- 1Department of Psychiatry, National Clinical Research Center for Mental Disorders, The Second Xiangya Hospital of Central South University, Changsha, China
- 2Department of Neurology, The First Affiliated Hospital of Guangxi Medical University, Nanning, China
- 3Department of Radiology, Tianjin Medical University General Hospital, Tianjin, China
- 4Department of Psychiatry, The Third People's Hospital of Foshan, Foshan, China
Whether brain function is altered in patients with dry eye disease (DED) remains unclear. Twenty patients with DED and 23 healthy controls (HCs) were scanned using resting-state functional magnetic resonance imaging. Regional homogeneity (ReHo) and support vector machine (SVM) were used to analyze the imaging data. Relative to the HCs, the patients with DED showed significantly increased ReHo values in the left inferior occipital gyrus (IOG), left superior temporal gyrus, and right superior medial prefrontal cortex, and significantly decreased ReHo values in the right superior frontal gyrus/middle frontal gyrus and bilateral middle cingulum (MC). SVM results indicated that the combination of ReHo values in the left MC and the left IOG in distinguishing patients with DED from HCs had a sensitivity of 95.00%, a specificity of 91.30%, and an accuracy of 93.02%. The present study found that the patients with DED had abnormal ReHo values in the limbic-cortical circuits. A combination of ReHo values in the left MC and the left IOG could be applied as a potential imaging biomarker to distinguish patients with DED from HCs. The dysfunction of limbic-cortical circuits may play an important role in the pathophysiology of DED.
Introduction
Dry eye disease (DED) is a common health problem because of its morbidity, prevalence (5–35%), and cost implications (Smith, 2007; Messmer, 2015). DED is more frequently reported by women than by men, and its likelihood increases with age (Moss et al., 2000; Schaumberg et al., 2003). Dry eye (DE) is defined as “a multifactorial disease of the ocular surface characterized by a loss of homeostasis of the tear film accompanied by ocular symptoms, in which tear film instability and hyperosmolarity, ocular surface inflammation and damage, and neurosensory abnormalities play etiological roles” by the Tear Film and Ocular Surface Society Dry Eye Workshop II (Craig et al., 2017). “Neurosensory abnormalities” are indeed included in the DE definition, but the neuropathophysiology mechanism of DED remains unclear.
The tear film plays an important role in providing a refractive interface for the optical light path, and protection and lubrication for the ocular surface (Willcox et al., 2017). The major ingredients of the tear film are aqueous tear, lipid components, and mucins. Aqueous tear is mainly produced by lacrimal glands. Lipid components are generally synthesized by meibomian glands. Mucins are mainly synthesized by conjunctival goblet cells (Ellingham et al., 1999) and stratified squamous epithelial cells (Argüeso et al., 2003). DED may be caused by the dysfunction of these glands and cells (Mathers, 2000; Mantelli and Argüeso, 2008) or the neuronal circuits regulating tear secretion (van Bijsterveld et al., 2003; Dartt, 2009). In DED, aberrations and scattering induced by alterations of the tear film directly cause disturbances in vision quality (Benito et al., 2011; Tan et al., 2015). In addition to visual disturbance, most patients with DED also complain of ocular discomfort accompanied by increased blinks. Ocular discomfort comprises ocular fatigue and unpleasant sensations such as pain, itching, and dryness. The unpleasant sensations are induced by the pathological processes affecting the trigeminal sensory nerves innervating the ocular and periocular tissues. In some studies, the terms “dryness,” “itching,” “foreign body sensation,” and “burning” are applied to describe the ocular pain associated with DED (Mertzanis et al., 2005; Kalangara et al., 2016). Ocular pain is an uncomfortable and unpleasant sensory and emotional experience induced by the interconnected peripheral nervous system (PNS) and central nervous system (CNS). The enhanced excitability of central pain pathways result from the local activation of microglia and weakened inhibitory descending modulation (Tulleuda et al., 2011). The inhibitory descending control systems come from higher brain centers and modulate ascending excitatory nociceptive pathways by influencing the trigeminal and spinal sensory input (Tracey and Mantyh, 2007; Khasabov et al., 2015). However, the role of descending control systems in DED has not been comprehensively studied. Ocular pain in patients with DED is mainly induced by peripheral insults to the innervated ocular and periocular tissues. However, in certain circumstances, ocular pain in patients with DED is induced by direct injuries to, or dysfunctions of, the cortical and subcortical structures, which process the peripheral nociceptive input, the peripheral nociceptive sensory neurons located in the trigeminal and dorsal root ganglion, and the higher-order neurons located in the spinal cord, brain stem, and thalamus (von Hehn et al., 2012; Belmonte et al., 2015). This pain is called “neuropathic pain.” An emerging concern is that a subset of DED should be represented as a chronic neuropathic pain (Kalangara et al., 2016). In DED, the persistent deficiency of tears results in peripheral nerve damage and ocular inflammation. Peripheral nerve damage and ocular inflammation have complex interactions (Ordovas-Montanes et al., 2015). Long-term peripheral nerve damage and ocular inflammation induce alterations in the structures and functions of PNS and CNS involved in ocular sensory pathways (Belmonte et al., 2015, 2017; Rahman et al., 2015; Levitt et al., 2017), thereby leading to neuropathic pain and central sensitization. Central sensitization is caused by decreased activation thresholds and abnormal amplifying signals within the CNS through neuroplastic processes (Latremoliere and Woolf, 2009; Galor et al., 2015). In DED, the increased blinks result from the enhanced activities of the ocular surface sensory nerve evoked by the stimulation of an unstable tear film (Nakamori et al., 1997) and/or from the redistribution of the tear film over the cornea to obtain enhanced image quality (Tan et al., 2015). The level of dopamine released by the basal ganglia can modulate the blink rate in DED, as observed in a rat model study (Kaminer et al., 2011).
In sum, one can reasonably hypothesize that abnormal brain function plays an important role in DED symptoms' maintenance and development. Abnormal brain structure and function in Sjögren syndrome, a subset of DED (Craig et al., 2017), have been reported in many neuroimaging studies. A study applied computed tomography and magnetic resonance imaging (MRI) and identified 24 patients with white matter abnormalities from a total of 49 patients with Sjögren syndrome with DE (Akasbi et al., 2012). White matter hyperintensities were also found in patients with Sjögren syndrome in the studies that applied MRI and voxel-based morphometry (Tzarouchi et al., 2011, 2014). In a study that applied voxel-wise and global brain volume analyses, the patients with Sjögren syndrome showed lower white matter volumes, not gray matter volumes, than the healthy controls (HCs) (Lauvsnes et al., 2014). Another study found decreased gray matter volume in the cortex and cerebellum in patients with Sjögren syndrome (Tzarouchi et al., 2011). A study applied resting-state functional magnetic resonance imaging (rs-fMRI) and functional connectivity analysis and found altered hippocampal functional connectivity in patients with primary Sjögren syndrome (Zhang et al., 2020). Another study applied rs-fMRI and regional homogeneity (ReHo) analysis and found abnormal ReHo values in the frontoparietal junction area and visual cortex in patients with Sjögren syndrome (Xing et al., 2018). Nevertheless, whether brain function is altered in DED remains unclear. Therefore, a study on brain function will facilitate the understanding of the underlying neuropathophysiology of DED.
