- 1Department of Community Medicine and Rehabilitation, Physiotherapy, Umeå University, Umeå, Sweden
- 2Umeå Center for Functional Brain Imaging (UFBI), Umeå University, Umeå, Sweden
- 3Department of Integrative Medical Biology, Umeå University, Umeå, Sweden
- 4Department of Statistics, Umeå School of Business, Economics and Statistics, Umeå University, Umeå, Sweden
- 5Department of Radiation Sciences, Diagnostic Radiology, Umeå University, Umeå, Sweden
- 6Danish Research Centre for Magnetic Resonance, Centre for Functional and Diagnostic Imaging and Research, Copenhagen University Hospital Amager and Hvidovre, Hvidovre, Denmark
- 7Department of Neurology, Institute of Sports Medicine Copenhagen (ISMC), Copenhagen University Hospital Bispebjerg, Copenhagen, Denmark
- 8Faculty of Medical and Health Sciences, Institute for Clinical Medicine, University of Copenhagen, Copenhagen, Denmark
Background: Autonomous motivation to exercise occurs when the activity is voluntary and with a perceived inherent satisfaction from the activity itself. It has been suggested that autonomous motivation is related to striatal dopamine D2/3-receptor (D2/3R) availability within the brain. In this study, we hypothesized that D2/3R availability in three striatal regions (nucleus accumbens, caudate nucleus, and putamen) would be positively associated with self-reported autonomous motivation to exercise. We also examined this relationship with additional exploratory analyses across a set of a priori extrastriatal regions of interest (ROI).
Methods: Our sample comprised 49 older adults (28 females) between 64 and 78 years of age. The D2/3R availability was quantified from positron emission tomography using the non-displaceable binding potential of [11C]-raclopride ligand. The exercise-related autonomous motivation was assessed with the Swedish version of the Behavioral Regulations in Exercise Questionnaire-2.
Results: No significant associations were observed between self-reported autonomous motivation to exercise and D2/3R availability within the striatum (nucleus accumbens, caudate nucleus, and putamen) using semi-partial correlations controlling for ROI volume on D2/3R availability. For exploratory analyses, positive associations were observed for the superior (r = 0.289, p = 0.023) and middle frontal gyrus (r = 0.330, p = 0.011), but not for the inferior frontal gyrus, orbitofrontal cortex, anterior cingulate cortex, or anterior insular cortex.
Conclusion: This study could not confirm the suggested link between striatal D2/3R availability and subjective autonomous motivation to exercise among older adults. The exploratory findings, however, propose that frontal brain regions may be involved in the intrinsic regulation of exercise-related behaviors, though this has to be confirmed by future studies using a more suitable ligand and objective measures of physical activity levels.
Introduction
The beneficial effects of staying physically active throughout life are well established (Lee et al., 2012; Cunningham et al., 2020; Holmlund et al., 2020). Still, many older adults do not manage to engage in the recommended physical activity levels (Hagströmer et al., 2015; Keadle et al., 2016). A fundamental component in why people succeed or fail to engage in exercise is motivation, more specifically, autonomous motivation (Teixeira et al., 2012). According to the Self-Determination Theory (SDT), one of the major motivational frameworks within physical activity and behavioral change (Rhodes et al., 2019), autonomous motivation occurs when activities are engaged for the inherent interest and satisfaction of the activity itself, being voluntary as well as aligned with our personal values (Deci and Ryan, 2000; Ryan and Deci, 2000b). The perceived internal locus of causality is central within SDT and autonomous motivation. While external motivators also facilitate behaviors, such as a monetary reward or avoidance of negative feelings such as shame or guilt, internal motivators generate the more high-quality form of autonomous motivation (Ryan and Deci, 2000b). Sensations such as delight and having fun during the activity are important characteristics of activities taxing autonomous motivation, as well as common reasons for exercising among those older adults that exercise regularly (Dacey et al., 2008; Cancela et al., 2021). Further, autonomous motivation was more positively associated with higher exercise volumes (Teixeira et al., 2012) and long-term adoption of physical exercise among older adults (Kekäläinen et al., 2018).
It has been suggested that the facilitation of autonomous motivation is supported by the dopaminergic system within the brain (Di Domenico and Ryan, 2017). Central to the neurobiology of motivation is the neurotransmitter dopamine (Botvinick and Braver, 2015), and due to its richness in dopamine receptors, the striatum is the brain region most often associated with motivation and reward processing (Haber and Knutson, 2010; Tziortzi et al., 2014). Neuroimaging studies have linked striatal dopamine to internal rewards and traits closely related to autonomous motivation, such as positive affect (Ashby et al., 1999), behavioral perseverance (Salamone and Correa, 2012, 2016), and flow (de Manzano et al., 2013), leading to the proposal that an individual’s capacity for autonomous motivation is related to dopamine receptor availability within the striatum (Di Domenico and Ryan, 2017).
Regarding dopamine and exercise, behavior selection of physical efforts in rodents has been linked to tonic dopamine signaling in the ventral striatum (Niv et al., 2007). Injections of a dopamine antagonist decreased the willingness to perform physical but not cognitive effort among mice (Hosking et al., 2015), and a reduced dopamine D2/3-receptor (D2/3R) availability down-regulated both energy expenditure and physically effortful behaviors among obese mice (Mourra et al., 2020). Similar results were recently detected when modulating dopamine homeostasis in humans (Michely et al., 2020). Also, among older adults, striatal D2/3R availability positively correlates with self-reported physical activity ratings (Köhncke et al., 2018) and cardiorespiratory fitness (Jonasson et al., 2019).
Neuroimaging research on intrinsic regulation of motivation has mainly focused on the striatum, however, there is support also for the involvement of extrastriatal regions such as the frontal cortex, anterior insular cortex, and anterior cingulate cortex (Di Domenico and Ryan, 2017; Cromwell et al., 2020). Even though not examining the direct link to dopamine, functional Magnetic Resonance Imaging (fMRI) studies have observed increased response in these frontal regions during intrinsically motivating settings (Murayama et al., 2010, 2015; Ellwood et al., 2017; Lee and Reeve, 2020). The action of dopamine within these regions has been suggested to be specifically involved in the valuation and consolidation of reward outcome projections from the striatum (Bromberg-Martin et al., 2010; Oldham et al., 2018), processes important for the maintenance and long-term perseverance of motivation (Ryan and Di Domenico, 2016; Myers et al., 2016).
Accordingly, this study aimed to examine the suggested link between dopamine receptor availability and autonomous motivation (Di Domenico and Ryan, 2017). We hypothesized that striatal D2/3R availability would be positively associated with a self-report measurement of exercise-related autonomous motivation among older adults. A secondary aim was to explore this association in a priori specified extrastriatal regions (superior frontal gyrus, middle frontal gyrus, inferior frontal gyrus, orbitofrontal gyrus, anterior insular cortex, and the anterior cingulate cortex).
Materials and methods
Participants and procedure
The present study used baseline data from the Physical Influences on Brain in Aging (PHIBRA) study (Jonasson et al., 2017). In short, the PHIBRA study recruited sixty older adults (64–78 years) via a local newspaper advertisement to participate in a 6-month exercise intervention (aerobic training or stretching and toning control). Exclusion criteria in PHIBRA included having a neurological disease, dopamine-influencing medication, a Mini-Mental State Examination (MMSE) score below 27, diabetes, regularly performing moderately high to high-intensity training, or magnetic resonance imaging (MRI)/positron emission tomography (PET)-incompatible factors such as claustrophobia or metal implants. The Swedish Ethics Review Authority approved the PHIBRA study (Umeå, Sweden; registration number: 2013-238-31M). The study was carried out per the WMA Declaration of Helsinki, and all participants provided written consent before testing.
