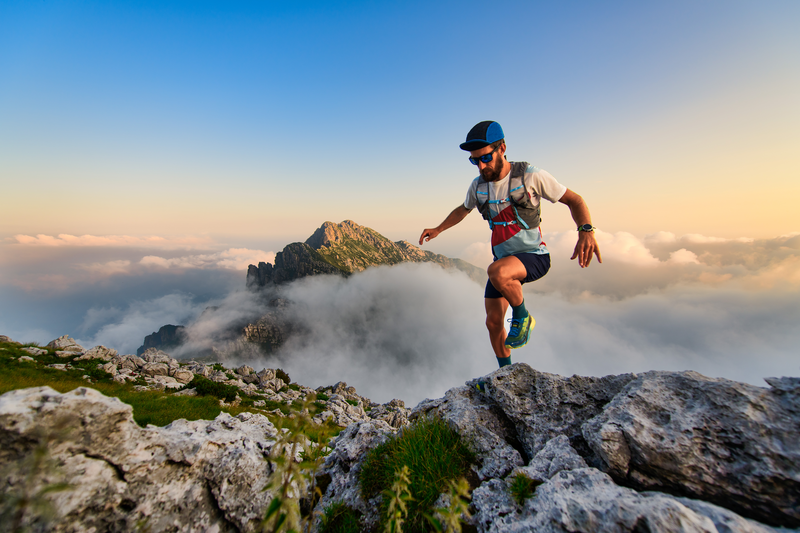
94% of researchers rate our articles as excellent or good
Learn more about the work of our research integrity team to safeguard the quality of each article we publish.
Find out more
HYPOTHESIS AND THEORY article
Front. Hum. Neurosci. , 17 November 2022
Sec. Cognitive Neuroscience
Volume 16 - 2022 | https://doi.org/10.3389/fnhum.2022.1032339
This article is part of the Research Topic Electromagnetic Field Theories of Consciousness: Opportunities and Obstacles View all 15 articles
Electromagnetic field (EMF) theories of mind/brain integration have been proposed to explain brain function for over seventy years. Interest in this theory continues to this day because it explains mind-brain integration and it offers a simple solution to the “binding problem” of our unified conscious experience. Thus, it addresses at least in part the “hard problem” of consciousness. EMFs are easily measured and many corelates have been noted for field activity; associated with loss and recovery of consciousness, sensory perceptions, and behavior. Unfortunately, the theory was challenged early on by experiments that were thought to have ruled out a role of EMFs in brain activity, and the field of neuroscience has since marginalized EMF theories. Here I explain why early evidence against EMFs contributing to consciousness was misinterpreted and offer an alternative view to help direct future research.
Electromagnetic field (EMF) theories of mind/brain integration posit that current flow across neuronal membranes generates an electromagnetic field which, in turn, permits computation and integration of information, that produces a conscious mind (Pockett, 2014; McFadden, 2020). Thus, consciousness arises from a dynamic electromagnetic field that reflects synaptic and discharge currents of neurons throughout the brain (Köhler and Held, 1949; Jones, 2013). The prevailing idea is that the EMF forms an aura-like three-dimensional energy cloud emanating from our brains, and extending beyond our skulls, where it can be recorded as EEG and/or MEG signals that exhibit complex patterns (Figure 1). I present a new way to visualize these complex patterns, using non-linear dynamic analyses of EEG recordings. 3-D plots of phase information derived from EEG signals nicely track levels of consciousness in humans and animals (MacIver and Bland, 2014; Eagleman and MacIver, 2021). These findings support EMF theories of consciousness, but provide only a crude measure of the complexity and integrating power of EMFs because they only measure the least powerful and most diffuse part of our conscious energy field.
Figure 1. A stylistic representation showing how brain neural circuitry (A,B) underlies the generation of EMFs (C) that unifies brain electrical activity into a conscious whole–that is our mind. Image was modified from freepik.com.