Herein, we applied rs-fMRI to map functional brain and decipher spontaneous cerebral neuro-activities. Since Bharat Biswal et al.'s study using rs-fMRI (Biswal et al., 1995), rs-fMRI has been widely used to map functional brain and decipher spontaneous cerebral neuro-activities by measuring the blood oxygen level-dependent (BOLD) signal. Relative to task-based fMRI, rs-fMRI can observe cerebral neurophysiological processes without requiring task performance. Therefore, applying rs-fMRI avoids the potential limitation of applying task-based fMRI in fMRI studies.
After accessing the neuroimaging data of patients with DED and HCs via rs-fMRI, ReHo was utilized to depict the local features of BOLD signals and thus reflect the local synchronization of spontaneous brain activities. Kendall's coefficient concordance (KCC) of the voxel similarity of the time series of a given voxel with the nearest neighboring voxels was applied to measure the ReHo values (Zang et al., 2004). ReHo has been successfully utilized to explore the abnormalities of regional functional synchronization in some ophthalmologic illnesses, such as glaucoma, amblyopia, and corneal ulcer (Lin et al., 2012; Chen et al., 2017; Xu et al., 2019). However, whether patients with DED have abnormal ReHo in certain brain regions, particularly in the brain sensory and visual processing regions, remains unclear.
Support vector machine (SVM) learning is a robust classification tool. This supervised learning algorithm is usually used to recognize patterns and analyze data. Relative to Bayesian networks, decision trees, and artificial neural networks, SVM has significant strengths, such as high accuracy, direct geometric interpretation, and excellent mathematical tractability (Zhang and Wu, 2012). Moreover, SVM does not need large training samples to avoid overfitting (Li et al., 2010); this feature is particularly effective in classification when the sample size is small (Chen and Chen, 2017). Therefore, SVM was applied in this work to determine whether abnormal ReHo values in certain brain areas could be used to distinguish patients with DED from HCs.
We hypothesized that patients with DED would show abnormal ReHo in certain brain regions, particularly in the brain sensory and visual processing regions, and that such abnormal ReHo in relevant regions might serve as possible imaging biomarkers to distinguish patients with DED from HCs via SVM.
Materials and Methods
Participants
A total of 20 right-handed patients with DED (age ≥18 years) were eligible to participate in the whole study. The diagnosis of DED was confirmed by an ophthalmologist by using DED diagnostic guidelines published in 2007 by the Dry Eye Workshop (Lemp, 2007). The exclusion criteria were as follows: (1) patients with other ophthalmic diseases, such as glaucoma, cataract, diabetic retinopathy, and amblyopia; (2) patients with a history of metabolic encephalopathy, hypertensive encephalopathy, CNS infection, and CNS lesions induced by other causes; and (3) patients who were pregnant.
We recruited 23 right-handed HCs (age ≥18 years) from the community. The sex ratio, age, and years of education of the HCs and patients were group-matched. They were also interviewed using the DED diagnostic guidelines published in 2007 by the Dry Eye Workshop. They were excluded if they had a history of neuropsychiatric illness or brain injury or if they were pregnant.
The ethics committee of the First Affiliated Hospital of Guangxi Medical University approved the study. The study was executed according to the Helsinki Declaration. All participants provided an informed written consent.
Scan Acquisition
In this study, rs-fMRI was performed using a Siemens 3.0 T scanner and a standard head coil. The participants lay on the scanner bed with their eyes closed. They were instructed to remain calm and awake. They used foam pads and soft earplugs to reduce head motion and scanning noise. A gradient-echo-planar imaging (EPI) sequence was used to acquire the imaging data with the following parameters: repetition time = 2,000 ms, echo time = 30 ms, 30 slices, 90° flip angle, 64 × 64 matrix, 240 mm field of view, 4 mm slice thickness, 0.4 mm gap, and 250 volumes (500 s).
Data Preprocessing
Statistical parametric mapping software (SPM12; http://www.fil.ion.ucl.ac.uk/spm/) and the Data Processing Assistant for Resting-State fMRI were applied to preprocess the images. The first 10 images of each participant were discarded due to unstable initial MRI signals. We corrected the fMRI images for head motion and acquisition delay between slices. The participants must have translations of <2 mm and rotations of <2° in the x, y, or z axis. Thereafter, all imaging data were spatially normalized to the standard Montreal Neurological Institute (MNI) EPI template in SPM12 and sampled again to 3 mm cubic voxels. The resulting fMRI images were subjected to bandpass filtering (0.01–0.08 Hz) and time-course linear detrending to reduce the influence of high-frequency physiological noise and low-frequency drift.
ReHo Analysis
ReHo is an rs-fMRI measurement that is utilized to explore regional functional synchronization. The Resting-State fMRI Data Analysis Toolkit (REST, http://resting-fmri.sourceforge.net) was utilized to conduct the ReHo analysis. Individual ReHo maps were produced by calculating the KCC of the time series of a given voxel with those of its nearest voxels (26 voxels). The formula for calculating the KCC value was introduced in a previous study (Zang et al., 2004). The ReHo maps were normalized to reduce the influence of individual variations on the KCC values by dividing the KCC values among each voxel by the whole brain average KCC. Thereafter, the ReHo maps were smoothed with a Gaussian kernel of 4 mm full width at half maximum.
Statistical Analysis
SPSS 18.0 (Chicago, IL) was used to compare the demographic characteristics of the two groups. To assess the differences in sex distribution, we performed a chi-square test on the two groups. For the differences in ages and years of education, we performed two-sample t-tests. The differences in the ReHo values of the HCs and patients with DED were compared by two-sample t-tests with years of education and age as the covariates of no interest. The Gaussian random field method was utilized to correct for multiple comparisons with the REST software (voxel significance: P < 0.001, cluster significance: P < 0.05).
Correlation Analysis
To assess the possible relationship between abnormal ReHo values and illness duration in patients with DED, we conducted Pearson's correlation analyses between the abnormal ReHo values and illness duration (significance level: P < 0.05). In the correlation analysis, the average values of the clusters with abnormal ReHo were used.
Classification Analysis
Classification analysis was performed to examine whether ReHo values and a combination of ReHo values in relevant regions could serve as possible imaging biomarkers to distinguish patients with DED from HCs. SVM analysis was applied to the classification analysis by using the LIBSVM software package (http://www.csie.ntu.edu.tw/~cjlin/libsvm/) in MATLAB. SVM was performed to measure the capacity of the abnormal ReHo values to distinguish patients with DED from HCs. In SVM, differences between groups are learned by a training dataset, and classification performance in unobserved data is evaluated by a test dataset. To train data, we provided label pairs (xi, ci), i = 1, …, l. In the label pairs, , with xi representing ReHo values and ci represents class label. Class label “c = +1” was assigned to the patients with DED, and “c = 0” was assigned to the HCs. We applied Gaussian radial basis function kernel and Grid search method to implement parameter optimization. We also conducted a “leave-one-out” cross-validation approach in the LIBSVM software to obtain the highest specificity and sensitivity. The form of radial basis function kernel used in the present study is “” (Liu et al., 2018).