All participants underwent a baseline data collection distributed on a total of six separate days, described in Jonasson et al. (2017), including the Behavioral Regulations in Exercise Questionnaire-2 (BREQ-2)(Markland and Tobin, 2004) to assess exercise-related motivation, and MRI and PET-scans to assess dopamine D2/3-receptor (D2/3R) availability. By routine, all structural MRI images were screened for abnormalities by a radiologist.
Autonomous motivation
Exercise-related autonomous motivation was assessed with BREQ-2 (Markland and Tobin, 2004). This questionnaire stems from the organismic integration theory within the SDT, a sub-theory focused on behavior regulation in relation to the quality of motivation (i.e., to what extent motivation is autonomous or controlled) (Ryan and Deci, 2000a). The BREQ-2 consists of 19 items measured on a five-point Likert scale, ranging from 0 (not true for me) to 4 (very true for me), examining five behavior regulation styles (intrinsic, identified, introjected, external and amotivated). The BREQ-2 has been validated with high reliability, and the Swedish version of BREQ-2 has demonstrated Cronbach’s alpha between 0.73 and 0.86 within groups of Swedish adults between 18 and 78 years of age (Weman-Josefsson et al., 2015; Lindwall et al., 2017).
Participants missing no more than one value within a domain were dealt with by imputing the participant’s average score of the items loading on the same motivational construct. Item-aggregation was used to compute a unit-weighted composite score for each motivational construct (Wilson et al., 2012). The scores for the intrinsic and identified constructs were then further averaged into a composite score of autonomous motivation (Standage et al., 2008).
Neuroimaging
Magnetic resonance imaging
Structural MRI data was acquired on a 3T 750 MR scanner (General Electric, WI, US) equipped with a 32-channel head coil. T1-weighted images were acquired using a 3D fast spoiled gradient-echo sequence (180 slices with 1 mm thickness, repetition time (TR) 8.2 ms, time to echo (TE) 3.2 ms, flip angle 12°, field of view 25 × 25 cm).
Positron emission tomography
PET data was acquired on a Discovery PET/CT 690 (General Electric, WI, US) using the non-displacable binding potential (BPND) of [11C]-raclopride ligand to quantify D2/3R availability. Head movements were minimized by individually fitted thermoplastic masks (Positocasts Thermoplastic; CIVCO medical solutions, IA, US). Initially, a low-dose helical CT-scan (20 mA, 120 kV, 0.8 s/revolution) was performed for attenuation-correction purposes. This was followed by an intravenous bolus injection of 250 MBq [11C]-raclopride, and a 55-min, 18-frame dynamic PET scan was acquired (9 × 120 s, 3 × 180 s, 3 × 260 s, 3 × 300 s) during resting-state conditions. Attenuation-, scatter- and decay-corrected PET images (slices = 47, field of view = 25 cm, 256 × 256 matrix, voxel size = 0.98 × 0.98 × 3.27 mm3) were reconstructed with the SharpIR algorithm (Bettinardi et al., 2011), and yielded a full width half maximum (FWHM) of 3.2 mm (Wallstén et al., 2013; for more detailed information about the PET-imaging acquisition, see Jonasson et al., 2019; Karalija et al., 2020).
Preprocessing
Processing of T1 images and extraction of cortical (Desikan et al., 2006) and subcortical (Fischl et al., 2002; Destrieux et al., 2010) gray matter segmentation for each region of interest (ROI) was done with Freesurfer version 6 (Figure 1). The a priori specified ROIs included the striatum, divided into three sub-regions (nucleus accumbens, caudate nucleus, and putamen). The included extrastriatal regions were based on previous findings of good test-retest reliability for the [11C]-raclopride ligand within the PHIBRA Study control group (Karalija et al., 2020), and their suggested involvement in motivational processes (Bromberg-Martin et al., 2010; Di Domenico and Ryan, 2017): superior frontal gyrus (SFG), middle frontal gyrus (MFG; rostral and caudal middle frontal), inferior frontal gyrus (IFG; pars opercularis, pars triangularis, pars orbitalis) and orbitofrontal cortex (OFC; lateral and medial orbitofrontal), the anterior insular cortex (AIC), and the anterior cingulate cortex (ACC; rostral and caudal anterior cingulate).
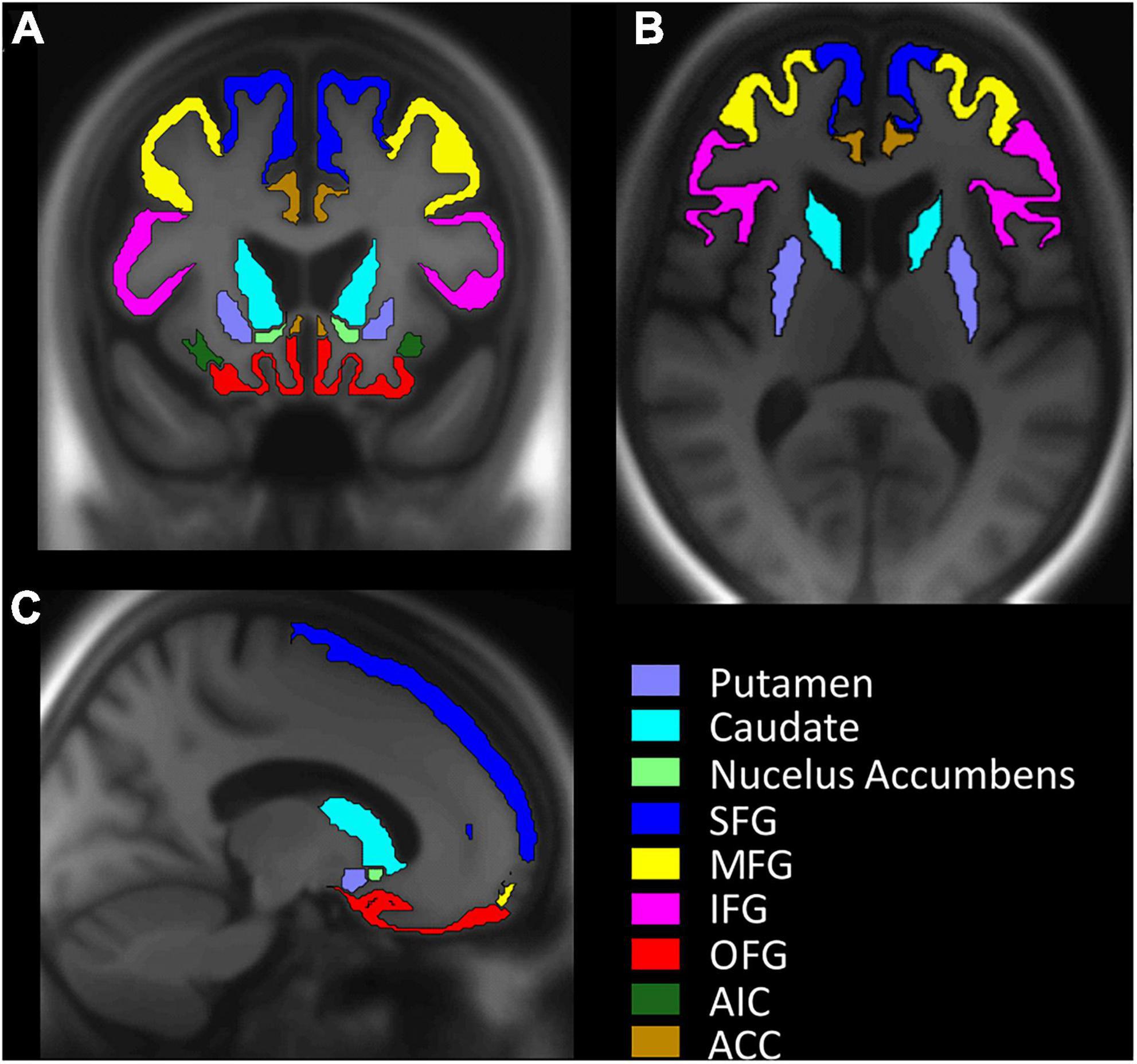
Figure 1. Visualization of gray matter segmentation for each region of interest (ROI). (A) Coronal plane. (B) Horizontal plane. (C) Sagittal plane. SFG, Superior frontal gyrus; MGF, middle frontal gyrus; IFG, inferior frontal gyrus; OFG, orbitofrontal gyrus; AIC, anterior insular cortex; ACC, anterior cingulate cortex.
