- 1Department of Health Management and Policy, University of New Hampshire, Durham, NH, United States
- 2Department of Psychology, University of Texas at Austin, Austin, TX, United States
- 3Department of Communication Sciences and Disorders, University of New Hampshire, Durham, NH, United States
- 4Wentworth Health Partners Seacoast Physiatry, Somersworth, NH, United States
Over 100 million Americans suffer from chronic pain (CP), which causes more disability than any other medical condition in the United States at a cost of $560–$635 billion per year (Institute of Medicine, 2011). Opioid analgesics are frequently used to treat CP. However, long term use of opioids can cause brain changes such as opioid-induced hyperalgesia that, over time, increase pain sensation. Also, opioids fail to treat complex psychological factors that worsen pain-related disability, including beliefs about and emotional responses to pain. Cognitive behavioral therapy (CBT) can be efficacious for CP. However, CBT generally does not focus on important factors needed for long-term functional improvement, including attainment of personal goals and the psychological flexibility to choose responses to pain. Acceptance and Commitment Therapy (ACT) has been recognized as an effective, non-pharmacologic treatment for a variety of CP conditions (Gutierrez et al., 2004). However, little is known about the neurologic mechanisms underlying ACT. We conducted an ACT intervention in women (n = 9) with chronic musculoskeletal pain. Functional magnetic resonance imaging (fMRI) data were collected pre- and post-ACT, and changes in functional connectivity (FC) were measured using Network-Based Statistics (NBS). Behavioral outcomes were measured using validated assessments such as the Acceptance and Action Questionnaire (AAQ-II), the Chronic Pain Acceptance Questionnaire (CPAQ), the Center for Epidemiologic Studies Depression Scale (CES-D), and the NIH Toolbox Neuro-QoLTM (Quality of Life in Neurological Disorders) scales. Results suggest that, following the 4-week ACT intervention, participants exhibited reductions in brain activation within and between key networks including self-reflection (default mode, DMN), emotion (salience, SN), and cognitive control (frontal parietal, FPN). These changes in connectivity strength were correlated with changes in behavioral outcomes including decreased depression and pain interference, and increased participation in social roles. This study is one of the first to demonstrate that improved function across the DMN, SN, and FPN may drive the positive outcomes associated with ACT. This study contributes to the emerging evidence supporting the use of neurophysiological indices to characterize treatment effects of alternative and complementary mind-body therapies.
Introduction
Over 100 million Americans suffer from chronic pain (CP), which causes more disability than any other medical condition in the United States at a cost of $560–$635 billion per year (Institute of Medicine, 2011). Opioid analgesics are frequently used to treat CP. However, long term use of opioids can cause brain changes such as opioid-induced hyperalgesia that, over time, increase pain sensation. Also, opioids fail to treat complex psychological factors that worsen pain-related disability, including beliefs and emotional responses to pain. Cognitive behavioral therapy (CBT) can be efficacious for CP (Lim et al., 2018). However, CBT does not focus on important factors needed for long-term functional improvement, including attainment of personal goals and the psychological flexibility to choose responses to pain (Wetherell et al., 2011).
Acceptance and Commitment Therapy (ACT) is a mindfulness-based therapy that focuses on enabling individuals to accept what is out of their control, and to commit to valued actions that enrich their lives (Vowles and McCracken, 2008). ACT was developed in 1986 by Stephen C. Hayes who began to examine how language and thought influence internal experiences (Harris, 2006). By emphasizing acceptance instead of avoidance, ACT differs from many other forms of CBT. Although not originally designed for CP, ACT has been shown to be efficacious in terms of clinical outcomes, adherence to treatment, and retention, earning the status of a “well-established” treatment for CP from the American Psychological Association. ACT aims to increase psychological flexibility, and has been associated with improved health outcomes in many randomized controlled clinical trials (Feliu-Soler et al., 2018), including three systematic reviews specific to CP (Hann and McCracken, 2014; Veehof et al., 2016; Hughes et al., 2017). Psychological flexibility is defined as an individual’s ability to “recognize and adapt to various situational demands; shift mindsets or behavioral repertoires when these strategies compromise personal or social functioning; maintain balance among important life domains; and be aware, open, and committed to behaviors that are congruent with deeply held values” (Kashdan and Rottenberg, 2010, p. 865). ACT is a “third wave” behavioral treatment that has been shown to be efficacious for treating CP, as well as co-morbid conditions and factors (e.g., goal selection) related to long-term functional improvement (Vowles and McCracken, 2008; Yu et al., 2017). Additionally, patients who participate in ACT report greater long-term satisfaction compared to CBT (Wetherell et al., 2011). ACT is transdiagnostic and associated with improvements in physical functioning and pain-related disability, as well as decreases in emotional distress regardless of perceived pain intensity (Hann and McCracken, 2014).
Resting-state functional magnetic resonance imaging (rsfMRI) allows for data to be collected while individuals with CP rest in the MRI scanner for a short period of time (<10 min). Thus, data provides information about the natural state of brain function in CP without having to apply any external sensory or cognitive stimulation. Analysis methods of rsfMRI have focused on multiple regions in the brain, targeting inherent and altered measures of connectivity between brain regions and within brain networks (Fox et al., 2005). Further, alterations in brain structure and function have been demonstrated in multiple CP syndromes (Jensen et al., 2013; Smallwood et al., 2013, 2019; Reddan and Wager, 2018). Prior imaging research has suggested that CP results in abnormal hyper-connectivity of brain networks associated with self-reflection (default mode, DMN), emotion (salience, SN), and cognitive control (frontal parietal, FPN) networks (Napadow et al., 2010; Hemington et al., 2016; van Ettinger-Veenstra et al., 2019). While ACT has been successful in helping those with CP create a more functional and personally meaningful life (Vowles et al., 2009), a critical gap in our understanding of the neural mechanisms underlying ACT remains.
Only two prior investigations have used fMRI to assess neural mechanisms of ACT-based interventions for CP. Jensen et al. (2012) investigated task fMRI activation using pressure evoked pain. Participants with fibromyalgia showed increased activation in the ventrolateral prefrontal cortex (vlPFC) and orbitofrontal cortex (OFC) post-ACT after 12 weeks of ACT. Additionally, results showed pain-evoked changes in connectivity between the vlPFC and thalamus after ACT. Smallwood et al. (2016) conducted an 8-week ACT intervention vs. health education control (HEC) for participants with comorbid CP and opioid addiction. Focusing on DMN and pain regions in the brain, participants receiving ACT exhibited decreased activation during evoked pain in the middle frontal gyrus (MFG), inferior parietal lobule (IPL), insula, anterior cingulate cortex (aCC), posterior cingulate cortex (pCC), and superior temporal gyrus (STG) compared with HEC participants.
In the present study, ACT was delivered to nine women with CP using a quasi-experimental (pre–post) design. fMRI was used to identify changes in brain networks underlying ACT-related behavioral outcomes in CP. Based on our prior work examining ACT in CP (Smallwood et al., 2016, 2019), we hypothesize that: (1) ACT will reduce connectivity strength within and between the DMN, SN, and FPN, and that (2) changes in connectivity strength will correlate with changes in behavioral outcomes from pre-to post-ACT.
Materials and Methods
Participants
Nine female participants (47.59 ± 16.54 years, 8 right:1 left-handed) with musculoskeletal pain who did not self-report misusing opioids were enrolled in a 4-week group ACT intervention program (Table 1). Participants were referred from the outpatient practice of a physician certified in Physical Medicine and Rehabilitation with subspecialty certification in Pain Medicine. The practice involves rehabilitation and management of both acute pain and CMP, with a higher prevalence of females versus male patients reporting CMP (Meier et al., 2021).
Participants were required to be at least 18 years of age, speak English, have been living with musculoskeletal CP for 3 or more months, have a Brief Pain Inventory (BPI) Score of ≥4, and have no history of cancer or malignancy, head or severe body trauma in the past 6 months. Participants with neurologic (e.g., history of stroke, brain lesions, or intracranial surgery) or psychiatric disorders not commonly comorbid with CP were excluded. Patients who were not addicted to opioids but were taking opiates on a PRN (“as needed”) basis were eligible to participate, in order to reflect real-world clinic conditions as closely as possible. Only one participant self-reported using PRN opioid medication. Most participants reported having more than one type of chronic pain. Specifically, four patients reported suffering from fibromyalgia, one reported Ehlers–Danlos syndrome, one reported Conradi–Hünermann syndrome, two reported chronic neck pain, three reported chronic lower back pain, and one reported trigeminal neuralgia (for further details regarding the participants’ medical histories, please see Supplementary Table 4).
Acceptance Commitment Therapy Protocol
Patients completed two 90-min manualized ACT sessions per week for 4 weeks (Luoma et al., 2007; Potter, 2012). ACT sessions were administered by two licensed, trained Certified Therapeutic Recreation Specialists (CTRS/L). Behavioral outcomes were measured using validated assessments including the Acceptance and Action Questionnaire (AAQ-II) (Bond et al., 2011), the Center for Epidemiologic Studies Scale (CES-D) (Radloff, 1977; Vilagut et al., 2016), the Chronic Pain Acceptance Questionnaire (CPAQ) (McCracken et al., 2005; McCracken and Vowles, 2006; Vowles et al., 2008; Vowles and Thompson, 2011), the NIH Toolbox Neuro-QoLTM (Quality of Life in Neurological Disorders) scales (Cella et al., 2012), and the NIH Patient-Reported Outcome Measurement Information System (PROMIS) measures of pain interference (Amtmann et al., 2010, 2011), administered using an iPad (see Supplementary Material). The study protocol (#6991) was approved by the University of New Hampshire Institutional Review Board on July 19, 2018. All participants provided informed consent.
