- Department of Orthopedic, West China Hospital of Sichuan University, Chengdu, China
Decision-making is an advanced cognitive function that promotes information processes in complex motor situations. In recent years, many neuroimaging studies have assessed the effects of long-term motor training on athletes’ brain activity while performing decision-making tasks, but the findings have been inconsistent and a large amount of data has not been quantitatively summarized until now. Therefore, this study aimed to identify the neural mechanism of long-term motor training affecting the decision-making function of athletes by using activation likelihood estimation (ALE) meta-analysis. Altogether, 10 studies were included and comprised a total of 350 people (168 motor experts and 182 novices, 411 activation foci). The ALE meta-analysis showed that more brain regions were activated for novices including the bilateral occipital lobe, left posterior cerebellar lobe, and left middle temporal gyrus (MTG) in decision-making tasks compared to motor experts. Our results possibly suggested the association between long-term motor training and neural efficiency in athletes, which provided a reference for further understanding the neural mechanisms of motor decision-making.
Introduction
Decision-making refers to the advanced cognitive function to process information in complex situations (Johnson, 2006). The motor has the characteristics of behavior initiation, memory, and decision-making that make it easy to observe or measure the results of decisions, thus motor psychologists consider that the most suitable for decision-making research is the field of sports (Raab et al., 2019). Numerous studies have shown that long-term motor training can substantially change the neurological activation of the cerebral cortex (Kelly and Garavan, 2005; Leff et al., 2011; Morgan et al., 2015; Fernandes et al., 2017). Presently, motor-induced decreases in activity might occur in brain areas related to visual processing, such as the occipital pole (Duru and Balcioglu, 2018) and occipital fusiform gyrus (Herold et al., 2020). Motor psychologists used the “neural efficiency” hypothesis to explain the result (Babiloni et al., 2010; Li and Smith, 2021). Neural efficiency refers to the phenomenon in which task execution becomes an automatic and neural activity in specific brain regions decreases as skill levels increase (Neubauer and Fink, 2009; Karim et al., 2017). Meanwhile, long-term motor training promoted the formation of internal models in the brain that enabled athletes to perform tasks in a relatively stable and efficient manner (Imamizu et al., 2000). For example, a study on archers when performing aiming tasks found that experts invoked smaller and more focused neural networks, whereas novices activated a wide range of brain regions including superior frontal gyrus, inferior frontal gyrus, prefrontal lobe, primary motor cortex, superior parietal lobe and primary somatosensory cortex (Kim et al., 2014). In contrast, some authors have found that the ventromedial prefrontal cortex (Hiser and Koenigs, 2018), orbitofrontal cortex (Rudebeck and Rich, 2018), infralimbic cortex (Roughley and Killcross, 2021), and amygdala (Chang et al., 2015) were activated in experts during decision-making. To summarize, changes in cortical activation caused by extensive motor training might be to satisfy better decision-making.
An “expert-novice” paradigm has been developed in the field of motor cognition (Del Villar et al., 2007). An important approach to study the effect of motor training on cortical activation during executive decision-making was to recruit professionals with intensive motor experience (motor experts) as an experimental group and compare their brain activation with that of a control group (novices).
Currently, image fixation and eye-movement recording methods are mostly used to compare the decision-making function of experts and novices in different motor scenarios, yet these methods are likely to make the obtained data content inaccurate due to the subjectivity of the subjects (Brunyé and Gardony, 2017). The rapid development of neuroimaging techniques such as functional magnetic resonance imaging (fMRI) and positron emission tomography (PET) has informed further understanding of the neural mechanisms underlying the differences in decision-making behavior between experts and novices (Kable and Levy, 2015; Bertollo et al., 2020). However, these studies have reported inconsistent results, probably due to small samples or inconsistent analysis methods.
Several studies have found that in decision-making tasks, experts showed decreased activation in specific brain regions compared to novices (Del Percio et al., 2008; Babiloni et al., 2010; Blain et al., 2019). An fMRI study comparing the intensity of brain activation during the ball response task in table tennis players and non-athletes found that the bilateral middle frontal gyrus, right middle orbitofrontal area, left inferior temporal gyrus, left middle temporal gyrus, right angular gyrus, and bilateral lingual gyrus was significantly less activated in the players (Guo Z. et al., 2017) and similar results were found by another study (Kellar et al., 2018). These authors suggested that task-related brain networks were organized more centrally and efficiently as athletes improved their skills, so a possible reflected more efficient utilization of specific neural circuits or automation of task execution. A study investigated the brain activation of objects tracking decisions in basketball players and novices. The results showed that less cortical activation of the bilateral middle frontal gyrus, right middle orbitofrontal area, right paracentral lobe, right precuneus, left supramarginal gyrus, right angular gyrus, left inferior temporal gyrus, left middle temporal gyrus and bilateral lingual gyrus was observed in basketball players than in novices (Qiu et al., 2019). This might indicate that long-term motor training promoted higher decision-making efficiency and athletes did not need to use more information systems to make decisions from complex situations, which led to less activation of neural network areas associated with decision-making (Wang et al., 2017; Ludyga et al., 2020; Blazhenets et al., 2021).
However, two fMRI studies (Abernethy and Russell, 1987; Wong and Gauthier, 2010) showed that expert players were able to pick up more relevant information than novices in a selective decision-making task, electrophysiological data indicated more prefrontal positive activities in experts (Javier et al., 2014). Several recent studies have found that experts compared with novices could quickly perceive the actions of opponents and successfully respond, and the frontal areas were more activated, which might be related to the expert’s better ability in action planning and action understanding (Callan and Naito, 2014; Okazaki et al., 2015; Vernon et al., 2018). A randomized controlled trial recruited 15 basketball expert athletes and 15 novices to participate in an action decision-making task to analyze the correlation between gaze behavior and decision-making. The results showed that expert athletes had stable gaze fixation, accurate rate, and activation in the inferior parietal lobe and inferior frontal gyrus compared to novices (Wu et al., 2013). This suggested that experts might need more activation in the brain’s attention and sensorimotor networks to achieve better decision-making performance.
Taken together, the above evidence has suggested that long-term motor training could alter brain activation in decision-related areas, but the findings were inconsistent. On the other hand, in existing many imaging studies, there was a small sample size of subjects, and the selection of motor items, duration, and intensity of each motor also vary, which might lead to low statistical power and effect sizes and even inconsistent findings (Yarkoni, 2009). Therefore, to overcome the limitations of single studies and further elucidate the neural mechanisms underlying the effects of motor training on decision-making functions, an activation likelihood estimation (ALE) algorithm based on large amounts of data need to be introduced into the field of motor cognition (Yarkoni et al., 2011). The quantitative approach for ALE has been increasingly improved, obtaining rich information within the whole brain. Therefore, the current study applied the ALE method to compare brain activation differences between experts and novices during the execution of decision-related tasks. Our results provide a reference for further understanding the neural mechanisms of motor decision-making and promote the development of motor cognitive neuroscience.