Motion correction and co-registration of PET images onto T1-weighted images were performed in SPM8. BPND was calculated for each ROI using orthogonal reference Logan analysis (Logan et al., 1996) with linear regression based on the median of ROI voxel values across both hemispheres from each time frame based on frame 10–18 from time 18 to 55 min, with the cerebellum as reference region. See Supplementary Figure 2 for map of [11C]-raclopride distribution volume ratio across gray matter.
Statistical analyses
Data were prepared and analyzed using R version 4.0.5 (R Core Team, 2020) and RStudio version 1.1.463 (RStudio Team, 2016). Plots were produced using the tidyverse (v. 1.3.1) and GridExtra (v. 2.3) packages (Auguie, 2017; Wickham et al., 2019), and semi-partial correlations was performed with the MASS (v. 7.3.53.1) and ppcor (v. 1.1) packages (Kim, 2015).
To examine the association between D2/3R availability and self-reported autonomous motivation, we performed semi-partial correlations on the autonomous motivation composite score and the [11C]-raclopride BPND for each of the a priori specified regions of interest, controlling for ROI volume on BPND to account for partial volume effects. We assumed a positive direction of the association between D2/3R availability and autonomous motivation and therefore used one-tailed significance testing with an α < 0.05. To control for multiple comparisons among the exploratory analyses, we used the false discovery rate (FDR) (Benjamini and Hochberg, 1995).
Results
In the present study, we excluded 11 participants due to missing or major incomplete BREQ-2 data. Thus, complete baseline data was obtained from 49 (females, n = 28) physically inactive but otherwise healthy older adults (see Table 1). Table 2 presents descriptive data on ROI BPND and Autonomous motivation, with the ROI segmentation visualized in Figure 1.
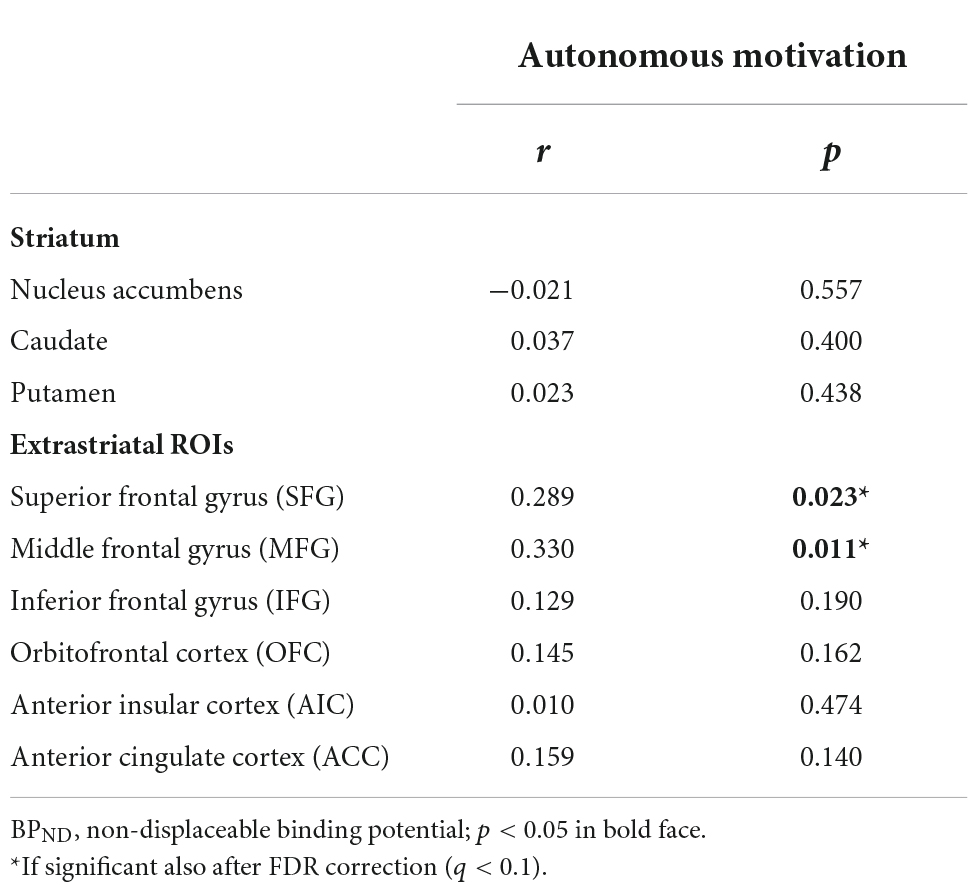
Table 2. Descriptive information of D2/3R availability as [11C]-raclopride BPND for each brain region of interest, and self-reported autonomous motivation.
No significant associations were observed for the semi-partial correlations between D2/3R availability (BPND) and self-reported exercise-related autonomous motivation within any of the three striatal regions; nucleus accumbens [r(46) = −0.021, p = 0.56], caudate [r(46) = 0.037, p = 0.40], and putamen [r(46) = 0.023, p = 0.44].
Exploratory analyses for extrastriatal regions revealed a significant positive correlation with the superior frontal gyrus [r(46) = 0.289, p = 0.023], and the middle frontal gyrus [r(46) = 0.330, p = 0.011], but not for the inferior frontal gyrus orbitofrontal gyrus, anterior insular cortex, and anterior cingulate cortex. Both significant associations survived an FDR correction of q < 0.1. See Table 3 for exact values and statistics, and Figure 2 for scatterplots of autonomous motivation and ROI BPND residuals (adjusted for ROI volume).
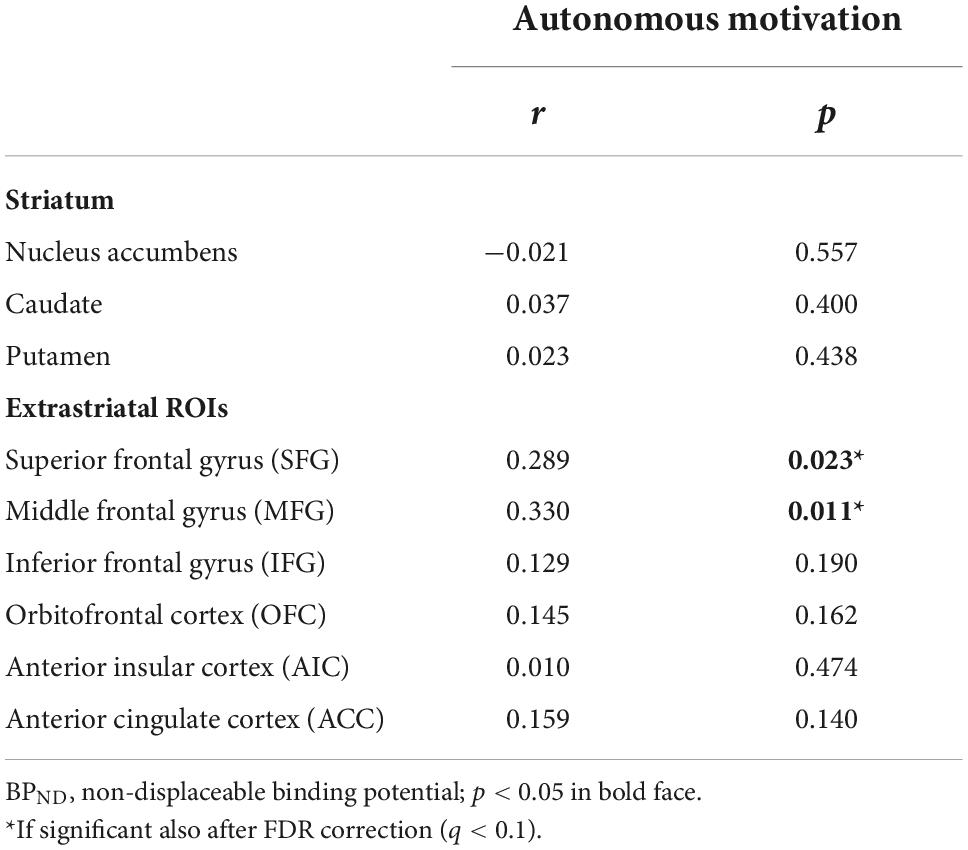
Table 3. Semi-partial correlations of ROI D2/3R availability measured as [11C]-raclopride BPND and self-reported autonomous motivation.
