- Department of Ophthalmology, The First Affiliated Hospital of Nanchang University, Jiangxi Center of National Ocular Disease Clinical Research Center, Nanchang, China
Objective: Previous studies have demonstrated altered brain activity in strabismic amblyopia (SA). In this study, low-frequency repetitive transcranial magnetic stimulation (rTMS) was applied in children with strabismic amblyopia after they had undergone strabismus surgery. The effect of rTMS was investigated by measuring the changes of brain features using the amplitude of low-frequency fluctuation (ALFF).
Materials and Methods: In this study, 21 SA patients (12 males and 9 females) were recruited based on their age (7–13 years old), weight, and sex. They all had SA in their left eyes and they received rTMS treatment one month after strabismus surgery. Their vision before and after surgery were categorized as pre-rTMS (PRT) and post-rTMS (POT). All participants received rTMS treatment, underwent magnetic resonance imaging (MRI), and their data were analyzed using the repeated measures t-test. The team used correlation analysis to explore the relationship between logMAR visual acuity and ALFF.
Results: Pre- versus post-rTMS values of ALFF were significantly different within individuals. In the POT group, ALFF values were significantly decreased in the Angular_R (AR), Parietal_Inf_L (PIL), and Cingulum_Mid_R (CMR) while ALFF values were significantly increased in the Fusiform_R (FR) and Frontal_Inf_Orb_L(FIL) compared to the PRT stage.
Conclusion: Our data showed that ALFF recorded from some brain regions was changed significantly after rTMS in strabismic amblyopes. The results may infer the pathological basis of SA and demonstrate that visual function may be improved using rTMS in strabismic amblyopic patients.
Introduction
Strabismus and amblyopia are two common visual developmental disorders that can occur in infancy and persist into adulthood if treatment is not successful (Tarczy-Hornoch et al., 2013; Chen et al., 2014). Strabismus is an optical manifestation disorder associated with the coordination of the external eye muscles. Different conditions of extraocular muscle incongruity may result in different types of diplopic images (Figure 1). Strabismus is generally considered to be associated with maldevelopment of the visual pathways in the brain that mediate eye movement (Min et al., 2018), and inimically affects stereopsis, binocularity, and depth perception (Gunton et al., 2015). Hyperopia, muscle dysfunction, trauma, brain disease, and infection are all important causes of strabismus, and risk factors include premature birth and cerebral palsy. Diagnosis can be made by the observation of light reflected from the anterior eye offset from the center of the pupil. Treatments, including refractive correction and eye alignment surgery, address the impact on vision and are good choices for patients. The incidence rate of adult-onset strabismus is reportedly 54.2 per 100,000 individuals (Mohney et al., 2012), and in adults strabismus is often associated with undiagnosed amblyopia in early childhood and symptoms associated with aging (Chan et al., 2004).
Amblyopia, also known as lazy eye, is a visual disorder with causes which affect cooperation between the eyes and brain (National Eye Institute, 2016). Because the condition is associated with changes in the brain, visual impairment such as ametropia cannot be fully treated after surgery (Schwartz et al., 2002; Levi, 2013). Early detection can improve the rate of successful treatment (Jefferis et al., 2015) and glasses may be an important part of treatment for children (Jefferis et al., 2015; Maconachie and Gottlob, 2015). Many patients with amblyopia, especially mild cases, are unaware of their condition because one eye is affected and the vision of the fellow eye is normal. However, because two eyes are needed for stereo vision (which is usually lacking for the patients), patients with amblyopia may have relatively poor vision, and relatively low sensitivity to contrast and movement in the affected eye (Fazzi and Bianchi, 2016). Amblyopia features reduced stereopsis, visual acuity (VA), visual sensitivity, and impaired spatial vision and binocular summation (Webber and Wood, 2005).
Diplopia refers to the abnormal phenomenon of one object being perceived as two identical but separated images. There are many causes of diplopia, some of which reflect anomalies of the brain (Cerulli Irelli et al., 2021). Diplopia may occur when one or more of the six muscles that control eye movement become inflamed, injured, or neurologically impaired, and the muscles in both eyes do not accurately coordinate (Goseki et al., 2021). Because strabismus causing diplopia and confusion (perceived superposition of two different images) can make the patient feel unwell, the image from the macula of the strabismic eye may be suppressed for a sustained period, and the amblyopia that accompanies this situation is known as strabismic amblyopia (Peña Urbina et al., 2021).
Magnetic resonance imaging (MRI) has been widely used in various pretreatment imaging diagnoses recently. As a non-invasive neuroimaging method, it is mainly used to evaluate the functional and structural changes of the human brain (Brown et al., 2016). Functional Magnetic Resonance Imaging (fMRI) is a technology with precise spatial resolution and is used for the analysis of brain function (Goodyear and Menon, 2001). This imaging method helps to reveal the mechanism underpinning eye diseases (Conner et al., 2007; Wang et al., 2012; Liang et al., 2017). Repetitive transcranial magnetic stimulation (rTMS) mainly achieve excitation or inhibition of local cerebral cortical function by changing the stimulation frequency, with the aim of effective treatment of disease. Thompson’s present data show that rTMS of the visual cortex can temporarily improve contrast sensitivity in the adult amblyopic visual cortex (Thompson et al., 2008). Spiegel have shown that a-tDCS to the visual cortex would improve contrast sensitivity in adult patients with amblyopia by enhancing the cortical response to inputs from the amblyopic eye (Spiegel et al., 2013). Rehn found that rTMS could improve the symptoms of patients with obsessive-compulsive disorder (Rehn et al., 2018). Chou demonstrated the effect of rTMS on Alzheimer’s disease (Chou et al., 2020). TMS can be used to stimulate the cerebral cortex, such as the visual cortex and somatosensory cortex, to induce local excitatory or inhibitory effects and affect the function of the system. In addition, TMS can also be used in learning, memory, language, and emotional research (Brunoni et al., 2017; Sebastianelli et al., 2017; McClintock et al., 2018; Arns et al., 2019; Yang et al., 2019; Chou et al., 2020; Malkani and Zee, 2020).
Studies suggested the neural networks modulating aspects of emotional behavior to be implicated in the pathophysiology of mood disorders. These networks involve the prefrontal cortex (Rigucci et al., 2010). AhmedA Abdelrahman’s findings revealed that HF-rTMS over L-DLPCF for 10 days reduced cigarette consumption, craving, dependence, and improved associated symptoms of anxiety and depression (Abdelrahman et al., 2021). The abnormal activity of dorsolateral prefrontal cortex (DLPFC) is related to the occurrence of anxiety and depression.
Materials and Methods
Subjects
Twenty-one patients (12 males and 9 females) were recruited at the First Affiliated Hospital of Nanchang University. Those eligible for participation met the following criteria: (1) Aged between 7 and 13 years old; (2) Their guardian allowed them to receive rTMS treatment within 1 month after strabismus surgery; (3) Clear diagnosis of strabismus. Exclusion criteria were as follows: (1) Patients suffering from other eye diseases, such as cataract, glaucoma, or retinitis pigmentosa; (2) Mental illness. The 21 patients who met the criteria underwent rTMS treatment before and after surgical treatment. The Medical Ethics Committee of the First Affiliated Hospital of Nanchang University approved the research plan. Patients and guardians were informed about the research and potential risks before signing consent forms voluntarily.
This study sought consent from all patients participating in the diagnosis, treatment, and evaluation stages of the study.
Transcranial Magnetic Stimulation
Repetitive transcranial magnetic stimulation (rTMS) was administered by trained researchers using the Magstim Rapid device (Magstim®, Whitland, Wales, United Kingdom) and Magstim d70-mm-air-cooled figure-of-eight coil. Use of stimulus frequency rate of 10Hz, intensity of 100% resting motion threshold, 5s stimulation, pulse 50/5s, the interval was 10s, with 2000 pulses in total, and the treatment lasted for 10min. The stimulation site is DLPFC. Stimulation was performed between baseline measurements and remeasurements. The two rTMS stimulation groups received 30 treatments 5 times a week for 6 weeks.