Results
Characteristics of Participants
A total of 20 patients with DED and 23 HCs were recruited in the study. The two groups did not show significant differences in sex ratio (P = 0.19), age (P = 0.23) or years of education (P = 0.10). The detailed characteristics of the participants are shown in Table 1.
Differences in ReHo Values of Patients With DED and HCs
Relative to the HCs, the patients with DED showed significantly enhanced ReHo in the left inferior occipital gyrus (IOG), left superior temporal gyrus (STG), and right superior medial prefrontal cortex (MPFC), and significantly reduced ReHo in the right superior frontal gyrus (SFG)/middle frontal gyrus (MFG) and bilateral middle cingulum (MC). Detailed information is presented in Table 2 and Figure 1.
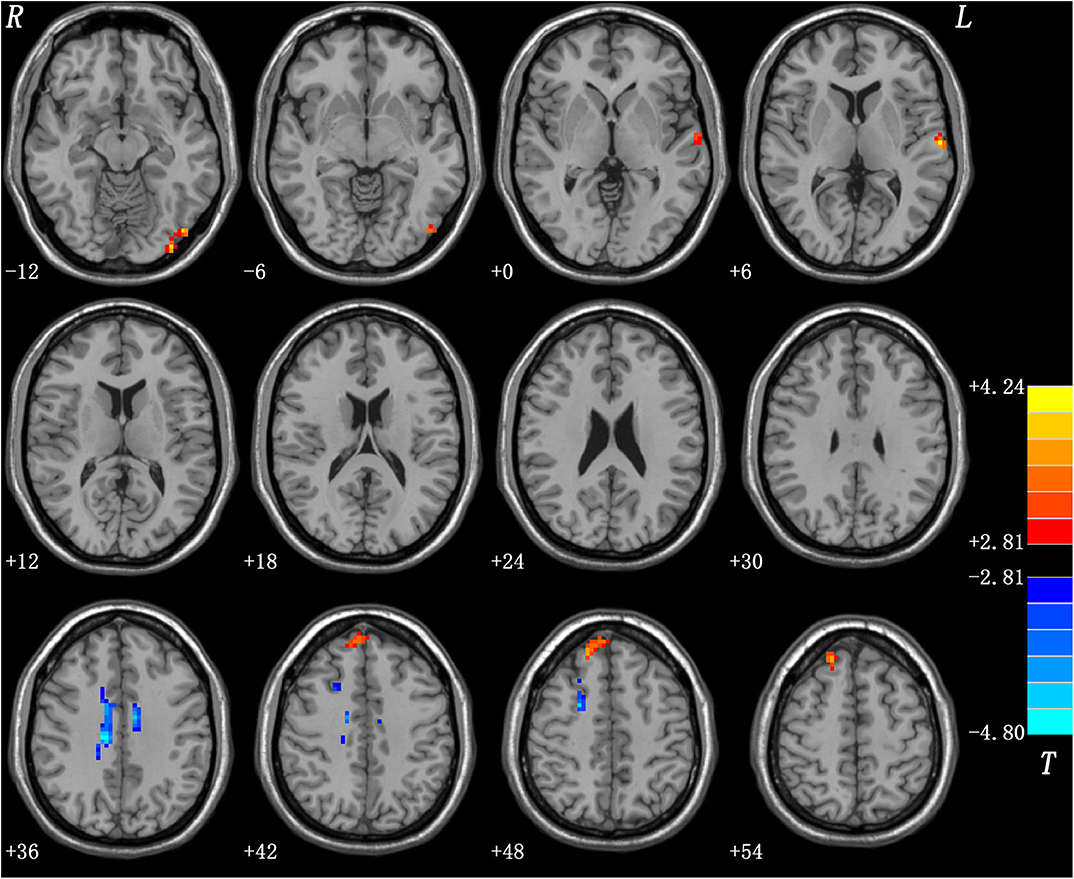
Figure 1. Regions with abnormal ReHo in patients with DED. Red and blue denote increased and decreased ReHo in patients with DED, respectively. DED, dry eye disease; ReHo, regional homogeneity.
Correlation Analysis Result
No correlation was observed between ReHo values and illness duration in patients with DED at the P < 0.05 level.
SVM Result
Figure 2 presents the accuracies for distinguishing patients with DED from HCs based on the ReHo values of six detected brain regions and a combination of these clusters. In the combination of ReHo values in the left MC and left IOG, 40 subjects were correctly classified with the highest accuracy. This combination was the optimal combination with a sensitivity of 95.00%, a specificity of 91.30%, and an accuracy of 93.02% (Figure 3).
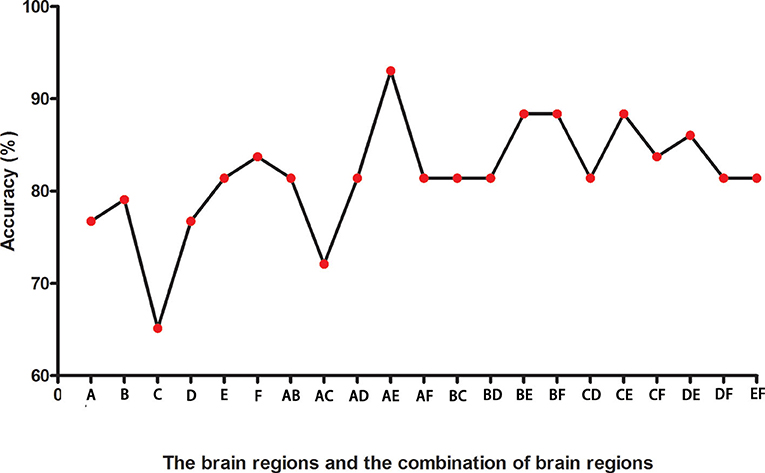
Figure 2. The accuracies in differentiating patients from controls of six brain regions with abnormal ReHo values and combinations of them. A represents the left middle cingulum; B represents the right middle cingulum; C represents the right superior MPFC; D represents the right superior frontal gyrus/middle frontal gyrus; E represents the left inferior occipital gyrus; F represents the left superior temporal gyrus. ReHo, regional homogeneity; MPFC, medial prefrontal cortex.
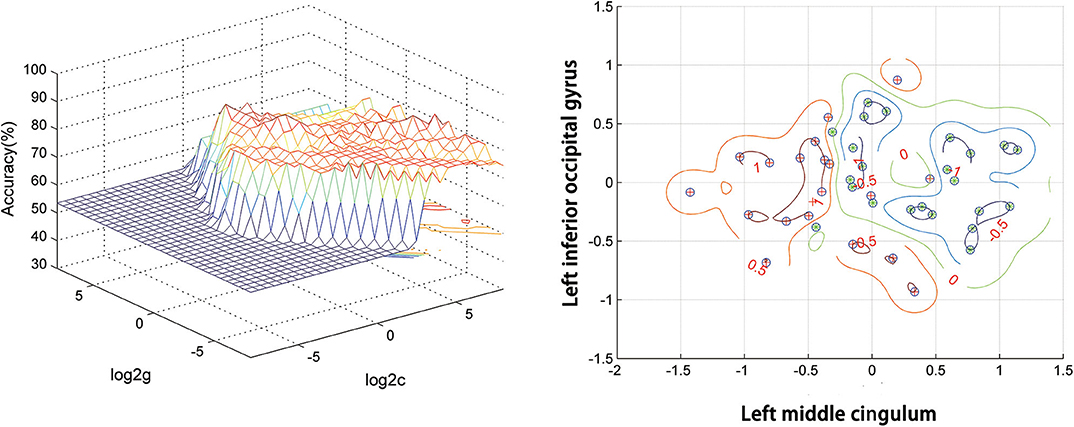
Figure 3. SVM analysis of the combination of ReHo values in the left middle cingulum and the left inferior occipital gyrus. Sensitivity = 95.00%, specificity = 91.30%, and accuracy = 93.02%. SVM, support vector machines; ReHo, regional homogeneity.
