- 1Sports Training College of Nanjing Sport Institute, Nanjing, China
- 2Department of Biomedical Engineering, Nanjing University of Aeronautics and Astronautics, Nanjing, China
Objective: The main goal of this study is to clarify the electroencephalogram (EEG) characteristics of the stress response caused by vestibular endurance training under real conditions.
Methods: Ten pilot trainees received a series of acute anti-vertigo training stimulations on the rotary ladder while recording electroencephalographic data (64 electrodes). Afterward, the anti-vertigo ability of the subject was tested for the best performance after 1 month of training and verified whether it is related to the EEG signals we collected before.
Results: (1) The absolute power of α waves in the C3 and C4 regions is the same as the difference between 1 min before and 2 min after stimulation, and their activity is enhanced by stimulation. Otherwise, the activation of the C3 region after 5 min of stimulation is still significantly changed. (2) Through Spearman's correlation analysis, we found that the α waves in the C3 and C4 the greater the power change, the better the performance of the subject in the proficient stage.
Conclusion: C3 and C4 areas are specific brain regions of the stress response of anti-vertigo endurance training, and the absolute power of the α wave can be used as a parameter for identifying the degree of motion sickness (MS). The absolute power changes of α waves in the C3 and C4 areas are positively correlated with their anti-vertigo potential.
Significance: Increasing the absolute power of α wave in the C3 and C4 is a manifestation of MS stress adaptability.
1. Introduction
Driving an aircraft requires substantial cognitive effort and attention from the brain of the operator. According to the statistics of the International Air Transport Association (IATA), more than 80% of air crashes are caused by human errors (Tietje and Brouder, 2009). Although modern aircraft tend to be automated, mental performance of the pilot is still the most common contributing factor in fatal accidents worldwide. Some stimuli in real flight may lead to some psychological damage to pilots. Motion sickness (MS) is one of the main stressors for pilots to be expelled. A large number of flight accidents are caused by MS. The survey showed that the air sickness response rate is 39%, among which the pilot trainees who were expelled due to MS accounted for 55.8% of the number of those who were expelled for physical reasons (Wang and Xue, 1994). Furthermore, most astronauts have also been troubled by MS problems. They often undergo MS training in the first 2–3 days in a microgravity environment and first few days after returning to earth (Heer and Paloski, 2006). MS is commonly used during simulated flight training for astronauts or pilots (Keshavarz et al., 2019). Therefore, MS sensitivity assessment is particularly necessary for selecting pilot trainees, training quasi-pilot personnel, and assessing before the restoration of the flight and after the vestibular damage is recovered (Shupak et al., 2003; Pasquier and Denise, 2019). At present, traditional questionnaires, scales, and physical examinations are still common methods for evaluating MS (Li et al., 2019).
Current MS assessment methods include subjective methods and objective methods. Traditionally, researchers have used subjective questionnaires as their primary tool for evaluating the mental states of pilots (Leandro et al., 2014), such as the MS Questionnaire (Lamb and Kwok, 2015; Liu et al., 2018) and Diagnostic Criteria for Grading the Severity of Acute MS (Graybiel et al., 1968; Liu et al., 2018). In addition, some researchers proposed to judge based on a specific medical history (Ouyang and Xianrong, 2020). However, due to a great influence of subjective factors, the assessment accuracy is poor. With the advancement of evaluation methods and techniques, objective methods have gradually attracted attention. Commonly used objective methods include electroencephalogram (EEG) (Lin et al., 2013; Chuang et al., 2016), electrogastrogram (EGG) (Hu et al., 1989; Zhang et al., 2016), cochlear electrogram (EcochG) (Brown et al., 2017), vestibular evoked myogenic potential (VEMP) (Verrecchia et al., 2012), heart rate variability, and other physiological parameters (Zhang et al., 2016).
Among objective evaluation methods, EEG recordings have been one of the most reliable contemporary methods to assess MS level of the operator, and they can be collected continuously without interfering with the tasks. EEG is the measurement of the electrical activity originated by the brain and recorded on the scalp surface through a net of regularly spaced electrodes. Wu (1992) found that θ power increased in the frontal and central areas when subjects were placed and moved in a parallel swing device (Wu, 1992). Wood et al. (1994) also found increased EEG θ wave in the frontal areas induced by a rotating drum (Wood et al., 1994). Yu et al. (2010) found that the occipital, parietal, and somatosensory brain regions have significantly increased α and θ bands (Yu et al., 2010). Chen et al. (2010) believed that the α power of the parietal and motor areas is suppressed, and the power of the θ and δ waves of the occipital areas increased (Chen et al., 2010). Chuang et al. (2016) pointed out increasing activation of α and γ in the areas of movement, parietal and occipital areas (Chuang et al., 2016). According to the above studies, we discovered that the results of MS-induced EEG power changes are inconsistent or even contradictory, such as inconsistent response areas in the cerebral cortex and conflicts of results on suppression and enhancement of brain wave signals. The reasons for this ending may be in the following three aspects, (1) the wide range of paradigms used to induce MS, (2) the EEG signal evaluation of MS level experiments is mainly based on virtual reality simulator scenes, and (3) it is limited to desktop device acquisition methods (Wood et al., 1994; Lin et al., 2013; Chuang et al., 2016; Li et al., 2019). Therefore, there is an urgent need to supplement EEG tracking data in real and harsh environments and analyze its characteristics to further compare and prove.
Every pilot should go through strict training before getting their flying license. Vestibular endurance training can improve vestibular function to enhance the ability to resist MS (Gordon and Shupak, 2006). The function of the vestibular system is to sense the three-dimensional positioning, maintain the balance of the body, and ensure that the stability of the sight is not disturbed when the head moves. Function of the vestibular system is closely related to MS and spatial disorientation in flight. About 20% of flight accidents caused by spatial disorientation are caused by vestibular and visual illusions (Paillard et al., 2014). Rotary ladder training is the most commonly used vestibular endurance training and vestibular function assessment methods for flying students (Gordon and Shupak, 2006). Based on this, we collected the EEG signals of the flight students in vestibular endurance training in real time online and combined them with the final vestibular endurance performance to verify the sensitivity of the EEG assessment.
