- 1Department of Neurology, Norman Fixel Institute for Neurological Diseases, University of Florida, Gainesville, FL, United States
- 2Department of Neurology, Nagoya University Graduate School of Medicine, Nagoya, Japan
- 3Department of Neurology, University Hospital Montpellier, Montpellier, France
- 4Department of Neurosurgery, University Hospital Montpellier, Montpellier, France
- 5Department of Neurology, University of California, San Francisco, San Francisco, CA, United States
- 6Department of Stereotactic and Functional Neurosurgery, Fukuoka Mirai Hospital, Fukuoka, Japan
- 7Division of Neurosurgery, Toronto Western Hospital Krembil Neuroscience Center, Toronto, ON, Canada
- 8Department of Neurosurgery, University of Toronto, Toronto, ON, Canada
- 9Department of Neurosurgery, KU Leuven, Leuven, Belgium
- 10Department of Neurology, Wake Forest School of Medicine, Winston-Salem, NC, United States
- 11Beijing Neurosurgical Institute, Beijing Tiantan Hospital, Capital Medical University, Beijing, China
- 12Department of Neurology, Massachusetts General Hospital, Charlestown, MA, United States
- 13Department of Applied Physiology and Kinesiology, University of Florida, Gainesville, FL, United States
- 14Department of Neurosurgery, Norman Fixel Institute for Neurological Diseases, University of Florida, Gainesville, FL, United States
Objective: To reveal clinical characteristics of suboptimal responses to deep brain stimulation (DBS) in a multi-country DYT1 dystonia cohort.
Methods: In this multi-country multi-center retrospective study, we analyzed the clinical data of DYT1 patients who experienced suboptimal responses to DBS defined as <30% improvement in dystonia scales at the last follow-up compared with baseline. We used a literature-driven historical cohort of 112 DYT1 patients for comparison.
Results: Approximately 8% of our study cohort (11 out of 132) experienced suboptimal responses to DBS. Compared with the historical cohort, the multi-country cohort with suboptimal responses had a significantly younger age at onset (mean, 7.0 vs. 8.4 years; p = 0.025) and younger age at DBS (mean, 12.0 vs. 18.6 years; p = 0.019). Additionally, cranial involvement was more common in the multi-country cohort (before DBS, 64% vs. 45%, p = 0.074; before or after DBS, 91% vs. 47%, p = 0.001). Mean motor improvement at the last follow-up from baseline were 0% and 66% for the multi-country and historical cohorts, respectively. All 11 patients of the multi-country cohort had generalization of dystonia within 2.5 years after disease onset. All patients experienced dystonia improvement of >30% postoperatively; however, secondary worsening of dystonia commenced between 6 months and 3 years following DBS. The improvement at the last follow-up was less than 30% despite optimally-placed leads, a trial of multiple programming settings, and additional DBS surgeries in all patients. The on-/off-stimulation comparison at the long-term follow-up demonstrated beneficial effects of DBS despite missing the threshold of 30% improvement over baseline.
Conclusion: Approximately 8% of patients represent a more aggressive phenotype of DYT1 dystonia characterized by younger age at onset, faster disease progression, and cranial involvement, which seems to be associated with long-term suboptimal responses to DBS (e.g., secondary worsening). This information could be useful for both clinicians and patients in clinical decision making and patient counseling before and following DBS implantations. Patients with this phenotype may have different neuroplasticity, neurogenetics, or possibly distinct neurophysiology.
Introduction
DYT1 (DYT-TOR1A) is the most common cause of inherited isolated dystonia, and almost all patients possess the same mutation in the TOR1A gene (c.907_909delGAG; Charlesworth et al., 2013). Dystonia symptoms of DYT1 patients most often begin in an arm or leg during childhood or adolescence (Lee et al., 2012). Dystonia spreads to other body regions and becomes generalized over months to years in up to half of patients (Fasano et al., 2006; Lee et al., 2012). Cranial involvement is however uncommon.
Globus pallidus internus deep brain stimulation (GPi DBS) improves motor function and quality of life in dystonia patients and is considered a therapeutic option for patients with medically-refractory dystonia (Vidailhet et al., 2005; Volkmann et al., 2012; Tsuboi et al., 2019). In 2000, excellent DBS outcomes in patients with DYT1 were first reported by Coubes et al. (2000). Subsequently, retrospective studies and a meta-analysis suggested that TOR1A mutation-positive status was a clinical predictive factor for a better DBS outcome (Vasques et al., 2009; Andrews et al., 2010; Borggraefe et al., 2010; FitzGerald et al., 2014; Moro et al., 2017; Artusi et al., 2020). Additionally, in the largest cohort to date of patients with DYT1 dystonia who underwent GPi DBS (n = 47), improvement in dystonia severity by >80% was maintained up to 7 years after surgery (Panov et al., 2013). In contrast, there have been a minority of individual DYT1 cases with suboptimal responses to GPi DBS (Krause et al., 2004, 2016; Starr et al., 2006; Mehrkens et al., 2009; Cif et al., 2010; Markun et al., 2012; Miyagi and Koike, 2013; Ben-Haim et al., 2016; Pauls et al., 2017; Tsuboi et al., 2019). These less-than-expected responses in some cases could be attributable to suboptimal lead positions, non-optimized programming, or skeletal deformities. However, there are cases with suboptimal outcomes that remain unexplained, and there seem to be emerging cases of secondary worsening (Cif et al., 2010; Miyagi and Koike, 2013; Tsuboi et al., 2019). Because only a few cases of DYT1 dystonia are operated each year even in expert centers, the number of reported patients with suboptimal responses is limited. Consequently, the clinical characteristics of this cohort remain undefined.