Magnetic Resonance Imaging Parameters
All patients underwent scanning using 3-TESLA MR scanners (Siemens, Germany) and a gradient-echo echo-planar imaging pulse sequence was used to acquire fMRI values with the following specific parameters: 240 functional images (repetition time = 2,000 ms, echo time = 30 ms, thickness = 4.0 mm, gap = 1.2 mm, acquisition matrix = 64 × 64, flip angle = 90°, field of view = 220 × 220 mm, 29 axial) were obtained. All MRI images were examined for structural abnormalities, and no subject was excluded on this basis.
Functional Magnetic Resonance Imaging Data Processing
The CAT12 toolkit (12.71) from the Statistical Parametric Mapping database (SPM122) was used to analyze the data. All procedures were performed using MATLAB 7.9.0 software (R2009b; The Mathworks, Inc., Natick, MA, United States). Preprocessing included calibration, correction for head movement, image structure and average echo planar imaging alignment, normalization to a standard template, and smoothing using a Gaussian of 8 mm full width at half maximum. The fMRI brain functional images of each subject were co-registered with the T1 brain structure image template of the Montreal Neurological Institute (MNI) space as the reference standard, and the spatial standardization was completed using the Diffeomorphic Anatomical Registration Through Exponentiated Lie algebra (DARTEL) method. In addition, the gray matter volumes were normalized and smoothed using a 6-mm full width at half maximum Gaussian kernel.
Correlation Analysis
LogMAR acuity tests were used to assess the patients’ visual monocular acuity. The correlations between rTMS and logarithmic MAR values in the AR (P = 0.0066), FR (P < 0.0001), and CMR (P = 0.0004) regions were analyzed using GRAPHIPAD Prism 8. Correlation graphs were produced.
Statistical Analysis
After controlling for age and sex, the repeated measures t-test was used to compare the amplitude of low-frequency fluctuation (ALFF) between PRT and POT. The significance level was set at P < 0.05, family wise error corrected, voxel level P < 0.001, and cluster level P < 0.05. A color map was created by overlapping the significant voxels by standardization of 3-dimensional magnetization prepared fast acquisition gradient echo sequences.
Results
Demographics and Visual Measurements
There were no significant differences in age and log MAR-R(BCVA) between the POT and PRT. There were statistically notable differences in the log MAR-L(BCVA) (P < 0.05) between the two groups (more details are presented in Table 1).
Differences in Amplitude of Low-Frequency Fluctuation
At the PRT stage, the ALFF values were significantly higher in the AR, PIL, and CMR [Figure 2 (red) and Table 2]. and were significantly lower in the FR and FIL compared to the POT stage [Figure 2 (blue), 3 and Table 2]. No significant difference was found in ALFF between PRT and POT in other brain regions (P > 0.05).
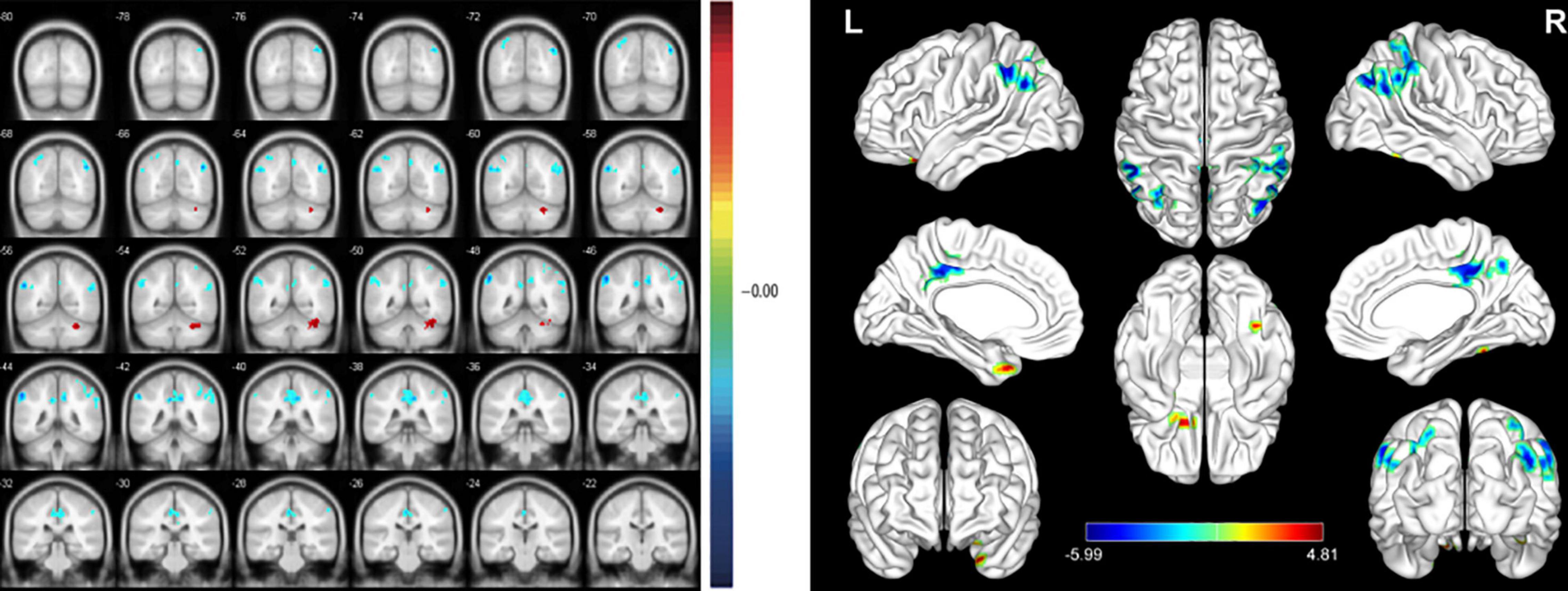
Figure 2. Significant differences in spontaneous brain activity between the pre-rTMS (PRT) and post-rTMS (POT). The sizes of the spots denote the degree of quantitative changes. The different brain regions were observed in the Angular_R (AR), Parietal_Inf_L (PIL), Cingulum_Mid_R (CMR), Fusiform_R (FR) and Frontal_Inf_Orb_L (FIL). The red areas denote that PRT exhibit higher amplitude of low-frequency fluctuation (ALFF) in brain areas than POT and the blue areas denote brain regions with a lower ALFF [P < 0.001, cluster > 13 voxels, Alphasim corrected)].
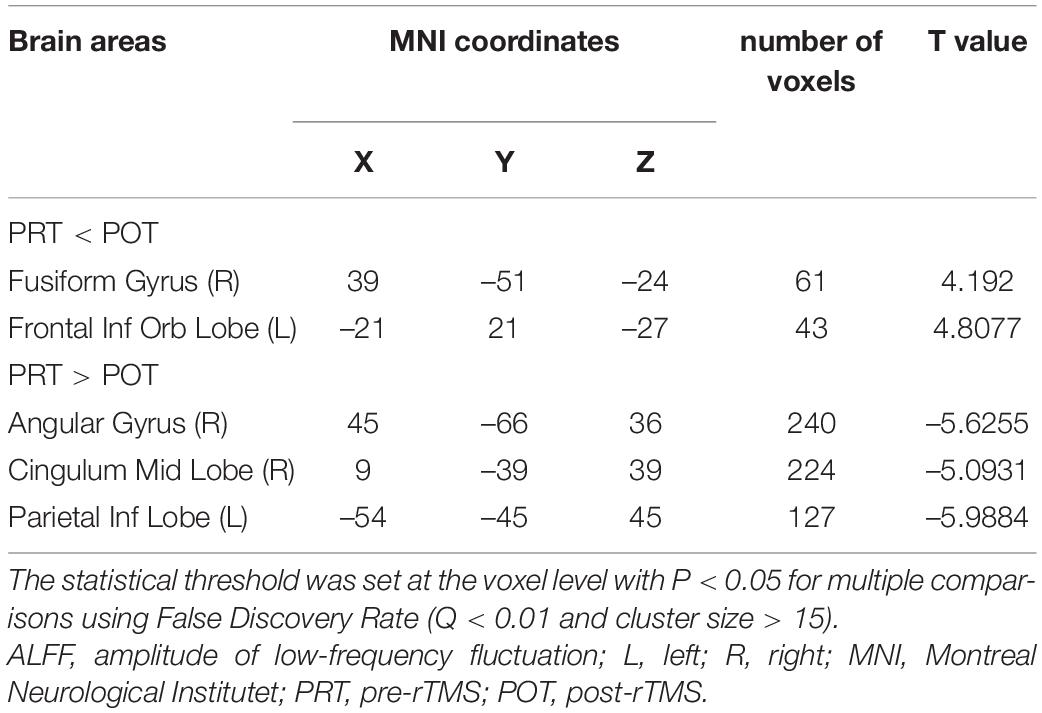
Table 2. Brain areas with significantly different amplitude of low-frequency fluctuation (ALFF) between groups.
