- 1College of Physical and Health Education, Guangxi Normal University, Guilin, China
- 2Institute of Sports Medicine and Health, Chengdu Sport University, Chengdu, China
Objective: After a stroke, patients usually suffer from dysfunction, such as decreased balance ability, and abnormal walking function. Whole-body vibration training can promote muscle contraction, stimulate the proprioceptive system, enhance the muscle strength of low limbs and improve motor control ability. The study aims to evaluate the effectiveness of whole-body vibration training on the balance and walking function of patients with stroke.
Methods: PubMed, CNKI, VIP, CBM, EBSCO, Embase and Web of Science were searched. According to the inclusion and exclusion criteria, randomized controlled trials on the effectiveness of whole-body vibration training on the balance and walking function of patients with stroke were collected. The search time ranged from the date of database construction to November 2022. The included trials were evaluated by the Cochrane risk-of-bias tool. The meta-analysis was performed using two software packages, consisting of RevMan 5.4 and Stata 12.2. If the results included in the literature were continuous variables, use the mean difference (MD) and 95% confidence interval (CI) for statistics.
Results: (1) A total of 22 randomized controlled trials (RCTs) with a total of 1089 patients were included. (2) The results of meta-analysis showed that: compared with the controls, step length (MD = 6.12, 95%CI [5.63, 6.62], p < 0.001), step speed (MD = 0.14, 95%CI [0.09, 0.20], p < 0.001), cadence (MD = 9.03, 95%CI [2.23, 15.83], p = 0.009), stride length (MD = 6.74, 95%CI [−3.47, 10.01], p < 0.001), Berg Balance Scale (BBS) (MD = 4.08, 95%CI [2.39, 5.76], p < 0.001), Timed Up-and-Go test (TUGT) (MD = −2.88, 95%CI [−4.94, 0.81], p = 0.006), 10-meter Walk Test (10MWT) (MD = −2.69, 95%CI [−3.35, −2.03], p < 0.001), functional ambulation category scale (FAC) (MD = 0.78, 95%CI [0.65, 0.91], p < 0.001), Fugl-Meyer motor assessment of lower extremity (FMA-LE) (MD = 4.10, 95%CI [2.01, 6.20], p = 0.0001). (3) The results of subgroup analysis showed that, compared with other vibration frequencies, at 20–30 Hz frequency, WBV training had an obvious improvement effect only in TUGT. (4) The safety analysis showed that WBV training may be safe.
Conclusion: Whole-body vibration training has a positive effect on the balance and walking function of patients with stroke. Thus, whole-body vibration training is a safe treatment method to improve the motor dysfunction of patients with stroke.
Systematic review registration: [http://www.crd.york.ac.uk/PROSPERO], identifier [CRD4202348263].
1. Introduction
Stroke is one of the most prevalent cerebrovascular diseases. According to the most recent data, stroke is the second leading cause of death worldwide. With a high disability rate, recurrence rate, and fatality rate, stroke is also the most common cause of adult disability (Sacco and Rundek, 2012; Khaku and Tadi, 2022; Yikun et al., 2023). In the world each year, 16 million people get a stroke, according to a report issued by WHO in 2020. Recently, stroke incidence has been progressively rising with each passing year and is inclining to be younger along with the prolongation of the human life span (Guzik and Bushnell, 2017; Khaku and Tadi, 2022; Zhang et al., 2022). This is a formidable challenge for the medical and health systems (Moreno-Segura et al., 2022). The effects of a stroke on the human body vary depending on the degree of severity and location of the damage, but motor dysfunction, which manifests as decreased muscle strength, muscle spasms, abnormal muscle movement patterns, joint stiffness, abnormal proprioception, and other symptoms, is the most frequent symptom (Van Criekinge et al., 2019; Wei and Cai, 2022). The symptoms above would lead to decreased balance ability and abnormal walking function. After a stroke, more than 70% of patients experience varying degrees of lower limb dysfunction with limited recovery of walking function, resulting in most of them being unable to maintain a healthy gait or walking speed (Wist et al., 2016; Virani et al., 2020). The physical and psychological health of patients, as well as their quality of life and ability to reintegrate into family and society, are all significantly impacted by these dysfunctions. For the recovery of the walking function of stroke patients, the primary therapeutic methods at present include medication, muscle paste, PNF, rehabilitation training, machine exoskeletons, and so on (Wang et al., 2019; Varvarousis et al., 2021; Calafiore et al., 2022; Moreno-Segura et al., 2022; Nguyen et al., 2022).
Whole-body vibration (WBV) training helps to improve the dysfunction of the nervous system and musculoskeletal system diseases (In et al., 2018; Cigdem Karacay et al., 2022; Wang et al., 2022), to prevent and relieve osteoporosis in the elderly (Pichler et al., 2013; Cheng et al., 2021), and to promote sports injury recovery and improve sports performance (Sierra-Guzmán et al., 2018; Marin-Puyalto et al., 2020; Cheng et al., 2021). WBV training is a training method to improve neuromuscular, which uses mechanical vibration and external resistance load to stimulate the body to cause muscle vibration and increase the adaptiveness of the central nervous system (Choi et al., 2017). The patients sit or stand on the vibration platform, then the exogenous stimuli with various amplitudes and frequencies are transmitted from the platform to the whole body through the sole of the foot. The “bone-muscle-nerve” series connection is established (Jaime et al., 2019). By causing local or entire body muscles to vibrate, the vibration stimulation can increase the activation degree of the muscle spindle, cause high-frequency discharge and recruit more motor units, thus promoting muscle contraction, stimulating the proprioception system, enhancing muscle strength of lower limbs, and improving motor control ability (Marín et al., 2015; Liu et al., 2022).
The meta-analyses on WBV training interventions for stroke patients performed separately by Yang et al. (2015) and Lu et al. (2015) showed that WBV training has little role in improving balance and walking function in stroke patients. Yang and Butler (2020) concluded that controlled whole-body vibration training may benefit balance and mobility immediately, but the effects may not persist in stroke patients. Park et al. (2018) found that the effect sizes of WBV training for balance and gait function were small, through a comparison of effect improvement in all aspects of stroke patients after WBV intervention. After a collated analysis of researches above, we found that the databases searched were mainly Embase, PubMed, EBSCO, and Web of Science. The results and conclusions may be influenced by the inadequacy of the number of literature searches. In addition, the assessment methods for gait in the studies above mainly included TUGT, 10/6MWT and FAC, and no valid evaluation of walking spatiotemporal paraments (step length, step speed, and cadence, etc.) was performed. Therefore, in conclusion, the range of database searching was increased and the evaluation indicators of walking spatiotemporal paraments were added for analysis in this study.
Some studies have found that WBV training plays a positive role in the recovery of balance and walking function in patients with stroke (Gu and Hwangbo, 2016; Yan et al., 2021; Chuan et al., 2022), while other studies have shown that there is no significant difference between WBV training and routine rehabilitation training (Ijaz Ahmed Burq et al., 2021; Liu et al., 2022). The objective of this meta-analysis is to ascertain the effect of WBV training on balance and walking function in the rehabilitation of stroke patients, compared with routine, sham, and no treatments. Additionally, it sought to ascertain whether WBV training can serve as an effective training intervention method to guide clinical practice.
2. Materials and methods
2.1. Retrieval strategy
This meta-analysis was planned and implemented according to the Preferred Reporting Items for Systematic Reviews and Meta-Analyses (PRISMA) guidelines (Moher et al., 2015). The protocol was registered on the international prospective register of systematic reviews (http://www.crd.york.ac.uk/PROSPERO), registration number: CRD42022348263.
PubMed, CNKI, VIP, CBM, EBSCO, Embase and Web of Science were searched. The search time ranged from the date of database construction to November 2022. The last retrieval date is November 30, 2022. The literature search was conducted using a combination of subject terms and free terms. The search terms included “stroke,” “cerebral apoplexy,” “cerebral infarction,” “encephalorrhagia,” “walk,” “gait,” “progression,” “balance,” “whole-body vibration training,” “vibration training,” “vibration,” “VT,” “WBVT.” In order to get all the randomized control trials related to the whole-body vibration training intervention on the balance and walking function of patients with stroke, we also traced the references of the retrieved literature to supplement the relevant literature. The full search strategy for each database is presented in Supplementary material.