We performed a multi-country multi-center retrospective study to investigate the characteristics of patients with DYT1 dystonia who experienced suboptimal responses to DBS. Additionally, we compared this unique cohort of patients to a historical cohort of DYT1 DBS patients to better define the potential characteristics which may differentiate responses.
Materials and Methods
Study Design
This retrospective study was approved by the University of Florida Institutional Review Board (IRB201900356), and each participating center had local IRB approval for the inclusion of their data in this study. We collected patient information that met the following inclusion criteria: (1) isolated dystonia; (2) a c.907_909delGAG mutation in the TOR1A gene; (3) bilateral GPi DBS performed at one of the participating expert centers; (4) no history of prior stereotactic brain surgery other than DBS; (5) preoperative clinical assessments with postoperative assessments at 1 year or longer; and (6) suboptimal responses to DBS (defined as <30% improvement in the Burke-Fahn-Marsden Dystonia Rating Scale motor score (BFMDRS-M) or the Unified Dystonia Rating Scale (UDRS) at the last follow-up compared with the baseline status (Burke et al., 1985; Comella et al., 2003). This 30% threshold was chosen arbitrarily as a clinically relevant response (Pauls et al., 2017). Earlier studies reported an excellent correlation between the BFMDRS-M and UDRS (0.977) and that percentage improvement after DBS in dystonia patients was similar between these scales (Comella et al., 2003; Susatia et al., 2010). Therefore, we considered percentage improvement based on these scales equivalent. The DBS programming data, the DBS lead positions, and the changes in motor scores, as well as detailed descriptive clinical information, were collected and analyzed.
The Historical Cohort
To create a historical cohort of DYT1 patients treated with GPi DBS, a literature search was conducted using PubMed/Medline, Embase, and Cochrane databases in May 2019 with the following search terms: deep brain stimulation, neurostimulation, DBS, DYT1, and TOR1A. The search syntax is available in Supplementary Material. The reference lists for each of the identified articles were used to explore additional relevant publications. We considered both pure DYT1 cohorts and mixed cohorts including DYT1 patients for inclusion. The inclusion criteria were as follows: (1) DYT1 dystonia; (2) bilateral GPi DBS; (3) no history of prior stereotactic brain surgery other than DBS; and (4) preoperative clinical assessments with postoperative assessments at 1 year or longer. The minimum required information for individual patients was the age at onset, the age at DBS surgery, disease duration before DBS, and preoperative and postoperative motor scores according to either the BFMDRS-M or the UDRS. For articles in which only two of the following were provided (i.e., age at onset, age at DBS, and disease duration before DBS), the missing value was calculated using the other values. For articles presenting motor scores only in line graphs, we extracted the data from the figures using a graph digitizer software (Plot digitizer)1. We identified and excluded all possible duplicated patients by examining demographics, motor scores, and the authors’ affiliations.
Statistical Analysis
Normal distribution of data was tested using the Shapiro–Wilk test. We compared the demographics and outcome measurements between the groups using independent t-tests, Mann–Whitney U tests, chi-square tests, or Fisher’s exact tests, as appropriate. Statistical significance was set at p ≤ 0.05. All analyses were performed using IBM SPSS statistics 25 (IBM Corp., Armonk, NY, USA).
Results
The Multi-country Cohort
From a total of 132 DYT1 patients who underwent DBS in our institutions, we collected the detailed clinical information of 18 DYT1 patients with reported suboptimal DBS responses. However, a thorough review of the case files led to an additional seven exclusions. Patients 12 and 13 had suboptimal responses to DBS as a result of suboptimal lead positions, and patient 14 had a secondary worsening of dystonia because of lead migration. These three patients revealed marked improvement following the surgical repositioning of the DBS leads. Patients 15, 16, and 17 had excellent responses to DBS (>80%) followed by worsening of dystonia; however, these patients had persistent improvement ≥30% at the last follow-up. Despite a marked improvement in the BFMDRS-M, functional improvement in patients 15 and 18 was compromised by skeletal deformities and action-induced muscle spasms, respectively. The clinical information of patients 12–18 is presented in Supplementary Table S1.