2. Methods
2.1. Participants
All subjects (n = 10) volunteered to participate in the study. Besides, all of them are pilot trainees who attended the School of Flight (Nanjing University of Aeronautics and Astronautics, Nanjing, China) for aviation training. All pilots underwent a full physical examination prior to study participation. Inclusion criteria were (1) normal vision; (2) flight status at the experiment time, indicating recent good health; and (3) average 7 h of sleep during the night previous to the experiment without consuming alcohol before the experiment. The final sample included nine men (one was excluded due to an equipment problem), whose average were (SD) 20.1±0.7 years age, 68.3±4.2 kg weight, and 174.6±4.9 cm height. This study was approved by the Ethics Committee of China Pharmaceutical University, and all subjects signed informed consent.
2.2. Experimental Design
Participants who had experienced 35±8.21 min rotary ladder training before firstly experienced a resting state of 5 min, followed by rotary ladder training stimulation, and finally rested for another 5 min. The EEG of all the above processes was recorded. After undergoing a rotary ladder training session for 1 month, the participants took the final performance test (see study protocol and Figure 1).
2.2.1. Study Protocol
Rotary ladder is a basic training course for pilot trainees of the Nanjing University of Aeronautics and Astronautics. Pilot trainees firstly participated in this study at the start of the rotary ladder course, i.e., in the novice stage, and all of them already have some basic rotary ladder skills. After wearing the EEG recording device on the scalp, subjects were asked to undergo a rest state for 5 min, while they were closing their eyes, listening to the white noise through headphones, and trying not to think. Then, subjects were asked to do the rotary ladder movement for 10 turns as shown in Figure 2C. Finally, subjects immediately underwent the resting state (same as before) for another 5 min. since wearing EEG equipment would take time and removing the instrument halfway would affect the stimulation effect, the whole process was recorded (see EEG recording). After the first experiment, all subjects underwent a training course on the rotary ladder for 1 month provided by the flight school. All subjects were trained at exactly the same time and were instructed by the same coach. In addition, we try to ensure that all subjects have the same practice time.The second experiment is carried out simultaneously with the final examination, each pilot student has five examination opportunities during the exam week (in fact subjects conducted an average of 3.5 tests), and the best performance score was recorded and taken into the final examination score. The performance score has been decided by the time on how long subjects used to complete the required number of rotary ladder rotations.
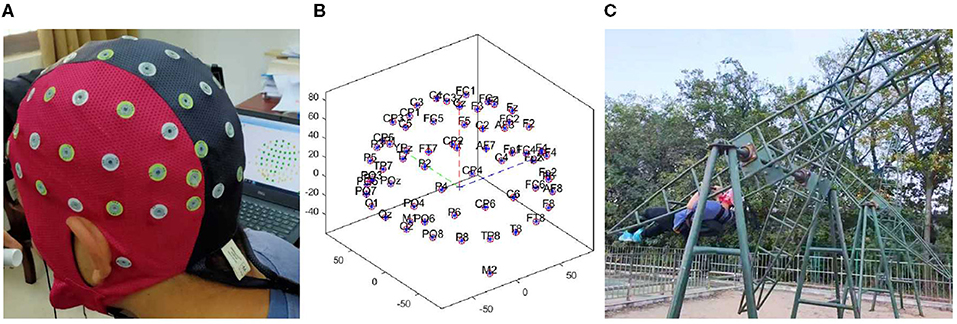
Figure 2. (A) Showed the diagram of device wearing. (B) Showed the location of channels. (C) Showed the rotary ladder movement.
2.2.2. Electroencephalogram Recording
We recorded the EEG signals using a portable EEG recorder (Waveguard Original Cap, 64 Channels, 10/10, Shielded, Tyco68). The device consisted of three parts (Waveguard Original Cap, computer, and EEG headbox with electrodes) fastened to the back of the subject with flexible belts and was fixed stably enough to not be moved by the stimulus (i.e., artifacts from electrode movement that lead to changes in contact impedance or even the generation of a triboelectric response on the wires), as shown in Figure 2A. In order to avoid interrupting the experiment, we recorded all EEG data including the data of rotary ladder movement. The device sampled data at 1,000 Hz. Impedance was kept below 5 kΩ for all electrodes. We used a monopolar montage with gold cup electrodes (Natus Neurology Incorporated-Grass Products Warwick, US) at five active scalp sites: F3, F4, C3, C4, and Cz placed according to the international 10/20 system (Jasper, 1958), and used the mastoids (A1 and A2) as references. The location of channels has been shown in Figure 2B. Ground was placed at FpZ. The channel Cz was recorded by default as an internal device requirement (internal reference). We analyzed the EEG activity of channels F3, F4, C3, and C4 (see EEG analysis). This combination was optimum for avoiding recording errors due to device vibration, electromagnetic interference, and subject movements (Guezennec et al., 2004).
2.2.3. Electroencephalogram Analysis
We analyzed EEG data using the Matlab EEGlab software package (Iversen and Makeig, 2014). The electrode locations were based on a standard 64 channel system provided by EEGlab. The acquired EEG signals were firstly inspected to remove bad EEG channels and the gross areas. To reduce the influence of artifacts, both physiological and non-physiological, in the analysis, we discarded data segments containing voltage values outside the (–100 μV 100 μV) interval. We intercepted the 5-min data before and after the stimulation according to the markers and changed the data sampling rate to 256 Hz. A high-pass filter with a cut off frequency at 1 Hz was used to remove baseline-drifting artifacts. Then, a low-pass filter with a cut off frequency of 40 Hz was applied to the signal to remove muscular artifacts and line noise.
The filtered EEG signals were decomposed into independent brain sources by independent component analysis (ICA) to separate the eye activities, such as eye blinking and lateral eye movements (LEM). The ICA algorithm can separate N source components from N channels of EEG signals and assumed the time courses of the sources are statistically independent. Therefore, The multi-channel EEG record is considered as mixtures of underlying brain sources and artificial signals. The source signals contribute to the scalp EEG signals through a fixed spatial filter. Such a spatial filter can be reflected by the rows of the inverse of unmixed matrix, W in u = Wx, where u is the source matrix and x is the scalp-recorded EEG. The spatial filters can be plotted as the scalp topography of the independent component. The ICA component decomposition result can be seen in Figure 3A.
