzgerald, D., and Phan, K. (2013). Anterior cingulate cortex and insula response during indirect and direct processing of emotional faces in generalized social anxiety disorder. Biol. Mood Anxiety Disord. 3:7. doi: 10.1186/2045-5380-3-7
Kopell, B., Halverson, J., Butson, C., Dickinson, M., Bobholz, J., Harsch, H., et al. (2011). Epidural cortical stimulation of the left dorsolateral prefrontal cortex for refractory major depressive disorder. Neurosurgery 69, 1015–1029. doi: 10.1227/NEU.0b013e318229cfcd
Korgaonkar, M., Williams, L., Song, Y., Usherwood, T., and Grieve, S. (2014). Diffusion tensor imaging predictors of treatment outcomes in major depressive disorder. Br. J Psychiatry 205, 321–328. doi: 10.1192/bjp.bp.113.140376
Lisanby, S., Husain, M., Rosenquist, P., Maixner, D., Gutierrez, R., Krystal, A., et al. (2009). Daily left prefrontal repetitive transcranial magnetic stimulation in the acute treatment of major depression: Clinical predictors of outcome in a multisite, randomized controlled clinical trial. Neuropsychopharmacology 34, 522–534. doi: 10.1038/npp.2008.118
Liston, C., Chen, A., Zebley, B., Drysdale, A., Gordon, R., Leuchter, B., et al. (2014). Default mode network mechanisms of transcranial magnetic stimulation in depression. Biol. Psychiatry 76, 517–526. doi: 10.1016/j.biopsych.2014.01.023
Lozano, A., Giacobbe, P., Hamani, C., Rizvi, S., Kennedy, S., Kolivakis, T., et al. (2012). A multicenter pilot study of subcallosal cingulate area deep brain stimulation for treatment-resistant depression. J. Neurosurg. 116, 315–322. doi: 10.3171/2011.10.JNS102122
Malone, D. (2010). Use of deep brain stimulation in treatment-resistant depression. Cleve. Clin J. Med. 77(Suppl. 3) S77–S80. doi: 10.3949/ccjm.77.s3.14
Malone, D., Dougherty, D., Rezai, A., Carpenter, L., Friehs, G., Eskandar, E., et al. (2009). Deep brain stimulation of the ventral capsule/ventral striatum for treatment-resistant depression. Biol. Psychiatry 65, 267–275. doi: 10.1016/j.biopsych.2008.08.029
Manoliu, A., Riedl, V., Zherdin, A., Mühlau, M., Schwerthöffer, D., Scherr, M., et al. (2014). Aberrant dependence of default mode/central executive network interactions on anterior insular salience network activity in schizophrenia. Schizophr. Bull. 40, 428–437. doi: 10.1093/schbul/sbt037
Matthews, S., Strigo, I., Simmons, A., Yang, T., and Paulus, M. (2008). Decreased functional coupling of the amygdala and supragenual cingulate is related to increased depression in unmedicated individuals with current major depressive disorder. J. Affect. Disord. 111, 13–20. doi: 10.1016/j.jad.2008.05.022
Mayberg, H., Brannan, S., Mahurin, R., Jerabek, P., Brickman, J., Tekell, J., et al. (1997). Cingulate function in depression: A potential predictor of treatment response. Neuroreport 8, 1057–1061. doi: 10.1097/00001756-199703030-00048
Mayberg, H., Brannan, S., Tekell, J., Silva, J., Mahurin, R., McGinnis, S., et al. (2000). Regional metabolic effects of fluoxetine in major depression: Serial changes and relationship to clinical response. Biol. Psychiatry 48, 830–843. doi: 10.1016/s0006-3223(00)01036-2
Mayberg, H., Lozano, A., Voon, V., McNeely, H., Seminowicz, D., Hamani, C., et al. (2005). Deep brain stimulation for treatment-resistant depression. Neuron 45, 651–660. doi: 10.1016/j.neuron.2005.02.014