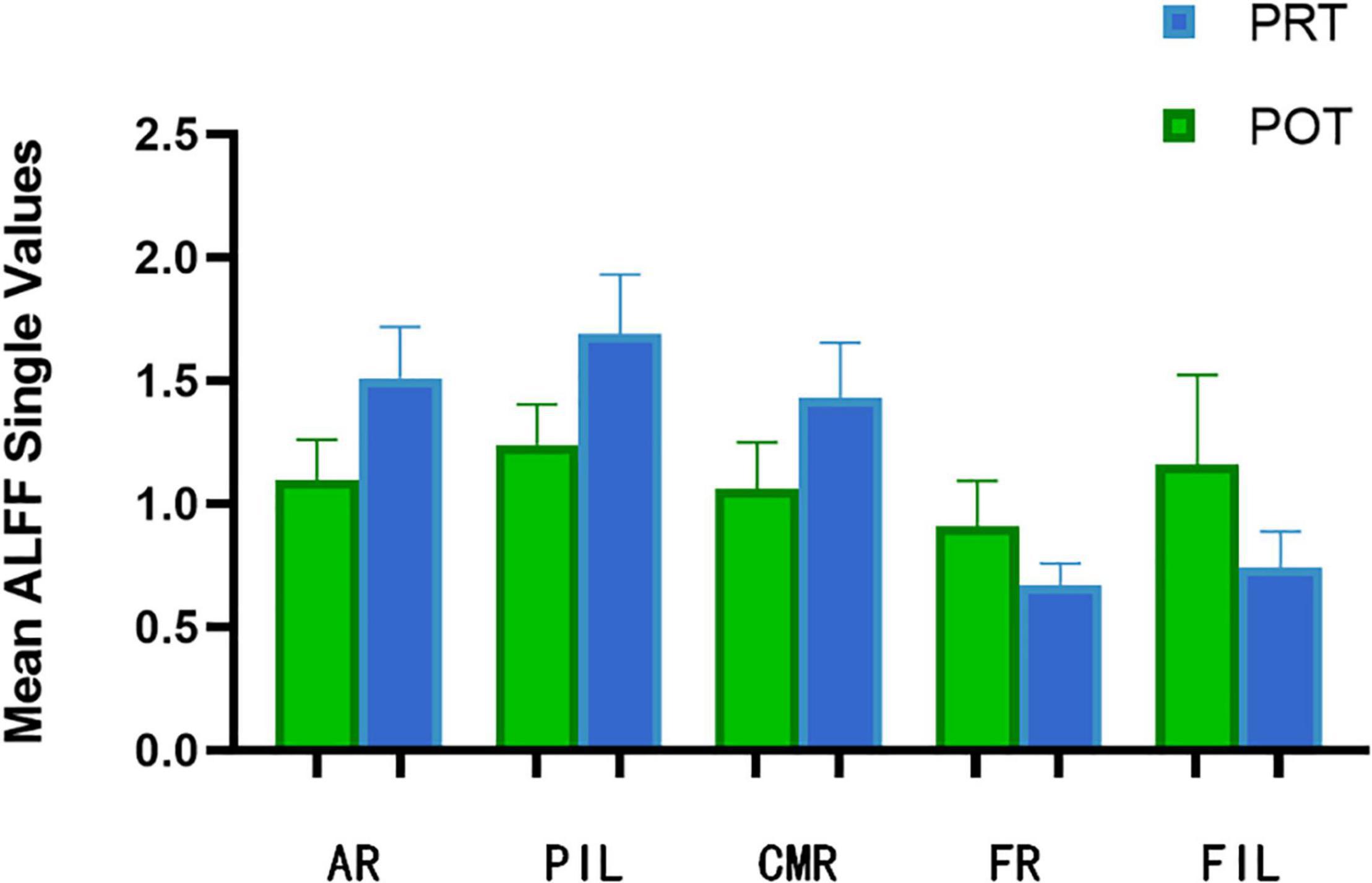
Figure 3. The mean ALFF values between POT and PRT. ALFF, amplitude of low-frequency fluctuation; PRT, pre-rTMS; POT, post-rTMS; L-left; R-right; AR, Angular_R; PIL, Parietal_Inf_L; CMR, Cingulum_Mid_R; FR, Fusiform_R; FIL, Frontal_Inf_Orb_L.
Correlation Analysis
At the POT stage, significant correlations between ALFF signal value and logMAR acuity were found and were positive at AR (r2 = 0.3286, P = 0.0066; Figure 4A) and negative at FR (r2 = 0.8466, P < 0.0001; Figure 4B) and positive at CMR (r2 = 0.4940, P = 0.0004; Figure 4C). Changes in ALFF values in brain regions are associated with changes in visual acuity and brain activity (Figures 5, 6).
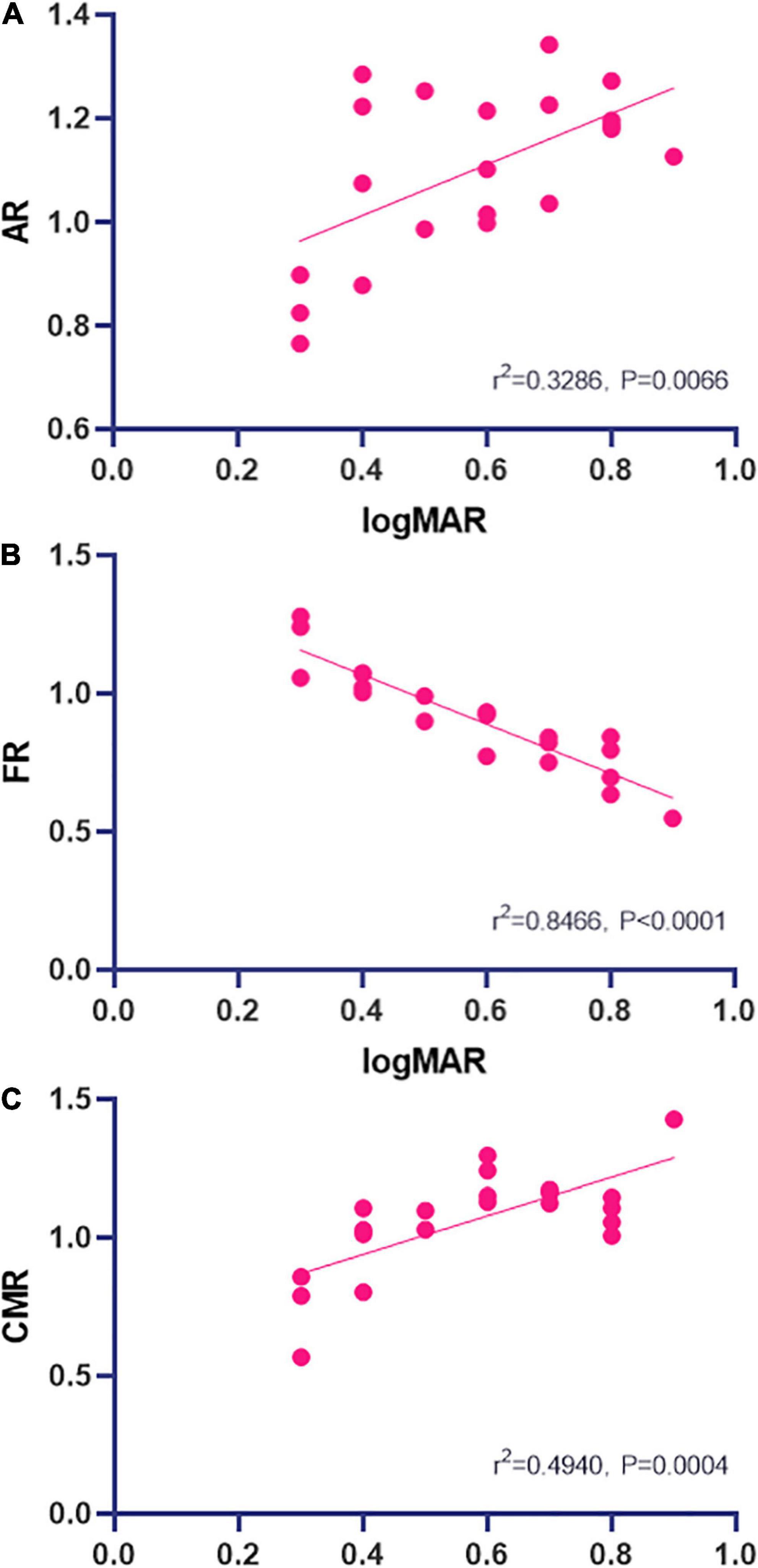
Figure 4. Correlations between mean ALFF signal values and logMAR acuity. The ALFF signal value of AR showed a positive correlation with logMAR [r2 = 0.3286, P = 0.0066; (A)] and the ALFF signal value of FR showed a negative correlation with logMAR [r2 = 0.8466, P < 0.0001; (B)]. The logMAR showed a positive correlation with the ALFF signal value of CMR [r2 = 0.4940, P = 0.0004; (C)] (A lower LogMAR value means better acuity). ALFF, amplitude of low-frequency fluctuation; AR, Angular_R; CMR, Cingulum_Mid_R; FR, Fusiform_R.