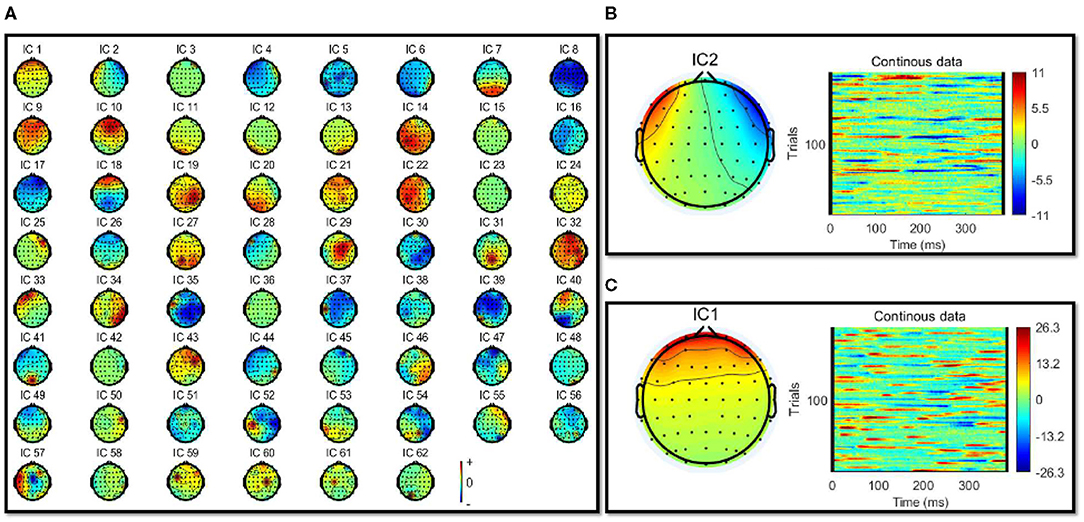
Figure 3. (A) Showed the independent component analysis (ICA) component decomposition result. (B,C) Showed the removed IC components' topographic map and time-frequency results of removed IC components. (B) Showed the eye blink activity component. (C) Shows the lateral eye movements component.
Eye artifacts are always present in EEG datasets. They are usually in leading positions in the component array (because they tend to be big). The eye artifact component can be identified for two reasons: (1) The smoothly decreasing EEG spectrum is typical of an eye artifact; (2) the scalp map shows a strong far-frontal projection typical of eye artifacts. Take one of the subjects as an example, the removed components have been shown in Figures 3B,C. Figure 3B shows a typical component of eye blink activities, and Figure 3C shows a component of lateral eye movements.
After the removal of eye artifacts components, the EEG datasets were segmented in five epochs of 1 min each, so that the first and second epochs (b1, b5) comprised the first and the last minutes of the data before the stimulation, and the second, fourth, and fifth epochs (a1, a2, a5) comprised the first, the second, and the last minutes of the data after the stimulation. The fast Fourier transform (FFT) implemented in the Matlab was used to perform spectral analysis and calculate power spectra for the δ (0.5–4 Hz), θ (4.0–8 Hz), α (8.0–13 Hz), and β (13–30 Hz) frequency bands. Then, we computed the average power for each frequency band, channel, and epoch.
2.3. Statistical Analysis
Electroencephalogram power spectrum changes after stimulation were tested with the one-way repeated-measures ANOVA, which was applied with change from baseline as the dependent variable and treatment as independent variable. The primary focus of the analyses was the changes in the outcome before and after rotary ladder training intervention. When the overall P-value for the interaction between epochs was less than 0.05, prespecified comparisons were used to test two hypotheses: that changes in the second minute after the intervention would differ from that in the resting 1 min before the intervention and that changes in the fifth minute after the stimulation would differ from that in the first minute of the resting stage before intervention. Spearman's correlation coefficient was used to test the correlation between novice EEG power spectrum changes in specific brain regions after rotary ladder stimulation and performance of the rotary ladder test after becoming an expert, and the P-value for the correlation was less than 0.05. Data for EEG power spectrum are presented as means (±SE) and 95% CI. Analyses were carried out using SPSS, version 26.0.
3. Result
We monitored the EEG power spectrum of pilot trainee before and after the rotary ladder stimulation, in relationship to the final vestibular endurance performance.
3.1. Effects of Rotary Ladder Movement on EEG Activity
In order to ensure that the C3 and C4 regions have been activated by the rotary ladder stimulation, we analyzed the EEG power spectrum before and after the stimulation, which has been shown in Figures 4A–D.