Discussion
Relative to the HCs, the patients with DED showed abnormal ReHo in the limbic-cortical circuits. Increased or decreased ReHo values in the patients with DED, relative to the HCs, indicated spontaneous brain activities in certain regions having more or less synchronization. No correlation was found between the ReHo values in these brain regions and illness duration in the patients. Moreover, the SVM analysis showed that a combination of ReHo values in the left MC and left IOG could facilitate the differentiation of the patients with DED from the HCs with satisfactory sensitivities, specificities, and accuracies.
Increased ReHo Values in Left IOG, Left STG, and Right Superior MPFC
The occipital gyrus is the visual cortex, a crucial brain region for visual processing. The IOG plays a critical role in visual processing, particularly in visual processing of faces, and is known as the occipital face area. In neuroimaging studies, participants showed more activity in the IOG when observing faces than when observing other stimuli (Sergent et al., 1992; Liu et al., 2010). A damaged IOG results in the impaired identity recognition of faces (Allison et al., 1994; Rossion et al., 2003). The electrical stimulation of the IOG induces impairments in the perception of facial features and configurations (Pitcher et al., 2007; Jonas et al., 2012). Moreover, the IOG has connections to the limbic system, and a number of studies have shown neural networks consisting of the amygdala and the IOG being responsible for the visual processing of faces (Rossion et al., 2011; Sato et al., 2017). In the present study, we found significantly increased ReHo in the left IOG of the patients with DED relative to the HCs, and this result indicated reinforced activities in this brain area. Reinforced activities in the left IOG enhance visual processing. DED impairs vision, especially functional vision, in patients (Miljanović et al., 2007). Hence, increased ReHo values in the left IOG may compensate for visual impairment in patients with DED.
Repetitive transcranial magnetic stimulation creating “virtual lesions” in the STG results in disturbed visual search (Ellison et al., 2004). Another study applied intraoperative electrical stimulation and found that the inactivation of the STG induces a disturbed visual search (Gharabaghi et al., 2006). Event-related potential (Reale et al., 2007) and fMRI (Robins et al., 2009) studies have suggested that the STG plays an important role in the integration of auditory—visual cues. The STG has connections to the limbic system. The connection between the amygdala and the STG may be correlated with auditory input and the transfer of complex sensory information (Kosmal et al., 1997). Small STG and amygdala were found in patients with schizophrenia relative to HCs, and these features may be correlated with auditory hallucinations (Barta et al., 1990; Yoshida et al., 2009). In the present study, we found increased ReHo values in the left STG of the patients with DED. Therefore, increased ReHo values in the left STG may also account for visual impairment in patients with DED.
MPFC plays a crucial role in fear extinction (Milad and Quirk, 2002; Milad et al., 2004; Santini et al., 2004). A rat under persistent stress shows structural and functional changes in the MPFC (Radley et al., 2006, 2008). The MPFC is a part of the brain's reward system, and stimulating it can induce an antidepressant effect (Tzschentke, 2000; Hamani et al., 2010). The MPFC has connections to the limbic system. In these connections, the amygdala–MPFC circuit is involved in emotional processing (Delli Pizzi et al., 2017; Thijssen et al., 2017). In the present study, we found significantly increased ReHo in the right superior MPFC in the patients with DED. Patients with DED often complain of negative moods, such as depression or anxiety (Li et al., 2011; van der Vaart et al., 2015). Therefore, significantly increased ReHo in the right superior MPFC may be correlated with a negative mood in patients with DED, although anxiety and depression severity were not assessed in the present study.
Decreased ReHo Values in the Right SFG/MFG and Bilateral MC
The right SFG generally plays a crucial role in cognitive control and emotion regulation (Rose et al., 2011; Tully et al., 2014; McDonald et al., 2020). The SFG is also involved in the experience of pain. Fulbright et al. found that the SFG, especially the right SFG, shows pain-related activation when an individual experiences pain stimuli (Fulbright et al., 2001). The frontal cortex and cingulum are parts of the “pain matrix,” which transforms nociceptive signals into a perception of pain and perceived pain intensity (Rainville, 2002; Tracey and Mantyh, 2007; Legrain et al., 2011; Favilla et al., 2014). The MFG and SFG play a vital role in modulating the nociceptive pathways of the cortical and subcortical regions (Yang et al., 2013). Decreased gray matter volume in the MFG (Absinta et al., 2012; Yang et al., 2013) and SFG (Lutz et al., 2008) was observed in painful diseases, such as cluster headaches and fibromyalgia. In the present study, we found significantly decreased ReHo values in the right SFG and MFG in the patients with DED relative to the HCs. In a majority of the patients with DED, ocular pain was a major discomfort. Hence, the dysfunction of the right SFG and MFG may be related to pain in patients with DED.
Mazzola et al. found that the stimulation of the insular cortex can evoke a pain sensation around the eye (Mazzola et al., 2006). A number of studies have found that ocular neurons have projections to the posterior thalamus and the parabrachial area and not to the main somatosensory thalamic areas (Meng et al., 1997; Aicher et al., 2013). Connections exist across the insular cortex, amygdala, and cingulate cortex, thereby suggesting the crucial role of the cerebral limbic system in the autonomic aspects of pain and affection of patients with DED (Bernard et al., 1996). The cingulate cortex is a critical region of the “pain matrix” (Favilla et al., 2014). The MC is a part of the limbic system, which is involved in emotional processing when an individual experiences pain (Zubieta et al., 2003). In the present study, we found significantly decreased ReHo values in the bilateral MC of the patients with DED relative to the HCs. In sum, the decreased ReHo values of the bilateral MC may be related to pain in patients with DED.
In the present study, we found significantly increased ReHo values in the left IOG and left STG, both of which may compensate for visual impairment in patients with DED. Increased ReHo values were also noted in the right superior MPFC, and they may be correlated with a negative mood in patients with DED. The IOG, STG, and MPFC have strong connections to the amygdala. We also found significantly decreased ReHo values in the right SFG/MFG and bilateral MC, and they may be related to the pain of patients with DED; all these four brain regions together make up the “pain matrix.” The amygdala and cingulate gyrus are part of the limbic system. The cerebral limbic system has a key role in the autonomic aspects of pain of DED patients (Bernard et al., 1996). Hence, the abnormal ReHo values found in these brain regions of the patients with DED suggested that limbic-cortical circuits may play a crucial role in the pathophysiology of DED. The dysfunction of the limbic-cortical circuits and DED symptoms may be reciprocal, and the heterogeneity of clinically observed DED symptoms can be explained by the dysfunction of the limbic-cortical circuits in combination with active intrinsic compensatory processes.