According to the view of motor psychologists, athletes acquired motor skills through training rapid stimulus discrimination, decision-making, and specialized attention, but novices did not have. So they concluded that motor experts were better able to perform specific tasks with fewer neural resources, suggesting that long-term motor training could improve the neural efficiency of experts, reflecting the automation process of motor skills (Seiler, 2010; Denadai et al., 2017). Based on this view we predicted that experts might indicate an activity decrease in areas relevant to the motor decision process. The opposite hypothesis was that there was no significant difference in brain activation between experts and novices when performing decision-making tasks.
Materials and Methods
Literature Search
This ALE meta-analysis has been conducted following a strict protocol by using the Preferred Reporting Items for Systematic Reviews and Meta-Analyses (PRISMA) guidelines (Moher et al., 2009).
A literature search was conducted by PubMed,1 ISI Web of Science,2 Elsevier3 and Cochrane Library.4 All included articles were published in the English language until March 2021. The following search keywords were used: “athlete” OR “expert” OR “novice” OR “non-athlete,” “decision-making” OR “decision,” “functional magnetic resonance imaging” OR “fMRI” OR “positron emission computed tomography” OR “PET.” The retrieval formula was (“athlete” [Mesh Terms] OR expert OR novice OR non-athlete) AND (“decision-making” [Mesh Terms] OR decision) AND (“functional magnetic resonance imaging” [Mesh Terms] OR “fMRI” [Mesh Terms] OR “positron emission computed tomography” [Mesh Terms] OR PET).
The titles and abstracts were independently screened by two trained reviewers (YD and LXH). Two independent reviewers completed the entire article inclusion process, and only articles that both reviewers reached an agreement on were finally included. When two independent reviewers disagreed on whether to include articles, resolved the differences through discussion, and seed the assistance of a third reviewer (DBL) if they still could not be resolved.
Inclusion and Exclusion Criteria
We selected studies considering the following inclusion criteria: (1) the samples included a group motor expert and a group novice; (2) motor experts with extensive experience were awarded the title of experts by their country, region, or school; (3) subjects performed motor decision-making task stimulus; (4) study with fMRI or PET; (5) the peak coordinates of brain activation areas were explicitly reported of motor experts and novices; (6) the reported results of activated foci were normalized to the Montreal Neurological Institute (MNI) (Collins et al., 1994) or the Talairach standardized stereotactic spaces (Paus et al., 1996); (7) whole-brain voxel analysis or at least one whole-brain analysis was performed; (8) subjects were healthy; (9) subjects were adults; (10) the language of studies was limited to English; (11) original research articles.
Exclusion criteria: (1) report coordinates were incomplete, or complete results have not been obtained after contacting the corresponding author; (2) research published in the form of conference reports, abstracts, etc.
These criteria identified 10 studies including 350 people (168 experts and 182 novices, 411 activation foci). Figure 1 shows the outcomes of the search process.
Data Extraction
The two reviewers (YD and LXH) extracted the following information from each included study: authors, age, year of publication, study name, type of coordinates, brain analysis method, number of participants, the ratio of male to female participants, comparison conditions, handedness, Foci.
Activation Likelihood Estimation Meta-Analysis
ALE is a quantitative meta-analysis method that uses a spatial variance model (3D Gaussian) to calculate the likelihood of each voxel being activated under a certain condition, thus obtaining the consistency of brain activation across multiple experiments (Laird et al., 2005; Eickhoff et al., 2009). Data processing was performed using GingerALE software (version 3.0.2)5 (Eickhoff et al., 2012). The difference in coordinate space (MNI vs. Talairach space) could be explained by converting the coordinates in Talairach to MNI space using icbm2tal in GingerALE (Lancaster et al., 2007). Finally, all activation coordinates were displayed in MNI space. We adopted a threshold for the map of the final ALE score graph with a familywise error (FWE) at p < 0.05 and a minimum cluster size of k > 10 mm3. For visualization, the ALE whole-brain maps were imported into Mango software (version 4.0.1)6 overly on a standardized anatomical MNI template (Colin27_T1_seg_MNI) (Dehghan et al., 2016).
Results
Study Selection and Characteristics
A total of 1,323 records were initially retrieved, and only 1,191 studies remained after deleting duplicates (n = 132). We screened potentially relevant articles by applying the inclusion and exclusion criteria (see Supplementary Material 1). Finally, 10 articles focusing on the brain activation of experts and novices while performing decision-making tasks were included in the present study. All included articles tested decisions made by experts and novices under the same stimuli. Among those papers, six (Wright et al., 2011; Wu et al., 2013; Balser et al., 2014; Wimshurst et al., 2016; Qiu et al., 2019; Blazhenets et al., 2021) determined the accuracy of the ball’s flight direction, three (Bishop et al., 2013; Wright et al., 2013; Xu et al., 2016) tested to determine the accuracy of opponent behavior, one (Guo-Zheng, 2016) tested response accuracy rate of ball block. Regarding the types of sports including basketball, tennis, hockey, volleyball, handball, badminton, soccer, etc. Table 1 described the detailed information of the included study.
Single Dataset Activation Likelihood Estimation Analysis Results
Experts had 93 foci in 5 different experiments, the 3 regions activated included the right inferior temporal gyrus (ITG) (BA 37), right sub-gyral (BA 37), right middle occipital gyrus (MOG) (BA 19).
Whereas novices had 124 foci in 7 different experiments. After completing the single data ALE analysis, novices activated the left precuneus (BA 7), left superior parietal lobule (SPL) (BA 7), left inferior parietal lobule (IPL) (BA 40), left middle frontal gyrus (MFG) (BA 6), left precentral gyrus (BA 4, BA 6), left cingulate gyrus (BA 24), left postcentral gyrus (BA 2), left supramarginal gyrus (BA 40), right middle temporal gyrus (MTG) (BA 37, BA 39), right superior temporal gyrus (STG) (BA22). Table 2 and Figure 2 showed the results of the single-dataset ALE analysis.