Compared to the preoperative baseline status, the remaining 11 patients (Table 1) had improvement that was less than 30% (range −81.8% to 29.7%) at the last clinical follow-up with a mean follow-up duration after implantation of 12.6 years (range 1.0–18.3 years). Nine patients had a family history of dystonia. The region of onset was the upper extremity in four patients and the lower extremity in seven with a mean age at onset of 6.9 years (range 5–10 years). In all patients, the dystonia became generalized within 2.5 years (mean 1.9 years, range 0.8–2.5 years), and the patients underwent DBS at a mean age of 12.5 years (range 8–23 years) with a mean disease duration before DBS of 5.6 years (range 2–17 years). All of the patients were initially treated with bilateral GPi DBS. Individual changes in the BFMDRS-M/UDRS are summarized in Figure 1A. Importantly, all patients showed motor improvement of ≥30% postoperatively (mean improvement of 59% at 6 months) but subsequently experienced secondary worsening of dystonia symptoms starting between 6 months and 3 years after DBS. Maximal motor improvement was observed between 6 months and 2 years with the improvement of 30–50% in four patients, 50–80% in four, and >80% in three. Cranial involvement was observed in six patients before surgery, and four patients developed cranial dystonia following surgery. Pseudo-dystonic orofacial movement or speech disorders due to the current spread into the surrounding structures were carefully ruled out by the participating expert DBS centers by use of empirical programming of the device and/or stopping the stimulation temporarily. The subscores, as well as the total scores of the BFMDRS/UDRS, were available for nine patients (Supplementary Table S2). In all the patients, the worsening of the subscores at long-term follow-ups was observed not only in the cranial regions but also in the limbs and trunk. The lead positions were measured at each expert center and judged optimal (Supplementary Table S3). Various stimulation settings were attempted through multiple programming sessions: i.e., single monopolar, double monopolar, or bipolar stimulation with combinations of different voltage, pulse width, and stimulation frequency. All patients underwent additional DBS surgeries targeting the GPi or subthalamic nucleus (STN). The timing of additional implantations for individual patients is shown in Figure 1A. Five patients underwent implantation of a second pair of leads within the bilateral GPi; three underwent additional bilateral STN implantation; one underwent replacement of bilateral GPi leads because of lead fractures; considering the asymmetric nature of dystonia symptoms, one underwent additional implantation within the unilateral STN, and one underwent additional implantation within the unilateral STN and GPi. There were no patients with meaningful benefits following additional implantations.
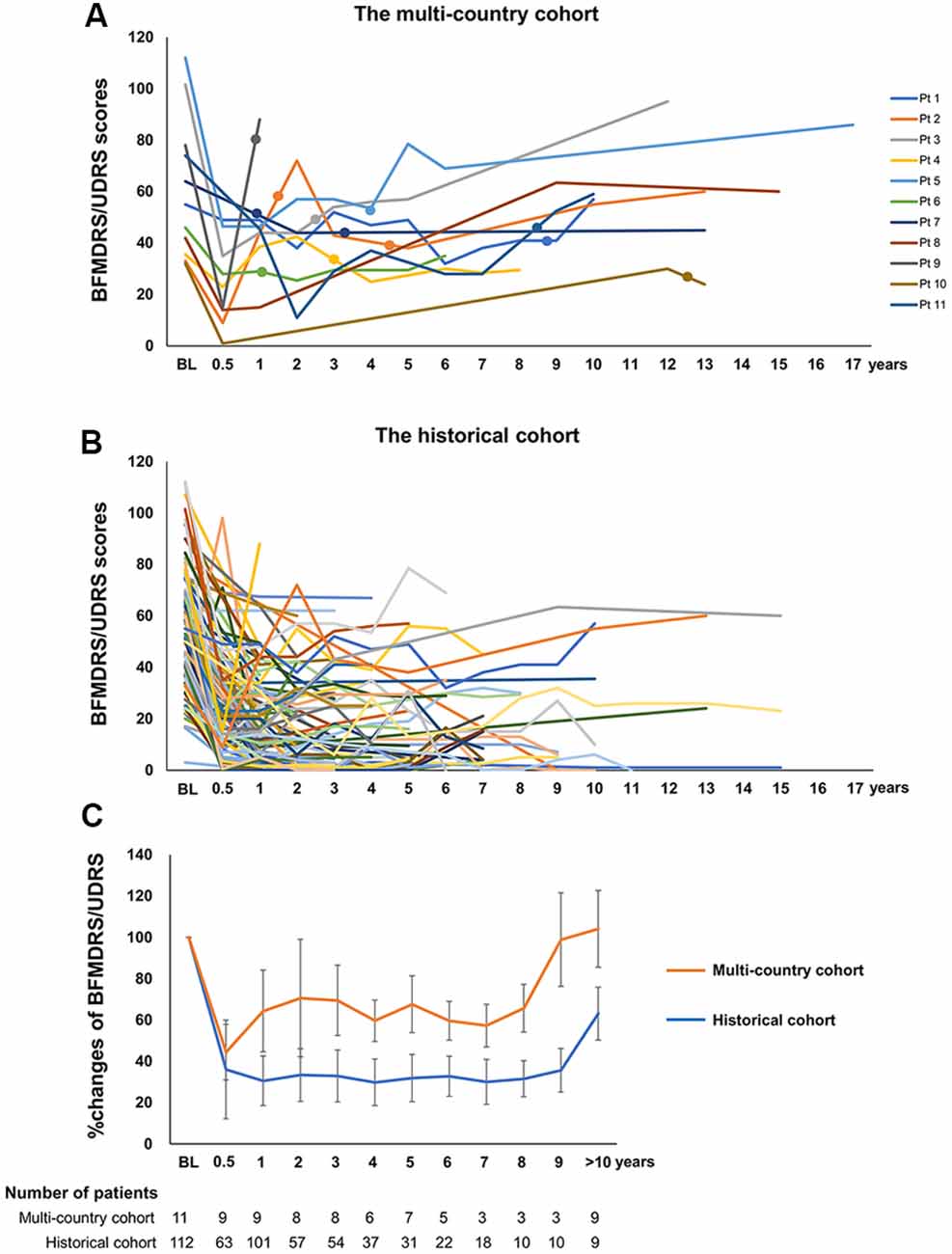
Figure 1. (A) Individual changes of the Burke-Fahn-Marsden Dystonia Rating Scale motor (BFMDRS-M) or Unified Dystonia Rating Scale (UDRS) in the multi-country cohort. (B) The closed circles on each line indicate additional GPi or subthalamic nucleus (STN) implantations. Individual changes of the BFMDRS-M or UDRS in the historical cohort. (C) Comparison of percent improvement of the BFMDRS-M/UDRS in the multi-country cohort and the historical cohort. Orange and blue line graphs represent mean scores for the multi-country cohort and the historical cohort, respectively. Whiskers represent standard errors. BFMDRS-M, Burke-Fahn-Marsden Dystonia Rating Scale motor score; UDRS, Unified Dystonia Rating Scale.