The behavioral assessment data were entered into Excel spreadsheets using Qualtrics software (Qualtrics, 2005) for data management. Statistical analyses were conducted using SAS® v.9.4. (SAS Institute Inc., 2019). Paired Student’s t-tests and Wilcoxon signed rank tests were used to assess differences in behavioral measures from pre-to-post ACT (subtracting post minus pre scores). Positive or negative change scores indicated satisfactory results, depending on the specific test in question (e.g., reduced CES-D scores indicated improvements in depression while higher AAQ scores indicated improvements in pain acceptance).
Resting State fMRI Data Collection
MRI data were collected before and immediately after 4 weeks of ACT using a Siemens Three Tesla Magnetom Prisma scanner at Boston University, Boston, MA, United States. Structural MPRAGE was collected (TR/TE = 2.53 s/1.32 ms, flip angle = 7°, field of view (FOV) = 256 × 320 mm, 0.8 mm3 resolution) to allow for anatomical registration. Subsequently, two runs of 8 min resting-state fMRI data were obtained using a T2∗ weighted Echo Planar Imaging sequence (2.5 mm3 resolution, 60 slices, TR/TE = 1.2 s/30 ms, 300 volumes, FOV = 205 mm, multi-slice interleaved ascending) for all participants. During the resting state scans, participants were instructed to lie still in the scanner with their eyes open, fixating on a crosshair placed in their field of view. Only the first of the two resting state scans were used for analysis. Two rsfMRI scans were collected in the case that one set was unusable (e.g., movement artifact). The first scan set was of high enough quality to use.
Resting State fMRI Data Analysis
Standard preprocessing steps were carried out using Statistical Parametric Mapping, version 12 (SPM12, Penny et al., 2006). First, all scan data were imported in the form of DICOM images and converted to Nifti files using the DICOM Import function in SPM12. Functional data were realigned and co-registered to the standard Montreal Neurological Institute (MNI) template in SPM12. Motion correction, band-pass filtering (0.0078–0.08 Hz), slice-timing correction, normalization to remove individual variability for between subject comparisons, and smoothing to increase signal to noise ratio were carried out using SPM12 (Figure 1, step 1). Next, each participant’s brain was parcellated into discrete regions of interest representing nodes from the Power atlas (Power et al., 2011) using Mango (Multi-image Analysis GUI; Lancaster et al., 2010). The mean time course within seed regions were extracted from the residual images using REX (Duff, 2008) (Figure 1, step 2). FC estimates were then calculated using the pairwise Pearson correlation of seed regions located time course across all 264 nodes resulting with a 264 by 264 connectivity matrix (Figure 1, step 3). Finally, connectivity matrices were reduced to a subset of 101 nodes that were associated with the FPN, DMN, and SN.
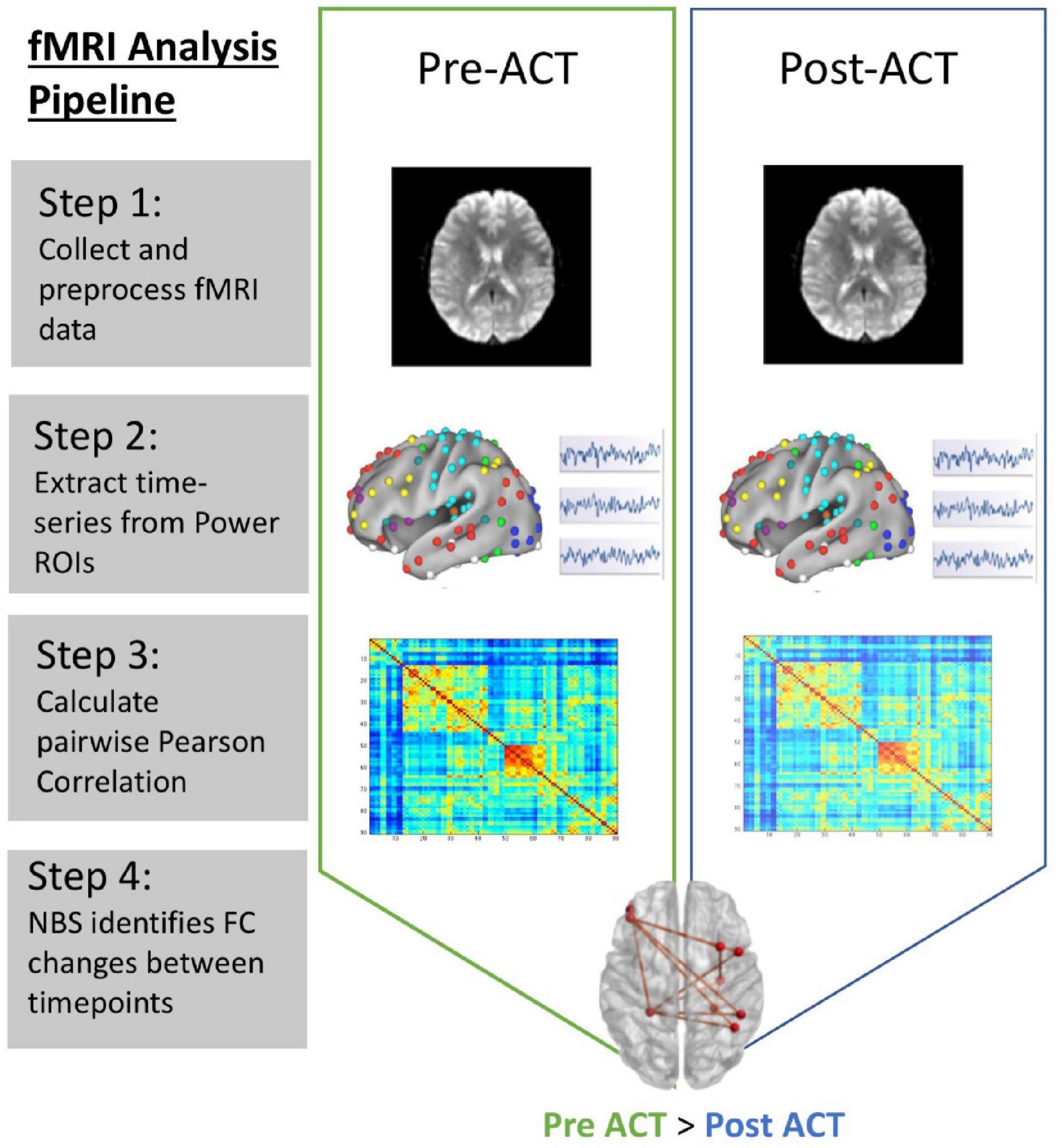
Figure 1. fMRI data analysis pipeline. Step 1: rsfMRI data preprocessed using SPM12; Step 2: Mean time series extraction from Power ROIs; Step 3: Estimate FC between all nodes using Pearson correlation; Step 4: Use NBS to identify changes in connectivity between pre- and post-ACT.
Graph Analysis
Graph analysis applied to fMRI is a powerful way of characterizing brain networks. In this context, a network represents a collection of nodes, and the functional connections between pairs of nodes. Nodes in large-scale brain networks represent brain regions, with connections being anatomical, functional, or effective, depending on the type of imaging data analyzed. Application of graph theory-based approaches have identified biologically plausible brain networks found to topologically organize in a non-trivial manner (e.g., network integration and modular structure) and support efficient information processing of the brain (Bullmore and Sporns, 2009; Rubinov and Sporns, 2010; Wang et al., 2010; Bullmore and Bassett, 2011). These network analyses allow us to visualize the connectivity pattern across the entire brain and also quantitatively characterize its global organization (Wang et al., 2010; Sporns, 2018). In the current study, we leverage the ability of graph analytics to identify network connections linking rsfMRI connectivity to ACT treatment.
Network Based Statistic
We used the Network Based Statistic (NBS; Zalesky et al., 2010) to examine FC in the DMN, SN, and FPN. This graph theory-based method provides a statistical approach to identify changes in FC associated with diagnostic status or changing psychological contexts (Zalesky et al., 2010). The NBS is based on the principles underpinning traditional cluster-based thresholding of statistical parametric maps. We use the NBS to identify significant network connectivity differences between pre-ACT and post-ACT (Figure 1, step 4). We tested for within network connectivity changes for each of the three networks of interest independently, and then we tested for network connectivity changes across all 101 nodes that make up the FPN, DMN, and SN. Results presented represent functional network differences for t > 2.5 (10,000 permutations). We further examine whether specific pairwise connections in brain networks affected by ACT are related to behavior, and whether connectivity changes associated with ACT correlate with changes in behavior/outcome measures (using Pearson’s r).
Causal Mediation Analysis
Causal mediation analyses were conducted, with confounder adjustment, to evaluate associations between FC changes and behavioral changes (pre- to post-ACT). In other words, we tested whether connectivity changes reflected by the NBS (Figure 1, step 4) were mediators of changes in depression, social role, and CP acceptance scores. A counterfactual mediation approach was implemented in this analysis using SAS PROC CAUSALMED (Valeri and VanderWeele, 2013; VanderWeele, 2015, 2016). Although structural equation modeling (SEM) is frequently used to examine mediation (Baron and Kenny, 1986), its limitations include the following: (1) It does not have a clear theoretical foundation for defining causal mediation effects; (2) It does not deal with confounding and interaction effects effectively; (3) It does not treat binary outcomes and binary mediators in a unified manner. The counterfactual approach overcomes these limitations (MacKinnon et al., 2007; SAS Institute Inc., 2019).