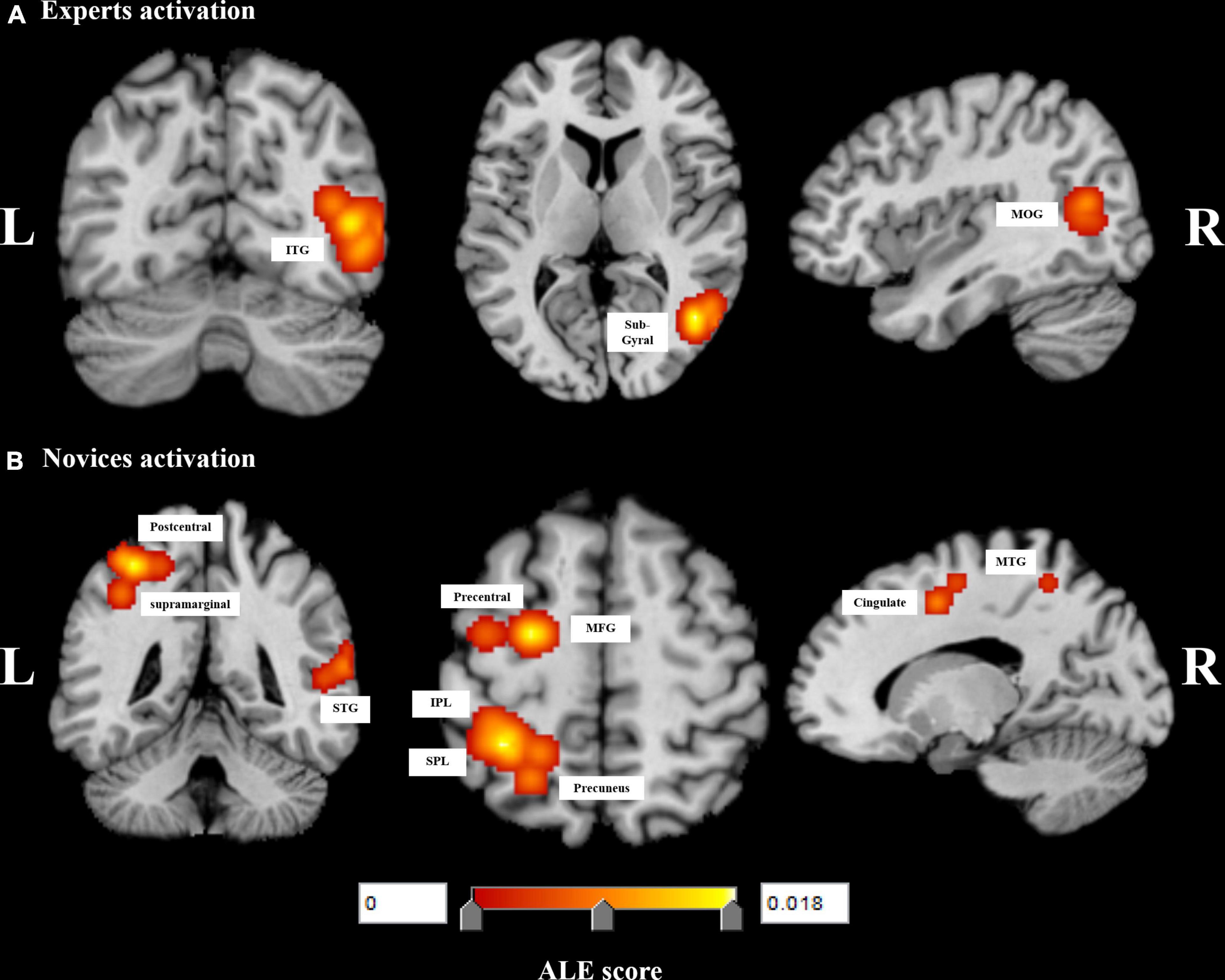
Figure 2. Significant meta-analysis results for (A) experts and (B) novices performing decision-making tasks. L, left, R, right, inferior temporal gyrus, ITG, middle occipital gyrus, MOG, superior parietal lobule, SPL, inferior parietal lobule, IPL, middle frontal gyrus, MFG, middle temporal gyrus, MTG.
Dual Dataset Comparison Activation Likelihood Estimation Analysis
Experts did not have significant activation compared to novices. In contrast, novices were activated in the left middle temporal gyrus (MTG) (BA 19, BA 39), bilateral MOG (BA 18, BA 19), left posterior lobe, right lingual gyrus (BA 18), left precuneus (BA 31), right fusiform gyrus (BA 19), bilateral inferior occipital gyrus (IOG) (BA 17). Table 2 and Figure 3 showed the results of the ALE analysis for experts and novices comparisons.
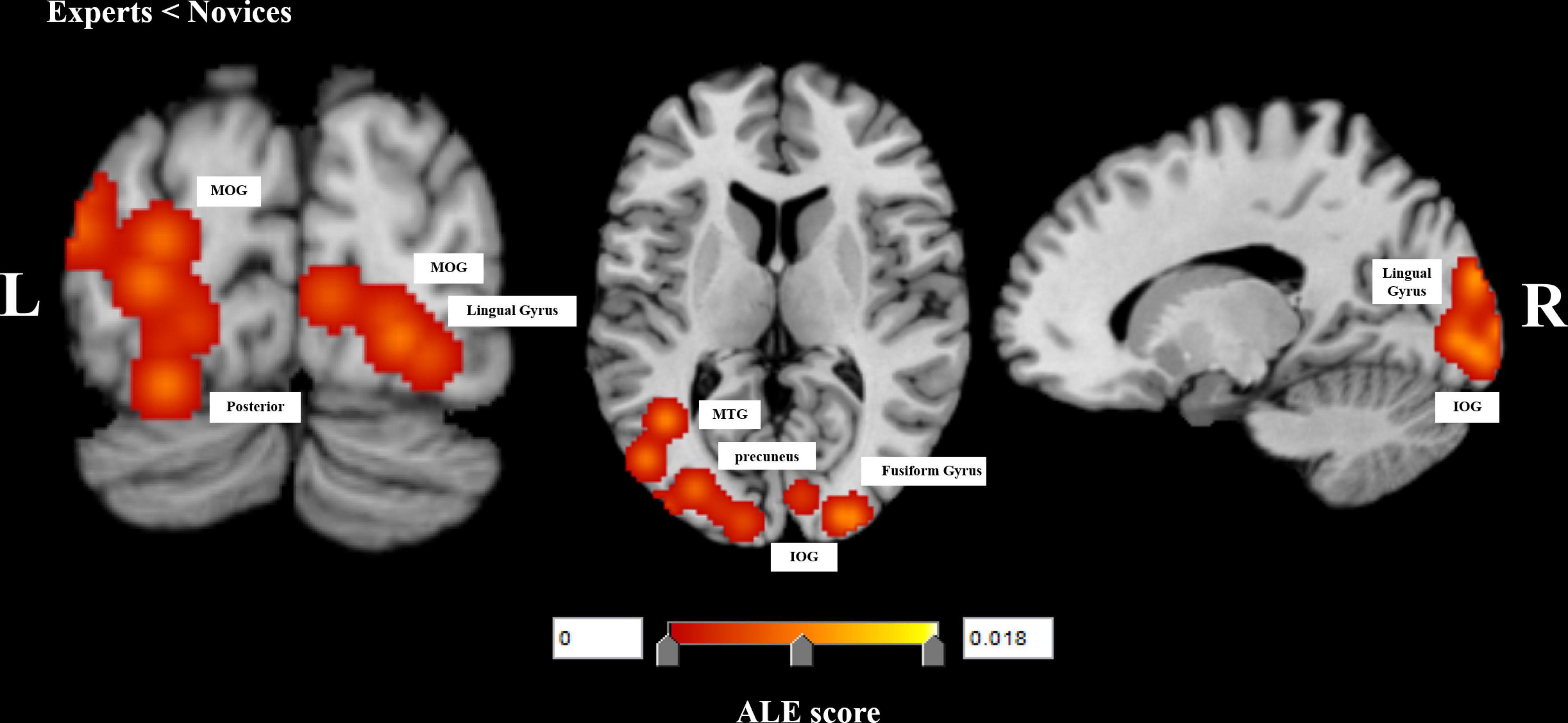
Figure 3. Significant meta-analysis results for comparison between experts and novices performing decision-making tasks. L, left, R, right, middle temporal gyrus, MTG, middle occipital gyrus, MOG, inferior occipital gyrus, IOG.