The standard view of brain/mind integration is illustrated in Figure 1, showing how neuronal circuit electrical activity in the brain produces a cloud of energy which radiates as our brain’s EMF. The idea being that synaptic and discharge currents in neurons (Figure 1A), especially large numbers of connected and synchronously and often rhythmically active neurons (Figure 1B) produce an EMF “cloud” of energy that changes moment by moment as underlying brain electrical activity changes. There is no doubt that this energy cloud exists since it is easily measured using both electrical and magnetic detectors (EEG and MEG) (Dyba et al., 2021; Keppler, 2021; Young et al., 2021, 2022; Hales and Ericson, 2022; Kitchener and Hales, 2022). From these measures we know EMFs radiate in 3 dimensions at varying powers and frequencies, represented in Figure 1C as differing colors on the right side of the image.
Electromagnetic fields are produced (generated) by neurons that are connected by chemical and/or electrical synapses, as well as via ephaptic connections. In Figure 1A, an ephaptic connection between two nearby neurons is shown by the synaptic current flow in apical dendrites of the first neuron (red arrows) inducing a depolarizing current flow in an adjacent neuron (blue arrows). Electrical synapses connect dendrites of adjacent neurons via gap junctions that are essential for the generation of EEG rhythms (Gołebiewski et al., 2006; Bocian et al., 2011). Larger groups of neurons are connected into circuits, mostly via chemical synapses, which are thought to underlie memory engrams and brain computational units (Figure 1B). When these circuits are active they produce synchronized synaptic and discharge activity across wide regions of the brain. This synchronized neuronal activity summates to generate the EMFs we record as EEG and MEG signals (Figure 1C). We know these fields extend for relatively long distances because they can be measured through at least the 7 mm of the human skull and scalp.
The strengths, supporting evidence, and utility of an EMF model have been well reviewed, and a number of proposed weaknesses were refuted by McFadden (2020) as well as in a scholarpedia article (Pockett, 2022). However, the earliest refutations of EMF theories have not been well addressed (Lashley et al., 1951; Sperry et al., 1955). A question remains, why do not EMF shields or perturbations of the EMF affect mental processes?
Placing gold leaf shields or other conductive materials (i.e., electrode arrays) on the brain’s surface, to short circuit (shunt) electric current flow, should disrupt or deteriorate an EMF such that an effect on mental processing would be apparent. Yet experiments testing this have failed to show altered mental processes (Lashley et al., 1951; Sperry et al., 1955; Endemann et al., 2022). Why not?
Figure 2 shows the electric current lines generated by just 3 synaptic current sink/sources located in three neocortical regions: frontal, midline and occipital areas. Of course, this is a very simplistic view of actual field generators which are active over wide ranging cortical and subcortical neuronal generators in an ever-changing pattern of complex current paths. The associated magnetic fields are not shown, but would be perpendicular to these current lines. Electrical and magnetic paths radiate in three dimensions, not just in the two dimensions shown in Figure 2.
Figure 2. Three current sources (e.g., synaptic ion channels) located in upper cortical layers of frontal, midline and occipital areas are shown, together with the charge paths they would produce (dotted lines with small arrows). Synaptic currents are usually carried by positive charges (Na+ and Ca++) that enter cells across dendritic membranes, so current lines show negative charge movement. These charge paths flow bidirectionally to produce fields above the cortex; these are what we measure with EEG surface electrodes. There are also charge paths directed inward, toward the thalamus and brainstem regions. Inward directed charge pathways would extend further because they are propagating through an electrolytic media that is not impeded by the dura and skull. I propose that these current paths would be concentrated toward the center of brains and generate much stronger EMFs compared to outward directed fields. Brain image modified from ProProfs.com.