2.2. Literature inclusion, exclusion criteria and outcome indicator
The inclusion criteria: (1) Participants: stroke patients at any stage and time, regardless of sex, age, race and nationality; (2) Study design: randomized controlled trials (RCTs); (3) Primary treatment methods: vibration training alone or in combination with other treatments; (4) Treatment methods for the control group: any other interventions, including routine treatment, sham treatment, and no treatment; (5) Literature type: journal articles.
The exclusion criteria: (1) Literature not published in English or Chinese; (2) Literature published repeatedly; (3) Literature that was unable to effectively extract data and obtain original texts; (4) Animal studies or cross-sectional studies.
The primary outcome indicators: (1) walking spatiotemporal parament, consisting of step length (cm), step speed (m/s), cadence (step/min), single support time (s), double support time (s), stride length (cm) and step time (s). (2) Berg Balance Scale (BBS): BBS is a comprehensive scale to assess balance function in stroke patients, including the combined abilities of dynamic and static balance in sitting, standing and center of gravity movement. The scale is consisted of 14 items, each of which has a score of 0–4, with a maximum score of 56 points. The higher the score, the better the balance ability of the patient (ICC = 0.92) (Godi et al., 2013). (3) 10-meter Walk Test (10MWT): 10MWT is a commonly used measure for assessing dynamic walking function, which evaluating the time for patients to walk 10 meters at a natural pace (ICC = 0.96–0.98) (Peters et al., 2013). (4) Timed Up-and-Go test (TUGT): TUGT is a widely used performance test for the evaluation of coordination and stability in dynamic walking. TUGT requires participants to stand up from a chair, walk 3 meters, turn around, return to the chair, and sit down again (ICC > 0.95) (Hafsteinsdóttir et al., 2014).
The secondary outcome indicators: (1) Functional ambulation category scale (FAC): FAC is adopted to assess the walking ability of stroke patients. The test results of the scale were divided into 6 grades. The higher the grade, the better the walking ability (ICC = 0.95) (Mehrholz et al., 2007). (2) Fugl-Meyer motor assessment of lower extremity (FMA-LE): FAM-LE was conducted to evaluate the motor ability of lower limbs, containing contents from five domains (motion, sensation, balance, joint range of movement and pain) as well as 17 assessment items, with a full score of 34 (Gladstone et al., 2002).
2.3. Literature screening and data extraction
Step 1: Import retrieved literature to the literature management software EndNote X9.1 Step 2: Exclude duplicate materials. Step 3: Perform the first round of screening by reading titles and abstracts. Step 4: After downloading full texts, conduct a second round of screening to determine if inclusion criteria were met.
Two independent reviewers, ZY and LZ, conducted the literature screening and data extraction. Then a cross-checking was performed. When a possible disagreement occurred, we solved it through discussion or negotiation with a third independent reviewer, HC. In literature screening, we first read the title to exclude irrelevant literature, and then, we further read the abstract and the full text to determine whether to include it. If necessary, we would contact the author of the original research by email or telephone to obtain the unconfirmed information.
The extracted data: (1) General information of the included literature: the title, the first author and the year of publication; (2) General characteristics of the patients: the number of cases in each group, the age and the duration of the disease; (3) Treatment specifics and the follow-up time; (4) Key elements of bias risk assessment; (5) Focused outcome indicators.
2.4. Quality assessment
Two independent reviewers used the Cochrane Collaboration tool to examine the risk of bias for the included studies (Higgins et al., 2011; Cumpston et al., 2019), and cross-checking was conducted. A literature quality grade was performed according to the Jadad Scale. A score of 1–3 was considered low quality, and a score of 4–7 was considered high quality. The grading was also conducted by two independent reviewers, with the disagreement consulting the opinions of a third independent reviewer.
2.5. Statistical analysis
The statistical analysis was based on RevMan5.4 (the Review Manager software 5.4, The Nordic Cochrane Center, The Cochrane Collaboration). If the results included in the literature were continuous variables, use the mean difference (MD) and 95% confidence interval (CI) for statistics. The p value and the I2 index were used as indicators to assess the heterogeneity among studies. There was no heterogeneity between studies when p ≥ 0.10, while p < 0.10 indicates that there was heterogeneity between studies. The I2 index represented the degree of heterogeneity between studies. If I2 < 50%, it indicates that there was slight heterogeneity between the studies, and the fixed effect model was used for analysis. If I2 ≥ 50%, there was heterogeneity in the study, and the random effect model was used for analysis (Cochrane et al., 2021). The α value was set at 0.05 and Stata 12.0 software was used to conduct the publication bias analysis and sensitivity analysis of Begg’s test for the studies with more than 5 included outcome indicators. The threshold for statistical significance was set at p < 0.05.
Considering differences in WBV training frequencies, a subgroup analysis was conducted. When the vibration frequency was set at 20–50 Hz or 20–45 Hz, higher EMG activity was induced (Rittweger, 2010; Alam et al., 2018). Thus, muscle strength was enhanced and muscle training was more effective and several studies (Cardinale and Lim, 2003; Rittweger et al., 2003; Liu et al., 2022) have found vibration frequencies between 20 and 30 Hz to be more effective in stroke patients. Therefore, we planned to divide into two subgroups by frequency of WBV training: a subgroup of vibration frequencies at 20–30 Hz, and another subgroup of the other vibration frequencies.
And the safety analysis was also conducted to confirm the safety of WBV training, through the observed changes in blood pressure and heart rate or some terrible symptoms such as headache and nausea in stroke patients during WBV training in the included studies.
3. Results
The initial search resulted in a total of 673 studies, and 8 studies were selected in other ways. EndNote X9 was used to remove duplicate documents, and there were 487 studies left. After reading the titles and abstracts, 122 studies were selected. Then, after reading the full texts, 98 studies were discarded because they did not meet the inclusion and exclusion criteria, and 22 studies were finally included (van Nes et al., 2006; Brogårdh et al., 2012; Chan et al., 2012; Guo et al., 2015; Choi et al., 2016, 2017; Gu and Hwangbo, 2016; Qianhao et al., 2018; Lee, 2019; Zhanyu et al., 2019; Zhen-hua et al., 2019; Sade et al., 2020; Xin-xin et al., 2020; Ijaz Ahmed Burq et al., 2021; Jin-Ming et al., 2021; Kim and Lee, 2021; Xie et al., 2021; Yan et al., 2021; Chuan et al., 2022; Le et al., 2022; Wei and Cai, 2022; Zhenying et al., 2022). The process is shown in Figure 1.
3.1. Characteristics of included studies
A total of 1,089 patients were included in the 22 studies. The age ranged from 31.8 to 78.31 years old. The sample size of each study ranged from 20 to 130 patients. 15 articles were published in the past five years, accounting for 68%. In most studies, the intervention in experimental groups was WVT combined with routine rehabilitation training. And in three studies, the experimental groups only used WVT intervention. In addition, the experimental groups in the other articles respectively added lower limb weight bearing training, basic walking training, extracorporeal shock wave therapy, music therapy, virtual reality technology, neuro-developmental treatment and treadmill training to routine rehabilitation training. For the control groups, in most studies, WVT was removed. However, there were five studies using the sham WVT intervention. The details of the research characteristics are shown in Table 1.
In 11 of the included studies, the walking spatiotemporal parament was evaluated (step length, step speed, cadence, single support time, double support time, stride length and step times). In 13 of the included studies, Berg Balance Scale (BBS) was used. And TUGT, 10MWT, FAC, and FMA-L were respectively used in 10, 7, 4 and 4 studies.
The risk of bias assessment was performed through RevMan5.4 software, according to the Cochrane Handbook for Systematic Reviews. The results are shown in Figures 2, 3. The quality of the literature was graded according to the Jadad scale, with two studies judged to be of low quality and the remaining studies considered to be of high quality. The details are presented in Supplementary material.