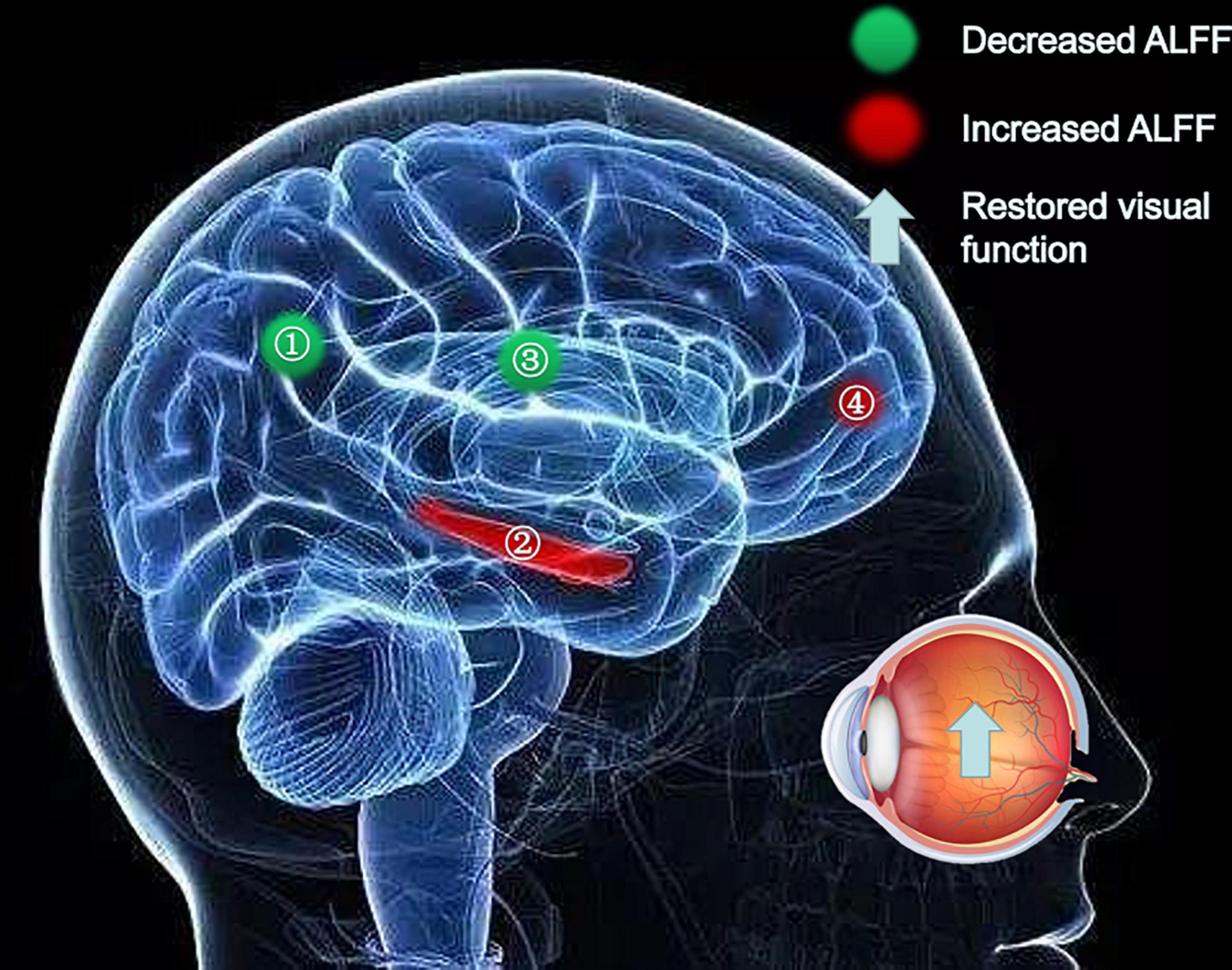
Figure 5. Significant differences in spontaneous brain activity between the PRL and POL stages. Different brain regions that were observed: (1) Angular_R and Parietal_Inf_L; (2) Fusiform_R; (3) Cingulum_Mid_R; (4) Frontal_Inf_Orb_L. The red areas indicate increased ALFF values, and the green areas indicate decreased ALFF values. ALFF, amplitude of low-frequency fluctuation; L, left; R, right.
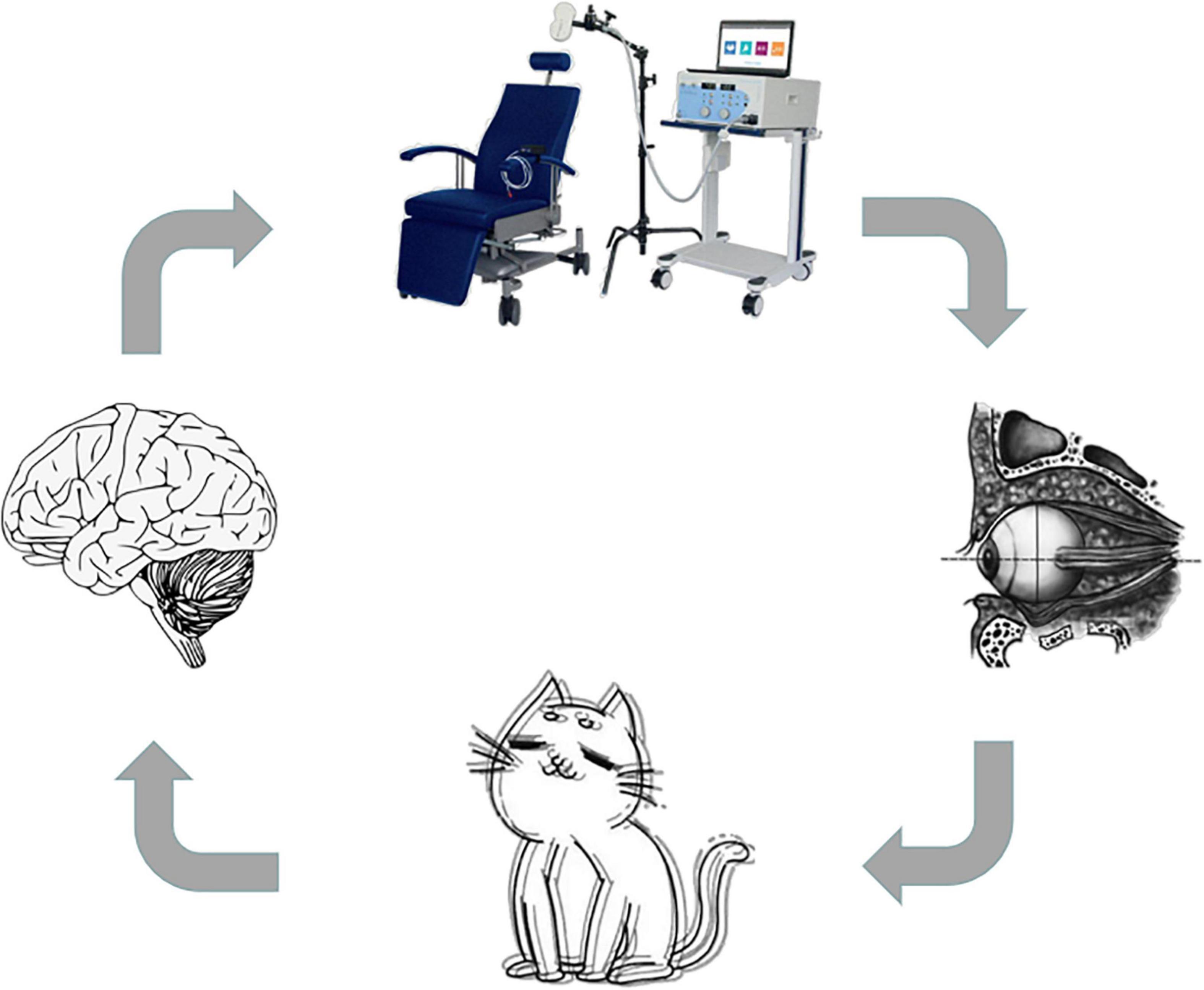
Figure 6. Relationship between rTMS and diplopia. Patients with SA may develop diplopia after surgery. Once diplopia occurs, visual function may be affected, leading to abnormal neural activity in brain regions. SA, oblique amblyopia disease.