Since synaptic current will take the path of least resistance through interstitial fluid and membranes of the brain, they will extend further into the brain, rather than outward, due to the increased resistance of our skull tissue. Measurements comparing deep electrode responses to surface electrode recordings consistently demonstrate similar or higher signal amplitudes (Hashimoto et al., 1981; Mishra et al., 2021). If the mind is “located” in these centralized overlapping EMFs, then it provides a stronger possibility of unification, “binding” very divergent brain activities into a central whole (Kitchener and Hales, 2022). We can also see (Figure 2) that outward fields would play only a small role in modifying neuronal activity through ephaptic influences on neurons. This is critical because we know that EMFs influence the discharge of neurons and this closes a loop for mind-brain duality by linking EMF energy back to controlling neuronal discharge (McFadden, 2013). The increased and focused density of inward directed EMFs would provide stronger ephaptic control of neurons, especially those in the brain’s central regions. Placing shields or introducing EMFs from external sources outside of the skull would hardly alter inward directed energies and would, hence, not appreciably alter our brain, mind or consciousness; this is what has been observed experimentally (Lashley et al., 1951; Sperry et al., 1955). Similarly, implanted deep brain stimulating electrodes appear to produce too localized a perturbation to alter the mind’s EMF, although effects on cognition do occur in some patients (Agashe et al., 2022; Chang et al., 2022). It has long been known that high strength magnetic fields of MRI scanners effect both human and animal subjects, producing dizziness, altered behavioral responses and cognitive impairment (Antunes et al., 2012; Tkáč et al., 2021). Weaker EMFs produced by cell phones, radios and headphones do not appreciably alter mental activity, although long-term exposure to these weak fields may disrupt some brain functions (Bodewein et al., 2022; Schüz et al., 2022). Transcranial magnetic stimulation using strong magnetic pulses are well known to stimulate brain neurons, as does ultrasonic stimulation (Sarasso et al., 2015; Sanguinetti et al., 2020). EMF perturbations on the surface of the brain or outside it or very close to the surface do not affect mental processes, whereas EM perturbations that penetrate deeply into the brain do affect mental process.
Non-linear (chaos) analysis of EEG signals provide a measure of our brain’s electric field, and also provide a sensitive measure of human consciousness (Watt and Hameroff, 1988; Walling and Hicks, 2006). We have recently explored a new way to visualize the chaotic complexity of brain activity from EEG signals; as chaotic attractor 3-D clouds which capture phase and complexity information and track the level or degree of consciousness in subjects slowly anesthetized, and then allowed to recover (Figure 3). When subjects are awake and conscious, 3-D clouds are largely spherical, reflecting many degrees of freedom and complex brain activity. As loss of consciousness (LOC) is produced, either through sleep, or in this case following anesthetic exposure, 3-D clouds begin to collapse into ellipsoid shapes. Deepening anesthesia, beyond loss of consciousness, results in further flattening of the clouds until cigar-like clouds are seen at deep surgical planes of anesthesia. Flattened clouds readily return to more spherical shapes upon recovery and awakening (Eagleman et al., 2019).
Figure 3. Chaotic attractors provide a sensitive measure of levels of consciousness in subjects exposed to an anesthetic. EEG signals were recorded from frontal cortex and processed as previously described (Eagleman et al., 2018b). Attractor plots produce spherical clouds in awake subjects. Loss of consciousness (LOC) is associated with a flattening of the attractor cloud. A further flattening is seen at surgical planes of anesthesia, with a return to a more spherical shape upon recovery, following removal of the anesthetic. Grids indicate best fit boundaries for each 3-D matrix. Unpublished data from Eagleman and MacIver.
What can these attractor clouds tell us about the EMF that is generated by our brains? They certainly provide a way to visualize the electric field component of the EMF since they are derived from EEG (i.e., electrical) signals, but they contain no magnetic information. Magnetic (MEG) signals have yet to be analyzed with this method. Attractor clouds are thought to reflect the complexity and information content of signals, with higher information being associated with more spherical plots. A more spherical plot indicates higher degrees of freedom in EEG signals, allowing the attractor to explore more regions of the complexity landscape. The anesthetic-induced collapse of the attractor certainly fits with an anesthetic-induced collapse of information integration that occurs at loss of consciousness (Oizumi et al., 2014; Sarasso et al., 2015; Tononi et al., 2016; Eagleman and MacIver, 2018, 2021; Eagleman et al., 2018a,b, 2019; Ward and Guevara, 2022). A more sophisticated approach would measure both electric and magnetic fields, together with photonic energies (Salari et al., 2021), and combine these into multi-dimensional attractors. Even better would be approaches which allow us to record EMFs from deeper regions of the brain, like the thalamus, midbrain and brainstem, together with cortical level signals. This would provide an enriched view of brain function and the distribution of EMFs throughout our higher nervous system regions.