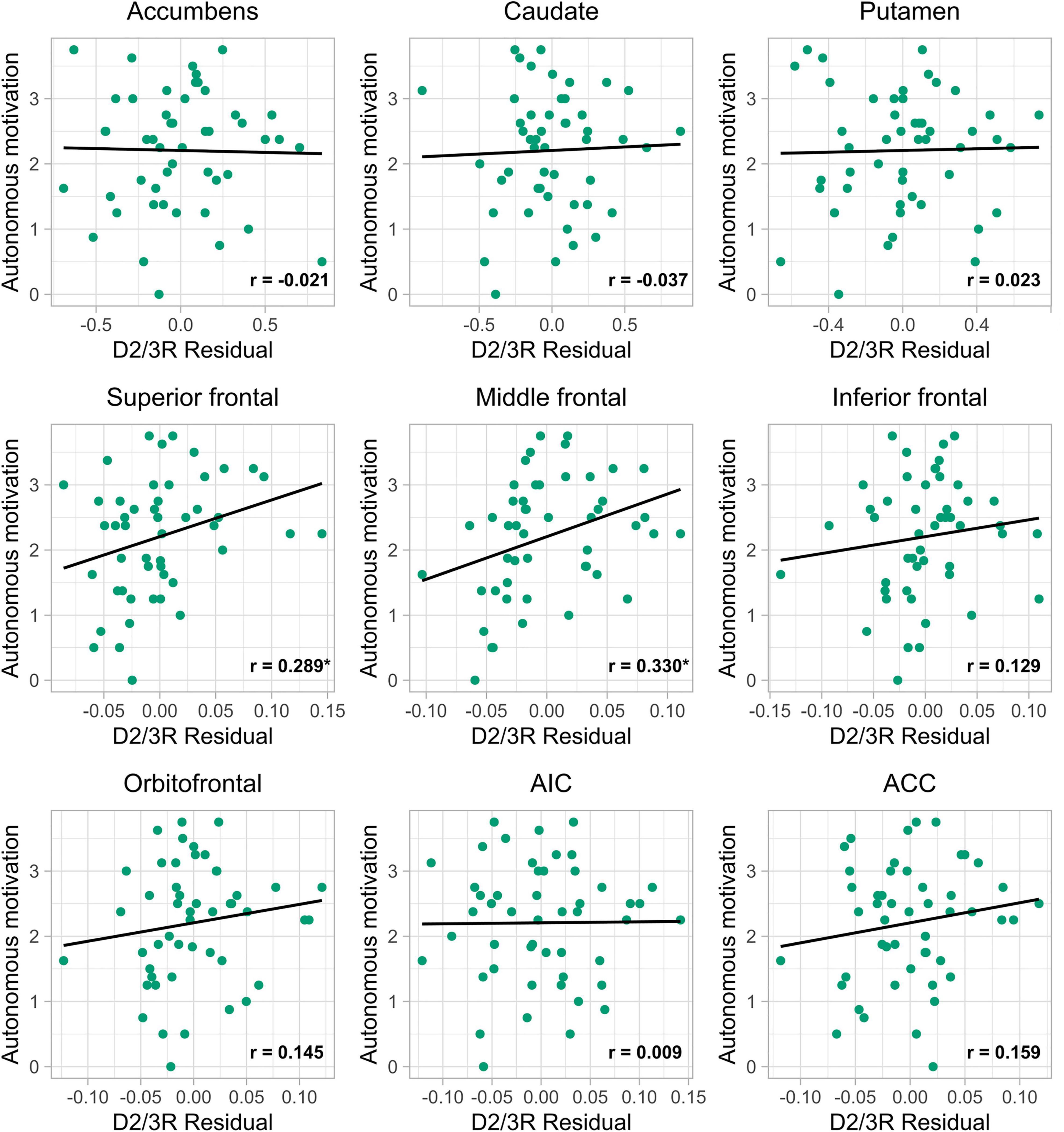
Figure 2. Scatterplots of D2/3R availability within each ROI and autonomous motivation. Self-reported autonomous motivation along the y-axis, and the residual (controlling for structural ROI volume) dopamine D2/3-receptor availability (D2/3R residual) estimated with [11C]-raclopride positron emission tomography (PET) along the x-axis. Nucleus accumbens (accumbens), caudate nucleus (caudate), superior frontal gyrus (superior frontal), middle frontal gyrus (middle frontal), inferior frontal gyrus (inferior frontal), orbitofrontal gyrus (orbitofrontal), anterior insular cortex (AIC), anterior cingulate cortex (ACC). *If p < 0.05.
Results remained essentially the same for post hoc sensitivity analyses not adjusting for ROI-volume (see Supplementary Table 1 for test statistics, and Supplementary Figure 1 for scatterplots of autonomous motivation and raw BPND data), adjusting for ROI-volume, age, and sex (Supplementary Table 2), or removing two outliers (≥ 2.5 SD in BPND change) detected during examination of longitudinal data/repeated measurements (Supplementary Table 3 controlling for ROI-volume, and Supplementary Table 4 controlling for ROI-volume, age, and sex).
An additional correlation between participants’ cardiorespiratory fitness level (VO2 peak) and self-reported autonomous motivation to exercise did not reach statistical significance [r(47) = 0.194, p = 0.181], neither when adjusted for age and sex [r(45) = 0.188, p = 0.205].
Discussion
This study examined the relationship between dopamine and autonomous motivation to exercise among older adults. We specifically aimed to examine the suggested link between D2/3R availability in the striatum and exercise-related autonomous motivation. We found no support for the hypothesis that D2/3R availability was to be positively associated with self-reported exercise-related autonomous motivation in neither of the three striatal regions (nucleus accumbens, caudate nucleus, and putamen). Exploratory analyses revealed statistically significant positive associations for the superior frontal gyrus and the medial frontal gyrus, but not for the inferior frontal gyrus, orbitofrontal gyrus, anterior insular cortex, or anterior cingulate cortex.
The absence of significant associations between striatal D2/3R availability and self-reported autonomous motivation to exercise may be explained by the striatum being more involved in acute reward processes during an activity (Bromberg-Martin et al., 2010; Olivetti et al., 2019). Striatal dopamine acts upon intrinsic and extrinsic reward stimuli (Jonasson et al., 2014; Oldham et al., 2018), and assessment of resting dopamine D2/3R availability in the striatum might not be enough to disentangle the specific influence on autonomous regulation. Also, while the acute processes are important in the generation phase of motivation, influencing maintenance and regulation of motivation, these acute aspects might not be covered by the BREQ-2 questionnaire (Markland and Tobin, 2004). This questionnaire focuses on why people engage in exercise, possibly only capturing already existing mental representations of previous exercise-related experiences necessary for future engagement of similar behaviors (Lee and Reeve, 2020). To explore the role of striatal D2/3R availability during the acute phase of internal reward processes and autonomous motivation, future studies PET studies should use a different study design, possibly including a memory recall or imaginary task designed to target autonomous motivation (Lee and Reeve, 2020). In addition, other reward-governing neurotransmitter systems influenced by exercise, for example involving opioids (Saanijoki et al., 2018) or endocannabinoids (Siebers et al., 2021) may also be relevant to explore in relation to autonomous exercise-related motivation.