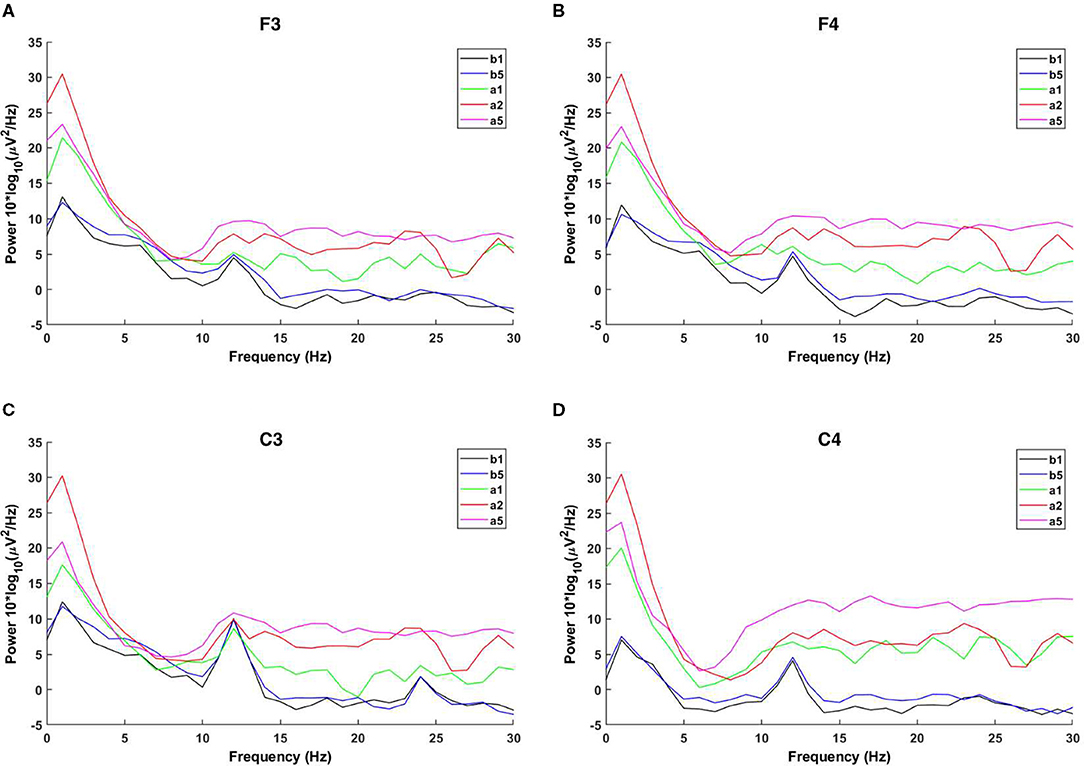
Figure 4. Power spectra. The power spectra of b1 are shown as black lines, the power spectra of b5 are shown as blue lines, the power spectra of a1 are shown as green lines, the power spectra of a2 are shown as red lines, and the power spectra of a5 are shown as pink lines. (A) Shows the power spectra of F3 area, (B) shows the power spectra of F4 area, (C) shows the power spectra of C3 area, and (D) shows the power spectra of C4 area.
Furthermore, we analyzed the EEG power spectrum of α band in C3 and C4 regions before and after the stimulation, which has been shown in Table 1.
One-way repeated measures ANOVA was used to determine the effect of anti-vertigo endurance training on EEG. The data of C3 are not abnormal as indicated by the box plot. After the Shapiro-Wilk test, the data of each group obey the normal distribution (p>0.05). According to Mauchly's spherical hypothesis test, the dependent variable variance-covariance matrix is equal, χ2 = 0.204, P = 0.903. The absolute EEG powers of the subject at the first minute before the intervention (b1), the second minute after the intervention (a2), and the fifth minute after the intervention (a5) were 36.22 ± 10.56, 62.07 ± 13.56, and 59.03 ± 10.00, respectively. The difference between the absolute power of EEG before and after the intervention is statistically significant, F(2, 14) = 7.584, p < 0.01. Compared with the first minute before the intervention (b1), the α absolute power of the EEG increased (25.842) significantly in the second minute after the intervention (a2), p < 0.05. The absolute power of EEG in the fifth minute after the intervention (a5) was significantly increased by 22.808 compared with the first minute before the intervention (b1), p < 0.05. Figure 5 shows the changes before and after the intervention. It can be appreciated in Figure 6 that the absolute power of alpha increased by 26% [95% CI (7.25, 44.436)] from b1 to a2 and 23% [95% CI (6.02, 35.59)] from b1 to a5 in the C3 channel.
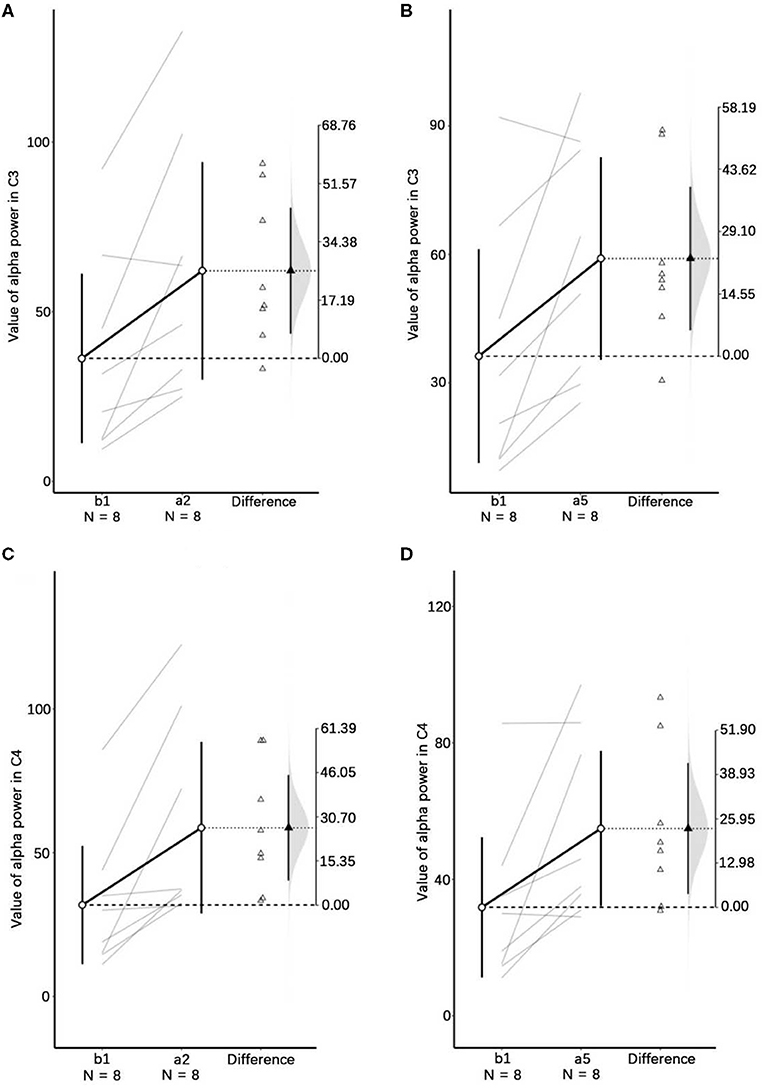
Figure 6. Visualizations emphasizing testing vs. estimation. In this plot, the lines represent the paired participants. The two ends of the line represent the pre- and post-stimulus values of the participant. The circles with error bars represent each group mean with their 95% CI. The triangles show the difference between groups in this paired samples. The shaded curve shows the entire distribution of expected sampling error for the difference between the means. Panels (A–D), respectively, represent the descriptive plots of b1 and a2, and b1 and a5 on the C3 channel, b1 and a2, and b1 and a5 on the C4 channel.