Looking inward at EMF energy clouds, as opposed to the outward view most of us have envisioned, can readily account for why external fields and shielding do not alter mental processes. This view also supports the idea that EMFs focused into the brain would provide stronger ephaptic connections to the brain’s neural circuits. Providing a stronger coupling between energies of the brain and the mind, from quantum energies of photons and particles, to atoms, molecules, microtubules, synapses and circuits of cells; to energy fields and conscious thought; and back again (Hameroff and Penrose, 2014; McFadden, 2020; Hameroff, 2022).
The original contributions presented in this study are included in the article, further inquiries can be directed to the corresponding author.
MM researched and envisioned this manuscript in its entirety and wrote and revised the manuscript as well as compiled the illustrations.
This research was funded by the Department of Anesthesiology, Perioperative and Pain Medicine at Stanford University.
The author thanks Randy Gelb, Peter Watson, and Joyce Hellard for their comments and suggestions on an early draft of this manuscript. The Frontiers reviewers offered many valuable suggestions to improve this manuscript. This inward current EMF model was first presented at a consciousness meeting in Banff, Alberta (08/2022), that included Sir Roger Penrose, Stuart Hameroff, Jack Tuszynski, Catalina Curceanu, and Christoph Simon. The author also thanks all for their comments and insights.
The author declares that the research was conducted in the absence of any commercial or financial relationships that could be construed as a potential conflict of interest.
All claims expressed in this article are solely those of the authors and do not necessarily represent those of their affiliated organizations, or those of the publisher, the editors and the reviewers. Any product that may be evaluated in this article, or claim that may be made by its manufacturer, is not guaranteed or endorsed by the publisher.
Agashe, S., Burkholder, D., Starnes, K., Van Gompel, J. J., Lundstrom, B. N., Worrell, G. A., et al. (2022). Centromedian nucleus of the thalamus deep brain stimulation for genetic generalized epilepsy: a case report and review of literature. Front. Hum. Neurosci. 16:858413. doi: 10.3389/fnhum.2022.858413
Antunes, A., Glover, P. M., Li, Y., Mian, O. S., and Day, B. L. (2012). Magnetic field effects on the vestibular system: calculation of the pressure on the cupula due to ionic current-induced Lorentz force. Phys Med. Biol. 57, 4477–4487. doi: 10.1088/0031-9155/57/14/4477
Bocian, R., osluszny, A., Kowalczyk, T., Kazmierska, P., and Konopacki, J. (2011). Gap junction modulation of hippocampal formation theta and local cell discharges in anesthetized rats. Eur. J. Neurosci. 33, 471–481. doi: 10.1111/j.1460-9568.2010.07545.x
Bodewein, L., Dechent, D., Graefrath, D., Kraus, T., Krause, T., and Driessen, S. (2022). Systematic review of the physiological and health-related effects of radiofrequency electromagnetic field exposure from wireless communication devices on children and adolescents in experimental and epidemiological human studies. PLoS One 17:e0268641. doi: 10.1371/journal.pone.0268641
Chang, B., Ni, C., Zhang, W., Mei, J., Xiong, C., Chen, P., et al. (2022). Nomogram to predict cognitive state improvement after deep brain stimulation for Parkinson’s Disease. Brain Sci. 12:759. doi: 10.3390/brainsci12060759
Dyba, J., Rossi, K. L., Żurek, M., and Rossi, E. L. (2021). Electrodynamics of clinical hypnosis. Am. J Clin. Hypnosis 64, 110–122.