Despite these interventions, the improvement at the last follow-up was less than 30% as compared with the baseline status. When the stimulation was turned off, all the patients in the cohort experienced immediate worsening at the long-term follow-up.
The Historical Cohort
The flowchart of the systematic search and review process is shown in Figure 2. The list of 37 studies used for the historical cohort is presented in Supplementary Table S4. Twenty-four duplicated patients and five patients with reported suboptimal lead positions were removed. Consequently, a total of 112 DYT1 patients who met the inclusion criteria were included in the historical cohort. All the patients were treated with bilateral GPi DBS. Individual changes of BFMDRS-M/UDRS revealed sustained improvement of dystonia in most patients (Figure 1B). As shown in Figure 1C, the multi-country and historical cohorts showed distinct trajectories in the long-term. At the group level, the historical cohort experienced mean improvement of 64% at 6 months after surgery and had sustained improvement of approximately 65–70% up to 9 years; mean improvement at ≥10 years was less impressive (37%) because of worsening of scores in a subset of patients with a variable length of follow-up periods. Note that the historical cohort included a total of eight patients with suboptimal DBS responses from our earlier studies who were also included in the multi-country cohort (Cif et al., 2010; Krause et al., 2016; Tsuboi et al., 2019).
Comparison Between the Multi-country Cohort and the Historical Cohort
We compared the historical cohort with the multi-country cohort who manifested suboptimal DBS responses for unexplained reasons (Table 2). Mean motor improvement at the last follow-up from baseline were 0% and 66% for the multi-country and historical cohorts, respectively. Compared with the historical cohort, the multi-country cohort had a significantly younger age at onset (p = 0.025), younger age at DBS (p = 0.019), and a significantly longer follow-up period after DBS (p < 0.001). Additionally, cranial involvement was more common in the multi-country cohort compared with the historical cohort (before DBS, 64% vs. 45%, p = 0.074; before or after DBS, 91% vs. 47%, p = 0.001).
Suboptimal and Good Responders in the Historical Cohort
In the historical cohort, 16 of 112 patients (14%) experienced suboptimal responses to DBS for unclear reasons at the last follow-up (<30% improvement). The clinical characteristics of the suboptimal and good responders are presented separately in Table 3. Individual data are shown in Supplementary Tables S5, S6, respectively. Mean motor improvement at the last follow-up from baseline was 4% and 77% for the suboptimal and good responders, respectively. Compared with the good responders, the suboptimal responders had a significantly younger age at onset (p = 0.005) and a significantly longer follow-up period after DBS (p = 0.020). Cranial involvement was more common in the suboptimal responders compared with the good responders (before DBS, 75% vs. 39%, p = 0.077; before or after DBS, 83% vs. 43%, p = 0.007).