Similarly, Mauchly's spherical hypothesis test of C4 region indicates that the dependent variable covariance matrices are equal, χ2 = 1.931, p = 0.381. The α absolute powers of the subject at the first minute, before intervention (b1), the second minute, and the fifth minute after intervention (a2 and a5) were 31.87 ± 8.70, 58.68 ± 12.64, and 54.91 ± 9.62, respectively. The difference in α absolute power before and after intervention was statistically significant, F(2, 14) = 8.471, p < 0.01. The absolute power of α at the second minute after the intervention (a2) was significantly increased by 26.899, p < 0.05, compared to the first minute before the intervention (b1). Also, the absolute power of the α at the fifth minute after the intervention (a5) was significantly increased by 23.124 than in before the intervention (b1), p = 0.074. The descriptive plots of estimate paired mean difference have been shown in Figure 6. Rotary stress stimulation is estimated to improve alpha power in the C4 channel by 27% from b1 to a2 with a 95% CI of (8.55, 45.25) and 23% from b1 to a5 with a 95% CI of (3.91, 42.33).
The results show that the absolute power of the α waves in the C3 and C4 regions is the same as the difference between the first minute before and the second minute after the stimulation, and their activity is enhanced by stimulation. Therefore, we choose the difference between a2 and b1 for further analysis.
3.2. Relationship Between EEG Activity and Vestibular Endurance Performance
To clarify the relationship between activation enhancement and the endurance training stimulation of the rotary ladder, we correlated the EEG response of the pilot trainee with the performance of the rotary ladder after long-term training as shown in Table 2.
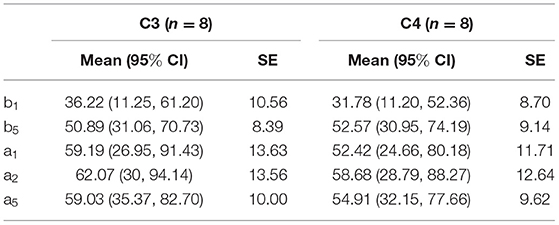
Table 2. Correlation between final performance of expert and EEG response of novice of acute stimulation.
The results show that the absolute power change in the α waves in the C3 and C4 before and after the stimulation in the novice stage is proportional to the performance score of the proficient stage, i.e., the α waves in the C3 and C4, the greater the power change, the better the performance of the subject in the proficient stage, ρX1 = −0.81, p = 0.027; ρX2 = −0.81, p = 0.027. Therefore, the novice acute rotary ladder stimulation may be used to predict the potential skill of the rotary ladder endurance of pilot students.
4. Discussion
Electroencephalogram signals reflect the amounts of neurons that discharge at the same time (Klimesch, 1999). Therefore, its time resolution is high enough to reflect the current oscillatory activity and is thought to be related to the cortical resources employed for information processing (Klimesch, 2012). In this study, we examined how EEG signals reflect vestibular endurance performance. Our results indicate a differential representation of high and low scores of vestibular endurance performance in the EEG signals of pilots. Pilots always need a high level of vestibular endurance that can resistant MS (Shupak et al., 2003). The previous studies have found a relationship between MS levels and EEG activity. Although these results are inconsistent, EEG is still the one of the most reliable contemporary methods to assess the MS level (Chen et al., 2010). Increased MS level may lead to changed power in the α bands, i.e., the 8–12 HZ bands (e.g., Chen et al., 2010), as found here. However, little is known about the EEG response characteristics of vestibular endurance training (rotary ladder movement can cause MS) under real and harsh environments. Also, the correlation between EEG characteristics and the training plasticity of pilot trainees is even more unknown. A qualified flight selection and training need to accurately assess the vestibular endurance performance of pilot trainees. However, the ability of vestibular endurance of pilot candidate is unpredictable. So, we have designed an EEG response experiment of vestibular endurance training in a real environment. We use EEG to continuously track the electrical signals of pilot students that cause MS during rotary ladder training and objectively extract specific changes in specific brain regions. Our results indicate that the vestibular endurance training caused significant changes in the power in of alpha band wave the C3 and C4 areas. Furthermore, we also found that the activations of α band in C3 and C4 were positively correlated with the performance of the rotary ladder after 1 month of systematic training. The following sections describe the details.
4.1. Rotary Ladder Movement Induced MS
The vestibular system refers to three pairs of perpendicular semicircular canals (anterior, posterior, and horizontal semicircular canals) and two otolithic organs (ellipsoid and balloon) in the labyrinth of the middle ear. The semicircular canals can sense rotational acceleration or deceleration of our head, such as when turning the head, starting, or stopping spinning, or somersaulting, and otolithic organ is to sense the gravity stimulation. The function of the vestibular organ is to sense the three-dimensional positioning, maintain the balance of the body, and ensure that the stability of the sight is not disturbed when the head moves. The vestibular system plays an important role in motion perception, eye movement, and posture control (Berthoz, 1996). These complex functions require the integration of information about the location of the eyes, head, and external environment of the vestibule, vision, and somatosensory. This process relies on the cortex and subcortical structures, such as at the subcortical level, especially in the brain stem and cerebellum, and the processing of a large amount of information from the vestibule is important to control the stability of sight and posture. In contrast, spatial orientation and motion perception also require massive processing of vestibular information at the cortical level. In the cerebral cortex of non-human primates, several multimodal sensory areas that integrate vestibular, visual, and somatic sensory signals have been described. These integrated regions can be located in specific areas of the brain (Eickhoff et al., 2006). The cortical areas activated by vestibular stimulation are also supported by extensive research studies, and four major pathways have been hypothesized to transmit vestibular information to the vestibular cortex (Hitier et al., 2014). Vestibular function is closely related to MS and spatial disorientation in flight (Golding et al., 2014). Vestibular endurance training can improve the vestibular function to enhance the ability. It includes two types of training: active training and passive training. The rotary ladder training is the most commonly used vestibular endurance training for pilots and astronauts. It has been considered to relate to the vestibular endurance performance (Gordon and Shupak, 2006). So rotary ladder trainning has been a reliable evaluation method for vestibular endurance performance (Gordon and Shupak, 2006). Therefore, we use the time for subjects to complete the required number of rotary ladder rotations as the standard to assess their vestibular endurance capability.