Discussion
The Effect of Handedness on the Results of Activation Likelihood Estimation Analysis
Handedness might affect an individual’s skills and athletic performance (Raymond et al., 1996). Some studies also have found that handedness was associated with cognitive function (Buckingham et al., 2011; Somers et al., 2015). The results of an EEG study found that left-handed athletes had greater P300 wave amplitude than right-handed athletes, suggesting that left-handed athletes had an advantage in cognitive perception and observation, but slower processing than the right-handed athletes (Nakamoto and Mori, 2008). Almost all of the subjects in the existing fMRI literature of motor decision-making were right-handed since there were fewer left-handed athletes. Among the 10 articles included in this study, only one article did not report whether the subjects were right-handed or not (Wimshurst et al., 2016), and the remaining nine articles reported that the subjects were right-handed. Therefore, we considered the articles included in this study to be general.
Summary of Activation Likelihood Estimation Meta-Analysis Results
Through systematic review and ALE meta-analysis of the included papers investigating brain activation in decision-making tasks by experts and novices, we found that experts activated the right ITG, right sub-gyral, and right MOG, but novices activated more brain regions including the left middle temporal gyrus (MTG) (BA 19, BA 39), bilateral MOG (BA 18, BA 19), left posterior lobe, right lingual gyrus (BA 18), left supramarginal gyrus (BA 40), right fusiform gyrus (BA 19), bilateral inferior occipital gyrus (IOG) (BA 17). Results of this ALE meta-analysis showed significant clusters in novices, and they were located in the bilateral occipital lobe (including the MTG, MOG, IOG, lingual gyrus, fusiform gyrus, and precuneus), left posterior cerebellar lobe, and left MTG compared to experts. This also confirmed that long-term motor training could improve the neural efficiency of motor experts, reflecting the automated process of motor skills.
Brain Activation Contrasts Between Motor Experts and Novices in Decision-Making Tasks
There was substantial evidence that the occipital lobe played an important role in the processing of visuospatial information (Todorov and Sousa, 2017; Buening and Brown, 2018). Motor cognitive psychologists argued that decision-making was based on information, of which visual information was particularly important (Darren et al., 2016). The results of the present study showed that the occipital lobe had in novices compared to experts, suggesting that the brain required more visual information processing when performing decision-making tasks. The results also corroborated previous research (Jie, 2014; Filgueiras et al., 2017; Guo Z. et al., 2017; Blazhenets et al., 2021). When the brain was stimulated by complex motor scenarios, motor experts first transferred the stimulus information into the brain’s perceptual system. The brain recognized the information and matched it with tactical information extracted from long-term memory and then made a final decision based on the learned motor skills (Smits et al., 2014). In contrast, novices performed the same task needing to find usable information and invalid information, which led to more visual information systems being activated to process and analyze information. Therefore, we believed that the primary mechanism for decreased activation in the expert’s occipital lobe was that experts had higher neural efficiency, which implied task-specific brain function plus sparing (Neubauer and Fink, 2009; Ludyga et al., 2016). This suggested that visual information processing played a key role in motor decision-making behavior.
Compared with experts, the results of ALE analysis showed that novices activated the left posterior cerebellar lobe during the decision-making process. The cerebellar was more involved in motor regulating and motor learning (Ashida et al., 2019; Schmahmann et al., 2019). Several studies have indicated that the activation of the cerebellum during performing decision-making tasks could enhance decision-making planning, initiation, and control (Yarrow et al., 2009; Nowrangi et al., 2014; Kim et al., 2015). Previous studies have found that long-term professional motor training could alter cerebellar activation. For example, a study comparing brain activation in elite archers and non-archers during archery found that non-archers had more cerebellar activation than elite archers (Chang et al., 2011), which was consistent with the findings of Guo Z. et al. (2017). Authors indicated that experts through years of sports skill learning and training might develop precise and professional sports skills, which included the ability to rapidly regulate motor information and motor learning, whereas novices did not have the ability. Imamizu et al. (2000) suggested that motor could be accurately controlled by using internal models of the body and that after the cerebellum acquired internal models through long-term motor training, athletes were able to perform tasks in a relatively automated, energy-conserving processing mode. Based on this view we believed that the reduced activation of the expert cerebellum was due to the formation of internal models of the cerebellum.