A previous study suggested that a specificity or sensitivity of more than 0.7 could be considered as an acceptable level for establishing a diagnostic index (Swets, 1988), whereas a specificity or sensitivity of <0.7 might lead to poor accuracy as a diagnostic indicator (Gong et al., 2011; Zhu et al., 2018; Li et al., 2019). In the present study, the SVM result showed that the combination of ReHo values in the left MC and left IOG in distinguishing patients with DED from HCs had a sensitivity of 95.00%, a specificity of 91.30%, and an accuracy of 93.02%. Therefore, this combination of ReHo values is appropriately applied as a potential image biomarker to distinguish patients with DED from HCs. In the correlation analysis, no correlation was observed between the ReHo values and illness duration of the patients with DED. This result suggested that the illness duration had no effect on the ReHo values.
Several limitations of the present study should be raised. The sample size is small, and it may lead to low-reliability results. Moreover, we cannot divide patients into different subgroups due to the small sample size. Large sample size studies are needed. The MNI template used in the present study was produced from a Caucasian population, and the results may be biased to Chinese subjects.
Conclusion
In the present study, patients with DED had abnormal ReHo values in the limbic-cortical circuits. A combination of ReHo values in the left MC and the left IOG could be applied as potential imaging biomarkers to distinguish patients with DED from HCs. The dysfunction of the limbic-cortical circuits may play an important role in the pathophysiology of DED.
Data Availability Statement
The raw data supporting the conclusions of this article will be made available by the authors, without undue reservation.
Ethics Statement
The studies involving human participants were reviewed and approved by the ethics committee of the First Affiliated Hospital of Guangxi Medical University. The patients/participants provided their written informed consent to participate in this study.
Author Contributions
WG and SL designed the study. SW, FL, WL, YL, XS, and HY collected the original imaging data. WG and FL analyzed the imaging data. WG, XS, and HY wrote the manuscript. All the authors contributed and approved the final manuscript.
Funding
This study was supported by grants from the National Key R&D Program of China (2016YFC1307100), National Natural Science Foundation of China (81771447), the Natural Science Foundation of Hunan (Grant No. 2020JJ4784), and Guangxi Appropriate Technology for Medical and Health Research and Development Project (S201415-05).
Conflict of Interest
The authors declare that the research was conducted in the absence of any commercial or financial relationships that could be construed as a potential conflict of interest.
Acknowledgments
All subjects were acknowledged for participating in this study.
References
Absinta, M., Rocca, M. A., Colombo, B., Falini, A., Comi, G., and Filippi, M. (2012). Selective decreased grey matter volume of the pain-matrix network in cluster headache. Cephalalgia 32, 109–115. doi: 10.1177/0333102411431334
Aicher, S. A., Hermes, S. M., and Hegarty, D. M. (2013). Corneal afferents differentially target thalamic- and parabrachial-projecting neurons in spinal trigeminal nucleus caudalis. Neuroscience 232, 182–193. doi: 10.1016/j.neuroscience.2012.11.033
Akasbi, M., Berenguer, J., Saiz, A., Brito-Zerón, P., Pérez-De-Lis, M., Bové, A., et al. (2012). White matter abnormalities in primary Sjögren syndrome. QJM 105, 433–443. doi: 10.1093/qjmed/hcr218
Allison, T., Ginter, H., McCarthy, G., Nobre, A. C., Puce, A., Luby, M., et al. (1994). Face recognition in human extrastriate cortex. J. Neurophysiol. 71, 821–825. doi: 10.1152/jn.1994.71.2.821
Argüeso, P., Spurr-Michaud, S., Russo, C. L., Tisdale, A., and Gipson, I. K. (2003). MUC16 mucin is expressed by the human ocular surface epithelia and carries the H185 carbohydrate epitope. Invest. Ophthalmol. Vis. Sci. 44, 2487–2495. doi: 10.1167/iovs.02-0862
Barta, P. E., Pearlson, G. D., Powers, R. E., Richards, S. S., and Tune, L. E. (1990). Auditory hallucinations and smaller superior temporal gyral volume in schizophrenia. Am. J. Psychiatry 147, 1457–1462. doi: 10.1176/ajp.147.11.1457
Belmonte, C., Acosta, M. C., Merayo-Lloves, J., and Gallar, J. (2015). What causes eye pain? Curr. Ophthalmol. Rep. 3, 111–121. doi: 10.1007/s40135-015-0073-9
Belmonte, C., Nichols, J. J., Cox, S. M., Brock, J. A., Begley, C. G., Bereiter, D. A., et al. (2017). TFOS DEWS II pain and sensation report. Ocul. Surf. 15, 404–437. doi: 10.1016/j.jtos.2017.05.002
Benito, A., Pérez, G. M., Mirabet, S., Vilaseca, M., Pujol, J., Marín, J. M., et al. (2011). Objective optical assessment of tear-film quality dynamics in normal and mildly symptomatic dry eyes. J. Cataract Refract. Surg. 37, 1481–1487. doi: 10.1016/j.jcrs.2011.03.036
Bernard, J. F., Bester, H., and Besson, J. M. (1996). Involvement of the spino-parabrachio -amygdaloid and -hypothalamic pathways in the autonomic and affective emotional aspects of pain. Prog. Brain Res. 107, 243–255. doi: 10.1016/S0079-6123(08)61868-3
Biswal, B., Yetkin, F. Z., Haughton, V. M., and Hyde, J. S. (1995). Functional connectivity in the motor cortex of resting human brain using echo-planar MRI. Magn. Reson. Med. 34, 537–541. doi: 10.1002/mrm.1910340409
Chen, H., and Chen, L. (2017). Support vector machine classification of drunk driving behaviour. Int. J. Environ. Res. Public Health 14:108. doi: 10.3390/ijerph14010108
Chen, W., Zhang, L., Xu, Y. G., Zhu, K., and Luo, M. (2017). Primary angle-closure glaucomas disturb regional spontaneous brain activity in the visual pathway: an fMRI study. Neuropsychiatr. Dis. Treat. 13, 1409–1417. doi: 10.2147/NDT.S134258
Craig, J. P., Nichols, K. K., Akpek, E. K., Caffery, B., Dua, H. S., Joo, C. K., et al. (2017). TFOS DEWS II definition and classification report. Ocul. Surf. 15, 276–283. doi: 10.1016/j.jtos.2017.05.008
Dartt, D. A. (2009). Neural regulation of lacrimal gland secretory processes: relevance in dry eye diseases. Prog. Retin. Eye Res. 28, 155–177. doi: 10.1016/j.preteyeres.2009.04.003