4.2. Motion Sickness-Related α Band Changes of Parietal Area
Motion sickness is associated with EEG reflection (e.g., Wu, 1992; Wood et al., 1994; Chen et al., 2010). α band of EEG (8–12 Hz) has been shown to be sensitive to sensory processing (Başar et al., 1997; Schürmann et al., 1997), and has been thought to reflect the integration of cortical oscillations in multi-modal sensory systems (Klimesch et al., 2007). These interactions of α rhythm characterize complex cortical calculations and the integration effect between multiple cortical regions. Besides, α EEG characteristics may be the cause of postural instability, which leads to MS (Stoffregen and Smart, 1998). The vestibular system is mainly controlled by the Parieto-Insular Vestibular Cortex (PIVC), which is located in the parietal lobe, and is related to the posterior parietal cortex (Eickhoff et al., 2005, 2006; Shinder and Newlands, 2014; Wirth et al., 2018). PIVC is related to spatial changes in the head (vestibular), neck rotation (proprioception), and movement of visual targets (Shinder and Newlands, 2014). The conclusion of our study that vestibular endurance training interferes with the absolute power of α in the parietal areas C3 and C4 is consistent with the mechanism of action of the α band and vestibular cortex mentioned above. In comparison, some studies on the EEG characteristics of MS have quite different conclusions. For example, visually induced MS is related to the reduction in the frontal area θ power and the temporal area β power when watching 2D and 3D movies (Ali, 2015). MS is also related to increased δ power, decreased frontal-temporal β power (Ali, 2015), and α and γ waves in parietal and occipital regions under virtual reality driving conditions (Chuang et al., 2016). Under simulated driving conditions, MS is associated with a decrease in θ power and an increase in the δ power of the Fz and Cz electrodes (Min et al., 2004), and parietal area, exercise, and occipital brain regions also showed significant changes in EEG power (Ko et al., 2011). HU et al. used optokinetic drums to induce MS and found that the δ power of C3 and C4 was stronger than the baseline (Hu, 1999). The reason for the difference in the conclusions of the previous studies is that the characteristics of EEG cannot be faithfully reflected under virtual or simulated conditions. Yu et al. found that MS is related to the increase in energy in the α and θ bands of the parietal area, occipital area, and somatosensory brain area when riding a car under the real condition (Yu et al., 2010). Vestibular stimulation under harsh, real conditions is greater. Reichenbach et al. found that during active vestibular exercise with higher stimulation intensity, EEG signal changes mainly occurred in the left posterior parietal cortex (Reichenbach et al., 2016). Our results also indicate that EEG is sensitive to MS. In this study, we found a significant difference between the EEG power in the 8–12 HZ spectrum (α bands) before and after the rotary ladder movement in the novice stage. These results are compatible with the recently observed EEG power in relation to the MS in C3 and C4 areas. After verification, we found no significant difference during the same period of time (before or after the stimulation). These results perhaps reflected the effects of rotary ladder stimulation on EEG activities. We believe that the rotary ladder movement activates in the α band covered the C3 and C4 areas. To some extent, this study reveals the EEG characteristics of high-intensity vestibular stimulation in real environments, broadens the way humans are studied, and provides objective support for the selection and evaluation of talents such as pilots and astronauts.
4.3. Electroencephalogram Reflected Vestibular Endurance Performance
We observed a significant relationship in the vestibular endurance performance (rotary ladder performance) and EEG activities. Our results show that the absolute power change of the α waves in the C3 and C4 before and after the stimulation in the novice stage is proportional to the vestibular endurance performance of the proficient stage. In the other words, the α waves in the C3 and C4 the greater the power change, the better the performance of the subject.
Our combined results indicate that EEG could be used to evaluate the vestibular endurance ability and potential performance of a pilot in flight. These findings may also enhance our understanding of the relationship between brain activity and vertigo in complex and reality situations.
5. Conclusion
This study represents the first step toward the collection of real-time online EEG signals from the flight trainees during acute regular vestibular endurance training; in addition, it can also be combined with the association of vestibular endurance performance after training to verify the sensitivity of EEG assessment. The study found that the acute anti-vertigo training triggered a stress response to the absolute power of the α waves in the C3 and C4 areas. The absolute power change of the α waves in the C3 and C4 areas before and after the intervention in the novice stage is proportional to the best movement performance (the time of the best efforts to complete the same number of rotations) of the proficient stage. That is, the greater the change in the absolute power of the α waves in the C3 and C4 areas, the better the performance of the pilots in a proficient stage in the later training. It can be seen that the EEG response of a novice is related to the performance of the rotary ladder after long-term training. The acute rotary ladder stimulation of a novice may be used to predict the potential of the rotary ladder endurance or the ability to resist MS of the pilot students. By this way, we may complete the screening of professionally qualified personnel with special requirements for vestibular function more accurately, early warning of operation safety, etc.
Data Availability Statement
The original contributions presented in the study are included in the article/Supplementary Material, further inquiries can be directed to the corresponding author/s.
Ethics Statement
The studies involving human participants were reviewed and approved by the Ethics Committee of Nanjing Medical University. The patients/participants provided their written informed consent to participate in this study.
Author Contributions
HH: study concept and design, statistical analysis, experimental design, drafting the manuscript, critical review, and editing the manuscript for content. ZF: data acquisition, computational image processing, statistical analysis, drafting the manuscript, critical review, and editing the manuscript for content. ZQ: study concept and design, critical review, and editing the manuscript for content. LY: data acquisition, critical review, and editing the manuscript for content. LT: data acquisition, critical review, study concept and design, and editing the manuscript for content. BQ: data acquisition, critical review, and editing the manuscript for content. All authors contributed to the article and approved the submitted version.
Funding
This work was supported by the China postdoctoral science foundation, grant no. 2019M661837.