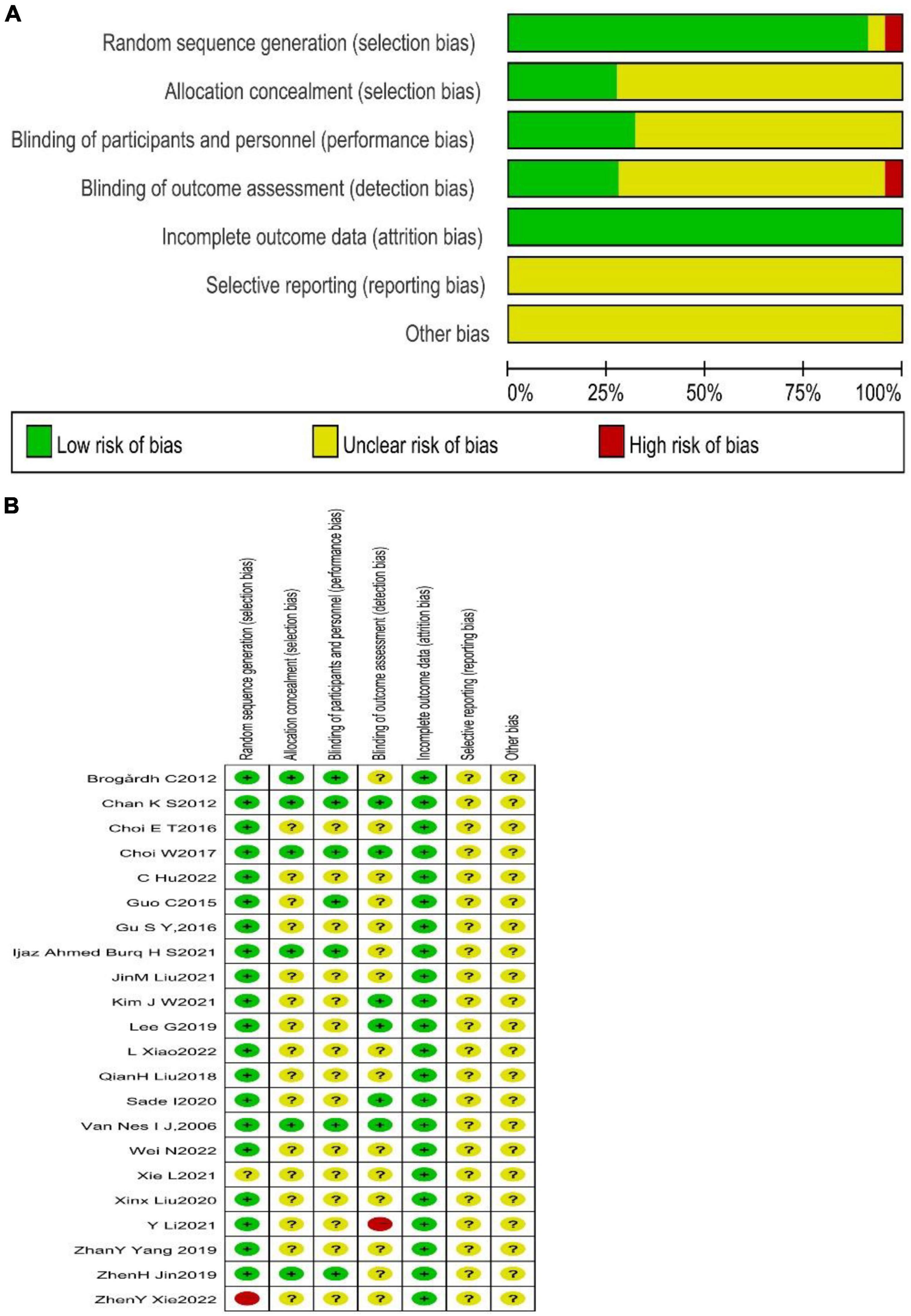
Figure 2. The risk assessment of bias by Cochrane. (A) This figure summarized the quality assessment of all included studies and presented an overall study quality assessment and risk of bias. Green means low risk of bias, red means high risk of bias, and yellow means ominous risk of bias. (B) This figure was presented separately for each included study and intuitively presented the quality assessment and risk of bias of each study. “+” Means low risk of bias, “–” means high risk of bias, and “?” means unknown risk of bias.
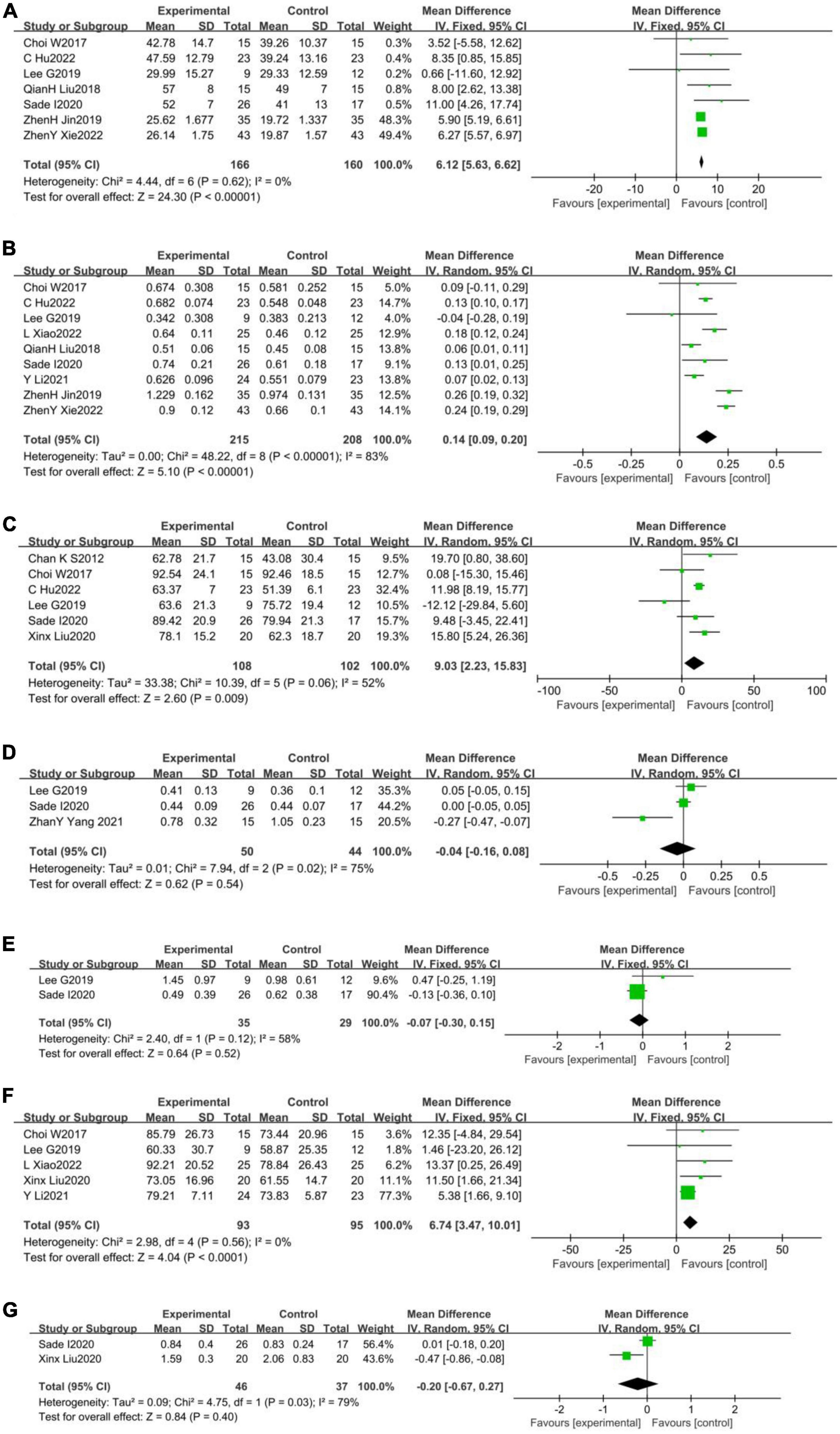
Figure 3. Forest plot of the effects of WBV training on walking spatiotemporal parament. (A) Step length (cm); (B) step speed (m/s); (C) cadence (step/min); (D) single support time(s); (E) double support time (s); (F) stride length (cm); and (G) step time (s).