Eagleman, S., and MacIver, M. B. (2021). Molecular diversity of anesthetic actions is evident in electroencephalogram effects in humans and animals. Int. J. Mol. Sci. 22:E495. doi: 10.3390/ijms22020495
Eagleman, S. L., Chander, D., Reynolds, C., Ouellette, N. T., and MacIver, M. B. (2019). Nonlinear dynamics captures brain states at different levels of consciousness in patients anesthetized with propofol. PLoS One 14:e0223921. doi: 10.1371/journal.pone.0223921
Eagleman, S. L., Drover, C. M., Drover, D. R., Ouellette, N. T., and MacIver, M. B. (2018a). Remifentanil and nitrous oxide anesthesia produces a unique pattern of EEG activity during loss and recovery of response. Front. Hum. Neurosci. 12:173. doi: 10.3389/fnhum.2018.00173
Eagleman, S. L., and MacIver, M. B. (2018). Can you hear me now? Information processing in primary auditory cortex at loss of consciousness. Br. J. Anaesth. 121, 526–529. doi: 10.1016/j.bja.2018.06.008
Eagleman, S. L., Vaughn, D. A., Drover, D. R., Drover, C. M., Cohen, M. S., Ouellette, N. T., et al. (2018b). Do complexity measures of frontal EEG distinguish loss of consciousness in geriatric patients under anesthesia? Front. Neurosci. 12:645. doi: 10.3389/fnins.2018.00645
Endemann, C. M., Krause, B. M., Nourski, K. V., Banks, M. I., and Veen, B. V. (2022). Multivariate autoregressive model estimation for high-dimensional intracranial electrophysiological data. NeuroImage 254:119057. doi: 10.1016/j.neuroimage.2022.119057
Gołebiewski, H., Eckersdorf, B., and Konopacki, J. (2006). Electrical coupling underlies theta rhythm in freely moving cats. Eur. J. Neurosci. 24, 1759–1770. doi: 10.1111/j.1460-9568.2006.04993.x
Hales, C. G., and Ericson, M. (2022). Electromagnetism’s bridge across the explanatory gap: how a neuroscience/physics collaboration delivers explanation into all theories of consciousness. Front. Hum. Neurosci. 16:836046. doi: 10.3389/fnhum.2022.836046
Hameroff, S. (2022). Consciousness, cognition and the neuronal cytoskeleton - a new paradigm needed in neuroscience. Front. Mol. Neurosci. 15:869935. doi: 10.3389/fnmol.2022.869935
Hameroff, S., and Penrose, R. (2014). Consciousness in the universe: a review of the “Orch OR” theory. Phys. Life Rev. 11, 39–78. doi: 10.1016/j.plrev.2013.08.002
Hashimoto, I., Ishiyama, Y., Yoshimoto, T., and Nemoto, S. (1981). Brain-stem auditory-evoked potentials recorded directly from human brain-stem and thalamus. Brain 104(Pt 4), 841–859. doi: 10.1093/brain/104.4.841
Keppler, J. (2021). Building blocks for the development of a self-consistent electromagnetic field theory of consciousness. Front. Hum. Neurosci. 15:723415. doi: 10.3389/fnhum.2021.723415
Kitchener, D., and Hales, C. G. (2022). What neuroscientists think, and don’t think, about consciousness. Front. Hum. Neurosci. 16:767612. doi: 10.3389/fnhum.2022.767612
Köhler, W., and Held, R. (1949). The cortical correlate of pattern vision. Science 110, 414–419. doi: 10.1126/science.110.2860.414
Lashley, K. S., Chow, K. L., and Semmes, J. (1951). An examination of the electrical field theory of cerebral integration. Psychol. Rev. 58, 123–136. doi: 10.1037/h0056603
MacIver, M. B., and Bland, B. H. (2014). Chaos analysis of EEG during isoflurane-induced loss of righting in rats. Front. Syst. Neurosci. 8:203. doi: 10.3389/fnsys.2014.00203
McFadden, J. (2020). Integrating information in the brain’s EM field: the cemi field theory of consciousness. Neurosci. Conscious. 2020:niaa016. doi: 10.1093/nc/niaa016
Mishra, A., Marzban, N., Cohen, M., and Englitz, B. (2021). Dynamics of neural microstates in the VTA-striatal-prefrontal loop during novelty exploration in the rat. J. Neurosci. 41, 6864–6877. doi: 10.1523/JNEUROSCI.2256-20.2021
Oizumi, M., Albantakis, L., and Tononi, G. (2014). From the phenomenology to the mechanisms of consciousness: integrated information theory 3.0. PLoS Computat. Biol. 10:e1003588. doi: 10.1371/journal.pcbi.1003588
Pockett, S. (2014). Problems with theories that equate consciousness with information or information processing. Front. Syst. Neurosci. 8:225. doi: 10.3389/fnsys.2014.00225