The suboptimal responders can be divided into two groups (Table 4 and Supplementary Table S5): those with short disease duration before DBS (2–9 years) and those with very long disease duration before DBS (17.5–36 years). Both groups had similar age at onset (7.1 vs. 7.7 years old, p = 0.681) and similarly high rates of cranial involvement (both 75%, p = 0.745). However, the possible contribution of fixed skeletal deformities to suboptimal DBS response was described only in those with very long disease duration before DBS (n = 3).
Discussion
The present study aimed to investigate the clinical characteristics of a multi-country cohort of patients with DYT1 dystonia who experienced suboptimal responses to DBS. Because only a few cases of DYT1 dystonia are operated each year even in expert centers, this dataset, though difficult to obtain, is crucial for the field. A few retrospective studies and a recent meta-analysis of mixed cohorts of inherited or idiopathic isolated dystonia patients reported that the TOR1A mutation-positive status was associated with a better DBS response, whereas the reasons underpinning suboptimal responses remained mostly unexplored (Vasques et al., 2009; Andrews et al., 2010; Borggraefe et al., 2010; FitzGerald et al., 2014; Moro et al., 2017). Our findings suggest that a subset of DYT1 patients may have a relatively more aggressive phenotype of DYT1 dystonia characterized by younger age at onset, faster disease progression before DBS, and cranial involvement. Patients with this phenotype may be more likely to experience suboptimal responses to DBS.
There is a surprising lack of published information on suboptimal responses to DBS in DYT1 dystonia despite a growing number of reported cases. Panov et al. (2013) reported that 47 DYT1 patients experienced an improvement in BFMDRS-M by greater than 80% up to 7 years after surgery; however, there was no description of patients with suboptimal DBS responses. Markun et al. (2012) observed improvement in BFMDRS-M by 70.3% in 14 DYT1 patients with a mean follow-up of 2.7 years and only commented on patients with skeletal deformities associated with suboptimal improvement. Cif et al. (2010) observed 59% improvement in 26 DYT1 patients with a mean follow-up of 6.2 years. Their cases showed less improvement compared with the former two cohorts; however, they included five cases (19%) who had suboptimal long-term DBS responses. Details of these (Cif et al., 2010) suboptimal patients are included as part of the multi-country cohort. Finally and importantly, most cases in the historical cohort also revealed sustained improvement in the long-term as contrasted to our multi-country cohort. The rate of suboptimal DBS responses due to unexplained reasons was approximately 8% (11 out of 132) in the multi-country cohort, which seems comparable to 14% in the historical cohort. Note that the data from the historical cohort should be interpreted with caution because of limited clinical information available on DBS programming and lead locations.
All 11 patients in our multi-country cohort initially experienced dystonia improvement of more than 30% postoperatively with subsequent worsening. Intriguingly, secondary worsening of dystonia symptoms began between 6 months and 3 years after DBS without any identifiable reasons, e.g., secondary skeletal deformities, cervical myelopathy, lead migrations, device malfunction, and suboptimal programming (Krauss et al., 2002; Anheim et al., 2008; Isaias et al., 2008; Picillo et al., 2016; Morishita et al., 2017; Pauls et al., 2017; Tsuboi et al., 2019). All the patients underwent additional DBS surgeries targeting the STN or GPi; however, these extra leads did not seem to provide a robust benefit. This finding is in agreement with the earlier study reporting variable responses to additional DBS implantations within DYT1 patients (Cif et al., 2012). Importantly, we observed a clear worsening of dystonia symptoms when DBS was turned off at the long-term follow-up visits, reinforcing the idea that DBS may still be a useful intervention even if the clinical outcomes are less robust. However, we cannot determine the relative contribution of disease progression and loss of benefits to secondary worsening because of the lack of formal on- and off-stimulation motor score comparisons (this was a limitation of the multi-country cohort and several cases treated worldwide). The involvement of body parts unaffected before surgery suggests disease progression likely played a role.
To the best of our knowledge, available studies have not analyzed the relationship between cranial involvement and DBS responses in DYT1 patients. Cranial involvement is less common in patients with DYT1 dystonia, with a range reported between 12.0% and 28.2% (Lee et al., 2012). However, cranial involvement in the multi-country cohort (before DBS, 64%; before or after DBS, 91%) was more frequently observed compared with the historical cohort and compared with the literature (Lee et al., 2012). Similarly, in the historical cohort, the suboptimal responders also showed a higher rate of cranial involvement as compared with the good responders. These findings strongly suggest the possible association between cranial involvement and suboptimal DBS responses in DYT1 patients.