Our results from the exploratory analyses suggest that D2/3R availability in the superior and middle frontal gyrus may be involved in the intrinsic regulation of autonomous motivation to exercise. These frontal regions may support the maintenance and long-term perseverance of autonomous motivation via dopamine-dependent salience processes of attention orientation toward consolidated positive memories relevant for decision-making to perform a similar activity again (Bromberg-Martin et al., 2010; Ott and Nieder, 2019). A possible explanation for why autonomously motivated individuals more successfully maintain a physically active lifestyle over time (Ryan and Deci, 2000b; Teixeira et al., 2012; Kekäläinen et al., 2018).
The suggested relationship between dopamine and autonomous motivation to exercise becomes particularly interesting in light of the known age-related deteriorations of the dopaminergic system (Karrer et al., 2017; Karalija et al., 2019). Notably, older adults with higher physical activity levels show less than expected age-related reduction in D2/3R availability (Dang et al., 2017), and inter-individual variation in dopamine-related genes that affects dopamine receptor efficacy was associated with less time spent in moderate to vigorous physical activity, and even more so among the oldest (Rosso et al., 2018; Dohrn et al., 2020). Thus, while physical exercise may have the potential to maintain or even strengthen the integrity of the dopamine system, aging as well as a person’s genetic makeup may cause difficulties in engaging in such activities due to decreased D2/3R efficacy.
The present study design cannot determine causality, and we do not address the potential exercise-related changes that might occur over time, such as plasticity mechanisms within the dopaminergic system (Jonasson et al., 2019), or the motivational profile (Kekäläinen et al., 2018). Still, we propose that individuals with higher D2/3R availability in frontal regions can, to a greater extent, rely more on autonomous motivation processes. In contrast, those with lower levels might be more dependent on controlled processes and external motivators. In addition to other factors important for successful exercise routines [e.g., environmental settings, external expectations, consolidated habits or social norms (Pelssers et al., 2018; Aaltonen et al., 2020; Jones et al., 2020; Cancela et al., 2021)], future studies should acknowledge that the individual variation in motivation might also be linked to biological differences in the dopaminergic system, and that aging per se might affect this system and shift the motivational profile (Yee et al., 2019).
It should be noted that the absence of associations between striatal D2/3R and subjective exercise-related autonomous motivation, and between autonomous motivation and VO2 peak, were despite a significant relationship between striatal D2/3R and VO2 peak previously observed (Jonasson et al., 2019). Striatal D2/3R among older adults has also been related to self-reported physical activity, with a positive association for higher levels of physical activity intensity but not physical activity volume (Köhncke et al., 2018). Possibly, frontal D2/3R and autonomous motivation operate in a parallel process to that of striatal D2/3R and physical fitness, with frontal D2/3R more involved in whether exercise will be performed or not (i.e., volume) rather than how it is performed (i.e., intensity). These remaining questions need to be addressed in future studies, in which it also should be included additional objective measures of physical activity, such as accelerometer data, to examine the intricate interplay of dopamine, motivation to exercise, and different activity levels.
Limitations
This study has limitations. First, the validity of [11C]-raclopride for BPND quantification in extrastriatal regions has recently been questioned (Svensson et al., 2019; Freiburghaus et al., 2021), emphasizing that the interpretation of [11C]-raclopride BPND in frontal regions should be made with caution (Freiburghaus et al., 2021). There is, however, evidence of high test-retest reliability (Alakurtti et al., 2015; Karalija et al., 2020), good measurement properties when linking functionally connected cortical areas in latent space (Papenberg et al., 2019), and strong linear relationships between [11C]-raclopride D2/3R estimates in cortical regions (Papenberg et al., 2019) with D2/3R measured in the post-mortem brain (Hall et al., 1994), all which speaks to the feasibility of using [11C]-raclopride to assess extrastriatal D2/3R in the living brain. Of note, in a subsample of the current study sample, the extrastriatal regions included have presented high test-retest reliability (superior frontal gurys, ICC: 0.93, r = 0.88; middle frontal gurys, ICC: 0.96, r = 0.93), and a coefficient of variance between 18.4 and 24.5% (Karalija et al., 2020). Still, we recommend that future studies examining extrastriatal D2/3R include a ligand with higher sensitivity, such as [11C]FLB457 (Freiburghaus et al., 2021). Second, with the [11C]-raclopride BPND we only assess receptor availability and therefore cannot conclude about the number of receptors (i.e., density), nor about the level of endogenous dopamine bound to the receptors. Hence, if motivated subjects have higher resting synaptic dopamine levels, this could potentially reduce D2/3R availability in these individuals and consequently reduce the estimated association between D2/3R availability and autonomous motivation. Third, our sample consisted of a group of non-exercising older adults that were interested (and motivated) in participating in a 6-month exercise intervention. Although our sample portrayed the whole range of autonomous motivation with BREQ-2 scores ranging from low to high, all participants had some degree of motivation to participate in an exercise intervention and this may have narrowed the autonomous motivation range in this study. Further exploration of autonomous motivation in relation to dopamine should also be done within more heterogeneous groups of older individuals. Fourth, a larger sample size would allow for the possibility of performing subgroup analyses not possible in the present study, such as comparing the different domains within the SDT (Chemolli and Gagné, 2014), genetic variations, gender differences, and physical activity levels (Dacey et al., 2008; Aaltonen et al., 2020). Finally, although widely used, the BREQ-2 questionnaire (Markland and Tobin, 2004) excludes the third regulation style of autonomous motivation in the SDT, integrated motivation. This part of the autonomous motivation spectrum has been considered highly relevant for maintaining physical activity and exercise (Wilson et al., 2012; Miquelon and Castonguay, 2017). However, concerns regarding high inter-factor correlations favor assessment only of the intrinsic and identified scales, as is done in the BREQ-2 questionnaire (Howard et al., 2017). In summary, future studies could preferably include additional measures of dopamine, larger sample sizes, and alternative designs to further explore and disentangle the biological mechanisms of autonomous motivation.
Conclusion
To summarize, D2/3R availability in the striatum was not associated with self-reported exercise-related autonomous motivation. The exploratory analyses suggest that D2/3R availability in the superior and middle frontal gyrus may be involved in the intrinsic regulation of exercise-related behaviors, potentially via salient processes enhancing decision-making and long-term maintenance. This suggestion should preferably be confirmed in larger studies, including a different assessment of D2/3R availability. Still, studies aimed at increasing motivation and engagement in physical activity among older adults could benefit from considering the possible variations in the support needed due to aging and inter-individual biological differences in dopamine. While some individuals might have better prerequisites to develop and consolidate autonomous forms of motivation to exercise, others might need more external support over time.
Data availability statement
The datasets used and analyzed during the current study are available from the corresponding author on reasonable request. Requests to access these datasets should be directed to ES, ZW1tYS5zaW1vbnNzb25AdW11LnNl.
Ethics statement
The studies involving human participants were reviewed and approved by the Swedish Ethics Review Authority (Umeå, Sweden; registration number: 2013-238-111 31M). The patients/participants provided their written informed consent to participate in this study.
Author contributions
ES performed the statistical analyses, initial interpretations of the results, and drafting of the manuscript. C-JB contributed during analyses, interpretations of the results, and editing of the manuscript. AL assisted during the analyses and interpretations of the results. LJS, ER, MH, and NL contributed equally to the interpretations of the results and editing of the manuscript. All authors read and approved the final manuscript.
Funding
This work was supported by the Swedish Research Council (grant no. 2012–00530), the Västerbotten County Council, the Swedish Research Council for Sport Science, the Umeå School of Sport Sciences, and the Kamprad Family Foundation.