Causal mediation analysis quantifies and estimates the total, direct, and indirect (or mediated) effects between an independent variable and an outcome. It enables causal interpretations of these effects under the assumptions of the counterfactual framework (Robins and Greenland, 1992; Pearl, 2011). The causal mediation model decomposes the total effect into a direct effect [e.g., the effect of an independent variable [A] on outcome [Y; A = 0 vs. A∗ = 1] and the natural indirect effect (NIE)]. The controlled direct effect (CDE) simulates a randomized controlled trial (RCT) by hypothetically assigning the same value of the mediator to all individuals in the sample, with bootstrapped standard errors (Naimi et al., 2014). The NIE captures the effect of the mediation pathway (e.g., the average change in Y if the exposure is fixed to the level of the intervention and the mediator changes accordingly [e.g., A = 0 to A∗ = 1]; VanderWeele, 2015). The mediation path is represented by arrows “B” and “C” in Figure 2.
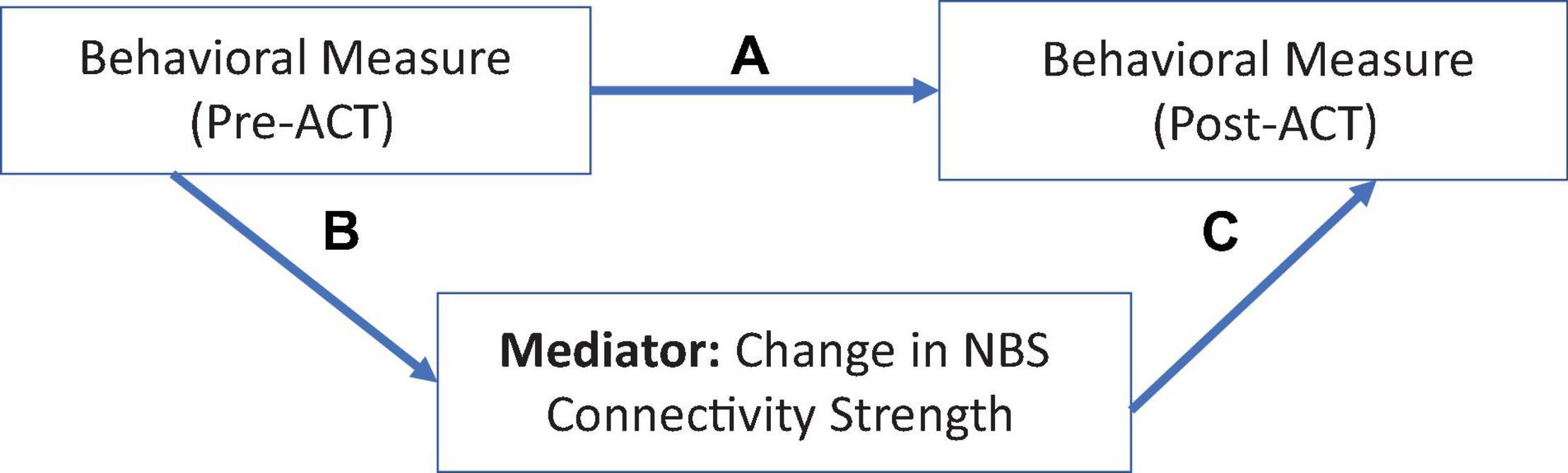
Figure 2. Rationale for the causal mediation analysis. Change in behavioral measures (pre- to post-ACT), mediated by FC changes. Path “A” shows the direct effect; mediation is estimated by combining paths B and C to produce the NIE.
For this analysis, we used the theoretical constructs underlying ACT to guide our approach (e.g., that psychological flexibility, in the context of CP, includes factors pertaining to acceptance and cognitive defusion (learning to experience uncomfortable thoughts, feelings, and sensations in a way that reduces their interference on valued activities and roles in one’s daily life); Chin and Hayes, 2017). Thus, we focused on indicators of CP acceptance, pain interference, depression, and social roles as key behavioral outcomes.
Results
Behavioral change scores from pre-to post- ACT showed statistically significant improvements in clinically relevant outcomes, including depression (measured via the CES-D and the NIH Toolbox Neuro-QoLTM), satisfaction with social role (measured via the NIH Toolbox Neuro-QoLTM), and pain acceptance (measured via the AAQ-II and CPAQ) (Table 2). Two specific sub-scores of the CPAQ indicated that there were significant decreases in pain interference and significant increases in willingness to engage in activities despite pain.
Network Based Statistic
Significant changes in FC from pre- to post-ACT in the DMN, SN, and FPN were observed. Using the NBS, within network effects of ACT were only observed in the SN which consisted of a sub-network of four nodes and three functional connections (Figure 3A, t > 2.5, p = 0.039). No effects of ACT were observed within the DMN or FPN. NBS tests comparing pre-ACT vs. post-ACT of the triple network (DMN, FPN, and SN nodes combined) identified a network of 10 nodes and 10 connections displaying decreases in FC associated with completing ACT (Figure 3B, t > 2.5, p = 0.05). Interestingly, the within network ACT effects observed in the SN were also present in the triple network. Between network changes were also observed in the triple network where all DMN and FPN nodes connected to SN nodes (Figure 3B).
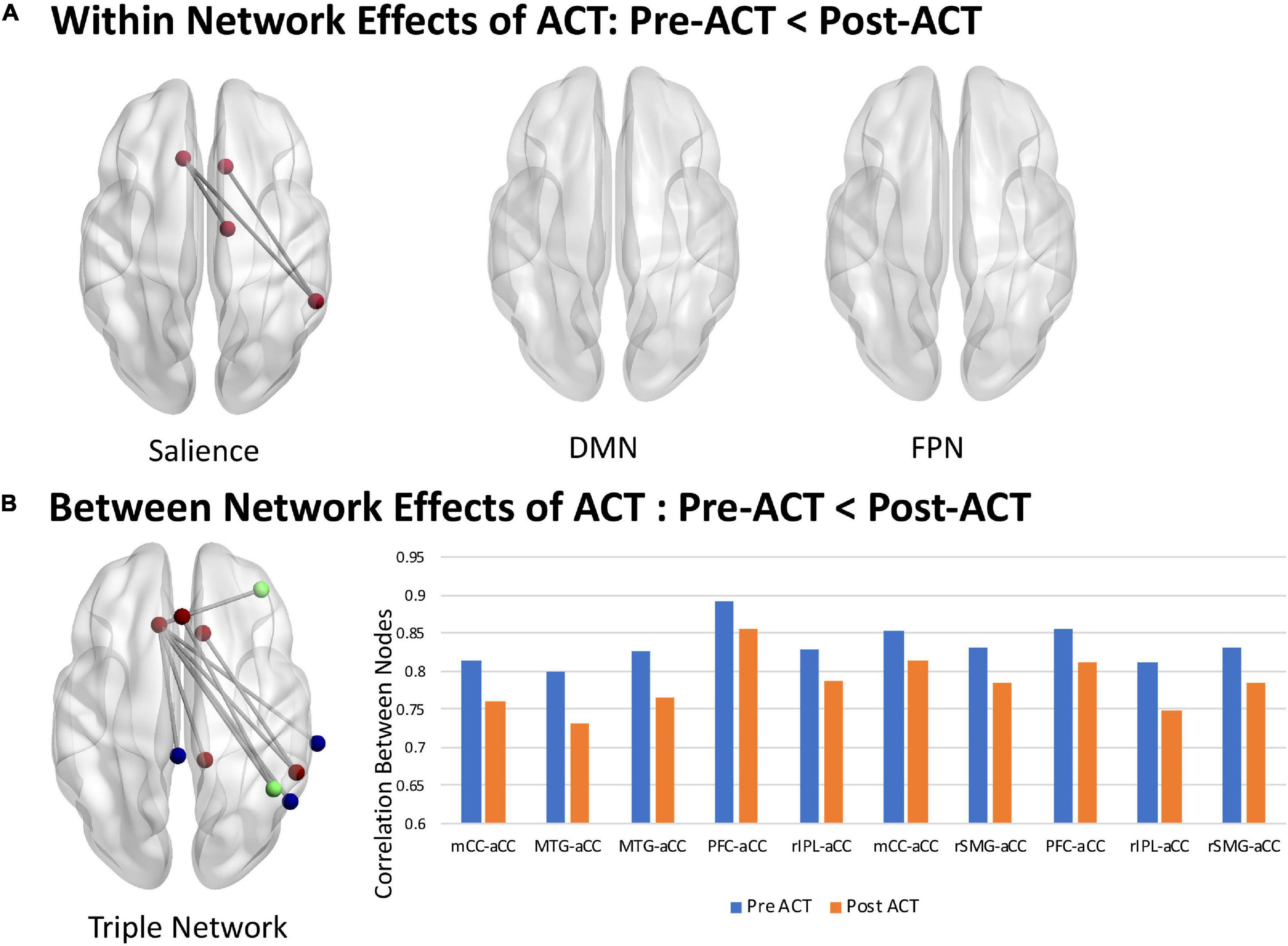
Figure 3. Within and between network effects of ACT. (A) Within network decreases in FC following ACT: Pre-ACT < Post-ACT (t > 2.5, p = 0.039, 10,000 iterations). (B) Between network decreases in FC following ACT: Pre-ACT < Post-ACT (t > 2.5, p = 0.05, 10,000 iterations). Mean functional connections exhibiting ACT effects are shown for Pre-ACT (blue) and Post-ACT (orange). SN, DMN, and FPN nodes are shown in red, blue, and green, respectively. mCC, midcingulate cortex; aCC, anterior cingulate cortex; MTG, medial temporal gyrus; PFC, prefrontal cortex; rIPL, right inferior parietal cortex; rSMG, right superior medial gyrus. Node numbering below anatomical labels correspond to the node ordering in the Power Atlas (see Supplementary Material).