McIntyre, C., and Foutz, T. (2013). Computational modeling of deep brain stimulation. Handb. Clin. Neurol. 116, 55–61. doi: 10.1016/B978-0-444-53497-2.00005-X
Mendlowitz, A., Shanbour, A., Downar, J., Vila-Rodriguez, F., Daskalakis, Z., Isaranuwatchai, W., et al. (2019). Implementation of intermittent theta burst stimulation compared to conventional repetitive transcranial magnetic stimulation in patients with treatment resistant depression: A cost analysis. PLoS One 14:e0222546. doi: 10.1371/journal.pone.0222546
Menon, V. (2011). Large-scale brain networks and psychopathology: A unifying triple network model. Trends Cogn. Sci. 15, 483–506. doi: 10.1016/j.tics.2011.08.003
Miocinovic, S., Somayajula, S., Chitnis, S., and Vitek, J. (2013). History, applications, and mechanisms of deep brain stimulation. JAMA Neurol. 70, 163–171. doi: 10.1001/2013.jamaneurol.45
Morishita, T., Fayad, S., Higuchi, M., Nestor, K., and Foote, K. (2014). Deep brain stimulation for treatment-resistant depression: Systematic review of clinical outcomes. Neurotherapeutics 11, 475–484. doi: 10.1007/s13311-014-0282-1
Mulders, P., van Eijndhoven, P., Schene, A., Beckmann, C., and Tendolkar, I. (2015). Resting-state functional connectivity in major depressive disorder: A review. Neurosci. Biobehav. Rev. 56, 330–344. doi: 10.1016/j.neubiorev.2015.07.014
Nahas, Z., Anderson, B., Borckardt, J., Arana, A., George, M., Reeves, S., et al. (2010). Bilateral epidural prefrontal cortical stimulation for treatment-resistant depression. Biol. Psychiatry 67, 101–109. doi: 10.1016/j.biopsych.2009.08.021
Neimat, J., Hamani, C., Giacobbe, P., Merskey, H., Kennedy, S., Mayberg, H., et al. (2008). Neural stimulation successfully treats depression in patients with prior ablative cingulotomy. Am. J. Psychiatry 165, 687–693. doi: 10.1176/appi.ajp.2008.07081298
Oishi, K., Faria, A., Hsu, J., Tippett, D., Mori, S., and Hillis, A. (2015). Critical role of the right uncinate fasciculus in emotional empathy. Ann. Neurol. 77, 68–74. doi: 10.1002/ana.24300
O’Reardon, J., Solvason, H., Janicak, P., Sampson, S., Isenberg, K., Nahas, Z., et al. (2010). Reply regarding “efficacy and safety of transcranial magnetic stimulation in the acute treatment of major depression: A multisite randomized controlled trial”. Biol. Psychiatry 67, e15–e17. doi: 10.1016/j.biopsych.2009.06.027
Pascual-Leone, A., Valls-Solé, J., Wassermann, E., and Hallett, M. (1994). Responses to rapid-rate transcranial magnetic stimulation of the human motor cortex. Brain 117, 847–858. doi: 10.1093/brain/117.4.847
Pashut, T., Wolfus, S., Friedman, A., Lavidor, M., Bar-Gad, I., Yeshurun, Y., et al. (2011). Mechanisms of magnetic stimulation of central nervous system neurons. PLoS Comput. Biol. 7:e1002022. doi: 10.1371/journal.pcbi.1002022
Peng, H., Zheng, H., Li, L., Liu, J., Zhang, Y., Shan, B., et al. (2012). High-frequency rTMS treatment increases white matter FA in the left middle frontal gyrus in young patients with treatment-resistant depression. J. Affect. Disord. 136, 249–257. doi: 10.1016/j.jad.2011.12.006
Philip, N., Barredo, J., van ’t Wout-Frank, M., Tyrka, A., Price, L., and Carpenter, L. (2018). Network Mechanisms of Clinical Response to Transcranial Magnetic Stimulation in Posttraumatic Stress Disorder and Major Depressive Disorder. Biol. Psychiatry 83, 263–272. doi: 10.1016/j.biopsych.2017.07.021