3.2. Results of the meta-analysis
3.2.1. Walking spatiotemporal parament
A total of seven studies (Choi et al., 2017; Qianhao et al., 2018; Lee, 2019; Zhen-hua et al., 2019; Sade et al., 2020; Chuan et al., 2022; Zhenying et al., 2022) reported the effect of WBV training on step length in stroke patients, with non-heterogeneity among them (p = 0.62, I2 = 0%). A fixed effects model was used to analyze the data (MD = 6.12, 95%CI [5.63, 6.62], p < 0.001; Figure 3A). A total of nine studies (Choi et al., 2017; Qianhao et al., 2018; Lee, 2019; Zhen-hua et al., 2019; Sade et al., 2020; Yan et al., 2021; Chuan et al., 2022; Le et al., 2022; Zhenying et al., 2022) reported the effect of WBV training on step speed in stroke patients, with substantial heterogeneity among them (p < 0.001, I2 = 83%). A random effects model was used to analyze the data (MD = 0.14, 95%CI [0.09, 0.20], p < 0.001) (Figure 3B). A total of six studies (Chan et al., 2012; Choi et al., 2017; Lee, 2019; Sade et al., 2020; Xin-xin et al., 2020; Chuan et al., 2022)reported the effect of WBV training on the cadence in stroke patients, with substantial heterogeneity among them (p = 0.06, I2 = 52%). A random effects model was used to analyze the data (MD = 9.03, 95%CI [2.23, 15.83], p = 0.009) (Figure 3C). A total of three studies (Lee, 2019; Zhanyu et al., 2019; Sade et al., 2020) reported the effect of WBV training on the single support time in stroke patients, with substantial heterogeneity among them (p = 0.02, I2 = 75%). A random effects model was used to analyze the data (MD = −0.04, 95%CI [−0.16, 0.08], p = 0.54) (Figure 3D). There were only two studies (Lee, 2019; Sade et al., 2020) reported the effect of WBV training on the double support time in stroke patients, with substantial heterogeneity among them (p = 0.12, I2 = 58%). A random effects model was used to analyze the data (MD = −0.07, 95%CI [−0.30, 0.15], p = 0.52) (Figure 3E). A total of five studies (Choi et al., 2017; Lee, 2019; Xin-xin et al., 2020; Yan et al., 2021; Le et al., 2022) reported the effect of WBV training on the stride length in stroke patients, with non-heterogeneity among them (p = 0.56, I2 = 0%). A fixed effects model was used to analyze the data (MD = 6.74, 95%CI [−3.47, 10.01], p < 0.001) (Figure 3F). Finally, a total of two studies (Sade et al., 2020; Xin-xin et al., 2020)reported the effect of WBV training on the step time in stroke patients, with substantial heterogeneity among them (p = 0.03, I2 = 79%). A random effects model was used to analyze the data (MD = −0.20, 95%CI [−0.67, 0.27], p = 0.40) (Figure 3G).
3.2.2. Berg Balance Scale (BBS)
A total of 13 studies (van Nes et al., 2006; Brogårdh et al., 2012; Gu and Hwangbo, 2016; Qianhao et al., 2018; Sade et al., 2020; Xin-xin et al., 2020; Jin-Ming et al., 2021; Kim and Lee, 2021; Xie et al., 2021; Yan et al., 2021; Le et al., 2022; Wei and Cai, 2022; Zhenying et al., 2022)reported the effect of WBV training on BBS in stroke patients, with substantial heterogeneity among them (p < 0.001, I2 = 80%). A random effects model was used to analyze the data (MD = 4.08, 95%CI [2.39, 5.76], p < 0.001; Figure 4).
3.2.3. Timed Up-and-Go test (TUGT) (s)
A total of 10 studies (Chan et al., 2012; Choi et al., 2016; Gu and Hwangbo, 2016; Sade et al., 2020; Ijaz Ahmed Burq et al., 2021; Jin-Ming et al., 2021; Kim and Lee, 2021; Xie et al., 2021; Chuan et al., 2022; Zhenying et al., 2022) reported the effect of WBV training on TUGT in stroke patients, with substantial heterogeneity among them (p < 0.001, I2 = 78%). A random effects model was used to analyze the data (MD = −2.88, 95%CI [−4.94, −0.81], p = 0.006; Figure 5).
3.2.4. 10-meter Walk Test (10MWT) (s)
A total of seven studies (Chan et al., 2012; Guo et al., 2015; Zhanyu et al., 2019; Ijaz Ahmed Burq et al., 2021; Kim and Lee, 2021; Xie et al., 2021; Chuan et al., 2022) reported the effect of WBV on 10MWT in stroke patients, with no-heterogeneity among them (p = 0.21, I2 = 29%). A fixed effects model was used to analyze the data (MD = −2.69, 95%CI [−3.35, −2.03], p < 0.001; Figure 6).
3.2.5. Functional ambulation category scale (FAC)
A total of four studies (Zhen-hua et al., 2019; Jin-Ming et al., 2021; Kim and Lee, 2021; Zhenying et al., 2022) reported the effect of WBV training on FAC in stroke patients, with no heterogeneity among them (p = 0.93, I2 = 0%). A fixed effects model was used to analyze the data (MD = 0.78, 95%CI [0.65, 0.91], p < 0.001; Figure 7).
3.2.6. Fugl-Meyer motor assessment of lower extremity (FMA-LE)
A total of four studies (Guo et al., 2015; Zhanyu et al., 2019; Jin-Ming et al., 2021; Le et al., 2022) reported the effect of WBV training on FMA-LE in stroke patients, with substantial heterogeneity among them (p < 0.001, I2 = 86%). A random effects model was used to analyze the data (MD = 4.10, 95%CI [2.01, 6.20], p = 0.0001; Figure 8).
In summary, the results showed that the improvements in step length, step speed, cadence, stride length, BBS, TUGT, 10MWT, FAC and FAM-LE in the experimental groups were better than those in the controls, and the improvements in single support time, double support time and step time in the experimental groups were not obviously better than those in the controls.
3.3. Subgroup analysis
It had been suggested that the frequency of WBV training set from 20 to 30 Hz may be more beneficial in stroke patients (Cardinale and Lim, 2003; Rittweger et al., 2003; Liu et al., 2022). Thus, a subgroup analysis was conducted. The results showed that a 20–30 Hz vibration frequency was not conducive to the improvement of step length, BBS and FAC. However, in terms of the results of TUGT, the 20–30 Hz vibration frequency was better than other frequencies. The details are presented in Supplementary Figures 1–4.
3.4. Publication bias and sensitivity analysis
The publication bias analysis was conducted for the outcome indicators included in five or more studies through Begg’s test. The results showed step length (t = 0.44, P = 0.681, P > 0.05), step speed (t = −2.14, P = 0.07, P > 0.05), cadence (t = −1.11, P = 0.328, P > 0.05), BBS (t = 1.43, P = 0.180, P > 0.05), TUGT (t = 1.56, P = 0.157, P > 0.05) and 10MWT (t = 1.61, P = 0.151, P > 0.05), indicating no significant publication bias. The details are presented in Supplementary Figures 5–11.
A sensitivity analysis was conducted on the results of the meta-analysis with substantial heterogeneity and significant differences through one-by-one elimination. The results showed that there was no heterogeneity after excluding two studies (Zhen-hua et al., 2019; Zhenying et al., 2022) in the sensitivity analysis for the indicator of step length. The heterogeneity may be caused by the different measurement methods for the step length, with respectively using the footprint measurement method and gait analysis system in the two studies. In addition, in the sensitivity analysis for the indicators of step speed, cadence, stride length, BBS, TUGT and 10MWT, there was no obvious change when excluding any one of the studies. It indicated that the result was robust.
3.5. Safety analysis
Safety and adverse reactions were mentioned in three studies (Sade et al., 2020; Chuan et al., 2022; Wei and Cai, 2022). No adverse reactions occurred in the study. In general, the whole-body vibration training was safe.
4. Discussion
After a stroke, patients usually suffered from decreased muscle strength, abnormal muscle tension, limb coordination disorder, and sensory abnormality, caused by the injury of upper motor neurons (Zhen-hua et al., 2019), leading to the decline of gait stability, which will seriously affect the lower limb balance and walking function. For 85% of patients with stroke, the primary rehabilitation target is the recovery of walking function (Candelise et al., 2007), reducing the time required for patients to return to family and society, and improving their quality of life. Therefore, the main goal of the treatment is to improve gait stability and enhance the walking function of stroke patients.
WBV training has been widely used to promote the rehabilitation of stroke patients, and its effectiveness in the recovery of dysfunction in neurological diseases has been demonstrated to a certain degree (Alizadeh-Meghrazi et al., 2014; Kim and Lee, 2021; Tekin and Kavlak, 2021). However, previous studies may have been limited by insufficient literature searches and evaluation indicators. And a small part of the research showed that WBV had no positive effect on balance and gait improvement in stroke patients (Ijaz Ahmed Burq et al., 2021; Liu et al., 2022). Therefore, according to the disadvantage in the previous research, we added the search range of the database and evaluation indicators of walking spatiotemporal paraments to the analysis. And 22 randomized controlled trials (RCTs), which were called studies with the highest reliability and quality of research data (Barton, 2000), were finally included. In addition, adverse events were also considered because safety was an important component of treatments.