The multi-country cohort had a young age at onset of dystonia ranging from 5 to 10 years old. The mean age at onset of the multi-country cohort was significantly younger when compared with the historical cohort (7.0 vs. 8.6 years old). The multi-country cohort required DBS treatment significantly younger than the historical cohort (mean, 12.0 vs. 19.0 years old) with relatively shorter disease duration (mean, 6.1 vs. 10.5 years, p > 0.05). Notably, dystonia evolved quickly to become generalized within 2.5 years after onset in all the patients of the multi-country cohort, and the motor symptoms progressed. Although the precise information on the time to a generalization of dystonia was not available for the historical cohort, the mean time to generalization of dystonia in DYT1 patients was previously reported at 3.1–8.4 (range, 1–30) years (Fasano et al., 2006; Lee et al., 2012), which was longer than that in the multi-country cohort. These results corroborate the multi-country cohort had faster disease progression with a younger age at onset.
Despite early intervention with DBS, the multi-country cohort experienced suboptimal outcomes in the long-term. This result appears to be contradictory to the earlier dystonia studies reporting the association between better outcomes and shorter disease duration before DBS (Isaias et al., 2011; Lumsden et al., 2013; Artusi et al., 2020). In the suboptimal responders included in the historical cohort, only those with very long disease duration before DBS were reported to have fixed skeletal deformities, and age at onset was similar between these two groups. Therefore, fixed skeletal deformities are thought to be responsible for their suboptimal DBS responses at least to some extent (Isaias et al., 2008; Pauls et al., 2017). Nevertheless, the multi-country cohort experienced secondary worsening following initial good responses to DBS without any identifiable reasons, and these patients were characterized by younger age at onset, faster disease progression before DBS, and cranial involvement. Because this phenotype of DYT1 dystonia is relatively less common (approximately 8%), analyses at the group level may not identify the presence of this phenotype. Thus, the present case series revealed a possible aggressive phenotype of DYT1 dystonia and provided detailed observations.
Although the genetics of DYT1 dystonia appear simple because virtually all cases have the same pathogenic deletion variant (c.907_909delGAG), the reduced penetrance and variable clinical phenomenology suggest that other genetic or environmental modifying factors influence phenotypic expression and that these factors may factor into responses to DBS or other treatments (Charlesworth et al., 2013; Weisheit et al., 2018). Dystonia patients carrying different variants in the TOR1A gene have been reported, but the consensus has yet to be reached regarding their pathogenicity (Martino et al., 2013; Siokas et al., 2019). A polymorphism in exon 4 of the DYT1 gene (rs1801968, D216H) was reported to affect the clinical penetrance of DYT1 (Risch et al., 2007). Additionally, the multi-country cohort was not tested for other dystonia-causing genes such as DYT-THAP1 and DYT-GNAL, which are known to have a higher incidence of cranial involvement. Furthermore, some variants in brain-derived neurotrophic factor (BDNF) and apolipoprotein E (APOE) have also been reported to lead to an increased incidence of dystonia, possibly via altered neural plasticity (Siokas et al., 2019). These findings lead us to speculate on the intriguing possibility that variants within the TOR1A gene or other genes may affect the phenotypic manifestation of dystonia and the consequent DBS responses. The data from the current study cannot support or refute this hypothesis. Another unanswered question is the reason for secondary worsening after good initial responses to DBS. Curiously, secondary worsening of dystonia has also been reported in other genetic forms of dystonia, such as DYT-THAP1 or GNAO1 mutations (Panov et al., 2012; Brüggemann et al., 2015; Koy et al., 2018). Future neurophysiological and functional imaging studies will hopefully shed further light on the underlying pathophysiology.
There are several weaknesses in this work that should be considered. Because only a few cases of suboptimal responses to DBS are recorded even in expert centers, accumulating enough cases is challenging. Assembling this cohort required a multi-country effort. We would argue that collecting 11 patients with suboptimal outcomes provided valuable information. We could not analyze the whole cohort of the participating centers, which included patients with successful DBS outcomes. Because most studies included were observational unblinded studies, we did not perform a formal risk of bias assessments for the historical cohort. Although the findings from the historical cohort were similar to those from earlier single-center cohorts, the possibility of publication bias must be considered (Markun et al., 2012; Panov et al., 2013). Also, we had to exclude some articles because of insufficient individual clinical information. The historical cohort lacked the data of disease duration before dystonia generalization. The data from the historical cohort should be interpreted with caution because detailed information on DBS programming and lead locations were not available in most publications. The rate of suboptimal DBS responses in the historical cohort might increase with longer follow-up periods. The leads in the multi-country cohort were subjectively judged to be well-placed locally in each DBS center, based on expert opinions. We could not analyze the relationship between lead positions and DBS responses with a unified methodology because the digital imaging data were no longer available for some patients. Ideally, the accuracy of targeting within the posteroventrolateral GPi should be examined using advanced imaging analyses. Importantly, lead locations may potentially impact short-term effects as well as long-term therapeutic outcomes. The multi-country cohort attempted various stimulation settings through multiple programming sessions without a standardized programming protocol. Because the therapeutic effects of DBS in DYT1 patients are highly variable, we cannot exclude the possibility that there is room for improvement with higher stimulation intensity or different combinations of stimulation settings (Kupsch et al., 2011; Cif et al., 2013; Picillo et al., 2016). Finally, the threshold for a suboptimal response was chosen arbitrarily to exceed a placebo effect.