Salari, V., Rodrigues, S., Saglamyurek, E., Simon, C., and Oblak, D. (2021). Are brain-computer interfaces feasible with integrated photonic chips? Front. Neurosci. 15:780344. doi: 10.3389/fnins.2021.780344
Sanguinetti, J. L., Hameroff, S., Smith, E. E., Sato, T., Tyler, W. J., Allen, J. B., et al. (2020). Transcranial focused ultrasound to the right prefrontal cortex improves mood and alters functional connectivity in humans. Front. Hum. Neurosci. 14:52. doi: 10.3389/fnhum.2020.00052
Sarasso, S., Boly, M., Napolitani, M., Gosseries, O., Charland-Verville, V., Casarotto, S., et al. (2015). Consciousness and complexity during unresponsiveness induced by propofol, xenon, and ketamine. Curr. Biol. 25, 3099–3105. doi: 10.1016/j.cub.2015.10.014
Schüz, J., irie, K., Reeves, G. K., Floud, S., and Beral, V. (2022). Cellular telephone use and the risk of brain tumors: update of the UK million women study. J. Natl. Cancer Inst. 114, 704–711. doi: 10.1093/jnci/djac042
Sperry, R. W., Miner, N., and Myers, R. E. (1955). Visual pattern perception following sub-pial slicing and tantalum wire implantations in the visual cortex. J. Comp. Physiol. Psychol. 48, 50–58. doi: 10.1037/h0043456
Tkáč, I., Benneyworth, M. A., Nichols-Meade, T., Steuer, E. L., Larson, S. N., Metzger, G. J., et al. (2021). Long-term behavioral effects observed in mice chronically exposed to static ultra-high magnetic fields. Magn. Reson. Med. 86, 1544–1559. doi: 10.1002/mrm.28799
Tononi, G., Boly, M., Massimini, M., and Koch, C. (2016). Integrated information theory: from consciousness to its physical substrate. Nat. Rev. Neurosci. 17, 450–461. doi: 10.1038/nrn.2016.44
Walling, T., and Hicks, K. N. (2006). Nonlinear changes in brain dynamics during emergence from sevoflurane anesthesia: preliminary exploration using new software. Anesthesiology 105, 927–935. doi: 10.1097/00000542-200611000-00013
Ward, L. M., and Guevara, R. (2022). Qualia and phenomenal consciousness arise from the information structure of an electromagnetic field in the brain. Front. Hum. Neurosci. 16:874241. doi: 10.3389/fnhum.2022.874241
Watt, R. C., and Hameroff, S. R. (1988). Phase space electroencephalography (EEG): a new mode of intraoperative EEG analysis. Int. J. Clin. Monitor. Comput. 5, 3–13. doi: 10.1007/BF01739226
Young, A., Hunt, T., and Ericson, M. (2021). The slowest shared resonance: a review of electromagnetic field oscillations between central and peripheral nervous systems. Front. Hum. Neurosci. 15:796455. doi: 10.3389/fnhum.2021.796455
Keywords: mind, electrodynamic, chaos, ephaptic, quantum fields
Citation: MacIver MB (2022) Consciousness and inward electromagnetic field interactions. Front. Hum. Neurosci. 16:1032339. doi: 10.3389/fnhum.2022.1032339
Received: 30 August 2022; Accepted: 12 October 2022;
Published: 17 November 2022.
Edited by:
Tam Hunt, University of California, Santa Barbara, United StatesReviewed by:
Ramon Guevara Erra, UMR8242 Laboratoire Psychologie de la Perception (LPP), FranceCopyright © 2022 MacIver. This is an open-access article distributed under the terms of the Creative Commons Attribution License (CC BY). The use, distribution or reproduction in other forums is permitted, provided the original author(s) and the copyright owner(s) are credited and that the original publication in this journal is cited, in accordance with accepted academic practice. No use, distribution or reproduction is permitted which does not comply with these terms.
*Correspondence: M. Bruce MacIver, bWFjaXZlckBzdGFuZm9yZC5lZHU=
Disclaimer: All claims expressed in this article are solely those of the authors and do not necessarily represent those of their affiliated organizations, or those of the publisher, the editors and the reviewers. Any product that may be evaluated in this article or claim that may be made by its manufacturer is not guaranteed or endorsed by the publisher.
Research integrity at Frontiers
Learn more about the work of our research integrity team to safeguard the quality of each article we publish.