The decreased muscle strength in the lower limbs is a major cause of the unhealthy gait in stroke patients (Lau et al., 2011). The muscle strength in lower limbs was positively associated with spatiotemporal variations in stride length, stride time, stance time and double support time in old adults (Abdul Jabbar et al., 2021). The meta-analysis revealed that different changes in walking spatiotemporal parameters occurred after the intervention of WBV training for stroke patients. The improvement of step length, step speed, cadence and stride length was significant (p < 0.05). The impact stimulation produced in WBV training transfers to the whole body, stimulates proprioception receptors such as muscle spindles and tendon spindles, increases the excitability of sensory nerve fiber endings, and induces the stretch reflex in skeletal muscle. Therefore, the degree of muscle activation is increased, and the latent motor units are further activated. In this way, muscles recruit more motor units (Fallon and Macefield, 2007; Miyara et al., 2022). The improvement of muscle recruiting efficiency is beneficial to enhance the strength of muscles and improve sports performance (Sohrabzadeh et al., 2022). Several studies (Lau et al., 2011; Sitjà-Rabert et al., 2012; Alam et al., 2018) have shown that WBV training can enhance electromyography (EMG) signals in the lower limbs, improve muscle activation, and increase muscle strength, flexibility, burst force, and balance ability in the elderly. WBV training can also improve musculoskeletal health by increasing the production of fibronectin type III domain-containing protein 5 (FNDC5) and regulating the expression of key markers like myostatin, which has effects on both muscle and bone tissue (Cariati et al., 2022). Furthermore, the meta-analysis revealed that WBV training improved single support time, double support time, and step time, but not significantly (p > 0.05) when compared to controls. Stroke patients mostly suffer from damage to one cerebral hemisphere, which leads to dysfunction of the contralateral limb (Sacco and Rundek, 2012). Therefore, there was a large variation in the amplitude of the left-right movement of the body’s center of gravity during walking. To provide adequate stability, the unaffected side requires greater autonomic control (Song et al., 2018). Besides that, because of abnormal proprioception in stroke patients, the compensatory phenomenon occurs during body postural control, which then triggers symptoms such as abnormal gait posture (Dingwell and Cavanagh, 2001). Song S et al. found that an 8-week WBV training could improve the sensation, stability, and motility in the feet and ankles, with single support time and double support time decreasing (Song et al., 2018). This is inconsistent with the results of the meta-analysis. The different intervention cycles could be the cause of this occurrence. The WBV training intervention cycle was 3 or 4 weeks in the included literature in the meta-analysis.
The meta-analysis showed that WBV training had an obvious positive effect on balance, walking function and dynamic stability in stroke patients. The vibration could influence the activity rhythm of spinal anterior horn neurons, activate the cerebral motor cortex excitability, promote the remodeling of neural function in injured regions, and improve the postural control ability of patients with stroke (Mouchnino and Blouin, 2013; Lapole et al., 2015; Jammes et al., 2018). The vibration stimulus will induce the presynaptic inhibition of Ia afferents and/or a neurotransmitter depletion in presynaptic terminals. This may decrease the monosynaptic reflex excitability, reduce the abnormal spinal reflex excitation, restrain the stretch and H reflexes of the muscles, and regulate muscle spasm. Therefore, the balance and walking function of patients with stroke were improved (Miyara et al., 2014; Gu and Hwangbo, 2016). And studies (Kipp et al., 2011; Kim and Lee, 2021), showed that amplitudes of H reflexes of the soleus and gastrocnemius in the lower leg were significantly reduced after WBV training in patients with stroke. The main feature of WBV training, as a relatively passive exogenous stimulus, is effective training achieved with a smaller load intervention, which reduces the cardiopulmonary burden, compared with other rehabilitation training methods (Muir et al., 2013). WBV training has also been shown to increase oxygen consumption by itself in stroke patients and to promote the release of vasodilators without additional effects on heart rate or blood pressure (Zhang et al., 2022). And the vibration stimulation can promote osteoblast differentiation and subsequently osteogenesis and increase bone mass by activating the Wnt signaling pathway of bone marrow stromal cells (Yu-Han et al., 2013), thereby preventing and alleviating osteoporosis in the elderly (Cheng et al., 2021).
The subgroup analysis revealed that, after WBV training at 20–30 Hz, only the improvement of TUGT was better than at other frequencies, while that of step length, BBS and FAC was not. It has been found that, even for healthy individuals, early muscle fatigue is induced when the WBV vibration frequency exceeds 30 Hz. And for patients with stroke, a similar situation will happen when the WBV training frequency is set at equal to or less than 30 Hz with a 3 mm amplitude (van Nes et al., 2006; Tihanyi et al., 2007; Rittweger, 2010; Pujari et al., 2019). It has also been found that WBV training at a frequency of 20–45 Hz produces a positive muscle training effect (Rittweger, 2010). And when the WBV training frequency is set from 20 to 50 Hz, the variation of EMG signals is great, thereby enhancing muscle strength (Alam et al., 2018). However, at present, there is no guideline for recommended frequencies and amplitudes of WBV training for patients with stroke. It is suggested that the research should focus on this, to identify a suitable treatment strategy for stroke patients.
WBV training can induce the expression of brain-derived neurotrophic factor (BDNF) and FNDC5 in the cerebellum and hippocampus of the mouse to stimulate learning ability and cognitive memory (Cariati et al., 2021). And WBV training can improve brain health and cognitive function, as well as slow the problem of muscle wasting and motor decline associated with aging and/or a sedentary lifestyle (Cariati et al., 2022). Therefore, in future studies, it is possible to explore the effects of WBV training on cognitive impairment in stroke patients or those with other neurological injuries, and further explore the potential physiological and molecular mechanisms of WBV training.
5. Conclusion and suggestion
The meta-analysis revealed that whole-body vibration training has a positive effect on the balance and walking function of patients with stroke. Whole-body vibration training is also a safe treatment method for recovering from the walking dysfunction of patients with stroke.
Due to the fewer follow-ups in the included RCTs, the lack of long-term treatment effect observation and the differences in intervention protocols, the results of the included RCTs were insufficiently consistent. Thus, further studies for the conclusion of the meta-analysis are required. It is suggested that a more unified and standardized research design and intervention protocol should be established in future studies, while the potential mechanism of the impact of the intensity and physical parameters of WBV training on the efficacy of stroke patients should be further researched. And, to further verify the authenticity of the efficacy, objective instruments should be added to evaluate the indicator such as walking spatiotemporal parament.
6. Limitation of meta-analysis
1) The included studies were only in two languages: Chinese and English. And the sample size of the included studies was relatively small, which may have made the results biased.
2) Overall, the heterogeneity among some studies was substantial, which may have had effects on the reliability of the meta-analysis.
3) The vibration frequency, amplitude and time of the included studies were not sufficiently identical. And the intervention methods for the controls were also not identical enough. Therefore, these may influence the accuracy of the results of the meta-analysis.
4) The difference in the measurement method of the walking spatiotemporal parament may result in a measurement bias, thus affecting the analytical results.
Data availability statement
The original contributions presented in this study are included in the article/Supplementary material, further inquiries can be directed to the corresponding author.
Author contributions
YY and JS designed the systematic review and supervised the entire program. JW, HC, LZ, and XL reviewed all the studies and extracted the information from the eligible trials. YY and JW analyzed the data and prepared the figures and table. YY, JW, and ZY wrote the manuscript. YY, ZY, and JS revised the manuscript. All authors reviewed and approved the manuscript.
Funding
This work was supported by the National Key R&D project “Research on key technologies for the prevention and control of sports injuries in racing and confrontation winter events” (2019YFF0301704).
Conflict of interest
The authors declare that the research was conducted in the absence of any commercial or financial relationships that could be construed as a potential conflict of interest.
Publisher’s note
All claims expressed in this article are solely those of the authors and do not necessarily represent those of their affiliated organizations, or those of the publisher, the editors and the reviewers. Any product that may be evaluated in this article, or claim that may be made by its manufacturer, is not guaranteed or endorsed by the publisher.