Qiu, C., Liao, W., Ding, J., Feng, Y., Zhu, C., Nie, X., et al. (2011). Regional homogeneity changes in social anxiety disorder: A resting-state fMRI study. Psychiatry Res. 194, 47–53. doi: 10.1016/j.pscychresns.2011.01.010
Riva-Posse, P., Choi, K., Holtzheimer, P., Crowell, A., Garlow, S., Rajendra, J., et al. (2018). A connectomic approach for subcallosal cingulate deep brain stimulation surgery: Prospective targeting in treatment-resistant depression. Mol. Psychiatry 23, 843–849. doi: 10.1038/mp.2017.59
Riva-Posse, P., Choi, K., Holtzheimer, P., McIntyre, C., Gross, R., Chaturvedi, A., et al. (2014). Defining critical white matter pathways mediating successful subcallosal cingulate deep brain stimulation for treatment-resistant depression. Biol. Psychiatry 76, 963–969. doi: 10.1016/j.biopsych.2014.03.029
Riva-Posse, P., Inman, C., Choi, K., Crowell, A., Gross, R., Hamann, S., et al. (2019). Autonomic arousal elicited by subcallosal cingulate stimulation is explained by white matter connectivity. Brain Stimul. 12, 743–751. doi: 10.1016/j.brs.2019.01.015
Roosevelt, R., Smith, D., Clough, R., Jensen, R., and Browning, R. (2006). Increased extracellular concentrations of norepinephrine in cortex and hippocampus following vagus nerve stimulation in the rat. Brain Res. 1119, 124–132. doi: 10.1016/j.brainres.2006.08.048
Sacher, J., Neumann, J., Fünfstück, T., Soliman, A., Villringer, A., and Schroeter, M. (2012). Mapping the depressed brain: A meta-analysis of structural and functional alterations in major depressive disorder. J. Affect. Disord. 140, 142–148. doi: 10.1016/j.jad.2011.08.001
Salanova, V., Witt, T., Worth, R., Henry, T., Gross, R., Nazzaro, J., et al. (2015). Long-term efficacy and safety of thalamic stimulation for drug-resistant partial epilepsy. Neurology 84, 1017–1025. doi: 10.1212/WNL.0000000000001334
Salomons, T., Dunlop, K., Kennedy, S., Flint, A., Geraci, J., Giacobbe, P., et al. (2014). Resting-state cortico-thalamic-striatal connectivity predicts response to dorsomedial prefrontal rTMS in major depressive disorder. Neuropsychopharmacology 39, 488–498. doi: 10.1038/npp.2013.222
Scangos, K., Khambhati, A., Daly, P., Makhoul, G., Sugrue, L., Zamanian, H., et al. (2021). Closed-loop neuromodulation in an individual with treatment-resistant depression. Nat. Med. 27, 1696–1700. doi: 10.1038/s41591-021-01480-w
Schluter, R., Jansen, J., van Holst, R., van den Brink, W., and Goudriaan, A. (2018). Differential Effects of Left and Right Prefrontal High-Frequency Repetitive Transcranial Magnetic Stimulation on Resting-State Functional Magnetic Resonance Imaging in Healthy Individuals. Brain Connect. 8, 60–67. doi: 10.1089/brain.2017.0542
Seeley, W., Menon, V., Schatzberg, A., Keller, J., Glover, G., Kenna, H., et al. (2007). Dissociable intrinsic connectivity networks for salience processing and executive control. J. Neurosci. 27, 2349–2356. doi: 10.1523/JNEUROSCI.5587-06.2007
Sheline, Y., Price, J., Yan, Z., and Mintun, M. (2010). Resting-state functional MRI in depression unmasks increased connectivity between networks via the dorsal nexus. Proc. Natl. Acad. Sci. U.S.A. 107, 11020–11025. doi: 10.1073/pnas.1000446107
Souery, D., Papakostas, G. I., and Trivedi, M. H. (2006). Treatment-Resistant Depression. J. Clin. Psychiatry 67(Suppl. 6) 16–22.