Correlation Analysis
Next, we assessed the relationships between brain connectivity changes and behavioral outcomes using Pearson correlation statistics. Six of the ten functional connections that showed significant differences in strength within the Triple Network from pre- to post-ACT (shown in Figure 3B) were significantly correlated with behavior change scores. Pearson correlations and corresponding p-values for the following significant relationships are shown in Table 3. Results indicated that ACT effects in connectivity (functional connections from NBS analyses shown in Figure 3) between the MTG and aCC (52, −59, 36; −11, 26, 25) and between the PFC and aCC (38, 43, 15; 0, 30, 27) were correlated with social role (Neuro-QoLTM) and acceptance (AAQ-II) scores. FC changes between the rIPL and aCC (two functional connections connecting 44, −53, 47 with −11, 26, 25 and 0, 30, 27) were correlated with Neuro-QoLTM depression scores; and changes between rSMG and aCC (55, −45, 37; 10, 22, 27) were correlated with both depression and social role scores. Changes between rSMG and aCC (55, −45, 37; −11, 26, 25) and rIPL and aCC (44, −53, 47; −11, 26, 25) were correlated with reduced pain severity scores.
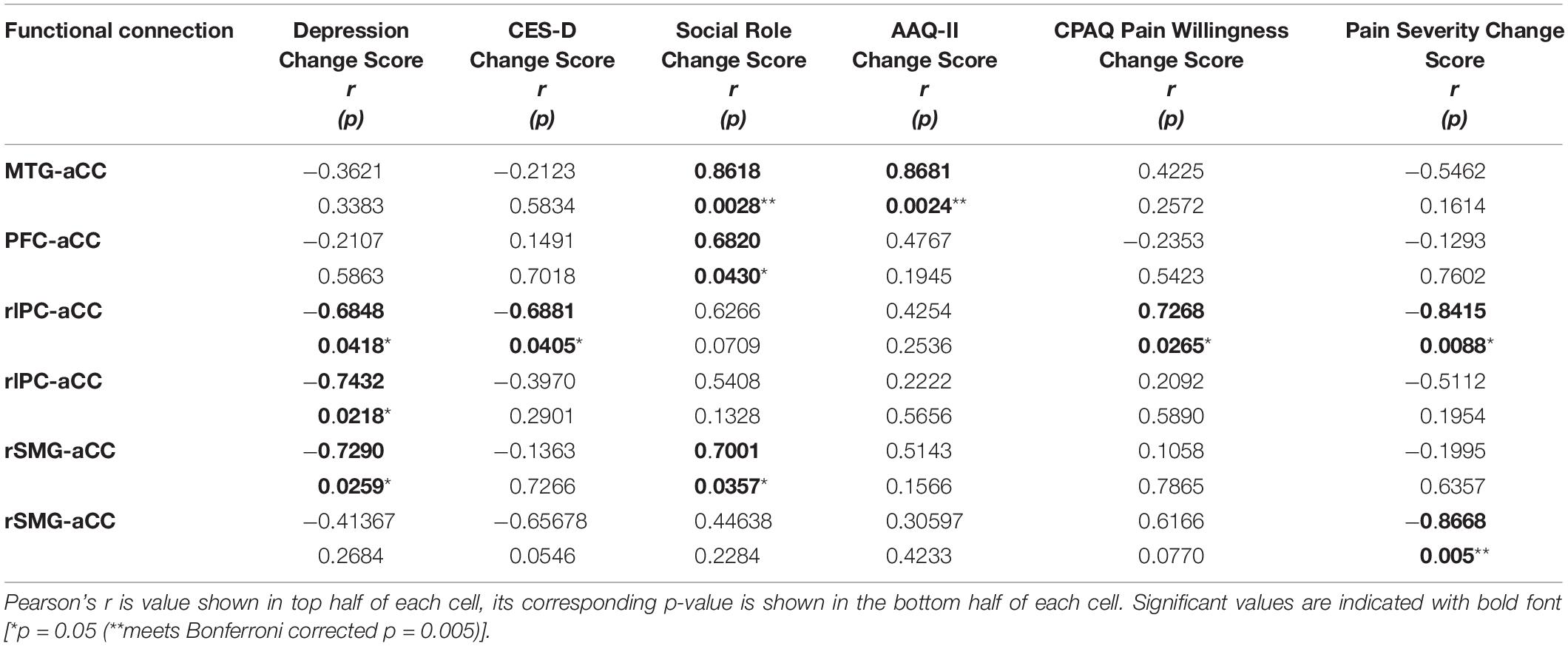
Table 3. Correlations between functional connections in triple network and behavioral assessment scores (post minus pre).
Causal Mediation Model Results
In the causal mediation framework for this study, a baseline behavioral variable (e.g., Pre-ACT Depression Score) was hypothesized to relate to an outcome variable (Post-ACT Depression score) via the causal mechanism that is represented in Figure 2. As depicted in the diagram, the total causal treatment effect pertaining to the outcome (Post-treatment behavioral score) consists of the following two parts: (1) a direct effect; (2) a mediated (or indirect) effect via the “functional connectivity” variable representing change in FC between two brain regions.
Results from our exploratory causal mediation models (Table 4) demonstrated that improvements in specific behavioral outcome scores were significantly related to both the direct pathway “A” (e.g., baseline behavior scores predicted post-treatment scores) and through the indirect mediation pathway “B and C” via changes in FC (the NIE). Statistically significant NIE provide evidence in support of our mediation hypothesis for rSMG-aCC for depression, MTG-aCC for social role, both rSMG-aCC and MTG-aCC for CP acceptance, and rlPL-aCC for pain interference. These relationships persisted after covariate adjustment for age, BMI, pain severity, and other potential confounders. By contrast, statistically significant mediation effects were not observed for rlPL-aCC for depression, PFC-aCC and social role, or MTG-aCC and AAQ-II. No significant interactions were detected.
Discussion
We examined rsfMRI data of nine women before and after completing ACT in efforts to better understand changes in brain FC and associations with specific behavioral outcomes. Importantly, we used NBS to assess network function with graph analyses and took an innovative approach to study of the relationship between imaging and behavioral measures known as causal mediation analysis. Our results showed significant improvements from pre-to post-ACT in clinically relevant behavioral outcomes, including depression, satisfaction with social role, and pain acceptance. These results confirm findings from other studies (McCracken et al., 2004, 2005; McCracken and Vowles, 2006; Wetherell et al., 2011, 2016) and align with the theoretical principles underlying ACT, specifically that constructs of psychological flexibility including acceptance and cognitive defusion (reduced pain interference) may play important roles in functional improvements for individuals suffering from CP (Chin and Hayes, 2017). ACT does not aim to limit exposure to negative experiences, but encourages persons with CP to decrease attention to pain and to move forward in valued life directions despite these experiences. In our study, two specific sub-scores of the CPAQ indicated that there were significant decreases in pain interference and significant increases in willingness to engage in activities despite pain. Other researchers studying mind-body therapies have documented similar results. For example, Haugmark et al. (2019) analyzed health effects of mindfulness- and acceptance-based interventions, including mindfulness-based stress reduction (MBSR), mindfulness-based cognitive therapy (MBCT), and ACT. The authors found small to moderate effects in favor of mindfulness- and acceptance-based interventions compared to controls in pain, depression, anxiety, mindfulness, sleep quality, and health-related quality of life.
We used the NBS (Zalesky et al., 2010) to examine FC in the DMN, SN, and FPN and found within SN effects that extend to brain regions that are components of the DMN and FPN. Prior fMRI studies on mindfulness approaches for treating CP have shown that increased regional activation in the aCC and OFC were associated with reduced ratings of the unpleasantness of pain (Zeidan et al., 2011, 2012, 2015; Lazaridou et al., 2016; Yoshino et al., 2018). Additional multidisciplinary pain treatment programs comprised of daily physical and occupational therapies plus CBT for pain treatment resulted in improved pain measures that correlated with connectivity changes in DMN, SN, and FPN (Simons et al., 2014). Specifically, findings showed treatment driven reductions of hyper-connectivity from the left amygdala to the motor cortex, parietal lobe, and CC. Simons et al. (2014) also found that connectivity to several regions of the fear circuitry (PFC), bilateral middle temporal lobe, bilateral CC, and hippocampus correlated with higher pain-related fear scores, and that decreases in pain-related fear correlated with decreased connectivity among the amygdala and the motor and somatosensory cortex, CC, and the FPN. Across the few studies utilizing longitudinal randomized controlled designs with active control groups, aCC, PFC, pCC, insula, striatum (caudate, putamen), and amygdala show relatively consistent changes associated with mindfulness meditation (Holzel et al., 2011; Tang et al., 2012; Tang and Leve, 2016).
These studies, in conjunction with our results, suggest that the neural correlates of ACT for CP affect sensory brain networks and cognitive function. Thus, our results suggest that the neural mechanisms underlying the multi-faceted nature of ACT for CP are not only related to the DMN, but also to the DMN’s relationship to other networks. A consistent finding across several studies is that CP results in hyper-connectivity among the DMN, SN (Hemington et al., 2016; van Ettinger-Veenstra et al., 2019), and FPN (Napadow et al., 2010). Supporting the current analyses, the transition from acute to CP over a 1-year period has been found to result in a gradual ‘shift’ in fMRI activations from nociceptive networks to emotional brain networks (Hashmi et al., 2013). Collectively, these findings provide impetus for further study of associations between rsfMRI and clinical outcomes.