Supplementary material
The Supplementary Material for this article can be found online at: https://www.frontiersin.org/articles/10.3389/fnhum.2022.1076665/full#supplementary-material
Footnotes
References
Abdul Jabbar, K., Seah, W. T., Lau, L. K., Pang, B. W., Ng, D. H., Tan, Q. L., et al. (2021). Fast gait spatiotemporal parameters in adults and association with muscle strength – The Yishun study. Gait Posture 85, 217–223. doi: 10.1016/j.gaitpost.2021.01.001
Alam, M. M., Khan, A. A., and Farooq, M. (2018). Effect of whole-body vibration on neuromuscular performance: A literature review. Work 59, 571–583. doi: 10.3233/wor-182699
Alizadeh-Meghrazi, M., Masani, K., Zariffa, J., Sayenko, D. G., Popovic, M. R., and Craven, B. C. (2014). Effect of whole-body vibration on lower-limb EMG activity in subjects with and without spinal cord injury. J. Spinal Cord Med. 37, 525–536. doi: 10.1179/2045772314y.0000000242
Barton, S. (2000). Which clinical studies provide the best evidence? The best RCT still trumps the best observational study. BMJ 321, 255–256. doi: 10.1136/bmj.321.7256.255
Brogårdh, C., Flansbjer, U. B., and Lexell, J. (2012). No specific effect of whole-body vibration training in chronic stroke: A double-blind randomized controlled study. Arch. Phys. Med. Rehabil. 93, 253–258. doi: 10.1016/j.apmr.2011.09.005
Calafiore, D., Negrini, F., Tottoli, N., Ferraro, F., Ozyemisci-Taskiran, O., and de Sire, A. (2022). Efficacy of robotic exoskeleton for gait rehabilitation in patients with subacute stroke: A systematic review. Eur. J. Phys. Rehabil. Med. 58, 1–8. doi: 10.23736/s1973-9087.21.06846-5
Candelise, L., Gattinoni, M., Bersano, A., Micieli, G., Sterzi, R., and Morabito, A. (2007). Stroke-unit care for acute stroke patients: An observational follow-up study. Lancet 369, 299–305. doi: 10.1016/s0140-6736(07)60152-4
Cardinale, M., and Lim, J. (2003). Electromyography activity of vastus lateralis muscle during whole-body vibrations of different frequencies. J. Strength Cond. Res. 17, 621–624. doi: 10.1519/1533-42872003017<0621:eaovlm<2.0.co;2
Cariati, I., Bonanni, R., Pallone, G., Annino, G., Tancredi, V., and D’Arcangelo, G. (2021). Modulation of synaptic plasticity by vibratory training in young and old mice. Brain Sci. 11:82. doi: 10.3390/brainsci11010082
Cariati, I., Bonanni, R., Pallone, G., Romagnoli, C., Rinaldi, A. M., Annino, G., et al. (2022). Whole body vibration improves brain and musculoskeletal health by modulating the expression of tissue-specific markers: FNDC5 as a key regulator of vibration adaptations. Int. J. Mol. Sci. 23:10388. doi: 10.3390/ijms231810388
Chan, K. S., Liu, C. W., Chen, T. W., Weng, M. C., Huang, M. H., and Chen, C. H. (2012). Effects of a single session of whole body vibration on ankle plantarflexion spasticity and gait performance in patients with chronic stroke: A randomized controlled trial. Clin. Rehabil. 26, 1087–1095. doi: 10.1177/0269215512446314
Cheng, L., Qian, L., Chang, S., and He, B. (2021). Effects of whole-body vibration training with the same amplitude and different frequencies on the proximal femoral bone density in elderly women. J. Sports Med. Phys. Fitness 61, 923–927. doi: 10.23736/s0022-4707.20.11514-7
Choi, E. T., Kim, Y. N., Cho, W. S., and Lee, D. K. (2016). The effects of visual control whole body vibration exercise on balance and gait function of stroke patients. J. Phys. Ther. Sci. 28, 3149–3152. doi: 10.1589/jpts.28.3149
Choi, W., Han, D., Kim, J., and Lee, S. (2017). Whole-body vibration combined with treadmill training improves walking performance in post-stroke patients: A randomized controlled trial. Med. Sci. Monit. 23, 4918–4925. doi: 10.12659/msm.904474
Chuan, H., Xiao, Y., Ying, G., Min, L., and Xin, W. (2022). Effect of whole body vibration training on walking function in patients with hemiplegia after stroke. J. Integr. Cardiovasc. Cerebrovasc. Dis. 20, 337–340.
Cigdem Karacay, B., Sahbaz, T., Gurtekin, B., Yildiz, S., and Ozcan, E. (2022). Effectiveness of whole-body vibration exercise and core stabilization exercise in chronic non-specific low back pain: A randomized-controlled study. Turk. J. Phys. Med. Rehabil. 68, 184–194. doi: 10.5606/tftrd.2022.7060
Cochrane, M., Mitchell, E., Hollingworth, W., Crawley, E., and Trépel, D. (2021). Cost-effectiveness of interventions for chronic fatigue syndrome or myalgic encephalomyelitis: A systematic review of economic evaluations. Appl. Health Econ. Health Policy 19, 473–486. doi: 10.1007/s40258-021-00635-7
Cumpston, M., Li, T., Page, M. J., Chandler, J., Welch, V. A., Higgins, J. P., et al. (2019). Updated guidance for trusted systematic reviews: A new edition of the cochrane handbook for systematic reviews of interventions. Cochrane Database Syst. Rev. 10:Ed000142. doi: 10.1002/14651858.Ed000142
Dingwell, J. B., and Cavanagh, P. R. (2001). Increased variability of continuous overground walking in neuropathic patients is only indirectly related to sensory loss. Gait Posture 14, 1–10. doi: 10.1016/s0966-6362(01)00101-1
Fallon, J. B., and Macefield, V. G. (2007). Vibration sensitivity of human muscle spindles and Golgi tendon organs. Muscle Nerve 36, 21–29. doi: 10.1002/mus.20796
Gladstone, D. J., Danells, C. J., and Black, S. E. (2002). The fugl-meyer assessment of motor recovery after stroke: A critical review of its measurement properties. Neurorehabil. Neural Repair. 16, 232–240. doi: 10.1177/154596802401105171
Godi, M., Franchignoni, F., Caligari, M., Giordano, A., Turcato, A. M., and Nardone, A. (2013). Comparison of reliability, validity, and responsiveness of the mini-BESTest and berg balance scale in patients with balance disorders. Phys. Ther. 93, 158–167. doi: 10.2522/ptj.20120171
Gu, S. Y., and Hwangbo, K. (2016). Effects of horizontal- and vertical-vibration exercises using a blade on the balance ability of patient with hemiplegic. J. Phys. Ther. Sci. 28, 896–899. doi: 10.1589/jpts.28.896
Guo, C., Mi, X., Liu, S., Yi, W., Gong, C., Zhu, L., et al. (2015). Whole body vibration training improves walking performance of stroke patients with knee hyperextension: A randomized controlled pilot Study. CNS Neurol. Disord. Drug Targets 14, 1110–1115. doi: 10.2174/1871527315666151111124937
Guzik, A., and Bushnell, C. (2017). Stroke epidemiology and risk factor management. Continuum (Minneap Minn) 23, 15–39. doi: 10.1212/con.0000000000000416
Hafsteinsdóttir, T. B., Rensink, M., and Schuurmans, M. (2014). Clinimetric properties of the timed up and go test for patients with stroke: A systematic review. Top. Stroke Rehabil. 21, 197–210. doi: 10.1310/tsr2103-197
Higgins, J. P., Altman, D. G., Gøtzsche, P. C., Jüni, P., Moher, D., Oxman, A. D., et al. (2011). The cochrane collaboration’s tool for assessing risk of bias in randomised trials. BMJ 343:d5928. doi: 10.1136/bmj.d5928
Ijaz Ahmed, Burq, H. S., Karimi, H., Ahmad, A., Gilani, S. A., and Hanif, A. (2021). The effects of whole body vibration on gait after chronic stroke: A randomized controlled clinical trial. J. Pak. Med. Assoc. 71, 2511–2514. doi: 10.47391/jpma.711
In, T., Jung, K., Lee, M. G., and Cho, H. Y. (2018). Whole-body vibration improves ankle spasticity, balance, and walking ability in individuals with incomplete cervical spinal cord injury. NeuroRehabilitation 42, 491–497. doi: 10.3233/nre-172333
Jaime, S. J., Maharaj, A., Alvarez-Alvarado, S., and Figueroa, A. (2019). Impact of low-intensity resistance and whole-body vibration training on aortic hemodynamics and vascular function in postmenopausal women. Hypertens. Res. 42, 1979–1988. doi: 10.1038/s41440-019-0328-1
Jammes, Y., Ferrand, E., Fraud, C., Boussuges, A., and Weber, J. P. (2018). Adding body load modifies the vibratory sensation of the foot sole and affects the postural control. Mil. Med. Res. 5:28. doi: 10.1186/s40779-018-0175-4
Jin-Ming, L., Guo-jun, W., Li-guo, Y., and Yan, M. (2021). Virtual reality technology combined with alternating vertical vibration training can improve the balance function and walking ability of stroke patients during the recovery period. Neural Inj. Funct. Reconstr. 16, 419–422. doi: 10.16780/j.cnki.sjssgncj.20210047
Khaku, A. S., and Tadi, P. (2022). “Cerebrovascular disease,” in StatPearls (Treasure Island, FL: StatPearls Publishing).