Conflict of Interest
The authors declare that the research was conducted in the absence of any commercial or financial relationships that could be construed as a potential conflict of interest.
Publisher's Note
All claims expressed in this article are solely those of the authors and do not necessarily represent those of their affiliated organizations, or those of the publisher, the editors and the reviewers. Any product that may be evaluated in this article, or claim that may be made by its manufacturer, is not guaranteed or endorsed by the publisher.
Supplementary Material
The Supplementary Material for this article can be found online at: https://www.frontiersin.org/articles/10.3389/fnhum.2021.582636/full#supplementary-material
References
Ali, S. (2015). Eeg based time and frequency dynamics analysis of visually induced motion sickness (vims). Australasian Phys. Eng. Sci. Med. 38, 1–9. doi: 10.1007/s13246-015-0379-9
Başar, E., Schürmann, M., Başar-Eroglu, C., and Karakaş, S. (1997). Alpha oscillations in brain functioning: an integrative theory. Int. J. Psychophysiol. 26, 5–29. doi: 10.1016/S0167-8760(97)00753-8
Berthoz, A. (1996). “How does the cerebral cortex process and utilize vestibular signals?” in Disorders of the Vestibular System, eds R. W. Baloh and G. M. Halmagyi (New York, NY: Oxford University Press), 113–125.
Brown, D. J., Pastras, C. J., and Curthoys, I. S. (2017). Electrophysiological measurements of peripheral vestibular function—a review of electrovestibulography. Front. Syst. Neurosci. 11:34. doi: 10.3389/fnsys.2017.00034
Chen, Y. C., Duann, J. R., Chuang, S. W., Lin, C. L., Ko, L. W., Jung, T. P., et al. (2010). Spatial and temporal eeg dynamics of motion sickness. Neuroimage 49, 2862–2870. doi: 10.1016/j.neuroimage.2009.10.005
Chuang, S. W., Chuang, C. H., Yu, Y. H., King, J. T., and Lin, C. T. (2016). Eeg alpha and gamma modulators mediate motion sickness-related spectral responses. Int. J. Neural Syst. 26:1650007. doi: 10.1142/S0129065716500076
Eickhoff, S. B., Schleicher, A., Zilles, K., and Amunts, K. (2005). The human parietal operculum. i. cytoarchitectonic mapping of subdivisions. Cereb. Cortex 16, 254–267. doi: 10.1093/cercor/bhi105
Eickhoff, S. B., Weiss, P. H., Amunts, K., Fink, G. R., and Zilles, K. (2006). Identifying human parieto-insular vestibular cortex using fmri and cytoarchitectonic mapping. Hum. Brain Mapp. 27, 611. doi: 10.1002/hbm.20205
Golding, J. F., Paillard, A. C., and Denise, D. (2014). “Motion sickness in zero-g parabolic flight, in 6th Aerospace Medical Association (ASMA), May 10–14th, Lake Buena Vista, FL.
Gordon, C. R., and Shupak, A. (2006). Motion sickness: Advances in pathogenesis, prediction, prevention, and treatment. Aviat. Space Environ. Med. 77, 1213–1223.
Graybiel, A., Wood, C. D., Miller, E. F., and Cramer, D. B. (1968). Diagnostic criteria for grading the severity of acute motion sickness. Aerosp. Med. 39, 453–455.
Guezennec, C. Y., Jouanin, J. C., and Dussault, C. (2004). Eeg and ecg changes during selected flight sequences. Aviat. Space Environ Med. 75, 889–897.
Heer, M., and Paloski, W. H. (2006). Space motion sickness: incidence, etiology, and countermeasures. Auton. Neurosci. 129, 77–79. doi: 10.1016/j.autneu.2006.07.014
Hitier, M., Besnard, S., and Smith, P. F. (2014). Vestibular pathways involved in cognition. Front. Integr. Neurosci. 8:59. doi: 10.3389/fnint.2014.00059
Hu, S. (1999). Systematic investigation of physiological correlates of motion sickness induced by viewing an optokinetic rotating drum. Aviat. Space Environ. Med. 70, 757–765.
Hu, S., Stern, R. M., Vasey, M. W., and Koch, K. L. (1989). Motion sickness and gastric myoelectric activity as a function of speed of rotation of a circular vection drum. Aviat. Space Environ. Med. 60, 411–414.
Iversen, J. R., and Makeig, S. (2014). MEG/EEG Data Analysis Using EEGLAB. Berlin; Heidelberg: Springer.
Jasper, H. (1958). The ten-twenty electrode system of the international federation. Electroenc. Clin. Neurophysiol. 10, 370–375.
Keshavarz, B., Philipp-Muller, A. E., Hemmerich, W., Riecke, B. E., and Campos, J. L. (2019). The effect of visual motion stimulus characteristics on vection and visually induced motion sickness. Displays 58, 71–81. doi: 10.1016/j.displa.2018.07.005
Klimesch, W. (1999). Eeg alpha and theta oscillations reflect cognitive and memory performance: a review and analysis. Brain Res. Rev. 29, 169–195. doi: 10.1016/S0165-0173(98)00056-3
Klimesch, W. (2012). Alpha-band oscillations, attention, and controlled access to stored information. Trends Cogn. Sci. 16, 606–617. doi: 10.1016/j.tics.2012.10.007
Klimesch, W., Sauseng, P., and Hanslmayr, S. (2007). Eeg alpha oscillations: the inhibition–timing hypothesis. Brain Res. Rev. 53, 63–88. doi: 10.1016/j.brainresrev.2006.06.003
Ko, L. W., Wei, C. S., Jung, T. P., and Lin, C. T. (2011). Estimating the Level of Motion Sickness Based on EEG Spectra. Berlin; Heidelberg: Springer.