Behavioral Outcomes and fMRI
Several studies have evaluated the association between fMRI and behavioral outcomes in mindfulness-based interventions (e.g., MBSR), though the majority of these evaluations are not specific to ACT (Tang and Leve, 2016; Feliu-Soler et al., 2018; Zeidan et al., 2018). A recent study (Yoshino et al., 2018) used a CBT intervention with behavioral activation (an ACT component) and found that the OFC played an important role in improvements in pain intensity post-treatment. Activation of the dorsal pCC at pre-treatment was also associated with improvements of clinical symptoms. ACT has also demonstrated sustained medium-large effect sizes on social functioning (Dahl et al., 2004; Feliu-Soler et al., 2018).
Yu et al. (2019) examined ACT-oriented treatment for fatigue in 354 adults with CP. Pearson’s correlations and hierarchical regression were conducted to investigate associations between improvement in fatigue interference and improvements in outcomes. Mixed effects models were used to explore associations between baseline fatigue interference and changes in outcome measures. Results suggested that participants improved in perceptions of fatigue interference, pain, psychological flexibility (PF) processes, and daily functioning. Changes in fatigue interference were associated with changes in pain, PF processes, and daily functioning | r| = 0.20–0.46. Changes in fatigue interference were associated with changes in pain acceptance independent of changes in pain perception. The authors concluded that individuals with fatigue appeared to benefit from the ACT−oriented interdisciplinary treatment for CP, and relatively higher levels of fatigue did not appear to decrease the treatment benefit. Although fatigue was not a focus in our study, the results are similar in terms of demonstrating that the behavioral improvements associated with ACT may persist regardless of pain severity and the presence of other co-morbidities.
Smallwood et al. (2013) examined gray matter volume (GMV) differences between CP patients and healthy controls and found that there were 12 clusters where GMV was decreased in CP patients compared with controls. These clusters included many regions that are considered part of the “pain matrix” involved in pain perception, but also included many other regions that are not commonly regarded as pain-processing areas. The authors also reported that the most common behavioral domains associated with these regions were cognitive, affective, and perceptual domains, suggesting that many of the regions may relate to the constellation of comorbidities that often accompany CP (e.g., fatigue, depression, cognitive, and emotional impairments).
Integrating Behavioral and Neural Network Changes Using Causal Mediation Analysis
Using causal mediation analysis to assess whether changes in connectivity strength mediated changes in specific behavioral outcomes, we observed statistically significant mediation effects for rSMG-aCC with depression, MTG-aCC with social role, rSMG-aCC and MTG-aCC with CP acceptance, and rlPL-aCC with pain interference. Because the models were adjusted for age, BMI, pain severity, and other behavioral covariates, we were able to determine that the relationships were not confounded by these factors.
We also observed significant mediation effects for rSMG-aCC and rlPL-aCC with pain severity, despite the fact that changes in perceived pain severity are not considered direct targets of ACT. In our unadjusted analyses, perceived pain severity scores did not change significantly from pre- to post-ACT, yet the controlled direct effect in the causal mediation models, with confounder adjustment, demonstrated significant changes in both direct and indirect effects. These exploratory analyses underscore the complexity of measuring pain perception, particularly as other therapeutically targeted behavioral changes and associated neural connectivity changes may be occurring simultaneously.
Notably, the median baseline CES-D score among our participants was 16, indicative of high depressive symptomatology (Vilagut et al., 2016) concurrent with CP. After the ACT intervention, the median CES-D score was reduced to 7, and this change appears to be mediated by decreased rSMG-aCC hyperconnectivity.
By contrast, statistically significant mediation effects were not observed for rlPL-aCC with depression, MTG-aCC with AAQ-II, or PFC-aCC with social role. In these cases, the relationships were confounded by other factors and may operate via more complex multiple mediation pathways that could not be tested in this small exploratory sample. For example, although we observed a statistically significant mediation effect for PFC-aCC and social role in unadjusted models, inclusion of body mass index (BMI), pain severity, and baseline depression nullified this relationship.
A growing body of literature has begun to employ mediation analysis to assess the mechanisms underlying behavioral and clinical outcomes (Atlas et al., 2014; DasMahapatra et al., 2015; Gu et al., 2015; Lee et al., 2015; Cederberg et al., 2016; Sanders et al., 2017; Mischkowski et al., 2019; Whibley et al., 2019). However, few studies have assessed behavioral changes with respect to fMRI data using causal mediation analysis, particularly with respect to CP. Lindquist (2012) described an extension of SEMs applied to data from a fMRI study of thermally induced pain. The results suggested that many classic “pain-responsive regions” such as the anterior insula showed significant mediation of the temperature-induced relationship, and that subjective pain ratings increased near the end of the stimulation period. Other regions, such as the insular cortex appeared to be active during pain judgment. Like our study, this study supports the use of mediation modeling in future research to better understand how connectivity changes among different brain regions affect the subjective experience of pain, and may inform pain management approaches.
Limitations and Future Directions
In addition to the small sample size, the limitations of our study include the quasi-experimental research design and the lack of long-term follow-up data beyond the immediate post-ACT period. A randomized controlled trial would have provided more methodological rigor to the study, and randomized trials with longer follow-up periods are needed to confirm the findings reported here.
Villanueva et al. (2019) investigated three key aspects of ACT, including its effectiveness, long-term follow-up, social context, and social processes. The authors contend that researchers should include longer follow up periods in clinical studies (Gloster et al., 2013). This is especially important for treatment-resistant patients (e.g., patients who do not respond to standard, first line treatments), for whom viable treatment options are limited (Gloster et al., 2020). The variable number of years that participants suffered from chronic pain is also a limitation in our study, although all participants meet the clinical definition of chronic pain lasting more than 6 months. The heterogeneity of the participants’ pain conditions also precludes inference about how ACT affects specific types of chronic pain. Due to the comorbid pain conditions experienced by the participants in our sample, we are unable to evaluate the results of ACT on any single type of musculoskeletal pain.
However, the mechanism of ACT may transcend specific genetic and acquired diagnoses that result in chronic pain, which clinicians may perceive as a strength of the intervention. Central sensitization (the enhanced activity of neurons and circuits in nociceptive pathways) underlies many of the changes in chronic pain (Latremoliere and Woolf, 2009), illustrating the contribution of the central nervous system to the generation of pain hypersensitivity across many types of pain diagnoses. Because of central sensitization, chronic pain is not necessarily coupled to the original stimuli source. Moreover, the abnormal pain sensation and sensitivity resulting from central sensitization is enhanced by stress. Aligning with the findings from our study, prior researchers (Lewis et al., 2012) have highlighted neuroplastic changes in the insula (within the salience network and the DMN) with respect to processes affecting mood disturbances, working memory, fatigue, and body awareness issues observed across different chronic pain conditions. More recently, Tang et al. (2020) found that disruptions of multiple networks, including the DMN, salience network, and limbic system, may contribute to the neurophysiological mechanisms underlying postherpetic neuralgia. A growing body of research also suggests that central sensitization may be driven by neuroinflammation in the peripheral and central nervous system, causing widespread chronic pain. For example, Ji et al. (2018) reported that sustained increase of cytokines and chemokines in the central nervous system may promote chronic pain that affects multiple body sites. These processes warrant further study in larger randomized controlled trials.
The fact that participants were all female also limits the generalizability of our findings. Although both males and females were recruited and referred for study participation, the study protocol required daytime commitment to therapy and out-of-state travel. Males cited inflexible work schedules as the main reason why they were unable to participate in the study. However, it is also important to note that prior researchers have documented that the prevalence of chronic pain is higher among females than males (Tsang et al., 2008). Researchers have reported that in addition to psychosocial characteristics that may vary between males and females, differences in genotype and endogenous opioid functioning play a causal role in these disparities, and considerable literature implicates sex hormones as factors influencing pain sensitivity (Bartley and Fillingim, 2013). However, the specific modulatory effect of sex hormones on pain among men and women requires further exploration. Thus, clinicians must consider a myriad of factors when developing a treatment approach to chronic pain, including gender.
Lastly, omitted variable bias may also pose a limitation in our study. For example, factors outside therapy itself, including social processes, may account for up to 33% of improvement in patients undergoing psychotherapy and group-based interventions (Cuijpers et al., 2012). It remains poorly understood how the influence of social surroundings longitudinally affects patients’ well-being, social function, and pain perception (Biglan and Embry, 2012; Wilson et al., 2014). Prior research suggests that both close and extended social ties may be relevant for positive outcomes (Kuehner and Huffziger, 2013). Additional research is needed to better understand the variation in treatment outcomes in relation to an individual’s social and environmental context, using an exposome lens (Juarez et al., 2014). Future research should also consider different ways of measuring pain perception and should evaluate both mediators and moderators of ACT in pain as well as in other chronic diseases.
Conclusion
The mechanistic knowledge generated from this study helps to build the evidence base underlying mind-body therapies such as ACT. ACT has been shown to be particularly efficacious for patients who are older (Wetherell et al., 2016) or have co-occurring mood disorders (Wolitzky-Taylor et al., 2012) who may be unresponsive to other psychosocial treatments. Findings from the present study facilitate identification of neural factors predicting patient responses to mind-body therapies. The outcomes of this study will also support the refinement of non-pharmacologic treatment protocols for CP. This is particularly important with the movement toward ‘stepped care’ models of pain management (Blair et al., 2015), which aim to treat pain within a primary care setting while enabling the use of a variety of integrated multidisciplinary treatment approaches.
Data Availability Statement
The datasets presented in this article are not readily available because due to the small sample size, we would need to ask our IRB about any requests to share data. Requests to access the datasets should be directed to SA, semra.aytur@unh.edu.
Ethics Statement
The study protocol (#6991) was approved by the University of New Hampshire Institutional Review Board on July 19, 2018. All participants provided informed consent.