Kim, J. W., and Lee, J. H. (2021). Effect of whole-body vibration therapy on lower extremity function in subacute stroke patients. J. Exerc. Rehabil. 17, 158–163. doi: 10.12965/jer.2142246.123
Kipp, K., Johnson, S. T., Doeringer, J. R., and Hoffman, M. A. (2011). Spinal reflex excitability and homosynaptic depression after a bout of whole-body vibration. Muscle Nerve 43, 259–262. doi: 10.1002/mus.21844
Lapole, T., Temesi, J., Arnal, P. J., Gimenez, P., Petitjean, M., and Millet, G. Y. (2015). Modulation of soleus corticospinal excitability during Achilles tendon vibration. Exp. Brain Res. 233, 2655–2662. doi: 10.1007/s00221-015-4336-3
Lau, R. W., Liao, L. R., Yu, F., Teo, T., Chung, R. C., and Pang, M. Y. (2011). The effects of whole body vibration therapy on bone mineral density and leg muscle strength in older adults: A systematic review and meta-analysis. Clin. Rehabil. 25, 975–988. doi: 10.1177/0269215511405078
Le, X., Chao, L., Yuan, L., Yanyao, D., Bing, X., Fangbo, L., et al. (2022). Effects of whole body vibration combined with extracorporeal shock wave therapy on spasticity and balance gait parameters in hemiplegic patients with stroke. J. Cent. South Univ. (Med. Sci.) 47, 755–761.
Lee, G. (2019). Whole-body vibration in horizontal direction for stroke rehabilitation: A randomized controlled trial. Med. Sci. Monit. 25, 1621–1628. doi: 10.12659/msm.912589
Liu, Y., Fan, Y., and Chen, X. (2022). Effects of whole-body vibration training with different body positions and amplitudes on lower limb muscle activity in middle-aged and older women. Dose Response 20:15593258221112960. doi: 10.1177/15593258221112960
Lu, J., Xu, G., and Wang, Y. (2015). Effects of whole body vibration training on people with chronic stroke: A systematic review and meta-analysis. Top. Stroke Rehabil. 22, 161–168. doi: 10.1179/1074935714z.0000000005
Marín, P. J., García-Gutiérrez, M. T., Da Silva-Grigoletto, M. E., and Hazell, T. J. (2015). The addition of synchronous whole-body vibration to battling rope exercise increases skeletal muscle activity. J. Musculoskelet. Neuronal Interact. 15, 240–248.
Marin-Puyalto, J., Gomez-Cabello, A., Gonzalez-Aguero, A., Matute-Llorente, A., Gomez-Bruton, A., Jürimäe, J., et al. (2020). Effects of whole-body vibration training on bone density and turnover markers in adolescent swimmers. J. Pediatr. Endocrinol. Metab. 33, 623–630. doi: 10.1515/jpem-2019-0400
Mehrholz, J., Wagner, K., Rutte, K., Meissner, D., and Pohl, M. (2007). Predictive validity and responsiveness of the functional ambulation category in hemiparetic patients after stroke. Arch. Phys. Med. Rehabil. 88, 1314–1319. doi: 10.1016/j.apmr.2007.06.764
Miyara, K., Etoh, S., Kawamura, K., Maruyama, A., Kuronita, T., Ohwatashi, A., et al. (2022). Effects of lower limb segmental muscle vibration on primary motor cortex short-latency intracortical inhibition and spinal excitability in healthy humans. Exp. Brain Res. 240, 311–320. doi: 10.1007/s00221-021-06257-8
Miyara, K., Matsumoto, S., Uema, T., Hirokawa, T., Noma, T., Shimodozono, M., et al. (2014). Feasibility of using whole body vibration as a means for controlling spasticity in post-stroke patients: A pilot study. Complement. Ther. Clin. Pract. 20, 70–73. doi: 10.1016/j.ctcp.2013.10.002
Moher, D., Shamseer, L., Clarke, M., Ghersi, D., Liberati, A., Petticrew, M., et al. (2015). Preferred reporting items for systematic review and meta-analysis protocols (PRISMA-P) 2015 statement. Syst. Rev. 4:1. doi: 10.1186/2046-4053-4-1
Moreno-Segura, N., Martín-San Agustín, R., García-Bafalluy, S., and Escriche-Escuder, A. (2022). Effects of core training on trunk function, balance, and gait in stroke patients: A systematic review and meta-analysis of randomised controlled trials. Clin. Rehabil. 36, 1635–1654. doi: 10.1177/02692155221117220
Mouchnino, L., and Blouin, J. (2013). When standing on a moving support, cutaneous inputs provide sufficient information to plan the anticipatory postural adjustments for gait initiation. PLoS One 8:e55081. doi: 10.1371/journal.pone.0055081
Muir, J., Kiel, D. P., and Rubin, C. T. (2013). Safety and severity of accelerations delivered from whole body vibration exercise devices to standing adults. J. Sci. Med. Sport 16, 526–531. doi: 10.1016/j.jsams.2013.01.004
Nguyen, P. T., Chou, L. W., and Hsieh, Y. L. (2022). Proprioceptive neuromuscular facilitation-based physical therapy on the improvement of balance and gait in patients with chronic stroke: A systematic review and meta-analysis. Life (Basel) 12:882. doi: 10.3390/life12060882
Park, Y. J., Park, S. W., and Lee, H. S. (2018). Comparison of the effectiveness of whole body vibration in stroke patients: A meta-analysis. Biomed Res. Int. 2018:5083634. doi: 10.1155/2018/5083634
Peters, D. M., Fritz, S. L., and Krotish, D. E. (2013). Assessing the reliability and validity of a shorter walk test compared with the 10-Meter Walk Test for measurements of gait speed in healthy, older adults. J. Geriatr. Phys. Ther. 36, 24–30. doi: 10.1519/JPT.0b013e318248e20d
Pichler, K., Loreto, C., Leonardi, R., Reuber, T., Weinberg, A. M., and Musumeci, G. (2013). RANKL is downregulated in bone cells by physical activity (treadmill and vibration stimulation training) in rat with glucocorticoid-induced osteoporosis. Histol. Histopathol. 28, 1185–1196. doi: 10.14670/hh-28.1185
Pujari, A. N., Neilson, R. D., and Cardinale, M. (2019). Effects of different vibration frequencies, amplitudes and contraction levels on lower limb muscles during graded isometric contractions superimposed on whole body vibration stimulation. J. Rehabil. Assist. Technol. Eng. 6:2055668319827466. doi: 10.1177/2055668319827466
Qianhao, L., Daojian, H., Yingzi, L., Xiangyu, Z., Ganghua, G., Lingling, X., et al. (2018). Effects of iliopsoas tensile vibration training on the walking ability of stroke survivors. Chin. J. Phys. Med. Rehabil. 40, 491–494.