Delli Pizzi, S., Chiacchiaretta, P., Mantini, D., Bubbico, G., Ferretti, A., Edden, R. A., et al. (2017). Functional and neurochemical interactions within the amygdala-medial prefrontal cortex circuit and their relevance to emotional processing. Brain Struct. Funct. 222, 1267–1279. doi: 10.1007/s00429-016-1276-z
Ellingham, R. B., Berry, M., Stevenson, D., and Corfield, A. P. (1999). Secreted human conjunctival mucus contains MUC5AC glycoforms. Glycobiology 9, 1181–1189. doi: 10.1093/glycob/9.11.1181
Ellison, A., Schindler, I., Pattison, L. L., and Milner, A. D. (2004). An exploration of the role of the superior temporal gyrus in visual search and spatial perception using TMS. Brain 127(Pt 10), 2307–2315. doi: 10.1093/brain/awh244
Favilla, S., Huber, A., Pagnoni, G., Lui, F., Facchin, P., Cocchi, M., et al. (2014). Ranking brain areas encoding the perceived level of pain from fMRI data. Neuroimage 90, 153–162. doi: 10.1016/j.neuroimage.2014.01.001
Fulbright, R. K., Troche, C. J., Skudlarski, P., Gore, J. C., and Wexler, B. E. (2001). Functional MR imaging of regional brain activation associated with the affective experience of pain. AJR Am. J. Roentgenol. 177, 1205–1210. doi: 10.2214/ajr.177.5.1771205
Galor, A., Levitt, R. C., Felix, E. R., Martin, E. R., and Sarantopoulos, C. D. (2015). Neuropathic ocular pain: an important yet underevaluated feature of dry eye. Eye 29, 301–312. doi: 10.1038/eye.2014.263
Gharabaghi, A., Fruhmann Berger, M., Tatagiba, M., and Karnath, H. O. (2006). The role of the right superior temporal gyrus in visual search-insights from intraoperative electrical stimulation. Neuropsychologia 44, 2578–2581. doi: 10.1016/j.neuropsychologia.2006.04.006
Gong, Q., Wu, Q., Scarpazza, C., Lui, S., Jia, Z., Marquand, A., et al. (2011). Prognostic prediction of therapeutic response in depression using high-field MR imaging. Neuroimage 55, 1497–1503. doi: 10.1016/j.neuroimage.2010.11.079
Hamani, C., Diwan, M., Macedo, C. E., Brandão, M. L., Shumake, J., Gonzalez-Lima, F., et al. (2010). Antidepressant-like effects of medial prefrontal cortex deep brain stimulation in rats. Biol. Psychiatry 67, 117–124. doi: 10.1016/j.biopsych.2009.08.025
Jonas, J., Descoins, M., Koessler, L., Colnat-Coulbois, S., Sauvée, M., Guye, M., et al. (2012). Focal electrical intracerebral stimulation of a face-sensitive area causes transient prosopagnosia. Neuroscience 222, 281–288. doi: 10.1016/j.neuroscience.2012.07.021
Kalangara, J. P., Galor, A., Levitt, R. C., Felix, E. R., Alegret, R., and Sarantopoulos, C. D. (2016). Burning eye syndrome: do neuropathic pain mechanisms underlie chronic dry eye? Pain Med. 17, 746–755. doi: 10.1093/pm/pnv070
Kaminer, J., Powers, A. S., Horn, K. G., Hui, C., and Evinger, C. (2011). Characterizing the spontaneous blink generator: an animal model. J. Neurosci. 31, 11256–11267. doi: 10.1523/JNEUROSCI.6218-10.2011
Khasabov, S. G., Malecha, P., Noack, J., Tabakov, J., Okamoto, K., Bereiter, D. A., et al. (2015). Activation of rostral ventromedial medulla neurons by noxious stimulation of cutaneous and deep craniofacial tissues. J. Neurophysiol. 113, 14–22. doi: 10.1152/jn.00125.2014
Kosmal, A., Malinowska, M., and Kowalska, D. M. (1997). Thalamic and amygdaloid connections of the auditory association cortex of the superior temporal gyrus in rhesus monkey (Macaca mulatta). Acta Neurobiol. Exp. 57, 165–188.
Latremoliere, A., and Woolf, C. J. (2009). Central sensitization: a generator of pain hypersensitivity by central neural plasticity. J. Pain 10, 895–926. doi: 10.1016/j.jpain.2009.06.012
Lauvsnes, M. B., Beyer, M. K., Appenzeller, S., Greve, O. J., Harboe, E., Gøransson, L. G., et al. (2014). Loss of cerebral white matter in primary Sjögren's syndrome: a controlled volumetric magnetic resonance imaging study. Eur. J. Neurol. 21, 1324–1329. doi: 10.1111/ene.12486
Legrain, V., Iannetti, G. D., Plaghki, L., and Mouraux, A. (2011). The pain matrix reloaded: a salience detection system for the body. Prog. Neurobiol. 93, 111–124. doi: 10.1016/j.pneurobio.2010.10.005
Lemp, M. A. (2007). The definition and classification of dry eye disease: report of the definition and classification subcommittee of the international dry eye workShop 2007. Ocul. Surf. 5, 75–92. doi: 10.1016/S1542-0124(12)70081-2
Levitt, A. E., Galor, A., Chowdhury, A. R., Felix, E. R., Sarantopoulos, C. D., Zhuang, G. Y., et al. (2017). Evidence that dry eye represents a chronic overlapping pain condition. Mol. Pain 13:1744806917729306. doi: 10.1177/1744806917729306
Li, D., Yang, W., and Wang, S. (2010). Classification of foreign fibers in cotton lint using machine vision and multi-class support vector machine. Comput. Electron. Agric. 74, 274–279. doi: 10.1016/j.compag.2010.09.002
Li, H., Guo, W., Liu, F., Chen, J., Su, Q., Zhang, Z., et al. (2019). Enhanced baseline activity in the left ventromedial putamen predicts individual treatment response in drug-naive, first-episode schizophrenia: results from two independent study samples. EBioMedicine 46, 248–255. doi: 10.1016/j.ebiom.2019.07.022
Li, M., Gong, L., Sun, X., and Chapin, W. J. (2011). Anxiety and depression in patients with dry eye syndrome. Curr. Eye Res. 36, 1–7. doi: 10.3109/02713683.2010.519850
Lin, X., Ding, K., Liu, Y., Yan, X., Song, S., and Jiang, T. (2012). Altered spontaneous activity in anisometropic amblyopia subjects: revealed by resting-state FMRI. PLoS ONE 7:e43373. doi: 10.1371/journal.pone.0043373
Liu, J., Harris, A., and Kanwisher, N. (2010). Perception of face parts and face configurations: an FMRI study. J. Cogn. Neurosci. 22, 203–211. doi: 10.1162/jocn.2009.21203
Liu, Y., Zhang, Y., Lv, L., Wu, R., Zhao, J., and Guo, W. (2018). Abnormal neural activity as a potential biomarker for drug-naive first-episode adolescent-onset schizophrenia with coherence regional homogeneity and support vector machine analyses. Schizophr. Res. 192, 408–415. doi: 10.1016/j.schres.2017.04.028
Lutz, J., Jäger, L., de Quervain, D., Krauseneck, T., Padberg, F., Wichnalek, M., et al. (2008). White and gray matter abnormalities in the brain of patients with fibromyalgia: a diffusion-tensor and volumetric imaging study. Arthritis Rheum. 58, 3960–3969. doi: 10.1002/art.24070
Mantelli, F., and Argüeso, P. (2008). Functions of ocular surface mucins in health and disease. Curr. Opin. Allergy Clin. Immunol. 8, 477–483. doi: 10.1097/ACI.0b013e32830e6b04
Mathers, W. D. (2000). Why the eye becomes dry: a cornea and lacrimal gland feedback model. CLAO J. 26, 159–165.