The MTG (BA 39) was already known to be significantly involved in cognitive functions such as motor planning and information processing (Guo L. et al., 2017; Xu et al., 2019). The results of this study found that the left MTG was activated when novices made decision-making in sports, which reflected the fact that experts did not need to activate more cognitive areas of the brain. Motor psychologists have suggested that cognitive mechanisms might be the main reason for the different decision-making levels between experts and novices (Dew et al., 2009). Experts with extensive motor experience could facilitate sports memory and attention, especially the ability to increase the depth of attention and reduce the waning of attentional information in complex motor scenarios. However, when novices made motor decisions in face of unfamiliar motor conditions, activation of the left MTG might be due to further processing of motor behavior information which reconfirmed the neural areas involved in motor information processing (Woods et al., 2014). Therefore, we argued that long-term motor training in athletes led to the more efficient cognitive neural network to adapt to the demands of high intensity and high correctness, which induced the brain to automatically process information. This was consistent with previous research findings (Babiloni et al., 2010; Zhang et al., 2019; Dobersek and Husselman, 2021).
Limitations and Future Research Directions
Potential limitations in this study should be mentioned. ALE analysis inevitably ignored the variation in each study and belonged to the statistical inference of fixed effects. Unlike a meta-analysis with complete activation maps, the data used in ALE were based on the reported data peak activation coordinates (Zhang et al., 2016). Therefore, it was unable to take into account studies without any significant categorical reporting, which might lead to systematic overestimation of biased results. Another limitation of this article was the gap between the designed motor situation and the real situation in included studies, which might make the findings not necessarily a true reflection of the actual movement, but rather an exploration of brain activation for decision-making tasks in a laboratory setting. Further development of realistic experimental designs or fMRI techniques could facilitate the direct exploration of decision-making in real motor situations, which was a direction of future research. Last, it was difficult to assess whether these activation regions were related to sports types due to the lack of comparisons between different motor experts, so further imaging studies were needed to compare brain activation alterations of different professional motor experts.
Conclusion
This study took a cognitive neuroscience perspective to reveal differences in the neural mechanisms underlying the motor decision-making processes of experts and novices. Our study provided new and meaningful evidence that greater activation for novices compared to experts in the bilateral occipital lobe, left posterior cerebellar lobe, and left MTG, but a decreased activation was not detected.
Data Availability Statement
The original contributions presented in the study are included in the article/Supplementary Material, further inquiries can be directed to the corresponding author/s.
Author Contributions
DL was responsible for the conception and design of the study, explained the data results, and critically revised the manuscript. YD and LH performed the data acquisition and statistical analysis. YW completed the document screening and literature search. YD extracted the data and edited the manuscript. All authors participated in the study.
Funding
The Science and Technology Department of Sichuan Provincial (Project No. 2020YFS0160), the West China Nursing Development Project of Sichuan University (Project No. HXHL19042), and the Sichuan Provincial Administration of Traditional Chinese Medicine (Project No. 2021MS122) funded the project. This work was supported by the West China Hospital of Sichuan University.
Conflict of Interest
The authors declare that the research was conducted in the absence of any commercial or financial relationships that could be construed as a potential conflict of interest.
Publisher’s Note
All claims expressed in this article are solely those of the authors and do not necessarily represent those of their affiliated organizations, or those of the publisher, the editors and the reviewers. Any product that may be evaluated in this article, or claim that may be made by its manufacturer, is not guaranteed or endorsed by the publisher.
Acknowledgments
We thank Yujun Lee of the Foreign Languages Department at North Sichuan Medical College for his assistance with conducting the literature searches. We thank Pan Yue of Chengdu University of Traditional Chinese Medicine for his assistance with image processing.
Supplementary Material
The Supplementary Material for this article can be found online at: https://www.frontiersin.org/articles/10.3389/fnhum.2022.854692/full#supplementary-material
Footnotes
- ^ https://pubmed.ncbi.nlm.nih.gov/
- ^ http://isiknowledge.com/wos
- ^ https://www.elsevier.com/zh-cn
- ^ https://www.cochranelibrary.com/
- ^ http://www.brainmap.org
- ^ http://rii.uthscsa.edu/mango/
References
Abernethy, B., and Russell, D. G. (1987). Expert-Novice Differences in an Applied Selective Attention Task. J. Sport Psychol. 9, 326–345. doi: 10.1123/jsp.9.4.326
Ashida, R., Cerminara, N. L., Edwards, R. J., Apps, R., and Brooks, J. C. W. (2019). Sensorimotor, language, and working memory representation within the human cerebellum. Hum. Brain Mapp. 40, 4732–4747. doi: 10.1002/hbm.24733
Babiloni, C., Marzano, N., Infarinato, F., Iacoboni, M., Rizza, G., Aschieri, P., et al. (2010). “Neural efficiency” of experts’ brain during judgment of actions: a high-resolution EEG study in elite and amateur karate athletes. Behav. Brain Res. 207, 466–475. doi: 10.1016/j.bbr.2009.10.034
Balser, N., Lorey, B., Pilgramm, S., Stark, R., Bischoff, M., Zentgraf, K., et al. (2014). Prediction of human actions: expertise and task-related effects on neural activation of the action observation network. Hum. Brain Mapp. 35, 4016–4034. doi: 10.1002/hbm.22455
Bertollo, M., Doppelmayr, M., and Robazza, C. (2020). “Using Brain Technologies in Practice”. in Handbook of Sport Psychology, eds G Tenenbaum and RC Eklund (Hoboken, NJ: Wiley)
Bishop, D. T., Wright, M. J., Jackson, R. C., and Abernethy, B. (2013). Neural bases for anticipation skill in soccer: an FMRI study. J. Sport Exerc. Psychol. 35, 98–109. doi: 10.1123/jsep.35.1.98
Blain, B., Schmit, C., Aubry, A., Hausswirth, C., Le Meur, Y., and Pessiglione, M. (2019). Neuro-computational Impact of Physical Training Overload on Economic Decision-Making. Curr. Biol. 29, 3289–3297.e4. doi: 10.1016/j.cub.2019.08.054
Blazhenets, G., Kurz, A., Frings, L., Leukel, C., and Meyer, P. T. (2021). Brain activation patterns during visuomotor adaptation in motor experts and novices: An FDG PET study with unrestricted movements. J. Neurosci. Methods 350:109061. doi: 10.1016/j.jneumeth.2020.109061
Brunyé, T. T., and Gardony, A. L. (2017). Eye tracking measures of uncertainty during perceptual decision making. Int. J. Psychophysiol. 120, 60–68. doi: 10.1016/j.ijpsycho.2017.07.008
Buckingham, G., Main, J. C., and Carey, D. P. (2011). Asymmetries in motor attention during a cued bimanual reaching task: Left and right handers compared. Cortex 47, 432–440. doi: 10.1016/j.cortex.2009.11.003
Buening, J., and Brown, R. D. (2018). “Visuospatial Cognition,” in Neuroscience of Mathematical Cognitive Development: From Infancy Through Emerging Adulthood, ed. V. P. Clark (New York, NY: Springer International Publishing).