Acknowledgments
We thank Lars Nyberg for valuable comments on earlier drafts and the staff at Umeå Center for Functional Brain Imaging (UFBI) and the University Hospital of Umeå for providing excellent research conditions. This study was accomplished within the context of the Swedish National Graduate School for Competitive Science on Ageing and Health (SWEAH) funded by the Swedish Research Council.
Conflict of interest
The authors declare that the research was conducted in the absence of any commercial or financial relationships that could be construed as a potential conflict of interest.
Publisher’s note
All claims expressed in this article are solely those of the authors and do not necessarily represent those of their affiliated organizations, or those of the publisher, the editors and the reviewers. Any product that may be evaluated in this article, or claim that may be made by its manufacturer, is not guaranteed or endorsed by the publisher.
Supplementary material
The Supplementary Material for this article can be found online at: https://www.frontiersin.org/articles/10.3389/fnhum.2022.997131/full#supplementary-material
References
Aaltonen, S., Waller, K., Vähä-Ypyä, H., Rinne, J., Sievänen, H., Silventoinen, K., et al. (2020). Motives for physical activity in older men and women: A twin study using accelerometer-measured physical activity. Scand. J. Med. Sci. Sports 30, 1409–1422. doi: 10.1111/sms.13673
Alakurtti, K., Johansson, J. J., Joutsa, J., Laine, M., Bäckman, L., Nyberg, L., et al. (2015). Long-Term Test–Retest Reliability of Striatal and Extrastriatal Dopamine D 2/3 Receptor Binding: Study with [11 C]Raclopride and High-Resolution PET. J. Cereb. Blood Flow Metab. 35, 1199–1205. doi: 10.1038/jcbfm.2015.53
Ashby, F. G., Isen, A. M., and Turken, A. U. (1999). A neuropsychological theory of positive affect and its influence on cognition. Psychol. Rev. 106, 529–550. doi: 10.1037/0033-295X.106.3.529
Benjamini, Y., and Hochberg, Y. (1995). Controlling the False Discovery Rate: A Practical and Powerful Approach to Multiple Testing. J. R. Stat. Soc. Ser. B 57, 289–300. doi: 10.1111/j.2517-6161.1995.tb02031.x
Bettinardi, V., Presotto, L., Rapisarda, E., Picchio, M., Gianolli, L., and Gilardi, M. C. (2011). Physical Performance of the new hybrid PETCT Discovery-690. Med. Phys. 38, 5394–5411. doi: 10.1118/1.3635220
Botvinick, M., and Braver, T. (2015). Motivation and Cognitive Control: From Behavior to Neural Mechanism. Annu. Rev. Psychol. 66, 83–113. doi: 10.1146/annurev-psych-010814-015044
Bromberg-Martin, E. S., Matsumoto, M., and Hikosaka, O. (2010). Dopamine in Motivational Control: Rewarding, Aversive, and Alerting. Neuron 68, 815–834. doi: 10.1016/j.neuron.2010.11.022
Cancela, J. M., Pereira, K., Mollinedo, I., Ferreira, M., and Bezerra, P. (2021). Reasons Why Older Adults Engage in Physical Exercise. Comparative Study Eastern Europe Versus Southern Europe. J. Aging Phys. Act. 29, 43–50. doi: 10.1123/japa.2019-0450
Chemolli, E., and Gagné, M. (2014). Evidence against the continuum structure underlying motivation measures derived from self-determination theory. Psychol. Assess. 26, 575–585. doi: 10.1037/a0036212
Cromwell, H. C., Abe, N., Barrett, K. C., Caldwell-Harris, C., Gendolla, G. H. E., Koncz, R., et al. (2020). Mapping the interconnected neural systems underlying motivation and emotion: A key step toward understanding the human affectome. Neurosci. Biobehav. Rev. 113, 204–226. doi: 10.1016/j.neubiorev.2020.02.032
Cunningham, C., O’ Sullivan, R., Caserotti, P., and Tully, M. A. (2020). Consequences of physical inactivity in older adults: A systematic review of reviews and meta-analyses. Scand. J. Med. Sci. Sports 30, 816–827. doi: 10.1111/sms.13616
Dacey, M., Baltzell, A., and Zaichkowsky, L. (2008). Older adults’ intrinsic and extrinsic motivation toward physical activity. Am. J. Health Behav. 32, 570–582. doi: 10.5993/AJHB.32.6.2
Dang, L. C., Castrellon, J. J., Perkins, S. F., Le, N. T., Cowan, R. L., Zald, D. H., et al. (2017). Reduced effects of age on dopamine D2 receptor levels in physically active adults. Neuroimage 148, 123–129. doi: 10.1016/j.neuroimage.2017.01.018
de Manzano, Ö, Cervenka, S., Jucaite, A., Hellenäs, O., Farde, L., and Ullén, F. (2013). Individual differences in the proneness to have flow experiences are linked to dopamine D2-receptor availability in the dorsal striatum. Neuroimage 67, 1–6. doi: 10.1016/j.neuroimage.2012.10.072
Deci, E. L., and Ryan, R. M. (2000). The “What” and “Why” of Goal Pursuits: Human Needs and the Self-Determination of Behavior. Psychol. Inq. 11, 227–268. doi: 10.1207/S15327965PLI1104_01
Desikan, R. S., Ségonne, F., Fischl, B., Quinn, B. T., Dickerson, B. C., Blacker, D., et al. (2006). An automated labeling system for subdividing the human cerebral cortex on MRI scans into gyral based regions of interest. Neuroimage 31, 968–980. doi: 10.1016/j.neuroimage.2006.01.021
Destrieux, C., Fischl, B., Dale, A., and Halgren, E. (2010). Automatic parcellation of human cortical gyri and sulci using standard anatomical nomenclature. Neuroimage 53, 1–15. doi: 10.1016/j.neuroimage.2010.06.010
Di Domenico, S. I., and Ryan, R. M. (2017). The emerging neuroscience of intrinsic motivation: A new frontier in self-determination research. Front. Hum. Neurosci. 11:145. doi: 10.3389/fnhum.2017.00145
Dohrn, I. M., Papenberg, G., Winkler, E., and Welmer, A. K. (2020). Impact of dopamine-related genetic variants on physical activity in old age - A cohort study. Int. J. Behav. Nutr. Phys. Act. 17:68. doi: 10.1186/s12966-020-00971-2
Ellwood, I. T., Patel, T., Wadia, V., Lee, A. T., Liptak, A. T., Bender, K. J., et al. (2017). Tonic or phasic stimulation of dopaminergic projections to prefrontal cortex causes mice to maintain or deviate from previously learned behavioral strategies. J. Neurosci. 37, 8315–8329. doi: 10.1523/JNEUROSCI.1221-17.2017
Fischl, B., Salat, D. H., Busa, E., Albert, M., Dieterich, M., Haselgrove, C., et al. (2002). Whole brain segmentation: Automated labeling of neuroanatomical structures in the human brain. Neuron 33, 341–355. doi: 10.1016/S0896-6273(02)00569-X
Freiburghaus, T., Svensson, J. E., Matheson, G. J., Plavén-Sigray, P., Lundberg, J., Farde, L., et al. (2021). Low convergent validity of [11C]raclopride binding in extrastriatal brain regions: A PET study of within-subject correlations with [11C]FLB 457. Neuroimage 226:117523. doi: 10.1016/j.neuroimage.2020.117523
Haber, S. N., and Knutson, B. (2010). The reward circuit: Linking primate anatomy and human imaging. Neuropsychopharmacology 35, 4–26. doi: 10.1038/npp.2009.129