Author Contributions
All authors contributed to the design and implementation of the research, and to the writing of the manuscript. SA, KR, SM, JC, NW, and DR contributed to the analysis. All authors discussed the results and commented on the manuscript. DR supervised the research.
Funding
This work was funded by the University of New Hampshire Collaborative Research Excellence (CoRE) program (DR and SA, The Neurobiology of Acceptance and Commitment Therapy in Treating Chronic Pain).
Conflict of Interest
The authors declare that the research was conducted in the absence of any commercial or financial relationships that could be construed as a potential conflict of interest.
Acknowledgments
We thank Tye Thompson, Airi Mooers, Bronwyn Leto, Grace Roy, Jennifer Potter, Kristen Rosen, Kathryn Kanzler, Robert Ross, Kerry Nolte, Linda Mamakos, Joseph Haviland, Julia Bushell, Evelyn DeRosa, and Jessica Davis for their assistance with this study.
Supplementary Material
The Supplementary Material for this article can be found online at: https://www.frontiersin.org/articles/10.3389/fnhum.2021.587018/full#supplementary-material
References
Amtmann, D., Cook, K. F., Johnson, K. L., and Cella, D. (2011). The PROMIS initiative: involvement of rehabilitation stakeholders in development and examples of application in rehabilitation research. Arch. Phys. Med. Rehabil. 92(Suppl. 10) S12–S19.
Amtmann, D. A., Cook, K. F., Jensen, M. P., Chen, W.-H., Choi, S. W., Revicki, D., et al. (2010). Development of a PROMIS item bank to measure pain interference. Pain 150, 173–182. doi: 10.1016/j.pain.2010.04.025
Atlas, L. Y., Lindquist, M. A., Bolger, N., and Wager, T. D. (2014). Brain mediators of the effects of noxious heat on pain. Pain 155, 1632–1648. doi: 10.1016/j.pain.2014.05.015
Baron, R. M., and Kenny, D. A. (1986). The moderator-mediator variable distinction in social psychological research: conceptual, strategic, and statistical considerations. J. Pers. Soc. Psychol. 51, 1173–1182. doi: 10.1037/0022-3514.51.6.1173
Bartley, E. J., and Fillingim, R. B. (2013). Sex differences in pain: a brief review of clinical and experimental findings. Br. J. Anaesth. 111, 52–58. doi: 10.1093/bja/aet127
Biglan, A., and Embry, D. D. (2012). A framework for intentional cultural change. J. Context. Behav. Sci. 2, 1–21. doi: 10.1086/449865
Blair, M. J., Ang, D., Wu, J., Outcalt, S. D., Sargent, C., Kempf, C., et al. (2015). Evalutaion of stepped are for Chronic Pain (ESCAPE) in veterans of Iraq and Afghanistan conflicts: a randomized clinical trial. Intern. Med. 175, 682–689. doi: 10.1001/jamainternmed.2015.97
Bond, F. W., Hayes, S. C., Baer, R. A., Carpenter, K. M., Guenole, N., Orcutt, H. K., et al. (2011). Preliminary psychometric properties of the acceptance and action Questionnaire-II: a revised measure of psychological inflexibility and experiential avoidance. Behav. Ther. 42, 676–688. doi: 10.1016/j.beth.2011.03.007
Bullmore, E. T., and Bassett, D. S. (2011). Brain graphs: graphical models of the human brain connectome. Annu. Rev. Clin. Psychol. 7, 113–140. doi: 10.1146/annurev-clinpsy-040510-143934
Bullmore, E. T., and Sporns, O. (2009). Complex Brain Networks: graph theoretical analysis of structural and functional systems. Nat. Rev. Neurosci. 10, 186–198.
Cederberg, J. T., Cernvall, M., Dahl, J., von Essen, L., and Ljungman, G. (2016). Acceptance as a mediator for change in acceptance and commitment therapy for persons with chronic pain? Int. J. Behav. Med. 23, 21–29. doi: 10.1007/s12529-015-9494-y
Cella, D., Lai, J. S., Nowinski, C. J., Victorson, D., Peterman, A., Miller, D., et al. (2012). Neuro-QOL: brief measures of health-related quality of life for clinical research in neurology. Neurology 78, 1860–1867. doi: 10.1212/wnl.0b013e318258f744
Cella, D., Riley, W., Stone, A., Rothrock, N., Reeve, B., Yount, S., et al. (2010). The patient-reported outcomes measurement information system (PROMIS) developed and tested its first wave of adult self-reported health outcome item banks: 2005-2008. J. Clin. Epidemiol. 63, 1179–1194. doi: 10.1016/j.jclinepi.2010.04.011
Chin, F., and Hayes, S. C. (2017). “Acceptance and commitment therapy and the cognitive behavioral tradition,” in The Science of Cognitive Behavioral Therapy, eds S. G. Hofmann and G. J. G. Asmundson (Cambridge, MA: Elsevier Academic Press), 155–173. doi: 10.1016/b978-0-12-803457-6.00007-6
Cleeland, C. S., and Ryan, K. M. (1994). Pain assessment: global use of the brief pain inventory. Ann. Acad. Med. Singapore 23, 129–138.
Cuijpers, P., Driessen, E., Hollon, S. D., van Oppen, P., Barth, J., and Andersson, G. (2012). The efficacy of non-directive supportive therapy for adult depression: a metaanalysis. Clin. Psychol. Rev. 32, 280–291. doi: 10.1016/j.cpr.2012.01.003
Dahl, J., Wilson, K. G., and Nilsson, A. (2004). Acceptance and commitment therapy and the treatment of persons at risk for long-term disability resulting from stress and pain symptoms: a preliminary randomized trial. Behav. Ther. 35, 785–801. doi: 10.1016/s0005-7894(04)80020-0
DasMahapatra, P., Chiauzzi, E., Pujol, L. M., Los, C., and Trudeau, K. J. (2015). Mediators and moderators of chronic pain outcomes in an online self-management program. Clin. J. Pain 31, 404–413. doi: 10.1097/AJP.0000000000000125
Duff, E. (2008). REX: Response Exploration for Neuroimaging Datasets: Guide and Tutorial. Melbourne: University of Melbourne, 37.
Feliu-Soler, A., Montesinos, F., Gutiérrez-Martínez, O., Scott, W., McCracken, L., and Luciano, J. (2018). Current status of acceptance and commitment therapy for chronic pain: a narrative review. J. Pain Res. 11, 2145–2159. doi: 10.2147/JPR.S144631
Fox, M. D., Snyder, A. Z., Vincent, J. L., Corbetta, M., Van Essen, D. C., and Raichle, M. E. (2005). The human brain is intrinsically organized into dynamic, anticorrelated functional networks. Proc. Natl. Acad. Sci. U.S.A. 102, 9673–9678. doi: 10.1073/pnas.0504136102
Gloster, A. T., Hauke, C., Höfler, M., Einsle, F., Fydrich, T., Hamm, A., et al. (2013). Long-term stability of cognitive behavioral therapy effects for panic disorder with agoraphobia: a two-year follow-up study. Behav. Res. Ther. 51, 830–839. doi: 10.1016/j.brat.2013.09.009
Gloster, A. T., Walder, N., Levin, M. E., Twohig, M. P., and Karekla, M. (2020). The empirical status of acceptance and commitment therapy: a review of meta-analyses. J. Contextual Behav. Sci. 18, 181–192. doi: 10.1016/j.jcbs.2020.09.009
Gu, J., Strauss, C., Bond, R., and Cavanagh, K. (2015). How do mindfulness-based cognitive therapy and mindfulness-based stress reduction improve mental health and wellbeing? A systematic review and meta-analysis of mediation studies [published correction appears in Clinical Psychology Review. 2016 Nov;49:119]. Clin. Psychol. Rev. 37, 1−12. doi: 10.1016/j.cpr.2015.01.006
Gutierrez, O., Luciano, M. C., Rodríguez, M., and Fink, B. (2004). Comparison between an acceptance-based and a cognitive-control-based protocol for coping with pain. Behav. Ther. 35, 767–783. doi: 10.1016/s0005-7894(04)80019-4
Hann, K. E., and McCracken, L. M. (2014). A systematic review of randomized controlled trials of acceptance and commitment therapy for adults with chronic pain: outcome domains, design quality, and efficacy. J. Context. Behav. Sci. 3, 217–227. doi: 10.1016/j.jcbs.2014.10.001
Harris, R. (2006). Embracing Your Demons: An Overview of Acceptance and Commitment Therapy. Available online at: https://www.actmindfully.com.au/upimages/Dr_Russ_Harris_-_A_Non-technical_Overview_of_ACT.pdf
Hashmi, J. A., Baliki, M. N., Huang, L., Baria, A. T., Torbey, S., Hermann, K. M., et al. (2013). Shape shifting pain: chronification of back pain shifts brain representation from nociceptive to emotional circuits. Brain 136, 2751–2768. doi: 10.1093/brain/awt211
Haugmark, T., Hagen, K. B., Smedslund, G., and Zangi, H. A. (2019). Mindfulness- and acceptance-based interventions for patients with fibromyalgia – A systematic review and meta-analyses. PLoS One 14:e0221897. doi: 10.1371/journal.pone.0221897
Hemington, K. S., Wu, Q., Kucyi, A., Inman, R. D., and Davis, K. D. (2016). Abnormal cross-network functional connectivity in chronic pain and its association with clinical symptoms. Brain Struct. Funct. 221, 4203–4219. doi: 10.1007/s00429-015-1161-1
Holzel, B. K., Carmody, J., Vangel, M., Congleton, C., Yerramsetti, S. M., Gard, T., et al. (2011). Mindfulness practice leads to increases in regional brain gray matter density. Psychiatry Res. 191, 36–43. doi: 10.1016/j.pscychresns.2010.08.006
Hughes, L. S., Clark, J., Colclough, J. A., Dale, E., and Mcmillan, D. (2017). Acceptance and commitment therapy (ACT) for chronic pain: a systematic review and meta-analyses. Clin. J. Pain 2017, 552–568. doi: 10.1097/ajp.0000000000000425
Institute of Medicine (2011). Relieving Pain in America: A Blueprint for Transforming Prevention, Care, Education, and Research. Washington, DC: The National Academies Press.