Sridharan, D., Levitin, D., and Menon, V. (2008). A critical role for the right fronto-insular cortex in switching between central-executive and default-mode networks. Proc. Natl. Acad. Sci. U.S.A. 105, 12569–12574. doi: 10.1073/pnas.0800005105
Stuhrmann, A., Suslow, T., and Dannlowski, U. (2011). Facial emotion processing in major depression: A systematic review of neuroimaging findings. Biol. Mood Anxiety Disord. 1:10. doi: 10.1186/2045-5380-1-10
Sylvester, C., Corbetta, M., Raichle, M., Rodebaugh, T., Schlaggar, B., Sheline, Y., et al. (2012). Functional network dysfunction in anxiety and anxiety disorders. Trends Neurosci. 35, 527–535. doi: 10.1016/j.tins.2012.04.012
Tofts, P. (1990). The distribution of induced currents in magnetic stimulation of the nervous system. Phys. Med Biol. 35, 1119–1128. doi: 10.1088/0031-9155/35/8/008
Treadway, M., and Zald, D. (2011). Reconsidering anhedonia in depression: Lessons from translational neuroscience. Neurosci. Biobehav. Rev. 35, 537–555. doi: 10.1016/j.neubiorev.2010.06.006
Tsolaki, E., Espinoza, R., and Pouratian, N. (2017). Using probabilistic tractography to target the subcallosal cingulate cortex in patients with treatment resistant depression. Psychiatry Res. Neuroimaging 261, 72–74. doi: 10.1016/j.pscychresns.2017.01.006
Tsolaki, E., Narr, K., Espinoza, R., Wade, B., Hellemann, G., Kubicki, A., et al. (2021). Subcallosal Cingulate Structural Connectivity Differs in Responders and Nonresponders to Electroconvulsive Therapy. Biol. Psychiatry Cogn. Neurosci. Neuroimaging 6, 10–19. doi: 10.1016/j.bpsc.2020.05.010
Uddin, L. (2015). Salience processing and insular cortical function and dysfunction. Nat. Rev. Neurosci. 16, 55–61. doi: 10.1038/nrn3857
Wada, M., Nakajima, S., Honda, S., Takano, M., Taniguchi, K., Tsugawa, S., et al. (2022). Reduced signal propagation elicited by frontal transcranial magnetic stimulation is associated with oligodendrocyte abnormalities in treatment-resistant depression. J. Psychiatry Neurosci. 47, E325–E335. doi: 10.1503/jpn.220102
Williams, L. M. (2016). Precision psychiatry: A neural circuit taxonomy for depression and anxiety. Lancet Psychiatry 3, 472–480. doi: 10.1016/S2215-0366(15)00579-9
Williams, N., Bentzley, B., Hopkins, T., Pannu, J., Sahlem, G., Takacs, I., et al. (2018). Optimization of epidural cortical stimulation for treatment-resistant depression. Brain Stimul. 11, 239–240. doi: 10.1016/j.brs.2017.09.001
Williams, N., Short, E., Hopkins, T., Bentzley, B., Sahlem, G., Pannu, J., et al. (2016). Five-Year Follow-Up of Bilateral Epidural Prefrontal Cortical Stimulation for Treatment-Resistant Depression. Brain Stimul. 9, 897–904. doi: 10.1016/j.brs.2016.06.054
Williams, N., Sudheimer, K., Cole, E., Varias, A., Goldstein-Piekarski, A., Stetz, P., et al. (2021). Accelerated neuromodulation therapy for Obsessive-Compulsive Disorder. Brain Stimul. 14, 435–437. doi: 10.1016/j.brs.2021.02.013
Wongsarnpigoon, A., and Grill, W. (2008). Computational modeling of epidural cortical stimulation. J. Neural Eng. 5, 443–454. doi: 10.1088/1741-2560/5/4/009
Yan, H., Elkaim, L., Loh, A., Boutet, A., Germann, J., Elias, G., et al. (2021). Lesions causing self-injurious behavior engage putative networks modulated by deep brain stimulation. Brain Stimul. 14, 273–276. doi: 10.1016/j.brs.2021.01.009
Keywords: deep brain stimulation, epidural cortical stimulation, neuromodulation, transcranial magnetic stimulation (repetitive), salience network, treatment-resistant depression, electroconvulsive therapy
Citation: Idlett-Ali SL, Salazar CA, Bell MS, Short EB and Rowland NC (2023) Neuromodulation for treatment-resistant depression: Functional network targets contributing to antidepressive outcomes. Front. Hum. Neurosci. 17:1125074. doi: 10.3389/fnhum.2023.1125074
Received: 26 December 2022; Accepted: 14 February 2023;
Published: 02 March 2023.
Edited by:
Sandra Carvalho, University of Aveiro, PortugalReviewed by:
Randall Espinoza, University of California, Los Angeles, United StatesKi Sueng Choi, Icahn School of Medicine at Mount Sinai, United States
Copyright © 2023 Idlett-Ali, Salazar, Bell, Short and Rowland. This is an open-access article distributed under the terms of the Creative Commons Attribution License (CC BY). The use, distribution or reproduction in other forums is permitted, provided the original author(s) and the copyright owner(s) are credited and that the original publication in this journal is cited, in accordance with accepted academic practice. No use, distribution or reproduction is permitted which does not comply with these terms.
*Correspondence: Shaquia L. Idlett-Ali, c2hhcXVpYS5pZGxldHQtYWxpQGN1YW5zY2h1dHouZWR1