Conclusion
Approximately 8% of patients represent a more aggressive phenotype of DYT1 dystonia characterized by younger age at onset, faster disease progression, and cranial involvement, which seems to be associated with suboptimal DBS responses in the long-term (e.g., secondary worsening). Importantly, the on-/off-stimulation comparison at the long-term follow-up demonstrated beneficial effects of DBS despite missing the 30% threshold for improvement over baseline. Therefore, DBS may still be a useful intervention, even if the clinical outcomes are less robust. Additional rescue STN or GPi DBS implantations may not provide meaningful improvement if the original leads were placed optimally. Patients with this phenotype may have different neuroplasticity, neurogenetics, or possibly distinct neurophysiology, although the exact differences underpinning DBS outcomes are unknown. Future studies should explore whether genetic variants within the TOR1A gene or other genes may determine DBS responses. This information could be useful for both clinicians and patients in clinical decision making and patient counseling before and following DBS implantations.
Data Availability Statement
The datasets generated for this study are available on request to the corresponding author.
Ethics Statement
The studies involving human participants were reviewed and approved by University of Florida Institutional Review Board. Written informed consent from the participants’ legal guardian/next of kin was not required to participate in this study in accordance with the national legislation and the institutional requirements.
Author Contributions
TT: research project conception, organization, and execution; statistical analysis design and execution; manuscript writing of the first draft. LC, PC, JO, DR, YM, AL, PD, IH, FM, NS, LO, AW, and KF: research project execution; manuscript review and critique. JC: statistical analysis review and critique; manuscript review and critique. MO: research project conception, organization, and execution; manuscript review and critique.
Funding
The work was supported by a research fellowship program of the Uehara Memorial Foundation (TT) and a grant NS087997 (LO, NS). JO reports grants from NIH, Michael J. Fox Foundation, Cala Health, DOD Defense Advanced Research Projects Agency. JO also reports training grant support/consulting from Medtronic corporation and research support, training grant support from Boston scientific. YM previously received lecture fees and relevant travel expenses from Kyowa Kirin Co., Otsuka Pharmaceutical Co., Sumitomo Dainippon Pharma Co., AbbVie GK, Novartis Pharmaceuticals Co., FP Pharmaceuticals Co., St. Jude Medical., Boston Scientific Japan, Medtronic Japan, and InSightec Japan K.K. YM also had fixed-term consulting business with Boston Scientific Japan, Medtronic Japan. AL serves as Consultant of Boston Scientific, Medtronic, Insightec, Functional Neuromodulation, and Abbott. PD is supported by the 2016 Research Prize of the European Society for Stereotactic and Functional Neurosurgery and the Healers Foundation. He received grants for education and traveling from Medtronic, Boston Scientific, and St. Jude-Abbott. IH receives partial salary support from the NINDS and NIAA. He has performed research for Allergan, Boston Scientific, Great Lakes Neurotechnology, the MJFF, NeuroDerm, PSG, and Pfizer, in the course of which he has also received partial salary support. He has performed paid consultations for Boston Scientific and Medtronics and unpaid consultations for Epic Systems. His potential conflicts of interest are managed by Wake Forest School of Medicine. FM reports a grant from Beijing Municipal Administration of Hospitals Clinical Medicine Development of special funding support (XMLX201833) and National Natural Science Foundation of China (81971070). NS is supported by grants from the NIH and serves as editor in chief of Brain and Behavior (Wiley publishing). LO is supported by grants from NIH and the Collaborative Center for X-linked Dystonia Parkinsonism. She receives royalties from Athena Diagnostics for patents related to dystonia gene testing. AW reports grants from the NIH and has received grant support from Benign Essential Blepharospasm Research Foundation, Dystonia Coalition, Dystonia Medical Research Foundation, National Organization for Rare Disorders, and grant support from NIH (KL2 and K23 NS092957-01A1). KF reports grants from NIH, and other funding from Donnellan/Einstein/Merz Chair, during this study; grants and non-financial support from Medtronic, grants from St Jude, Functional Neuromodulation, and Boston Scientific, and grants and other funding from Neuropace. Additionally, KF has a patent US 8295935 B2 issued for a DBS cranial lead fixation device. MO serves as a consultant for the National Parkinson Foundation and has received research grants from NIH, NPF, the Michael J. Fox Foundation, the Parkinson Alliance, Smallwood Foundation, the Bachmann-Strauss Foundation, the Tourette Syndrome Association, and the UF Foundation. MO’s DBS research is supported by R01 NR014852 and R01NS096008. MO has previously received honoraria, but in the past >60 months has received no support from industry. MO has received royalties for publications with Demos, Manson, Amazon, Smashwords, Books4Patients, and Cambridge (movement disorders books). MO is an associate editor for the New England Journal of Medicine Journal Watch Neurology. MO has participated in CME and educational activities on movement disorders (in the last 36 months) sponsored by PeerView, Prime, QuantiaMD, WebMD, Medicus, MedNet, Henry Stewart, and by Vanderbilt University. The institution and not MO receives grants from Medtronic, Abbvie, Allergan, and ANS/St. Jude and the principal investigator (PI) has no financial interest in these grants. MO has participated as a site PI and/or co-investigator for several NIH, foundation, and industry-sponsored trials over the years but has not received honoraria.