Mazzola, L., Isnard, J., and Mauguière, F. (2006). Somatosensory and pain responses to stimulation of the second somatosensory area (SII) in humans. A comparison with SI and insular responses. Cereb. Cortex 16, 960–968. doi: 10.1093/cercor/bhj038
McDonald, B., Becker, K., Meshi, D., Heekeren, H. R., and von Scheve, C. (2020). Individual differences in envy experienced through perspective-taking involves functional connectivity of the superior frontal gyrus. Cogn. Affect. Behav. Neurosci. 20, 783–797. doi: 10.3758/s13415-020-00802-8
Meng, I. D., Hu, J. W., Benetti, A. P., and Bereiter, D. A. (1997). Encoding of corneal input in two distinct regions of the spinal trigeminal nucleus in the rat: cutaneous receptive field properties, responses to thermal and chemical stimulation, modulation by diffuse noxious inhibitory controls, and projections to the parabrachial area. J. Neurophysiol. 77, 43–56. doi: 10.1152/jn.1997.77.1.43
Mertzanis, P., Abetz, L., Rajagopalan, K., Espindle, D., Chalmers, R., Snyder, C., et al. (2005). The relative burden of dry eye in patients' lives: comparisons to a U.S. normative sample. Invest Ophthalmol. Vis. Sci. 46, 46–50. doi: 10.1167/iovs.03-0915
Messmer, E. M. (2015). The pathophysiology, diagnosis, and treatment of dry eye disease. Dtsch. Arztebl. Int. 112, 71–81, quiz 82. doi: 10.3238/arztebl.2015.0071
Milad, M. R., and Quirk, G. J. (2002). Neurons in medial prefrontal cortex signal memory for fear extinction. Nature 420, 70–74. doi: 10.1038/nature01138
Milad, M. R., Vidal-Gonzalez, I., and Quirk, G. J. (2004). Electrical stimulation of medial prefrontal cortex reduces conditioned fear in a temporally specific manner. Behav. Neurosci. 118, 389–394. doi: 10.1037/0735-7044.118.2.389
Miljanović, B., Dana, R., Sullivan, D. A., and Schaumberg, D. A. (2007). Impact of dry eye syndrome on vision-related quality of life. Am. J. Ophthalmol. 143, 409–415. doi: 10.1016/j.ajo.2006.11.060
Moss, S. E., Klein, R., and Klein, B. E. (2000). Prevalence of and risk factors for dry eye syndrome. Arch. Ophthalmol. 118, 1264–1268. doi: 10.1001/archopht.118.9.1264
Nakamori, K., Odawara, M., Nakajima, T., Mizutani, T., and Tsubota, K. (1997). Blinking is controlled primarily by ocular surface conditions. Am. J. Ophthalmol. 124, 24–30. doi: 10.1016/S0002-9394(14)71639-3
Ordovas-Montanes, J., Rakoff-Nahoum, S., Huang, S., Riol-Blanco, L., Barreiro, O., and von Andrian, U. H. (2015). The regulation of immunological processes by peripheral neurons in homeostasis and disease. Trends Immunol. 36, 578–604. doi: 10.1016/j.it.2015.08.007
Pitcher, D., Walsh, V., Yovel, G., and Duchaine, B. (2007). TMS evidence for the involvement of the right occipital face area in early face processing. Curr. Biol. 17, 1568–1573. doi: 10.1016/j.cub.2007.07.063
Radley, J. J., Rocher, A. B., Miller, M., Janssen, W. G., Liston, C., Hof, P. R., et al. (2006). Repeated stress induces dendritic spine loss in the rat medial prefrontal cortex. Cereb. Cortex 16, 313–320. doi: 10.1093/cercor/bhi104
Radley, J. J., Rocher, A. B., Rodriguez, A., Ehlenberger, D. B., Dammann, M., McEwen, B. S., et al. (2008). Repeated stress alters dendritic spine morphology in the rat medial prefrontal cortex. J. Comp. Neurol. 507, 1141–1150. doi: 10.1002/cne.21588
Rahman, M., Okamoto, K., Thompson, R., Katagiri, A., and Bereiter, D. A. (2015). Sensitization of trigeminal brainstem pathways in a model for tear deficient dry eye. Pain 156, 942–950. doi: 10.1097/j.pain.0000000000000135
Rainville, P. (2002). Brain mechanisms of pain affect and pain modulation. Curr. Opin. Neurobiol. 12, 195–204. doi: 10.1016/S0959-4388(02)00313-6
Reale, R. A., Calvert, G. A., Thesen, T., Jenison, R. L., Kawasaki, H., Oya, H., et al. (2007). Auditory-visual processing represented in the human superior temporal gyrus. Neuroscience 145, 162–184. doi: 10.1016/j.neuroscience.2006.11.036
Robins, D. L., Hunyadi, E., and Schultz, R. T. (2009). Superior temporal activation in response to dynamic audio-visual emotional cues. Brain Cogn. 69, 269–278. doi: 10.1016/j.bandc.2008.08.007
Rose, J. E., McClernon, F. J., Froeliger, B., Behm, F. M., Preud'homme, X., and Krystal, A. D. (2011). Repetitive transcranial magnetic stimulation of the superior frontal gyrus modulates craving for cigarettes. Biol. Psychiatry 70, 794–799. doi: 10.1016/j.biopsych.2011.05.031
Rossion, B., Caldara, R., Seghier, M., Schuller, A. M., Lazeyras, F., and Mayer, E. (2003). A network of occipito-temporal face-sensitive areas besides the right middle fusiform gyrus is necessary for normal face processing. Brain 126(Pt 11), 2381–2395. doi: 10.1093/brain/awg241
Rossion, B., Dricot, L., Goebel, R., and Busigny, T. (2011). Holistic face categorization in higher order visual areas of the normal and prosopagnosic brain: toward a non-hierarchical view of face perception. Front. Hum. Neurosci. 4:225. doi: 10.3389/fnhum.2010.00225
Santini, E., Ge, H., Ren, K., Peña de Ortiz, S., and Quirk, G. J. (2004). Consolidation of fear extinction requires protein synthesis in the medial prefrontal cortex. J. Neurosci. 24, 5704–5710. doi: 10.1523/JNEUROSCI.0786-04.2004
Sato, W., Kochiyama, T., Uono, S., Matsuda, K., Usui, K., Usui, N., et al. (2017). Bidirectional electric communication between the inferior occipital gyrus and the amygdala during face processing. Hum. Brain Mapp. 38, 4511–4524. doi: 10.1002/hbm.23678
Schaumberg, D. A., Sullivan, D. A., Buring, J. E., and Dana, M. R. (2003). Prevalence of dry eye syndrome among US women. Am. J. Ophthalmol. 136, 318–326. doi: 10.1016/S0002-9394(03)00218-6
Sergent, J., Ohta, S., and MacDonald, B. (1992). Functional neuroanatomy of face and object processing. A positron emission tomography study. Brain 115(Pt 1), 15–36. doi: 10.1093/brain/115.1.15
Smith, J. A. (2007). The epidemiology of dry eye disease: report of the epidemiology subcommittee of the international dry eye workshop 2007. Ocul. Surf. 5, 93–107. doi: 10.1016/S1542-0124(12)70082-4