Receiver Operating Characteristic Curves
We tested the hypothesis that ALFF may be a potential diagnostic indicator of SA patients at the PRT stage by plotting the average ALFF values of each brain region on an ROC curve. Brain regions with significantly different ALFF values showed high accuracy as diagnostic markers (PRT > POT). The AUCs of the ALFF values of the different brain regions were as follows: CMR (0.907, p < 0.001), AR (0.961 p < 0.001), PIL (0.944, p < 0.001), FR (0.874, p < 0.001), FIL (0.899, p < 0.001) (Figure 7).
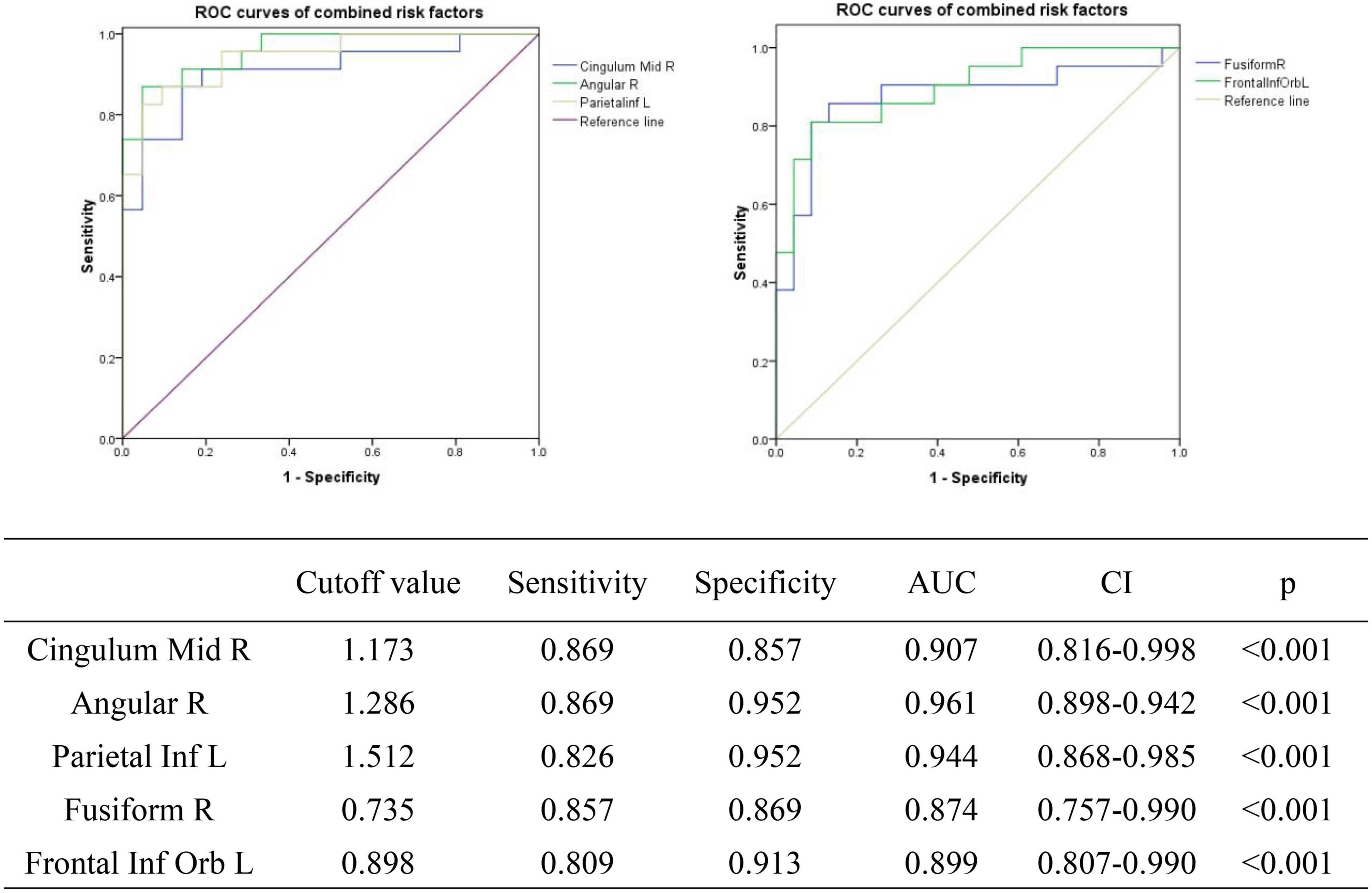
Figure 7. ROC curve analysis of the mean ALFF values of the affected brain regions in SA patients. ROC, receiver operating characteristic; AUC, area under the curve; CI, confidence interval; ROC, receiver operating characteristic; ALFF, amplitude of low-frequency fluctuation.
Discussion
Amplitude of low-frequency fluctuation (ALFF) is a commonly used clinical method which reflects changes in spontaneous brain activity and has been applied in the investigation of several ophthalmological diseases. Amplitude of low-frequency fluctuation is used to measure spontaneous fluctuations in blood oxygen level-dependent fMRI-signal intensity for nervous activity, reflecting the intensity of regional spontaneous brain activity at rest. The increase of ALFF indicated increased blood oxygen dependence level and increased activity in this brain region, on the contrary, activity of the brain area decreased. We used the ALFF sequence to compare the activity of different brain regions in POT and PRT. Our study is the first to determine whether there are ALFF differences in brain regions before and after transcranial magnetic stimulation within SA patients after surgery, and to identify those regions. Functional MRI is one of the most widely used functional brain imaging techniques. ALFF has been applied in ophthalmologic and neurogenic diseases, and in this study (Table 3) we demonstrated that the intrinsic patterns of activity in different brain regions of POT were changed after rTMS.
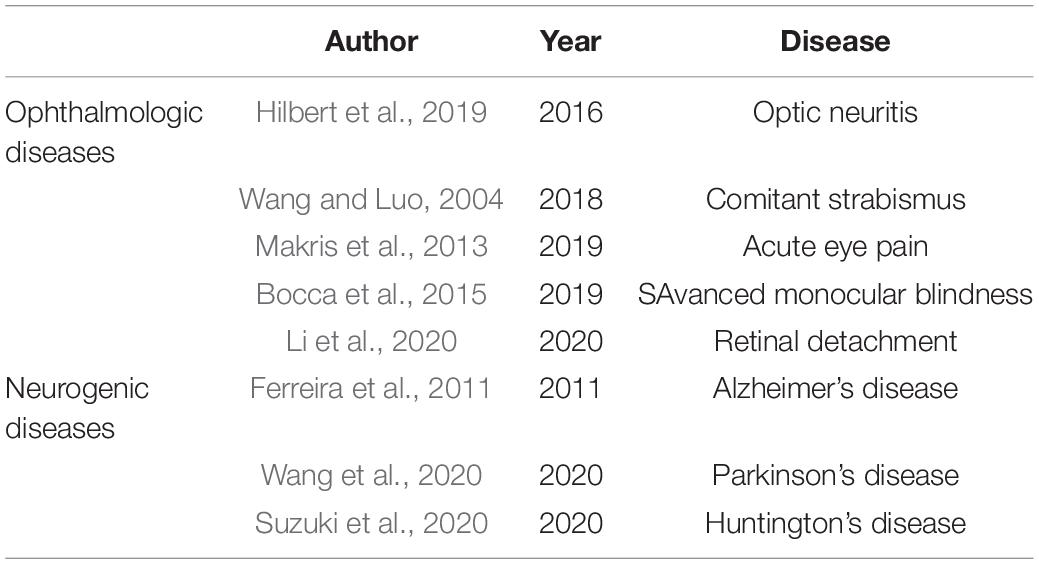
Table 3. Amplitude of low-frequency fluctuation (ALFF) method applied in Ophthalmologic and Neurogenic diseases.