Callan, D. E., and Naito, E. (2014). Neural processes distinguishing elite from expert and novice athletes. Cogn. Behav. Neurol. 27, 183–188. doi: 10.1097/wnn.0000000000000043
Chang, S. W., Fagan, N. A., Toda, K., Utevsky, A. V., Pearson, J. M., and Platt, M. L. (2015). Neural mechanisms of social decision-making in the primate amygdala. Proc. Natl. Acad. Sci. 112, 16012–16017. doi: 10.1073/pnas.1514761112
Chang, Y., Lee, J. J., Seo, J. H., Song, H. J., Kim, Y. T., Lee, H. J., et al. (2011). Neural correlates of motor imagery for elite archers. NMR Biomed. 24, 366–372. doi: 10.1002/nbm.1600
Collins, D. L., Neelin, P., Peters, T. M., and Evans, A. C. (1994). Automatic 3D Intersubject Registration of MR Volumetric Data in Standardized Talairach Space. J. Comp. Assist. Tomograph. 18, 192–205. doi: 10.1097/00004728-199403000-00005
Darren, J. P., Gabbett, T. J., and Nassis, G. P (2016). Agility in Team Sports: Testing, Training and Factors Affecting Performance. Sports Med. 46, 421–442. doi: 10.1007/s40279-015-0428-2
Dehghan, M., Schmidt-Wilcke, T., Pfleiderer, B., Eickhoff, S. B., Petzke, F., Harris, R. E., et al. (2016). Coordinate-based (ALE) meta-analysis of brain activation in patients with fibromyalgia. Hum. Brain Mapp. 37, 1749–1758. doi: 10.1002/hbm.23132
Del Percio, C., Rossini, P. M., Marzano, N., Iacoboni, M., Infarinato, F., Aschieri, P., et al. (2008). Is there a “neural efficiency” in athletes? A high-resolution EEG study. Neuroimage 42, 1544–1553. doi: 10.1016/j.neuroimage.2008.05.061
Del Villar, F., García González, L., Iglesias, D., Perla Moreno, M., and Cervelló, E. M. (2007). Expert-novice differences in cognitive and execution skills during tennis competition. Percept Mot. Skills 104, 355–365. doi: 10.2466/pms.104.2.355-365
Denadai, B. S., de Aguiar, R. A., de Lima, L. C., Greco, C. C., and Caputo, F. (2017). Explosive Training and Heavy Weight Training are Effective for Improving Running Economy in Endurance Athletes: A Systematic Review and Meta-Analysis. Sports Med. 47, 545–554. doi: 10.1007/s40279-016-0604-z
Dew, N., Read, S., Sarasvathy, S. D., and Wiltbank, R. (2009). Effectual versus predictive logics in entrepreneurial decision-making: Differences between experts and novices. J. Bus. Ventur. 24, 287–309. doi: 10.1016/j.jbusvent.2008.02.002
Dobersek, U., and Husselman, T.-A. (2021). The role of neural efficiency, transient hypofrontality and neural proficiency in optimal performance in self-paced sports: a meta-analytic review. Exp. Brain Res. 239, 1381–1393. doi: 10.1007/s00221-021-06078-9
Duru, A. D., and Balcioglu, T. H. (2018). Functional and Structural Plasticity of Brain in Elite Karate Athletes. J. Healthc. Eng. 2018:8310975. doi: 10.1155/2018/8310975
Eickhoff, S. B., Bzdok, D., Laird, A. R., Kurth, F., and Fox, P. T. (2012). Activation likelihood estimation meta-analysis revisited. Neuroimage 59, 2349–2361. doi: 10.1016/j.neuroimage.2011.09.017
Eickhoff, S. B., Laird, A. R., Grefkes, C., Wang, L. E., Zilles, K., and Fox, P. T. (2009). Coordinate-based activation likelihood estimation meta-analysis of neuroimaging data: a random-effects approach based on empirical estimates of spatial uncertainty. Hum. Brain Mapp. 30, 2907–2926. doi: 10.1002/hbm.20718
Fernandes, J., Arida, R. M., and Gomez-Pinilla, F. (2017). Physical exercise as an epigenetic modulator of brain plasticity and cognition. Neurosci. Biobehav. Rev. 80, 443–456. doi: 10.1016/j.neubiorev.2017.06.012
Filgueiras, A., Conde, E. Q., and Hall, C. R. (2017). The neural basis of kinesthetic and visual imagery in sports: an ALE metaanalysis. Brain Imag. Behav. 12, 1513–1523. doi: 10.1007/s11682-017-9813-9
Guo, L., Bai, G., Zhang, H., Lu, D., Zheng, J., and Xu, G. (2017). Cognitive Functioning in Temporal Lobe Epilepsy: A BOLD-fMRI Study. Mol. Neurobiol. 54, 8361–8369. doi: 10.1007/s12035-016-0298-0
Guo, Z., Li, A., and Yu, L. (2017). “Neural Efficiency” of Athletes’ Brain during Visuo-Spatial Task: An fMRI Study on Table Tennis Players. Front. Behav. Neurosci. 11:72. doi: 10.3389/fnbeh.2017.00072
Herold, F., Aye, N., Lehmann, N., Taubert, M., and Müller, N. G. (2020). The contribution of functional magnetic resonance imaging to the understanding of the effects of acute physical exercise on cognition. Brain Sci. 10:175. doi: 10.3390/brainsci10030175
Hiser, J., and Koenigs, M. (2018). The multifaceted role of the ventromedial prefrontal cortex in emotion, decision making, social cognition, and psychopathology. Biol. Psychiatry 83, 638–647. doi: 10.1016/j.biopsych.2017.10.030
Imamizu, H., Miyauchi, S., Tamada, T., Sasaki, Y., Takino, R., PuÈtz, B., et al. (2000). Human cerebellar activity reflecting an acquired internal model of a new tool. Nature 403, 192–195. doi: 10.1038/35003194
Javier, S. L., Thalia, F., Juan, S. P., Martinez, M., Di, R. F., and Bart, R. (2014). Differences in Visuo-Motor Control in Skilled vs. Novice Martial Arts Athletes during Sustained and Transient Attention Tasks: A Motor-Related Cortical Potential Study. PLoS One 9:e91112. doi: 10.1371/journal.pone.0091112
Jie, Y. (2014). The influence of motor expertise on the brain activity of motor task performance: A meta-analysis of functional magnetic resonance imaging studies. Cogn. Affect. Behav. Neurosci. 15, 381–394. doi: 10.3758/s13415-014-0329-0
Johnson, J. G. (2006). Cognitive modeling of decision making in sports. Psychol. Sport Exerc. 7, 631–652. doi: 10.1016/j.psychsport.2006.03.009