Hagströmer, M., Kwak, L., Oja, P., and Sjöström, M. (2015). A 6 year longitudinal study of accelerometer-measured physical activity and sedentary time in Swedish adults. J. Sci. Med. Sport 18, 553–557. doi: 10.1016/j.jsams.2014.07.012
Hall, H., Sedvall, G., Magnusson, O., Kopp, J., Halldin, C., and Farde, L. (1994). Distribution of D1- and D2-Dopamine Receptors, and Dopamine and Its Metabolites in the Human Brain. Neuropsychopharmacology 11, 245–256. doi: 10.1038/sj.npp.1380111
Holmlund, T., Ekblom, B., Börjesson, M., Andersson, G., Wallin, P., and Ekblom-Bak, E. (2020). Association between change in cardiorespiratory fitness and incident hypertension in Swedish adults. Eur. J. Prev. Cardiol. 28, 1515–1522. doi: 10.1177/2047487320942997
Hosking, J. G., Floresco, S. B., and Winstanley, C. A. (2015). Dopamine antagonism decreases willingness to expend physical, but not cognitive, effort: A comparison of two rodent cost/benefit decision-making tasks. Neuropsychopharmacology 40, 1005–1015. doi: 10.1038/npp.2014.285
Howard, J. L., Gagné, M., and Bureau, J. S. (2017). Testing a continuum structure of self-determined motivation: A meta-analysis. Psychol. Bull. 143, 1346–1377. doi: 10.1037/bul0000125
Jonasson, L. S., Axelsson, J., Riklund, K., Braver, T. S., Ögren, M., Bäckman, L., et al. (2014). Dopamine release in nucleus accumbens during rewarded task switching measured by [11C]raclopride. Neuroimage 99, 357–364. doi: 10.1016/j.neuroimage.2014.05.047
Jonasson, L. S., Nyberg, L., Axelsson, J., Kramer, A. F., Riklund, K., and Boraxbekk, C. J. (2019). Higher striatal D2-receptor availability in aerobically fit older adults but non-selective intervention effects after aerobic versus resistance training. Neuroimage 202:116044. doi: 10.1016/j.neuroimage.2019.116044
Jonasson, L. S., Nyberg, L., Kramer, A. F., Lundquist, A., Riklund, K., and Boraxbekk, C.-J. (2017). Aerobic Exercise Intervention, Cognitive Performance, and Brain Structure: Results from the Physical Influences on Brain in Aging (PHIBRA) Study. Front. Aging Neurosci. 8:336. doi: 10.3389/fnagi.2016.00336
Jones, S. A., Alicea, S. K., and Ortega, J. D. (2020). A Self-Determination Theory Approach for Exercise Motivation in Rural Dwelling Older Adults. Act. Adapt. Aging 44, 24–41. doi: 10.1080/01924788.2019.1581022
Karalija, N., Jonassson, L., Johansson, J., Papenberg, G., Salami, A., Andersson, M., et al. (2020). High long-term test–retest reliability for extrastriatal 11 C-raclopride binding in healthy older adults. J. Cereb. Blood Flow Metab. 40, 1859–1868. doi: 10.1177/0271678X19874770
Karalija, N., Wåhlin, A., Ek, J., Rieckmann, A., Papenberg, G., Salami, A., et al. (2019). Cardiovascular factors are related to dopamine integrity and cognition in aging. Ann. Clin. Transl. Neurol. 6, 2291–2303. doi: 10.1002/acn3.50927
Karrer, T. M., Josef, A. K., Mata, R., Morris, E. D., and Samanez-Larkin, G. R. (2017). Reduced dopamine receptors and transporters but not synthesis capacity in normal aging adults: A meta-analysis. Neurobiol. Aging 57, 36–46. doi: 10.1016/j.neurobiolaging.2017.05.006
Keadle, S. K., McKinnon, R., Graubard, B. I., and Troiano, R. P. (2016). Prevalence and trends in physical activity among older adults in the United States: A comparison across three national surveys. Prev. Med. 89, 37–43. doi: 10.1016/j.ypmed.2016.05.009
Kekäläinen, T., Kokko, K., Tammelin, T., Sipilä, S., and Walker, S. (2018). Motivational characteristics and resistance training in older adults: A randomized controlled trial and 1-year follow-up. Scand. J. Med. Sci. Sport 28, 2416–2426. doi: 10.1111/sms.13236
Kim, S. (2015). ppcor: An R Package for a Fast Calculation to Semi-partial Correlation Coefficients. Commun. Stat. Appl. Methods 22, 665–674. doi: 10.5351/CSAM.2015.22.6.665
Köhncke, Y., Papenberg, G., Jonasson, L., Karalija, N., Wåhlin, A., Salami, A., et al. (2018). Self-rated intensity of habitual physical activities is positively associated with dopamine D2/3 receptor availability and cognition. Neuroimage 181, 605–616. doi: 10.1016/j.neuroimage.2018.07.036
Lee, I. M., Shiroma, E. J., Lobelo, F., Puska, P., Blair, S. N., Katzmarzyk, P. T., et al. (2012). Effect of physical inactivity on major non-communicable diseases worldwide: An analysis of burden of disease and life expectancy. Lancet 380, 219–229. doi: 10.1016/S0140-6736(12)61031-9
Lee, W., and Reeve, J. (2020). Remembering pleasure and personal meaning from episodes of intrinsic motivation: An fMRI study. Motiv. Emot. 44, 810–818. doi: 10.1007/s11031-020-09855-1
Lindwall, M., Ivarsson, A., Weman-Josefsson, K., Jonsson, L., Ntoumanis, N., Patrick, H., et al. (2017). Stirring the motivational soup: Within-person latent profiles of motivation in exercise. Int. J. Behav. Nutr. Phys. Act. 14:4. doi: 10.1186/s12966-017-0464-4
Logan, J., Fowler, J. S., Volkow, D., Wang, G., Ding, Y., and Alexoff, D. L. (1996). Distribution Volume Ratios Without Blood Sampling from Graphical Analysis of PET Data. J. Cereb. Blood Flow Metab. 16, 834–840. doi: 10.20680/aees.10.0_55
Markland, D., and Tobin, V. (2004). A modification to the behavioural regulation in exercise questionnaire to include an assessment of amotivation. J. Sport Exerc. Psychol. 26, 191–196. doi: 10.1123/jsep.26.2.191
Michely, J., Viswanathan, S., Hauser, T. U., Delker, L., Dolan, R. J., and Grefkes, C. (2020). The role of dopamine in dynamic effort-reward integration. Neuropsychopharmacology 45, 1448–1453. doi: 10.1038/s41386-020-0669-0
Miquelon, P., and Castonguay, A. (2017). Integrated regulation, behavior consistency, and physical activity maintenance. Motiv. Sci. 3, 76–90. doi: 10.1037/mot0000049
Mourra, D., Gnazzo, F., Cobos, S., and Beeler, J. A. (2020). Striatal Dopamine D2 Receptors Regulate Cost Sensitivity and Behavioral Thrift. Neuroscience 425, 134–145. doi: 10.1016/j.neuroscience.2019.11.002
Murayama, K., Matsumoto, M., Izuma, K., and Matsumoto, K. (2010). Neural basis of the undermining effect of monetary reward on intrinsic motivation. Proc. Natl. Acad. Sci. U. S. A. 107, 20911–20916. doi: 10.1073/pnas.1013305107
Murayama, K., Matsumoto, M., Izuma, K., Sugiura, A., Ryan, R. M., Deci, E. L., et al. (2015). How Self-Determined Choice Facilitates Performance: A Key Role of the Ventromedial Prefrontal Cortex. Cereb. Cortex 25, 1241–1251. doi: 10.1093/cercor/bht317
Myers, C. A., Wang, C., Black, J. M., Bugescu, N., and Hoeft, F. (2016). The matter of motivation: Striatal resting-state connectivity is dissociable between grit and growth mindset. Soc. Cogn. Affect. Neurosci. 11, 1521–1527. doi: 10.1093/scan/nsw065
Niv, Y., Daw, N. D., Joel, D., and Dayan, P. (2007). Tonic dopamine: Opportunity costs and the control of response vigor. Psychopharmacology 191, 507–520. doi: 10.1007/s00213-006-0502-4