Swets, J. A. (1988). Measuring the accuracy of diagnostic systems. Science 240, 1285–1293. doi: 10.1126/science.3287615
Tan, C. H., Labbé, A., Liang, Q., Qiao, L., Baudouin, C., Wan, X., et al. (2015). Dynamic change of optical quality in patients with dry eye disease. Invest. Ophthalmol. Vis. Sci. 56, 2848–2854. doi: 10.1167/iovs.14-15757
Thijssen, S., Muetzel, R. L., Bakermans-Kranenburg, M. J., Jaddoe, V. W., Tiemeier, H., Verhulst, F. C., et al. (2017). Insensitive parenting may accelerate the development of the amygdala-medial prefrontal cortex circuit. Dev. Psychopathol. 29, 505–518. doi: 10.1017/S0954579417000141
Tracey, I., and Mantyh, P. W. (2007). The cerebral signature for pain perception and its modulation. Neuron 55, 377–391. doi: 10.1016/j.neuron.2007.07.012
Tulleuda, A., Cokic, B., Callejo, G., Saiani, B., Serra, J., and Gasull, X. (2011). TRESK channel contribution to nociceptive sensory neurons excitability: modulation by nerve injury. Mol. Pain 7:30. doi: 10.1186/1744-8069-7-30
Tully, L. M., Lincoln, S. H., Liyanage-Don, N., and Hooker, C. I. (2014). Impaired cognitive control mediates the relationship between cortical thickness of the superior frontal gyrus and role functioning in schizophrenia. Schizophr. Res. 152, 358–364. doi: 10.1016/j.schres.2013.12.005
Tzarouchi, L. C., Tsifetaki, N., Konitsiotis, S., Zikou, A., Astrakas, L., Drosos, A., et al. (2011). CNS involvement in primary Sjogren syndrome: assessment of gray and white matter changes with MRI and voxel-based morphometry. AJR Am. J. Roentgenol. 197, 1207–1212. doi: 10.2214/AJR.10.5984
Tzarouchi, L. C., Zikou, A. K., Tsifetaki, N., Astrakas, L. G., Konitsiotis, S., Voulgari, P., et al. (2014). White matter water diffusion changes in primary Sjögren syndrome. AJNR Am. J. Neuroradiol. 35, 680–685. doi: 10.3174/ajnr.A3756
Tzschentke, T. M. (2000). The medial prefrontal cortex as a part of the brain reward system. Amino Acids 19, 211–219. doi: 10.1007/s007260070051
van Bijsterveld, O. P., Kruize, A. A., and Bleys, R. L. (2003). Central nervous system mechanisms in Sjögren's syndrome. Br. J. Ophthalmol. 87, 128–130. doi: 10.1136/bjo.87.2.128
van der Vaart, R., Weaver, M. A., Lefebvre, C., and Davis, R. M. (2015). The association between dry eye disease and depression and anxiety in a large population-based study. Am. J. Ophthalmol. 159, 470–474. doi: 10.1016/j.ajo.2014.11.028
von Hehn, C. A., Baron, R., and Woolf, C. J. (2012). Deconstructing the neuropathic pain phenotype to reveal neural mechanisms. Neuron 73, 638–652. doi: 10.1016/j.neuron.2012.02.008
Willcox, M. D. P., Argüeso, P., Georgiev, G. A., Holopainen, J. M., Laurie, G. W., Millar, T. J., et al. (2017). TFOS DEWS II tear film report. Ocul. Surf. 15, 366–403. doi: 10.1016/j.jtos.2017.03.006
Xing, W., Shi, W., Leng, Y., Sun, X., Guan, T., Liao, W., et al. (2018). Resting-state fMRI in primary Sjögren syndrome. Acta Radiol. 59, 1091–1096. doi: 10.1177/0284185117749993
Xu, M. W., Liu, H. M., Tan, G., Su, T., Xiang, C. Q., Wu, W., et al. (2019). Altered regional homogeneity in patients with corneal ulcer: a resting-state functional MRI study. Front. Neurosci. 13:743. doi: 10.3389/fnins.2019.00743
Yang, F. C., Chou, K. H., Fuh, J. L., Huang, C. C., Lirng, J. F., Lin, Y. Y., et al. (2013). Altered gray matter volume in the frontal pain modulation network in patients with cluster headache. Pain 154, 801–807. doi: 10.1016/j.pain.2013.02.005
Yoshida, T., McCarley, R. W., Nakamura, M., Lee, K., Koo, M. S., Bouix, S., et al. (2009). A prospective longitudinal volumetric MRI study of superior temporal gyrus gray matter and amygdala-hippocampal complex in chronic schizophrenia. Schizophr. Res. 113, 84–94. doi: 10.1016/j.schres.2009.05.004
Zang, Y., Jiang, T., Lu, Y., He, Y., and Tian, L. (2004). Regional homogeneity approach to fMRI data analysis. Neuroimage 22, 394–400. doi: 10.1016/j.neuroimage.2003.12.030
Zhang, X. D., Zhao, L. R., Zhou, J. M., Su, Y. Y., Ke, J., Cheng, Y., et al. (2020). Altered hippocampal functional connectivity in primary Sjögren syndrome: a resting-state fMRI study. Lupus 29, 446–454. doi: 10.1177/0961203320908936
Zhang, Y., and Wu, L. (2012). Classification of fruits using computer vision and a multiclass support vector machine. Sensors 12, 12489–12505. doi: 10.3390/s120912489
Zhu, F., Liu, F., Guo, W., Chen, J., Su, Q., Zhang, Z., et al. (2018). Disrupted asymmetry of inter- and intra-hemispheric functional connectivity in patients with drug-naive, first-episode schizophrenia and their unaffected siblings. EBioMedicine 36, 429–435. doi: 10.1016/j.ebiom.2018.09.012
Keywords: dry eye disease, resting-state functional magnetic resonance imaging, regional homogeneity, support vector machine, limbic-cortical circuits
Citation: Yan H, Shan X, Wei S, Liu F, Li W, Lei Y, Guo W and Luo S (2020) Abnormal Spontaneous Brain Activities of Limbic-Cortical Circuits in Patients With Dry Eye Disease. Front. Hum. Neurosci. 14:574758. doi: 10.3389/fnhum.2020.574758
Received: 21 June 2020; Accepted: 06 October 2020;
Published: 09 November 2020.
Edited by:
Wei Liao, University of Electronic Science and Technology of China, ChinaReviewed by:
Qiu Jiang, Southwest Normal University, ChinaYong Xu, First Hospital of Shanxi Medical University, China
Copyright © 2020 Yan, Shan, Wei, Liu, Li, Lei, Guo and Luo. This is an open-access article distributed under the terms of the Creative Commons Attribution License (CC BY). The use, distribution or reproduction in other forums is permitted, provided the original author(s) and the copyright owner(s) are credited and that the original publication in this journal is cited, in accordance with accepted academic practice. No use, distribution or reproduction is permitted which does not comply with these terms.
*Correspondence: Wenbin Guo, guowenbin76@csu.edu.cn; Shuguang Luo, robert58243@sohu.com