We collected information on the brain regions where ALFF changed after rTMS treatment, including areas unrelated to visual processing (Table 4). There were significant differences in ALFF values in several brain areas between POT and PRT stages. Values were decreased in the FR and FIL regions, but increased in the AR, PIL, and CMR regions. These results demonstrate bidirectional changes in ALFF values between the POT and PRT stages. The ROC curve shows the clinical diagnostic significance of both the left parietal inferior, right cingulum middle, right angular, right fusiform, and left frontal inferior orb regions. The AUCs of all these brain areas are high, indicating that ALFF may be used in clinical diagnosis of other brain diseases in SA patients after surgery.
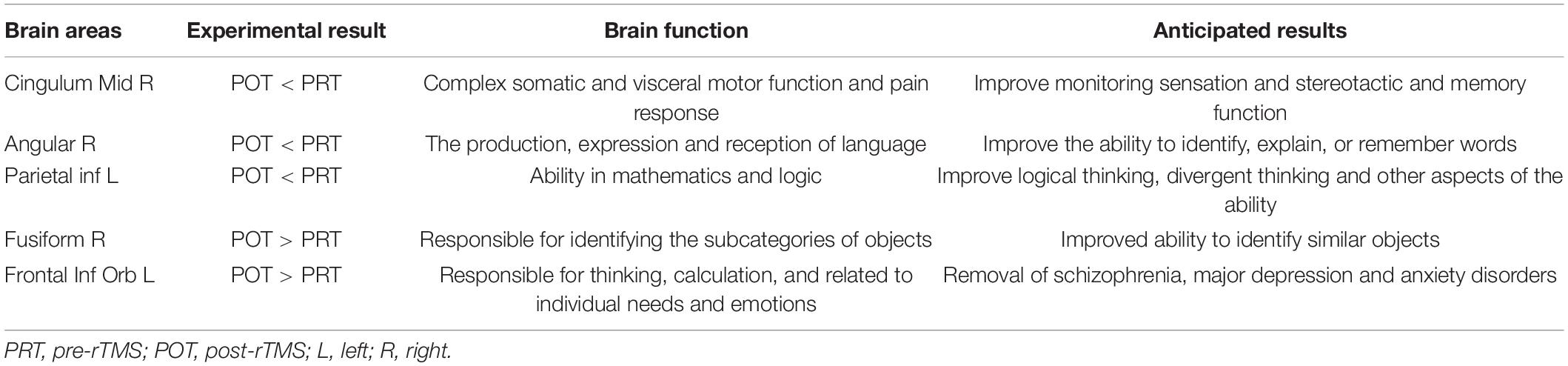
Table 4. Brain areas with altered amplitude of low-frequency fluctuation (ALFF) and potential impacts.
Of these areas, the fusiform gyrus is located at the middle inferior part of the visual association cortex. The fusiform gyrus is not only used for face recognition, it is also responsible for the recognition of subcategories of objects (SparkNotes, 2007). In the fusiform face area, most studies have shown that the right hemisphere is more important for face recognition than the left. The face recognition area is located in the right fusiform gyrus, but it is involved in the recognition of a variety of complex stimuli and is a key brain region for acquiring the skills to distinguish similar objects (Tordesillas-Gutierrez et al., 2018). In patients with strabismic amblyopia, rTMS treatment significantly improved the condition of postoperative visual recovery, which may be related to the changes in the activity of the fusiform gyrus.
The angular gyrus, above Wernicke’s area, at the parietal-occipital junction, is an important association area in the back of the brain. If the angular gyrus is removed, the visual and auditory perception of words will lose connection, causing dyslexia (a reading and writing disorder) and audio-visual aphasia. In the latter, the sufferer loses the connection between what is seen and what is heard and is unable to understand the meaning of the words (Tordesillas-Gutierrez et al., 2018; Palejwala et al., 2020).
The middle part of the right cingulate is the gyrus between the cingulate sulcus of the medial part of the brain hemisphere and the sulcus of the corpus callosum, belonging to the cortical part of the limbic system (Creel, 2012). This brain region transmits nerve impulses to the anterior cingulate gyrus and striatum and receives output from the amygdala, orbitofrontal gyrus, and medial frontal gyrus (Li et al., 2017). It has long been understood as an important part of the emotional circuit, involved in processes such as emotion and self-evaluation, and is closely associated with depressive symptoms. Audiovisual hallucinations, sensory illusions, and emotional symptoms caused by diplopia onset usually occur after surgery and accompanied by a slow recovery period of 12 months. After rTMS treatment, the condition will be greatly reduced (Sasaki et al., 2016; Craciun et al., 2018). Primary epileptic seizures in the cingulate gyrus are mainly complex partial seizures with characteristic automaticity, autonomic nervous dysfunction, affective changes, and urinary incontinence. We speculate that rTMS can effectively control epilepsy in part of the cingulate gyrus brain region. SA may cause abnormalities in cerebral blood flow and metabolism in the brain, resulting in hypofunction, which in turn leads to depression in patients (Craciun et al., 2018).
The lower and upper parietal gyrus contain visual segmentation groups, which can be distinguished according to structural or connection standards, topographic organization, or other functional standards. The conventional view that this brain area is a type of visual sensory area and contains numerous optic motor neurons and parietal visual function focuses on its role in spatial perception, so the lesions in this area will cause abnormalities in the relevant visual field. Therefore, our research showing a decreased ALFF of the left parietal inferior region may manifest as a defect in the visual field, and this pathological change will seriously affect the daily life of the patient (Hilbert et al., 2019).
Previous studies have demonstrated a link between the frontal cortex and emotion. The occurrence and development of the clinical symptoms of schizophrenia, major depression, and anxiety depend on the frontal cortex system. Evidence suggests that affective function, experience, expression, and load information processing have different neural representations in the frontal cortex (Wang and Luo, 2004; Makris et al., 2013; Bocca et al., 2015). Therefore, it is reasonable to infer that the effect of rTMS on the activity of the frontal cortex may be useful for the treatment of patients with schizophrenia, major depression, and anxiety disorders.
We believe that ALFF has clinical significance, it can detect abnormal changes in the brain activity of patients through fMRI in advance of this factor, and provide timely interventions to effectively reduce the sequelae and complications of SA such as diplopia. It is worth noting that the limitations of the current study, including differences in measurement standards and other factors, require further unification of measurement scales in in-depth studies to verify the findings. The clinical features we used in this study were not rigorous. For example, different SA patients have different degrees of visual acuity recovery after surgery, some of which were not significantly different from the preoperative stage. Therefore, attention should be paid to these problems in future studies and the sample size should be expanded to accurately evaluate the changes of brain ALFF indexes in SA patients after surgery. In addition, the control group of patients treated with rTMS after SA surgery was not included in this study, changes in ALFF values generated by longitudinal brain recombination after SA surgery were not measured. As a result, in the results obtained in this study, the difference in ALFF value is not solely affected by rTMS treatment, and part of the difference may be related to longitudinal brain reorganization, the specific differences need to be further studied. Despite these deficiencies, this study revealed specific changes and effects of rTMS on ALFF in the brain regions of SA patients.
Conclusion
In summary, we confirmed that within SA patients ALFF changes significantly in some brain regions after rTMS. Changes in ALFF reflect increases or decreases in activity within brain regions and may partly reflect the degree of improvement in visual dysfunction caused by postoperative complications in patients with SA. ALFF may be used for clinical diagnosis and evaluation of postoperative rehabilitation in patients with SA.