Jensen, K. B., Kosek, E., Wicksell, R., Kemani, M., Olsson, G., Merle, J. V., et al. (2012). Cognitive behavioral therapy increases pain-evoked activation of the prefrontal cortex in patients with fibromyalgia. Pain 153, 1495–1503. doi: 10.1016/j.pain.2012.04.010
Jensen, K. B., Srinivasan, P., Spaeth, R., Tan, Y., Kosek, E., Petzke, F., et al. (2013). Overlapping structural and functional brain changes in patients with long-term exposure to fibromyalgia pain. Arthritis Rheum. 65, 3293–3303. doi: 10.1002/art.38170
Ji, R. R., Nackley, A., Huh, Y., Terrando, N., and Maixner, W. (2018). Neuroinflammation and central sensitization in chronic and widespread pain. Anesthesiology 129, 343–366. doi: 10.1097/ALN.0000000000002130
Juarez, P. D., Matthews-Juarez, P., Hood, D. B., Im, W., Levine, R. S., Kilbourne, B. J., et al. (2014). The public health exposome: a population-based, exposure science approach to health disparities research. Int. J. Environ. Res. Public Health 11, 12866–12895. doi: 10.3390/ijerph111212866
Kashdan, T. B., and Rottenberg, J. (2010). Psychological flexibility as a fundamental aspect of health. Clin. Psychol. Rev. 30, 865–878. doi: 10.1016/j.cpr.2010.03.001
Kuehner, C., and Huffziger, S. (2013). Factors predicting the long-term illness course in a cohort of depressed inpatients. Eur. Arch. Psychiatry Clin. Neurosci. 263, 413–423. doi: 10.1007/s00406-012-0379-7
Lancaster, J. L., Cykowski, M. D., McKay, D. R., Kochunov, P. V., Fox, P. T., Rogers, W., et al. (2010). Anatomical global spatial normalization. Neuroinformatics 8, 171–182. doi: 10.1007/s12021-010-9074-x
Latremoliere, A., and Woolf, C. J. (2009). Central sensitization: a generator of pain hypersensitivity by central neural plasticity. J. Pain 10, 895–926. doi: 10.1016/j.jpain.2009.06.012
Lazaridou, A., Kim, J., Cahalan, C. M., Loggia, M. L., Franceschelli, O., Berna, C., et al. (2016). Effects of cognitive-behavioral therapy (CBT) on brain connectivity supporting catastrophizing in fibromyalgia. Clin. J. Pain 33, 215–221. doi: 10.1097/AJP.0000000000000422
Lee, H., Moseley, G. L., Hübscher, M., Kamper, S. J., Traeger, A. C., Skinner, I. W., et al. (2015). Understanding how pain education causes changes in pain and disability: protocol for a causal mediation analysis of the PREVENT trial. J. Physiother. 61, 156. doi: 10.1016/j.jphys.2015.03.004
Lewis, J. D., Wassermann, E. M., Chao, W., Ramage, A. E., Robin, D. A., and Clauw, D. J. (2012). Central sensitization as a component of post-deployment syndrome. NeuroRehabilitation 31, 367–372. doi: 10.3233/NRE-2012-00805
Lim, J. A., Choi, S. H., Lee, W. J., Jang, J. H., Moon, J. Y., Kim, Y. C., et al. (2018). Cognitive-behavioral therapy for patients with chronic pain: implications of gender differences in empathy. Medicine 97, e10867. doi: 10.1097/MD.0000000000010867
Lindquist, M. A. (2012). Functional causal mediation analysis with an application to brain connectivity. J. Am. Stat. Assoc. 107, 1297–1309. doi: 10.1080/01621459.2012.695640
Luoma, J. B., Hayes, S. C., and Walser, R. D. (2007). Learning ACT: An Acceptance and Commitment Therapy Skills-Training Manual for Therapists. New York, NY: Harbinger Publications.
MacKinnon, D. P., Fairchild, A. J., and Fritz, M. S. (2007). Mediation analysis. Annu. Rev. Psychol. 58, 593–614. doi: 10.1146/annurev.psych.58.110405.085542
McCracken, L. M., and Vowles, K. E. (2006). Acceptance of chronic pain. Curr. Sci. 10, 90–94. doi: 10.1007/s11916-006-0018-y
McCracken, L. M., Vowles, K. E., and Eccleston, C. (2004). Acceptance of chronic pain: component analysis and a revised assessment method. Pain 107, 159–166. doi: 10.1016/j.pain.2003.10.012
McCracken, L. M., Vowles, K. E., and Eccleston, C. (2005). Acceptance-based treatment for persons with complex, long standing chronic pain: a preliminary analysis of treatment outcome in comparison to a waiting phase. Behav. Res. Ther. 43, 1335–1346. doi: 10.1016/j.brat.2004.10.003
Meier, S. K., Ray, K. L., Waller, N. C., Gendron, B. C., Aytur, S. A., and Robin, D. A. (2021). Network analysis of induced neural plasticity post-acceptance and commitment therapy for chronic pain. Brain Sci. 11, 10. doi: 10.3390/brainsci11010010
Mischkowski, D., Palacios-Barrios, E. E., Banker, L., Dildine, T. C., and Atlas, L. Y. (2019). Pain or nociception? Subjective experience mediates the effects of acute noxious heat on autonomic responses – corrected and republished. Pain 160, 1469–1481. doi: 10.1097/j.pain.0000000000001573
Naimi, A. I., Moodie, E. M., Auger, N., and Kaufman, J. (2014). Stochastic mediation contrasts in epidemiologic research: interpregnancy interval and the educational disparity in preterm delivery. Am. J. Epidemiol. 180, 436–445. doi: 10.1093/aje/kwu138
Napadow, V., LaCount, L., Park, K., As-Sanie, S., Clauw, D. J., and Harris, R. E. (2010). Intrinsic brain connectivity in fibromyalgia is associated with chronic pain intensity. Arthritis Rheumatol. 62, 2545–2555. doi: 10.1002/art.27497
Pearl, J. (2011). The Mediation Formula: A Guide to the Assessment of Causal Pathways in Nonlinear Models. Chichester, UK: John Wiley and Sons, Ltd. 38.
Penny, W., Friston, K., Ashburner, J., Kiebel, S., and Nichols, T. (2006). Statistical Parametric Mapping: The Analysis of Functional Brain Images, 1 Edn. Cambridge, MA: Academic Press.
Potter, J. S. (2012). Treatment Integrated for Pain and Opioid Dependence: Therapist Manual. San Antonio, TX: University of Texas Health Science Center San Antonio.
Power, J. D., Cohen, A. J., Nelson, S. M., Wig, G. S., Barnes, K. A., Church, J. A., et al. (2011). Functional network organization of the human brain. Neuron 72, 665–678. doi: 10.1016/j.neuron.2011.09.006
Radloff, L. S. (1977). The CES-D scale: a self-report depression scale for research in the general population. Appl. Psychol. Meas. 1, 385–401. doi: 10.1177/014662167700100306
Reddan, M. C., and Wager, T. D. (2018). Modeling pain using fMRI: from regions to biomarkers. Neurosci. Bull. 34, 208–215. doi: 10.1007/s12264-017-0150-1
Robins, J. M., and Greenland, S. (1992). Identifiability and exchangeability for direct and indirect effects. Epidemiology 3, 143–155. doi: 10.1097/00001648-199203000-00013
Rubinov, M., and Sporns, O. (2010). Complex network measures of brain connectivity: uses and interpretations. Neuroimage 52, 1059–1069. doi: 10.1016/j.neuroimage.2009.10.003
Sanders, A. E., Akinkugbe, A. A., Fillingim, R. B., Ohrbach, R., Greenspan, J. D., Maixner, W., et al. (2017). Causal mediation in the development of painful temporomandibular disorder. J. Pain 18, 428–436. doi: 10.1016/j.jpain.2016.12.003
Simons, L. E., Pielech, M., Erpelding, N., Linnman, C., Moulton, E., Sava, S., et al. (2014). The responsive amygdala: treatment-induced alterations in functional connectivity in pediatric complex regional pain syndrome. Pain 155, 1727–1742. doi: 10.1016/j.pain.2014.05.023
Smallwood, R. F., Laird, A. R., Ramage, A. E., Parkinson, A. L., Lewis, J., Clauw, D. J., et al. (2013). Structural brain anomalies and chronic pain: a quantitative meta-analysis of gray matter volume. J. Pain 14, 663−675. doi: 10.1016/j.jpain.2013.03.001
Smallwood, R. F., Potter, J. S., and Robin, D. A. (2016). Neurophysiological mechanisms in acceptance and commitment therapy in opioid-addicted patients with chronic pain. Psychiatry Res. 250, 12–14. doi: 10.1016/j.pscychresns.2016.03.001
Smallwood, R. L., Price, L. R., Campbell, J. L., Garrett, A. S., Atalla, S. W., Monroe, T. B., et al. (2019). Network alterations in comorbid chronic pain and opioid addiction: an exploratory approach. Front. Hum. Neurosci. 13:174. doi: 10.3389/fnhum.2019.00174
Sporns, O. (2018). Graph theory methods: applications in brain networks. Translational Research 20, 11.