Kable, J. W., and Levy, I. (2015). Neural markers of individual differences in decision-making. Curr. Opin. Behav. Sci. 5, 100–107. doi: 10.1016/j.cobeha.2015.08.004
Karim, H. T., Huppert, T. J., Erickson, K. I., Wollam, M. E., Sparto, P. J., Sejdić, E., et al. (2017). Motor sequence learning-induced neural efficiency in functional brain connectivity. Behav. Brain Res. 319, 87–95. doi: 10.1016/j.bbr.2016.11.021
Kellar, D., Newman, S., Pestilli, F., Cheng, H., and Port, N. L. (2018). Comparing fMRI activation during smooth pursuit eye movements among contact sport athletes, non-contact sport athletes, and non-athletes. Neuroimage Clin. 18, 413–424. doi: 10.1016/j.nicl.2018.01.025
Kelly, A. M., and Garavan, H. (2005). Human functional neuroimaging of brain changes associated with practice. Cereb. Cortex 15, 1089–1102. doi: 10.1093/cercor/bhi005
Kim, J. H., Han, J. K., Kim, B. N., and Han, D. H. (2015). Brain networks governing the golf swing in professional golfers. J. Sports Sci. 33, 1980–1987. doi: 10.1080/02640414.2015.1022570
Kim, W., Chang, Y., Kim, J., Seo, J., Ryu, K., Lee, E., et al. (2014). An fMRI study of differences in brain activity among elite, expert, and novice archers at the moment of optimal aiming. Cogn. Behav. Neurol. 27, 173–182. doi: 10.1097/wnn.0000000000000042
Laird, A. R., Fox, P. M., Price, C. J., Glahn, D. C., Uecker, A. M., Lancaster, J. L., et al. (2005). ALE meta-analysis: controlling the false discovery rate and performing statistical contrasts. Hum. Brain Mapp. 25, 155–164. doi: 10.1002/hbm.20136
Lancaster, J. L., Tordesillas-Gutiérrez, D., Martinez, M., Salinas, F., Evans, A., Zilles, K., et al. (2007). Bias between MNI and Talairach coordinates analyzed using the ICBM-152 brain template. Hum. Brain Mapp. 28, 1194–1205. doi: 10.1002/hbm.20345
Leff, D. R., Orihuela-Espina, F., Elwell, C. E., Athanasiou, T., Delpy, D. T., Darzi, A. W., et al. (2011). Assessment of the cerebral cortex during motor task behaviours in adults: a systematic review of functional near infrared spectroscopy (fNIRS) studies. Neuroimage 54, 2922–2936. doi: 10.1016/j.neuroimage.2010.10.058
Li, L., and Smith, D. M. (2021). Neural Efficiency in Athletes: A Systematic Review. Front. Behav. Neurosci. 15:698555. doi: 10.3389/fnbeh.2021.698555
Ludyga, S., Gerber, M. Pühse, U., Looser, V. N., and Kamijo, K. (2020). Systematic review and meta-analysis investigating moderators of long-term effects of exercise on cognition in healthy individuals. Nat. Hum. Behav. 4, 603–612. doi: 10.1038/s41562-020-0851-8
Ludyga, S., Gronwald, T., and Hottenrott, K. (2016). The Athlete’s Brain: Cross-Sectional Evidence for Neural Efficiency during Cycling Exercise. Neural. Plast. 2016, 1–7. doi: 10.1155/2016/4583674
Guo-Zheng, M. (2016). An fMRI Study for Decision-Making Neural Efficiency of Volleyball Players. China Sport Science and Technology.
Moher, D., Liberati, A., Tetzlaff, J., and Altman, D. G. (2009). Preferred reporting items for systematic reviews and meta-analyses: the PRISMA statement. PLoS Med. 6:e1000097. doi: 10.1371/journal.pmed.1000097
Morgan, J. A., Corrigan, F., and Baune, B. T. (2015). Effects of physical exercise on central nervous system functions: a review of brain region specific adaptations. J. Mol. Psychiatry 3:3. doi: 10.1186/s40303-015-0010-8
Nakamoto, H., and Mori, S. (2008). Effects of stimulus–response compatibility in mediating expert performance in baseball players. Brain Res. 1189, 179–188. doi: 10.1016/j.brainres.2007.10.096
Neubauer, A. C., and Fink, A. (2009). Intelligence and neural efficiency. Neurosci. Biobehav. Rev. 33, 1004–1023. doi: 10.1016/j.neubiorev.2009.04.001
Nowrangi, M. A., Lyketsos, C., Rao, V., and Munro, C. A. (2014). Systematic Review of Neuroimaging Correlates of Executive Functioning: Converging Evidence From Different Clinical Populations. J. Neuropsychiatry Clin. Neurosci. 26, 114–125. doi: 10.1176/appi.neuropsych.12070176
Okazaki, V. H., Rodacki, A. L., and Satern, M. N. (2015). A review on the basketball jump shot. Sports Biomech. 14, 190–205. doi: 10.1080/14763141.2015.1052541
Paus, T., Otaky, N., Caramanos, Z., MacDonald, D., Zijdenbos, A., D’Avirro, D., et al. (1996). In vivo morphometry of the intrasulcal gray matter in the human cingulate, paracingulate, and superior-rostral sulci: hemispheric asymmetries, gender differences and probability maps. J. Comp. Neurol. 376, 664–673. doi: 10.1002/(sici)1096-9861(19961223)376:4<664::Aid-cne12<3.0.Co;2-m
Qiu, F., Pi, Y., Liu, K., Zhu, H., Li, X., Zhang, J., et al. (2019). Neural efficiency in basketball players is associated with bidirectional reductions in cortical activation and deactivation during multiple-object tracking task performance. Biol. Psychol. 144, 28–36. doi: 10.1016/j.biopsycho.2019.03.008
Raab, M., Bar-Eli, M., Plessner, H., and Araújo, D. (2019). The past, present and future of research on judgment and decision making in sport. Psychol. Sport Exer. 42, 25–32. doi: 10.1016/j.psychsport.2018.10.004
Raymond, M., Pontier, D., Dufour, A.-B., and Møller, A. P. (1996). Frequency-dependent maintenance of left handedness in humans. Proc. Royal Soc. London. Series B. 263, 1627–1633. doi: 10.1098/rspb.1996.0238
Roughley, S., and Killcross, S. (2021). The role of the infralimbic cortex in decision making processes. Curr. Opin. Behav. Sci. 41, 138–143. doi: 10.1016/j.cobeha.2021.06.003