Rittweger, J. (2010). Vibration as an exercise modality: How it may work, and what its potential might be. Eur. J. Appl. Physiol. 108, 877–904. doi: 10.1007/s00421-009-1303-3
Rittweger, J., Mutschelknauss, M., and Felsenberg, D. (2003). Acute changes in neuromuscular excitability after exhaustive whole body vibration exercise as compared to exhaustion by squatting exercise. Clin. Physiol. Funct. Imaging 23, 81–86. doi: 10.1046/j.1475-097x.2003.00473.x
Sacco, R. L., and Rundek, T. (2012). Cerebrovascular disease. Curr. Opin. Neurol. 25, 1–4. doi: 10.1097/WCO.0b013e32834f89b1
Sade, I., Çekmece, Ç, Ýnanir, M., SelÇuk, B., Dursun, N., and Dursun, E. (2020). The effect of whole body vibration treatment on balance and gait in patients with stroke. Noro Psikiyatr. Ars. 57, 308–311. doi: 10.29399/npa.23380
Sierra-Guzmán, R., Jiménez-Diaz, F., Ramírez, C., Esteban, P., and Abián-Vicén, J. (2018). Whole-body-vibration training and balance in recreational athletes with chronic ankle instability. J. Athl. Train. 53, 355–363. doi: 10.4085/1062-6050-547-16
Sitjà-Rabert, M., Rigau, D., Fort Vanmeerghaeghe, A., Romero-Rodríguez, D., Bonastre Subirana, M., and Bonfill, X. (2012). Efficacy of whole body vibration exercise in older people: A systematic review. Disabil. Rehabil. 34, 883–893. doi: 10.3109/09638288.2011.626486
Sohrabzadeh, E., Kalantari, K. K., Naimi, S. S., Daryabor, A., and Akbari, N. J. (2022). The immediate effect of a single whole-body vibration session on balance, skin sensation, and pain in patients with type 2 diabetic neuropathy. J. Diabetes Metab. Disord. 21, 43–49. doi: 10.1007/s40200-021-00933-w
Song, S., Lee, K., Jung, S., Park, S., Cho, H., and Lee, G. (2018). Effect of horizontal whole-body vibration training on trunk and lower-extremity muscle tone and activation, balance, and gait in a child with cerebral palsy. Am. J. Case Rep. 19, 1292–1300. doi: 10.12659/ajcr.910468
Tekin, F., and Kavlak, E. (2021). Short and long-term effects of whole-body vibration on spasticity and motor performance in children with hemiparetic cerebral palsy. Percept. Mot. Skills 128, 1107–1129. doi: 10.1177/0031512521991095
Tihanyi, T. K., Horváth, M., Fazekas, G., Hortobágyi, T., and Tihanyi, J. (2007). One session of whole body vibration increases voluntary muscle strength transiently in patients with stroke. Clin. Rehabil. 21, 782–793. doi: 10.1177/0269215507077814
Van Criekinge, T., Truijen, S., Schröder, J., Maebe, Z., Blanckaert, K., van der Waal, C., et al. (2019). The effectiveness of trunk training on trunk control, sitting and standing balance and mobility post-stroke: A systematic review and meta-analysis. Clin. Rehabil. 33, 992–1002. doi: 10.1177/0269215519830159
van Nes, I. J., Latour, H., Schils, F., Meijer, R., van Kuijk, A., and Geurts, A. C. (2006). Long-term effects of 6-week whole-body vibration on balance recovery and activities of daily living in the postacute phase of stroke: A randomized, controlled trial. Stroke 37, 2331–2335. doi: 10.1161/01.STR.0000236494.62957.f3
Varvarousis, D. N., Martzivanou, C., Dimopoulos, D., Dimakopoulos, G., Vasileiadis, G. I., and Ploumis, A. (2021). The effectiveness of botulinum toxin on spasticity and gait of hemiplegic patients after stroke: A systematic review and meta-analysis. Toxicon 203, 74–84. doi: 10.1016/j.toxicon.2021.09.020
Virani, S. S., Alonso, A., Benjamin, E. J., Bittencourt, M. S., Callaway, C. W., Carson, A. P., et al. (2020). Heart disease and stroke statistics-2020 update: A report from the american heart association. Circulation 141, e139–e596. doi: 10.1161/cir.0000000000000757
Wang, M., Pei, Z. W., Xiong, B. D., Meng, X. M., Chen, X. L., and Liao, W. J. (2019). Use of Kinesio taping in lower-extremity rehabilitation of post-stroke patients: A systematic review and meta-analysis. Complement. Ther. Clin. Pract. 35, 22–32. doi: 10.1016/j.ctcp.2019.01.008
Wang, Z., Zhang, X., and Sun, M. (2022). The application of whole-body vibration training in knee osteoarthritis. Joint Bone Spine 89:105276. doi: 10.1016/j.jbspin.2021.105276
Wei, N., and Cai, M. (2022). Optimal frequency of whole body vibration training for improving balance and physical performance in the older people with chronic stroke: A randomized controlled trial. Clin. Rehabil. 36, 342–349. doi: 10.1177/02692155211050564
Wist, S., Clivaz, J., and Sattelmayer, M. (2016). Muscle strengthening for hemiparesis after stroke: A meta-analysis. Ann. Phys. Rehabil. Med. 59, 114–124. doi: 10.1016/j.rehab.2016.02.001
Xie, L., Yi, S. X., Peng, Q. F., Liu, P., and Jiang, H. (2021). Retrospective study of effect of whole-body vibration training on balance and walking function in stroke patients. World J. Clin. Cases 9, 6268–6277. doi: 10.12998/wjcc.v9.i22.6268
Xin-xin, L., Pan-de, Z., An-shi, D., and Zhen, L. (2020). Effects of whole-body vibration training on lower-limb muscle tension and walking ability in stroke patients with hemiplegia. Chin. J. Mod. Drug Appl. 14, 4–7. doi: 10.14164/j.cnki.cn11-5581/r.2020.19.002
Yan, L., Wenwen, H., Yanfei, D., Hui, L., Jianming, F., Yunhai, Y., et al. (2021). Effects of whole body vibration training on proprioception and balance function in stroke patients with hemiplegia. Chin. J. Rehabil. Med. 36, 709–712.
Yang, F., and Butler, A. J. (2020). Efficacy of controlled whole-body vibration training on improving fall risk factors in stroke survivors: A meta-analysis. Neurorehabil. Neural Repair. 34, 275–288. doi: 10.1177/1545968320907073
Yang, X., Wang, P., Liu, C., He, C., and Reinhardt, J. D. (2015). The effect of whole body vibration on balance, gait performance and mobility in people with stroke: A systematic review and meta-analysis. Clin. Rehabil. 29, 627–638. doi: 10.1177/0269215514552829
Yikun, Y., Jialin, W., and Junzhi, S. (2023). Therapeutic effect of different-frequency repetitive transcranial magnetic stimulations on post-stroke cognitive impairment: A Meta-analysis. Chin. J. Tissue Eng. Res. 27, 3274–3280.
Yu-Han, W., Shu-Min, B., and Jian-Hong, W. (2013). Regulating effects of whole-body vibration on protein expression of p-GSK3β in bone marrow cells of ovariectomized osteoporosis rats. Acta Physiol. Sin. 65, 165–170. doi: 10.13294/j.aps.2013.02.015
Zhang, M., Wei, J., and Wu, X. (2022). Effects of whole-body vibration training on lower limb motor function and neural plasticity in patients with stroke: Protocol for a randomised controlled clinical trial. BMJ Open 12:e060796. doi: 10.1136/bmjopen-2022-060796
Zhanyu, Y., Xuemei, Z., Qingyan, L., Minxian, W., Ben, H., Yanping, G., et al. (2019). Improvement of walking ability of stroke patients with knee joint training by whole body vibration therapy apparatus combined with elastic belt. Chin. J Brain Dis. Rehabil. (Electron. Ed.) 9, 96–100.
Zhen-hua, J., Ling, C., and Xiang-ming, Y. (2019). Effect of whole body vibration on abilities of lower extremities for stroke patients. Chin. J. Rehabil. Theory Pract. 25, 347–351.
Keywords: stroke, balance, walking function, whole-body vibration training, meta-analysis
Citation: Yin Y, Wang J, Yu Z, Zhou L, Liu X, Cai H and Sun J (2023) Does whole-body vibration training have a positive effect on balance and walking function in patients with stroke? A meta-analysis. Front. Hum. Neurosci. 16:1076665. doi: 10.3389/fnhum.2022.1076665
Received: 21 October 2022; Accepted: 12 December 2022;
Published: 04 January 2023.
Edited by:
Hideki Nakano, Kyoto Tachibana University, JapanReviewed by:
Danúbia Da Cunha De Sá Caputo, Rio de Janeiro State University, BrazilTianrong Chen, The University of Hong Kong, Hong Kong SAR, China
Copyright © 2023 Yin, Wang, Yu, Zhou, Liu, Cai and Sun. This is an open-access article distributed under the terms of the Creative Commons Attribution License (CC BY). The use, distribution or reproduction in other forums is permitted, provided the original author(s) and the copyright owner(s) are credited and that the original publication in this journal is cited, in accordance with accepted academic practice. No use, distribution or reproduction is permitted which does not comply with these terms.
*Correspondence: Junzhi Sun, ✉ anpoc3VuQGNkc3UuZWR1LmNu