Lamb, S., and Kwok, K. C. S. (2015). Mssq-short norms may underestimate highly susceptible individuals: updating the mssq-short norms. Hum. Factors 57, 622–633. doi: 10.1177/0018720814555862
Leandro, L. D. S., McCamy, M. B., Stephen, L. M., Hooft, N., Catena, A., and Martinez-Conde, S. (2014). Saccadic eye movement metrics reflect surgical residents' fatigue. Ann. Surg. 259, 824–829. doi: 10.1097/SLA.0000000000000260
Li, Y., Liu, A., and Ding, L. (2019). Machine learning assessment of visually induced motion sickness levels based on multiple biosignals. Biomed. Signal Proc. Control 49, 202–211. doi: 10.1016/j.bspc.2018.12.007
Lin, C. T., Fellow, I. E. E. E., Tsai, S. F., and Member (2013). Eeg-based learning system for online motion sickness level estimation in a dynamic vehicle environment. IEEE Trans. Neural Netw. Learn. Syst. 24, 1689–1700. doi: 10.1109/TNNLS.2013.2275003
Liu, A., Ding, L., and Li, Y. (2018). “Motion sickness measurements for young male adults in vitality, endurance, profiles and sensitivity, in Advances in Human Aspects of Transportation. AHFE 2017. Advances in Intelligent Systems and Computing, Vol. 597, ed N. Stanton (Cham: Springer).
Min, B. C., Chung, S. C., Min, Y. K., and Sakamoto, K. (2004). Psychophysiological evaluation of simulator sickness evoked by a graphic simulator. Appl. Ergon. 35, 549–556. doi: 10.1016/j.apergo.2004.06.002
Ouyang, T., and Xianrong, X. Z. (2020). Motion sickness in military aircrews and candidates: case series and implication for aeromedical selection and assessment. Acad. J. Chin. PLA Med. Sch. 41, 32–34. doi: 10.3969/j.issn.2095-5227.2020.01.009
Paillard, A. C., Quarck, G., and Denise, P. (2014). Sensorial countermeasures for vestibular spatial disorientation. Aviat. Space Environ. Med. 85, 563–567. doi: 10.3357/ASEM.3735.2014
Pasquier, F., and Denise, P. G. A. (2019). Impact of galvanic vestibular stimulation on anxiety level in young adults. Front. Syst. Neurosci. 13:57. doi: 10.3389/fnsys.2019.00057
Reichenbach, A., Bresciani, J.-P., Bülthoff, H., and Thielscher, A. (2016). Reaching with the sixth sense: vestibular contributions to voluntary motor control in the human right parietal cortex. Neuroimage 124:869–875. doi: 10.1016/j.neuroimage.2015.09.043
Schürmann, M., BaAr-Eroglu, C., and BaAr, E. (1997). A possible role of evoked alpha in primary sensory processing: common properties of cat intracranial recordings and human eeg and meg. Int. J. Psychophysiol. 26, 149–170. doi: 10.1016/S0167-8760(97)00762-9
Shinder, M. E., and Newlands, S. D. (2014). Sensory convergence in the parieto-insular vestibular cortex. J. Neurophysiol. 111, 2445–2464. doi: 10.1152/jn.00731.2013
Shupak, A., Nachum, Z., Stern, Y., Tal, D., Gil, A., and Gordon, C. R. (2003). Vestibular neuronitis in pilots: follow-up results and implications for flight safety. Laryngoscope 113, 316–321. doi: 10.1097/00005537-200302000-00022
Stoffregen, T. A., and Smart, L. J. (1998). Postural instability precedes motion sickness. Brain Res. Bull. 47:448. doi: 10.1016/S0361-9230(98)00102-6
Tietje, C., and Brouder, A. (Eds.). (2009). Handbook of Transnational Economic Governance Regimes (Leiden: Brill), 1041–1054.
Verrecchia, L., Gennser, M., Tribukait, A., and Brantberg, K. (2012). Superior vestibular dysfunction in severe decompression sickness suggests an embolic mechanism. Aviat. Space Environ. Med. 83, 1097–1100. doi: 10.3357/ASEM.3284.2012
Wang, E. T., and Xue, S. Y. (1994). A review of otolaryngologic aircrew disqualification in the chinese air force, 1961-90. Aviat. Space Environ. Med. 65, 424–427.
Wirth, A. M., Frank, S. M., Greenlee, M. W., and Beer, A. L. (2018). White matter connectivity of the visual-vestibular cortex examined by diffusion-weighted imaging. Brain Connect 8, 235–244. doi: 10.1089/brain.2017.0544
Wood, C. D., Stewart, J. J., Wood, M. J., Struve, F. A., Straumanis, J. J., Mims, M. E., et al. (1994). Habituation and motion sickness. J. Clin. Pharmacol. 34, 628–634. doi: 10.1002/j.1552-4604.1994.tb02016.x
Wu, J. P. (1992). EEG changes in man during motion sickness induced by parallel swing. Space Med. Med. Eng. 5, 200–205.
Yu, Y., Lai, P., Ko, L., Chuang, C., Kuo, B., and Lin, C. (2010). “An eeg-based classification system of passenger's motion sickness level by using feature extraction/selection technologies, in The 2010 International Joint Conference on Neural Networks (IJCNN) (Barcelona: IEEE), 1–6.
Keywords: alpha rhythm, electroencephalogram, vestibular endurance, motion sickness, aviation flight students
Citation: Hu H, Fang Z, Qian Z, Yao L, Tao L and Qin B (2021) Stress Assessment of Vestibular Endurance Training for Civil Aviation Flight Students Based on EEG. Front. Hum. Neurosci. 15:582636. doi: 10.3389/fnhum.2021.582636
Received: 13 November 2020; Accepted: 05 July 2021;
Published: 19 August 2021.
Edited by:
Filippo Brighina, University of Palermo, ItalyReviewed by:
Enrique Soto, Meritorious Autonomous University of Puebla, MexicoSyed Anwar, University of Engineering and Technology, Lahore, Pakistan
Copyright © 2021 Hu, Fang, Qian, Yao, Tao and Qin. This is an open-access article distributed under the terms of the Creative Commons Attribution License (CC BY). The use, distribution or reproduction in other forums is permitted, provided the original author(s) and the copyright owner(s) are credited and that the original publication in this journal is cited, in accordance with accepted academic practice. No use, distribution or reproduction is permitted which does not comply with these terms.
*Correspondence: Zhiyu Qian, emhpeXVAbnVhYS5lZHUuY24=