Data Availability Statement
The raw data supporting the conclusions of this article will be made available by the authors, without undue reservation.
Ethics Statement
All research methods were approved by the Committee of the Medical Ethics of the First Affiliated Hospital of Nanchang University and were in accordance with the 1964 Helsinki declaration and its later amendments or comparable ethical standards. All subjects were explained the purpose, method, potential risks and signed an informed consent form. Written informed consent to participate in this study was provided by the participants’ legal guardian/next of kin. Written informed consent was obtained from the individual(s), and minor(s)’ legal guardian/next of kin, for the publication of any potentially identifiable images or data included in this article.
Author Contributions
Y-NW and Y-CP analyzed the data and drafted the manuscript. H-YS, Q-MG, and Y-CP assisted with data interpretation and figure composing. L-JZ, R-BL, and Q-YL collected the data. YS conceived, designed, and directed the study and finally revised and approved the manuscript.
Funding
The Central Government Guides Local Science and Technology Development Foundation (No: 20211ZDG02003); Key Research Foundation of Jiangxi Province (Nos: 20181BBG70004 and 20203BBG73059); Excellent Talents Development Project of Jiangxi Province (No: 20192BCBL23020); Natural Science Foundation of Jiangxi Province (No: 20181BAB205034); Grassroots Health Appropriate Technology “Spark Promotion Plan” Project of Jiangxi Province (No: 20188003); Health Development Planning Commission Science Foundation of Jiangxi Province (Nos: 20201032 and 202130210); Health Development Planning Commission Science TCM Foundation of Jiangxi Province (Nos: 2018A060 and 2020A0087); Education Department Foundation of Jiangxi Province (Nos: GJJ200157, GJJ200159, and GJJ200169).
Conflict of Interest
The authors declare that the research was conducted in the absence of any commercial or financial relationships that could be construed as a potential conflict of interest.
Publisher’s Note
All claims expressed in this article are solely those of the authors and do not necessarily represent those of their affiliated organizations, or those of the publisher, the editors and the reviewers. Any product that may be evaluated in this article, or claim that may be made by its manufacturer, is not guaranteed or endorsed by the publisher.
Footnotes
References
Abdelrahman, A. A., Noaman, M., Fawzy, M., Moheb, A., Karim, A. A., and Khedr, E. M. (2021). A double-blind randomized clinical trial of high frequency rTMS over the DLPFC on nicotine dependence, anxiety and depression. Sci. Rep. 11:1640. doi: 10.1038/s41598-020-80927-5
Arns, M., Bervoets, C., van Eijndhoven, P., Baeken, C., van den Heuvel, O. A., Aleman, A., et al. (2019). Consensusverklaring voor de toepassing van rTMS bij depressie in Nederland en België [Consensus statement on the application of rTMS in depression in the Netherlands and Belgium]. Tijdschr. Psychiatr. 61, 411–420.
Bocca, F., Töllner, T., Müller, H. J., and Taylor, P. C. (2015). The right angular gyrus combines perceptual and response-related expectancies in visual search: TMS-EEG evidence. Brain Stimul. 8, 816–822. doi: 10.1016/j.brs.2015.02.001
Brown, H. D. H., Woodall, R. L., and Kitching, R. E. (2016). Using magnetic resonance imaging to assess visual deficits: a review. Ophthalmic Physiol. Opt. 36, 240–265. doi: 10.1111/opo.12293
Brunoni, A. R., Chaimani, A., Moffa, A. H., Razza, L. B., Gattaz, W. F., Daskalakis, Z. J., et al. (2017). Repetitive Transcranial Magnetic Stimulation for the Acute Treatment of Major Depressive Episodes: A Systematic Review With Network Meta-analysis. JAMA Psychiatry 74, 143–152. doi: 10.1001/jamapsychiatry.2016.3644
Cerulli Irelli, E., Di Pietro, G., Fisco, G., Orlando, B., Asci, F., Salamone, E. M., et al. (2021). Acute-onset binocular diplopia in neurological unit: aetiological factors and diagnostic assessment. Acta Neurol. Scand. 144, 92–98. doi: 10.1111/ane.13425
Chan, S. T., Tang, K. W., Lam, K. C., Chan, L. K., Mendola, J. D., and Kwong, K. K. (2004). Neuroanatomy of adult strabismus: a voxel-based morphometric analysis of magnetic resonance structural scans. Neuroimage 22, 986–994. doi: 10.1016/j.neuroimage.2004.02.021
Chen, X., Fu, Z., Yu, J., Ding, H., Bai, J., Chen, J., et al. (2014). Prevalence of amblyopia and strabismus in Eastern China: results from screening of preschool children aged 36–72 months. Br. J. Ophthalmol. 100, 515–519. doi: 10.1136/bjophthalmol-2015-306999
Chou, Y. H., Ton That, V., and Sundman, M. (2020). A systematic review and meta-analysis of rTMS effects on cognitive enhancement in mild cognitive impairment and Alzheimer’s disease. Neurobiol. Aging 86, 1–10. doi: 10.1016/j.neurobiolaging.2019.08.020
Conner, I. P., Odom, J. V., Schwartz, T. L., and Mendola, J. D. (2007). Monocular activation of V1 and V2 in amblyopic adults measured with functional magnetic resonance imaging. J. AAPOS. 11, 341–350. doi: 10.1016/j.jaapos.2007.01.119
Craciun, L., Taussig, D., Ferrand-Sorbets, S., Pasqualini, E., Biraben, A., Delalande, O., et al. (2018). Investigation of paediatric occipital epilepsy using stereo-EEG reveals a better surgical outcome than in adults, especially when the supracalcarine area is affected. Epileptic Disord. 20, 346–363. doi: 10.1684/epd.2018.1000
Creel, D. J. (2012). Visually Evoked Potentials—Webvision—NCBI Bookshelf. Salt Lake City: University of Utah Health Sciences Center.
Fazzi, D. E., and Bianchi, P. E. (2016). “Visual development in childhood,” in Visual Impairments and Developmental Disorders: From diagnosis to rehabilitation, ed. L. John (London: Mariani Foundation Paediatric Neurology).