Tang, Y., Wang, M., Zheng, T., Xiao, Y., Wang, S., Han, F., et al. (2020). Structural and functional brain abnormalities in postherpetic neuralgia: a systematic review of neuroimaging studies. Brain Res. doi: 10.1016/j.brainres.2020.147219 [Epub ahead of print],
Tang, Y. Y., and Leve, L. D. (2016). A translational neuroscience perspective on mindfulness meditation as a prevention strategy. Transl. Behav. Med. 6, 63–72. doi: 10.1007/s13142-015-0360-x
Tang, Y. Y., Lu, Q., Fan, M., Yang, Y., and Posner, M. I. (2012). Mechanisms of white matter changes induced by meditation. Proc. Natl. Acad. Sci. U.S.A. 109, 10570–10574. doi: 10.1073/pnas.1207817109
Tsang, A., Von Korff, M., Lee, S., Alonso, J., Karam, E., Angermeyer, M. C., et al. (2008). Common chronic pain conditions in developed and developing countries: gender and age differences and comorbidity with depression-anxiety disorders. J. Pain 9, 883–891. doi: 10.1016/j.jpain.2008.05.005
Valeri, L., and VanderWeele, T. J. (2013). “Mediation analysis allowing for exposure–mediator interactions and causal interpretation: theoretical assumptions and implementation with SAS and SPSS macros”: correction to Valeri and VanderWeele (2013). Psychol. Methods 18, 474. doi: 10.1037/a0035596
van Ettinger-Veenstra, H., Lundberg, P., Alföldi, P., Södermark, M., Graven-Nielsen, T., Sjörs, A., et al. (2019). Chronic widespread pain patients show disrupted cortical connectivity in default mode and salience networks, modulated by pain sensitivity. J. Pain Res. 12, 1743–1755. doi: 10.2147/JPR.S189443
VanderWeele, T. J. (2015). Explanation in Causal Inference: Methods for Mediation and Interaction. Oxford: Oxford University Press.
VanderWeele, T. J. (2016). Mediation analysis: a Practitioner’s guide. Annu. Rev. Public Health 37, 17–32. doi: 10.1146/annurev-publhealth-032315-021402
Veehof, M. M., Trompetter, H. R., Bohlmeijer, E. T., and Schreurs, K. M. G. (2016). Acceptance- and mindfullness-based interventions for the treatment of chronic pain: a meta-analytic review. Cogn. Behav. Ther. 45, 5–31. doi: 10.1080/16506073.2015.1098724
Vilagut, G., Forero, C. G., Barbaglia, G., and Alonso, J. (2016). Screening for depression in the general population with the center for epidemiologic studies depression (CES-D): a systematic review with meta-analysis. PLoS One 11:e0155431. doi: 10.1371/journal.pone.0155431
Villanueva, J., Meyer, A. H., Rinner, M. T. B., Firsching, V. J., Benoy, C., Brogli, S., et al. (2019). “Choose change”: design and methods of an acceptance and commitment therapy effectiveness trial for transdiagnostic treatment-resistant patients. BMC Psychiatry 19:173. doi: 10.1186/s12888-019-2109-4
Vowles, K. E., and McCracken, L. M. (2008). Acceptance and values-based action in chronic pain: a study of treatment effectiveness and process. J. Consult. Clin. Psychol. 76, 397–407. doi: 10.1037/0022-006X.76.3.397
Vowles, K. E., McCracken, L. M., McLeod, C., and Eccleston, C. (2008). The chronic pain acceptance questionnaire: confirmatory factor analysis and identification of patient subgroups. Pain 140, 284–291. doi: 10.1016/j.pain.2008.08.012
Vowles, K. E., McCracken, L. M., and O’Brien, J. (2011). Acceptance and values-based action in chronic pain: a three-year follow-up analysis of treatment effectiveness and process. Behav. Res. Ther. 49, 748–755.
Vowles, K. E., and Thompson, M. (2011). “Acceptance and commitment therapy for chronic pain,” in Mindfulness and Acceptance in Behavioral Medicine: Current Theory and Practice, ed. L. M. McCracken (Oakland, CA: New Harbinger Publications), 31–60.
Vowles, K. E., Wetherell, J. L., and Sorrell, J. T. (2009). Targeting acceptance, mindfulness, and values-based action in chronic pain: findings of two preliminary trials of an outpatient group-based intervention. Cogn. Behav. Pract. 16, 49–58. doi: 10.1016/j.cbpra.2008.08.001
Wang, J., Zuo, X., and He, Y. (2010). Graph-based network analysis of resting-state functional MRI. Front. Syst. Neurosci. 4:16. doi: 10.3389/fnsys.2010.00016
Wetherell, J., Petkus, A., Alonso−Fernandez, M., Bower, E., Steiner, A., and Afari, N. (2016). Age moderates response to acceptance and commitment therapy vs. cognitive behavioral therapy for chronic pain. Geriatr. Psychiatry 31, 302–308. doi: 10.1002/gps.4330
Wetherell, J. L., Afari, N., Rutledge, T., Sorrell, J. T., Stoddard, J. A., Petkus, A. J., et al. (2011). A randomized, controlled trial of acceptance and commitment therapy and cognitive-behavioral therapy for chronic pain. Pain 152, 2098–2107. doi: 10.1016/j.pain.2011.05.016
Whibley, D., AlKandari, N., Kristensen, K., Barnish, M., Rzewuska, M., Druce, K. L., et al. (2019). Sleep and pain: a systematic review of studies of mediation. Clin. J. Pain 35, 544–558. doi: 10.1097/AJP.0000000000000697
Wilson, D. S., Hayes, S. C., Biglan, A., and Embry, D. D. (2014). Evolving the future: toward a science of intentional change. Behav. Brain Sci. 37, 395–460. doi: 10.1017/s0140525x13001593
Wolitzky-Taylor, K. B., Arch, J. J., Rosenfield, D., and Craske, M. G. (2012). Moderators and non-specific predictors of treatment outcome for anxiety disorders: a comparison of cognitive behavioral therapy to acceptance and commitment therapy. J. Consult. Clin. Psychol. 80, 786–799. doi: 10.1037/a0029418
Yoshino, A., Okamoto, Y., Okada, G., Takamura, M., Ichikawa, N., Shibasaki, C., et al. (2018). Changes in resting-state brain networks after cognitive–behavioral therapy for chronic pain. Psychol. Med. 48, 1148–1156. doi: 10.1017/S0033291717002598
Yu, L., Norton, S., and McCracken, L. M. (2017). Change in “Self-as-Context” (“Perspective-taking”) occurs in acceptance and commitment therapy for people with chronic pain and is associated with improved functioning. J. Pain 18, 664–672. doi: 10.1016/j.jpain.2017.01.005
Yu, L., Whitney, S., and McCracken, L. M. (2019). Change in fatigue in acceptance and commitment therapy-based treatment for chronic pain and its association with enhanced psychological flexibility. Eur. J. Pain 1, 234–247. doi: 10.1002/ejp.1480
Zalesky, A., Fornito, A., and Bullmore, E. T. (2010). Network-based statistic: identifying differences in brain networks. Neuroimage 53, 1197–1207. doi: 10.1016/j.neuroimage.2010.06.041
Zeidan, F., Emerson, N. M., Farris, S. R., Ray, J. N., Jung, Y., McHaffie, J. G., et al. (2015). Mindfulness meditation-based pain relief employs different neural mechanisms than placebo and sham mindfulness meditation-induced analgesia. J. Eurosci. 35, 15307–15325. doi: 10.1523/jneurosci.2542-15.2015
Zeidan, F., Grant, J. A., Brown, C. A., McHaffie, J. G., and Coghill, R. C. (2012). Mindfulness meditation-related pain relief: evidence for unique brain mechanisms in the regulation of pain. Neurosci. Lett. 520, 165–173. doi: 10.1016/j.neulet.2012.03.082
Zeidan, F., Martucci, K. T., Kraft, R. A., Gordon, N. S., McHaffie, J. G., and Coghill, R. C. (2011). Brain mechanisms supporting the modulation of pain by mindfulness meditation. J. Neurosci. 31, 5540–5548. doi: 10.1523/jneurosci.5791-10.2011
Keywords: Acceptance and Commitment Therapy, chronic pain, neural mechanism, brain networks, functional connectivity, graph theory, fMRI
Citation: Aytur SA, Ray KL, Meier SK, Campbell J, Gendron B, Waller N and Robin DA (2021) Neural Mechanisms of Acceptance and Commitment Therapy for Chronic Pain: A Network-Based fMRI Approach. Front. Hum. Neurosci. 15:587018. doi: 10.3389/fnhum.2021.587018
Received: 24 July 2020; Accepted: 12 January 2021;
Published: 05 February 2021.
Edited by:
Filippo Brighina, University of Palermo, ItalyReviewed by:
Carmelo Attilio Costa, Humanitas Centro Catanese di Oncologia, ItalyGiuseppe Messina, University of Palermo, Italy
Copyright © 2021 Aytur, Ray, Meier, Campbell, Gendron, Waller and Robin. This is an open-access article distributed under the terms of the Creative Commons Attribution License (CC BY). The use, distribution or reproduction in other forums is permitted, provided the original author(s) and the copyright owner(s) are credited and that the original publication in this journal is cited, in accordance with accepted academic practice. No use, distribution or reproduction is permitted which does not comply with these terms.
*Correspondence: Semra A. Aytur, U2VtcmEuYXl0dXJAdW5oLmVkdQ==