Oldham, S., Murawski, C., Fornito, A., Youssef, G., Yücel, M., and Lorenzetti, V. (2018). The anticipation and outcome phases of reward and loss processing: A neuroimaging meta-analysis of the monetary incentive delay task. Hum. Brain Mapp. 39, 3398–3418. doi: 10.1002/hbm.24184
Olivetti, P. R., Balsam, P. D., Simpson, E. H., and Kellendonk, C. (2019). Emerging roles of striatal dopamine D2 receptors in motivated behaviour: Implications for psychiatric disorders. Basic Clin. Pharmacol. Toxicol. 126, 47–55. doi: 10.1111/bcpt.13271
Ott, T., and Nieder, A. (2019). Dopamine and Cognitive Control in Prefrontal Cortex. Trends Cogn. Sci. 23, 213–234. doi: 10.1016/j.tics.2018.12.006
Papenberg, G., Jonasson, L., Karalija, N., Johansson, J., Köhncke, Y., Salami, A., et al. (2019). Mapping the landscape of human dopamine D2/3 receptors with [11C]raclopride. Brain Struct. Funct. 224, 2871–2882. doi: 10.1007/s00429-019-01938-1
Pelssers, J., Hurkmans, E., Scheerder, J., Vanbeselaere, N., Vos, S., Smits, T., et al. (2018). Acting one’s age in physical exercise: Do perceived age norms explain autonomous motivation among older adults? J. Aging Phys. Act. 26, 521–529. doi: 10.1123/japa.2016-0290
Rhodes, R. E., McEwan, D., and Rebar, A. L. (2019). Theories of physical activity behaviour change: A history and synthesis of approaches. Psychol. Sport Exerc. 42, 100–109. doi: 10.1016/j.psychsport.2018.11.010
Rosso, A. L., Metti, A. L., Glynn, N. W., Boudreau, R. M., Rejeski, W. J., Bohnen, N., et al. (2018). Dopamine-Related Genotypes and Physical Activity Change During an Intervention: The Lifestyle Interventions and Independence for Elders Study. J. Am. Geriatr. Soc. 66, 1172–1179. doi: 10.1111/jgs.15369
Ryan, R. M., and Deci, E. L. (2000b). Self-determination theory and the facilitation of intrinsic motivation, social development, and well-being. Am. Psychol. 55, 68–78. doi: 10.1037/0003-066X.55.1.68
Ryan, R. M., and Deci, E. L. (2000a). Intrinsic and Extrinsic Motivations: Classic Definitions and New Directions. Contemp. Educ. Psychol. 25, 54–67. doi: 10.1006/ceps.1999.1020
Ryan, R. M., and Di Domenico, S. I. (2016). “Epilogue - Distinct motivations and their differentiated mechanisms: reflections on the emerging neuroscience of human motivation,” in Recent developments in neuroscience research on human motivation (Advances in motivation and achievement), Vol. 19, eds S. Kim, J. Reeve, and M. Bong (Bingley: Emerald Group Publishing Limited), 349–369.
Saanijoki, T., Tuominen, L., Tuulari, J. J., Nummenmaa, L., Arponen, E., Kalliokoski, K., et al. (2018). Opioid release after high-intensity interval training in healthy human subjects. Neuropsychopharmacology 43, 246–254. doi: 10.1038/npp.2017.148
Salamone, J. D., and Correa, M. (2012). The Mysterious Motivational Functions of Mesolimbic Dopamine. Neuron 76, 470–485. doi: 10.1016/j.neuron.2012.10.021
Salamone, J. D., and Correa, M. (2016). “Neurobiology of effort and the role of mesolimbic dopamine,” in Recent developments in neuroscience research on human motivation, eds S. Kim, J. Reeve, and M. Bong (Bingley: Emerald Group Publishing Limited), 229–256. doi: 10.1108/S0749-742320160000019014
Siebers, M., Biedermann, S. V., Bindila, L., Lutz, B., and Fuss, J. (2021). Exercise-induced euphoria and anxiolysis do not depend on endogenous opioids in humans. Psychoneuroendocrinology 126:105173. doi: 10.1016/j.psyneuen.2021.105173
Standage, M., Sebire, S. J., and Loney, T. (2008). Does exercise motivation predict engagement in objectively assessed bouts of moderate-intensity exercise?: A self-determination theory perspective. J. Sport Exerc. Psychol. 30, 337–352. doi: 10.1123/jsep.30.4.337
Svensson, J. E., Schain, M., Plavén-Sigray, P., Cervenka, S., Tiger, M., Nord, M., et al. (2019). Validity and reliability of extrastriatal [11C]raclopride binding quantification in the living human brain. Neuroimage 202:116143. doi: 10.1016/j.neuroimage.2019.116143
Teixeira, P. J., Carraça, E. V., Markland, D., Silva, M. N., and Ryan, R. M. (2012). Exercise, physical activity, and self-determination theory: A systematic review. Int. J. Behav. Nutr. Phys. Act. 9:78. doi: 10.1186/1479-5868-9-78
Tziortzi, A. C., Haber, S. N., Searle, G. E., Tsoumpas, C., Long, C. J., Shotbolt, P., et al. (2014). Connectivity-based functional analysis of dopamine release in the striatum using diffusion-weighted MRI and positron emission tomography. Cereb. Cortex 24, 1165–1177. doi: 10.1093/cercor/bhs397
Wallstén, E., Axelsson, J., Sundström, T., Riklund, K., and Larsson, A. (2013). Subcentimeter tumor lesion delineation for high-resolution 18F-FDG pet images: Optimizing correction for partial-volume effects. J. Nucl. Med. Technol. 41, 85–91. doi: 10.2967/jnmt.112.117234
Weman-Josefsson, K., Lindwall, M., and Ivarsson, A. (2015). Need satisfaction, motivational regulations and exercise: Moderation and mediation effects. Int. J. Behav. Nutr. Phys. Act. 12:67. doi: 10.1186/s12966-015-0226-0
Wickham, H., Averick, M., Bryan, J., Chang, W., McGowan, L., François, R., et al. (2019). Welcome to the Tidyverse. J. Open Source Softw. 4:1686. doi: 10.21105/joss.01686
Wilson, P. M., Sabiston, C. M., Mack, D. E., and Blanchard, C. M. (2012). On the nature and function of scoring protocols used in exercise motivation research: An empirical study of the behavioral regulation in exercise questionnaire. Psychol. Sport Exerc. 13, 614–622. doi: 10.1016/j.psychsport.2012.03.009
Keywords: autonomous motivation, exercise motivation, self-determination theory (SDT), dopamine, PET, aging
Citation: Simonsson E, Stiernman LJ, Lundquist A, Rosendahl E, Hedlund M, Lindelöf N and Boraxbekk C-J (2022) Dopamine D2/3-receptor availability and its association with autonomous motivation to exercise in older adults: An exploratory [11C]-raclopride study. Front. Hum. Neurosci. 16:997131. doi: 10.3389/fnhum.2022.997131
Received: 18 July 2022; Accepted: 17 October 2022;
Published: 11 November 2022.
Edited by:
Björn H. Schott, Leibniz Institute for Neurobiology (LG), GermanyReviewed by:
Robert Henry Mach, University of Pennsylvania, United StatesDavid James Brooks, Newcastle University, United Kingdom
Copyright © 2022 Simonsson, Stiernman, Lundquist, Rosendahl, Hedlund, Lindelöf and Boraxbekk. This is an open-access article distributed under the terms of the Creative Commons Attribution License (CC BY). The use, distribution or reproduction in other forums is permitted, provided the original author(s) and the copyright owner(s) are credited and that the original publication in this journal is cited, in accordance with accepted academic practice. No use, distribution or reproduction is permitted which does not comply with these terms.
*Correspondence: Emma Simonsson, ZW1tYS5zaW1vbnNzb25AdW11LnNl