Ferreira, L. K., Diniz, B. S., Forlenza, O. V., Busatto, G. F., and Zanetti, M. V. (2011). Neurostructural predictors of Alzheimer’s disease: a meta-analysis of VBM studies. Neurobiol Aging 32, 1733–1741. doi: 10.1016/j.neurobiolaging.2009.11.008
Goodyear, B. G., and Menon, R. S. (2001). Brief visual stimulation allows mapping of ocular dominance in visual cortex using fMRI. Hum. Brain Mapp. 14, 210–217. doi: 10.1002/hbm.1053
Goseki, T., Suh, S. Y., Robbins, L., Pineles, S. L., Demer, J. L., and Velez, F. G. (2021). Reply to: comment on Prevalence of Sagging Eye Syndrome in Adults with Binocular Diplopia. Am. J. Ophthalmol. 221, 324–325. doi: 10.1016/j.ajo.2020.08.010
Hilbert, S., McAssey, M., Bühner, M., Schwaferts, P., Gruber, M., Goerigk, S., et al. (2019). Right hemisphere occipital rTMS impairs working memory in visualizers but not in verbalizers. Sci. Rep. 9:6307. doi: 10.1038/s41598-019-42733-6
Jefferis, J. M., Connor, A. J., and Clarke, M. P. (2015). Amblyopia. BMJ 351:h5811. doi: 10.1136/bmj.h5811
Levi, D. (2013). Linking assumptions in amblyopia. Vis. Neurosci. 30, 277–287. doi: 10.1017/S0952523813000023
Li, B., Liu, Y. X., Li, H. J., Yuan, Q., Zhu, P. W., Ye, L., et al. (2020). Reduced gray matter volume in patients with retinal detachment: evidence from a voxel-based morphometry study. Acta Radiol. 61, 395–403. doi: 10.1177/0284185119861898
Li, D., Zhang, H., Jia, W., Zhang, L., Zhang, J., Liu, W., et al. (2017). Significance of the Tentorial Alignment in Protecting the Occipital Lobe with the Poppen Approach for Tentorial or Pineal Area Meningiomas. World Neurosurg. 108, 453–459. doi: 10.1016/j.wneu.2017.08.013
Liang, M., Xie, B., Yang, H., Yin, X., Wang, H., Yu, L., et al. (2017). Altered interhemispheric functional connectivity in patients with anisometropic and strabismic amblyopia: a resting-state fMRI study. Neuroradiology 59, 517–524. doi: 10.1007/s00234-017-1824-0
Maconachie, G. D., and Gottlob, I. (2015). The challenges of amblyopia treatment. Biomed. J. 38, 510–516. doi: 10.1016/j.bj.2015.06.001
Makris, N., Preti, M. G., Wassermann, D., Rathi, Y., Papadimitriou, G. M., Yergatian, C., et al. (2013). Human middle longitudinal fascicle: segregation and behavioral-clinical implications of two distinct fiber connections linking temporal pole and superior temporal gyrus with the angular gyrus or superior parietal lobule using multi-tensor tractography. Brain Imag. Behav. 7, 335–352. doi: 10.1007/s11682-013-9235-2
Malkani, R. G., and Zee, P. C. (2020). Brain Stimulation for Improving Sleep and Memory. Sleep Med. Clin. 15, 101–115. doi: 10.1016/j.jsmc.2019.11.002
McClintock, S. M., Reti, I. M., Carpenter, L. L., McDonald, W. M., Dubin, M., Taylor, S. F., et al. (2018). Consensus Recommendations for the Clinical Application of Repetitive Transcranial Magnetic Stimulation (rTMS) in the Treatment of Depression. J. Clin. Psychiatry 79:16cs10905. doi: 10.4088/JCP.16cs10905
Min, Y. L., Su, T., Shu, Y. Q., Liu, W. F., Chen, L. L., Shi, W. Q., et al. (2018). Altered spontaneous brain activity patterns in strabismus with amblyopia patients using amplitude of low-frequency fluctuation: a resting-state fMRI study. Neuropsychiatr. Dis. Treatment 14, 2351–2359. doi: 10.2147/NDT.S171462
Mohney, B. G., Martinez, J. M., Holmes, J. M., and Diehl, N. N. (2012). Incidence of strabismus in an adult population. J. AAPOS. 16:e23. doi: 10.1016/j.jaapos.2011.12.089
Palejwala, A. H., O’Connor, K. P., Pelargos, P., Briggs, R. G., Milton, C. K., Conner, A. K., et al. (2020). Anatomy and white matter connections of the lateral occipital cortex. Surg. Radiol. Anat. 42, 315–328. doi: 10.1007/s00276-019-02371-z
Peña Urbina, P., Hernández García, E., Gómez de Liaño Sánchez, R., and Domingo Gordo, B. (2021). Restrictive strabismus and diplopia after conjunctivodacryocystorhinostomy with Jones tube. J. Fr. Ophtalmol. 44, e187–e190. doi: 10.1016/j.jfo.2020.08.005
Rehn, S., Eslick, G. D., and Brakoulias, V. (2018). A Meta-Analysis of the Effectiveness of Different Cortical Targets Used in Repetitive Transcranial Magnetic Stimulation (rTMS) for the Treatment of Obsessive-Compulsive Disorder (OCD). Psychiatry Q. 89, 645–665. doi: 10.1007/s11126-018-9566-7
Rigucci, S., Serafini, G., Pompili, M., Kotzalidis, G. D., and Tatarelli, R. (2010). Anatomical and functional correlates in major depressive disorder: the contribution of neuroimaging studies. World J. Biol. Psychiatry 11, 165–180. doi: 10.1080/15622970903131571
Sasaki, F., Kawajiri, S., Nakajima, S., Yamaguchi, A., Tomizawa, Y., Noda, K., et al. (2016). Occipital lobe seizures and subcortical T2 and T2* hypointensity associated with nonketotic hyperglycemia: a case report. J. Med. Case Rep. 10:228. doi: 10.1186/s13256-016-1010-8
Schwartz, M. W., Bell, L. M., Bingham, P. M., Chung, E. K., Cohen, M. I., Friedman, D. F., et al. (2002). The 5-minute Pediatric Consult, 3rd Edn. Philadelphia: Lippincott Williams & Wilkins.
Sebastianelli, L., Versace, V., Martignago, S., Brigo, F., Trinka, E., Saltuari, L., et al. (2017). Low-frequency rTMS of the unaffected hemisphere in stroke patients: A systematic review. Acta Neurol. Scand. 136, 585–605. doi: 10.1111/ane.12773
Spiegel, D. P., Byblow, W. D., Hess, R. F., and Thompson, B. (2013). Anodal transcranial direct current stimulation transiently improves contrast sensitivity and normalizes visual cortex activation in individuals with amblyopia. Neurorehabilit. Neural Repair 27, 760–769. doi: 10.1177/1545968313491006
Suzuki, F., Sato, N., Ota, M., Sugiyama, A., Shigemoto, Y., Morimoto, E., et al. (2020). Discriminating chorea-acanthocytosis from Huntington’s disease with single-case voxel-based morphometry analysis. J. Neurol. Sci. 408:116545. doi: 10.1016/j.jns.2019.116545
Tarczy-Hornoch, K., Cotter, S. A., Borchert, M., McKean-Cowdin, R., Lin, J., Wen, G., et al. (2013). Prevalence and causes of visual impairment in Asian and non-Hispanic white preschool children: multiethnic pediatric eye disease study. Ophthalmology 120, 1220–1226. doi: 10.1016/j.ophtha.2012.12.029
Thompson, B., Mansouri, B., Koski, L., and Hess, R. F. (2008). Brain plasticity in the adult: modulation of function in amblyopia with rTMS. Curr. Biol. 18, 1067–1071. doi: 10.1016/j.cub.2008.06.052
Tordesillas-Gutierrez, D., Ayesa-Arriola, R., Delgado-Alvarado, M., Robinson, J. L., Lopez-Morinigo, J., Pujol, J., et al. (2018). The right occipital lobe and poor insight in first-episode psychosis. PLoS One 13:e0197715. doi: 10.1371/journal.pone.0197715
Wang, N. Y., and Luo, Y. J. (2004). Emotional abnormalities in patients with prefrontal cortex damage. Adv. Psychol. Sci. 2, 161–167.
Wang, X., Cui, D., Zheng, L., Yang, X., Yang, H., and Zeng, J. (2012). Combination of blood oxygen level-dependent functional magnetic resonance imaging and visual evoked potential recordings for abnormal visual cortex in two types of amblyopia. Mol. Vis. 18, 909–919.
Wang, Z., Liu, Y., Ruan, X., Li, Y., Li, E., Zhang, G., et al. (2020). Aberrant amplitude of low-frequency fluctuations in different frequency bands in patients with Parkinson’s disease. Front. Aging Neurosci. 12:576682. doi: 10.3389/fnagi.2020.576682
Webber, A. L., and Wood, J. (2005). Amblyopia: prevalence, natural history, functional effects and treatment. Clin. Exp. Optometr. 88, 365–375. doi: 10.1111/j.1444-0938.2005.tb05102.x
Keywords: low-frequency rTMS, strabismic amblyopia, amplitude of low-frequency fluctuation (ALFF), spontaneous brain activity, ophthalmological
Citation: Wang Y-N, Pan Y-C, Shu H-Y, Zhang L-J, Li Q-Y, Ge Q-M, Liang R-B and Shao Y (2022) Altered Spontaneous Brain Activity Patterns in Children With Strabismic Amblyopia After Low-Frequency Repetitive Transcranial Magnetic Stimulation: A Resting-State Functional Magnetic Resonance Imaging Study. Front. Hum. Neurosci. 16:790678. doi: 10.3389/fnhum.2022.790678
Received: 22 November 2021; Accepted: 18 March 2022;
Published: 08 April 2022.
Edited by:
Nan-Kuei Chen, University of Arizona, United StatesReviewed by:
Jennifer Kate Evelyn Steeves, York University, CanadaGuanghui Liu, Fujian Provincial People’s Hospital, China
Copyright © 2022 Wang, Pan, Shu, Zhang, Li, Ge, Liang and Shao. This is an open-access article distributed under the terms of the Creative Commons Attribution License (CC BY). The use, distribution or reproduction in other forums is permitted, provided the original author(s) and the copyright owner(s) are credited and that the original publication in this journal is cited, in accordance with accepted academic practice. No use, distribution or reproduction is permitted which does not comply with these terms.
*Correspondence: Yi Shao, freebee99@163.com
†These authors have contributed equally to this work