Schmahmann, J. D., Guell, X., Stoodley, C. J., and Halko, M. A. (2019). The Theory and Neuroscience of Cerebellar Cognition. Annu. Rev. Neurosci. 42, 337–364. doi: 10.1146/annurev-neuro-070918-050258
Seiler, S. (2010). What is best practice for training intensity and duration distribution in endurance athletes? Int. J. Sports Physiol. Perform. 5, 276–291. doi: 10.1123/ijspp.5.3.276
Smits, B., Pepping, G. J., and Hettinga, F. J. (2014). Pacing and Decision Making in Sport and Exercise: The Roles of Perception and Action in the Regulation of Exercise Intensity. Sports Med. 44, 763–775. doi: 10.1007/s40279-014-0163-0
Somers, M., Shields, L. S., Boks, M. P., Kahn, R. S., and Sommer, I. E. (2015). Cognitive benefits of right-handedness: a meta-analysis. Neurosci. Biobehav. Rev. 51, 48–63. doi: 10.1016/j.neubiorev.2015.01.003
Todorov, O. S., and Sousa, A. (2017). “Evolution of the Occipital Lobe,” in Digital Endocasts, eds E. Bruner, N. Ogihara, and H. Tanabe (Tokyo: Springer), 259–273. doi: 10.1007/978-4-431-56582-6_17
Vernon, G., Farrow, D., and Reid, M. (2018). Returning Serve in Tennis: A Qualitative Examination of the Interaction of Anticipatory Information Sources Used by Professional Tennis Players. Front. Psychol. 9:895. doi: 10.3389/fpsyg.2018.00895
Wang, C. H., Yang, C. T., Moreau, D., and Muggleton, N. G. (2017). Motor expertise modulates neural oscillations and temporal dynamics of cognitive control. Neuroimage 158, 260–270. doi: 10.1016/j.neuroimage.2017.07.009
Wimshurst, Z. L., Sowden, P. T., and Wright, M. (2016). Expert-novice differences in brain function of field hockey players. Neuroscience 315, 31–44. doi: 10.1016/j.neuroscience.2015.11.064
Wong, Y. K., and Gauthier, I. (2010). Holistic processing of musical notation: Dissociating failures of selective attention in experts and novices. Cogn. Affect. Behav. Neurosci. 10, 541–551. doi: 10.3758/CABN.10.4.541
Woods, E. A., Hernandez, A. E., Wagner, V. E., and Beilock, S. L. (2014). Expert athletes activate somatosensory and motor planning regions of the brain when passively listening to familiar sports sounds. Brain Cogn. 87, 122–133. doi: 10.1016/j.bandc.2014.03.007
Wright, M. J., Bishop, D. T., Jackson, R. C., and Abernethy, B. (2011). Cortical fMRI activation to opponents’ body kinematics in sport-related anticipation: expert-novice differences with normal and point-light video. Neurosci. Lett. 500, 216–221. doi: 10.1016/j.neulet.2011.06.045
Wright, M. J., Bishop, D. T., Jackson, R. C., and Abernethy, B. (2013). Brain regions concerned with the identification of deceptive soccer moves by higher-skilled and lower-skilled players. Front. Hum. Neurosci. 7:851. doi: 10.3389/fnhum.2013.00851
Wu, Y., Zeng, Y., Zhang, L., Wang, S., Wang, D., Tan, X., et al. (2013). The role of visual perception in action anticipation in basketball athletes. Neuroscience 237, 29–41. doi: 10.1016/j.neuroscience.2013.01.048
Xu, H., Wang, P., Ye, Z., Di, X., Xu, G., Mo, L., et al. (2016). The Role of Medial Frontal Cortex in Action Anticipation in Professional Badminton Players. Front. Psychol. 7:1817. doi: 10.3389/fpsyg.2016.01817
Xu, J., Lyu, H., Li, T., Xu, Z., and Hu, Q. (2019). Delineating functional segregations of the human middle temporal gyrus with resting-state functional connectivity and coactivation patterns. Hum. Brain Mapp. 40, 5159–5171. doi: 10.1002/hbm.24763
Yarkoni, T. (2009). Big Correlations in Little Studies: Inflated fMRI Correlations Reflect Low Statistical Power-Commentary on Vul, et al. (2009). Perspect Psychol. Sci. 4, 294–298. doi: 10.1111/j.1745-6924.2009.01127.x
Yarkoni, T., Poldrack, R. A., Nichols, T. E., Van Essen, D. C., and Wager, T. D. (2011). Large-scale automated synthesis of human functional neuroimaging data. Nat. Methods 8, 665–670. doi: 10.1038/nmeth.1635
Yarrow, K., Brown, P., and Krakauer, J. W. (2009). Erratum: Inside the brain of an elite athlete: The neural processes that support high achievement in sports. Nat. Rev. Neurosci. 10:692. doi: 10.1038/nrn2672
Zhang, B., Lin, P., Shi, H., Öngür, D., Auerbach, R. P., Wang, X., et al. (2016). Mapping anhedonia-specific dysfunction in a transdiagnostic approach: an ALE meta-analysis. Brain Imag. Behav. 10, 920–939. doi: 10.1007/s11682-015-9457-6
Keywords: decision-making, motor training, neuroimaging, brainmap, activation likelihood estimation (ALE), fMRI
Citation: Du Y, He L, Wang Y and Liao D (2022) The Neural Mechanism of Long-Term Motor Training Affecting Athletes’ Decision-Making Function: An Activation Likelihood Estimation Meta-Analysis. Front. Hum. Neurosci. 16:854692. doi: 10.3389/fnhum.2022.854692
Received: 14 January 2022; Accepted: 03 March 2022;
Published: 13 April 2022.
Edited by:
Laura Piccardi, Sapienza University of Rome, ItalyReviewed by:
Andy Wai Kan Yeung, University of Hong Kong, ChinaTed Maldonado, Indiana State University, United States
Copyright © 2022 Du, He, Wang and Liao. This is an open-access article distributed under the terms of the Creative Commons Attribution License (CC BY). The use, distribution or reproduction in other forums is permitted, provided the original author(s) and the copyright owner(s) are credited and that the original publication in this journal is cited, in accordance with accepted academic practice. No use, distribution or reproduction is permitted which does not comply with these terms.
*Correspondence: Dengbin Liao, jiuyou2003@163.com