Conflict of Interest
The authors declare that the research was conducted in the absence of any commercial or financial relationships that could be construed as a potential conflict of interest.
Acknowledgments
We would like to acknowledge Tyler’s Hope for a Dystonia Cure. TT sincerely appreciates the Uehara Memorial Foundation for the research fellowship program.
Footnotes
Supplementary Material
The Supplementary Material for this article can be found online at: https://www.frontiersin.org/articles/10.3389/fnhum.2020.00242/full#supplementary-material.
References
Andrews, C., Aviles-Olmos, I., Hariz, M., and Foltynie, T. (2010). Which patients with dystonia benefit from deep brain stimulation? A metaregression of individual patient outcomes. J. Neurol. Neurosurg. Psychiatry 81, 1383–1389. doi: 10.1136/jnnp.2010.207993
Anheim, M., Vercueil, L., Fraix, V., Chabardès, S., Seigneuret, E., Krack, P., et al. (2008). Early stimulation of DYT1 primary generalized dystonia prevents from its secondary irreversible complications. Mov. Disord. 23, 2261–2263. doi: 10.1002/mds.22152
Artusi, C. A., Dwivedi, A., Romagnolo, A., Bortolani, S., Marsili, L., Imbalzano, G., et al. (2020). Differential response to pallidal deep brain stimulation among monogenic dystonias: systematic review and meta-analysis. J. Neurol. Neurosurg. Psychiatry 91, 426–433. doi: 10.1136/jnnp-2019-322169
Ben-Haim, S., Flatow, V., Cheung, T., Cho, C., Tagliati, M., and Alterman, R. L. (2016). Deep brain stimulation for status dystonicus: a case series and review of the literature. Stereotact. Funct. Neurosurg. 94, 207–215. doi: 10.1159/000446191
Borggraefe, I., Mehrkens, J. H., Telegravciska, M., Berweck, S., Bötzel, K., and Heinen, F. (2010). Bilateral pallidal stimulation in children and adolescents with primary generalized dystonia—report of six patients and literature-based analysis of predictive outcomes variables. Brain Dev. 32, 223–228. doi: 10.1016/j.braindev.2009.03.010
Brüggemann, N., Kühn, A., Schneider, S. A., Kamm, C., Wolters, A., Krause, P., et al. (2015). Short- and long-term outcome of chronic pallidal neurostimulation in monogenic isolated dystonia. Neurology 84, 895–903. doi: 10.1212/WNL.0000000000001312
Burke, R. E., Fahn, S., Marsden, C. D., Bressman, S. B., Moskowitz, C., and Friedman, J. (1985). Validity and reliability of a rating scale for the primary torsion dystonias. Neurology 35, 73–77. doi: 10.1212/wnl.35.1.73
Charlesworth, G., Bhatia, K. P., and Wood, N. W. (2013). The genetics of dystonia: new twists in an old tale. Brain 136, 2017–2037. doi: 10.1093/brain/awt138
Cif, L., Gonzalez-Martinez, V., Vasques, X., Corlobé, A., Moura, A. M., Bonafé, A., et al. (2012). Staged implantation of multiple electrodes in the internal globus pallidus in the treatment of primary generalized dystonia. J. Neurosurg. 116, 1144–1152. doi: 10.3171/2012.1.JNS102045
Cif, L., Ruge, D., Gonzalez, V., Limousin, P., Vasques, X., Hariz, M. I., et al. (2013). The influence of deep brain stimulation intensity and duration on symptoms evolution in an OFF stimulation dystonia study. Brain Stimul. 6, 500–505. doi: 10.1016/j.brs.2012.09.005
Cif, L., Vasques, X., Gonzalez, V., Ravel, P., Biolsi, B., Collod-Beroud, G., et al. (2010). Long-term follow-up of DYT1 dystonia patients treated by deep brain stimulation: an open-label study. Mov. Disord. 25, 289–299. doi: 10.1002/mds.22802
Comella, C. L., Leurgans, S., Wuu, J., Stebbins, G. T., Chmura, T., and Dystonia Study Group. (2003). Rating scales for dystonia: a multicenter assessment. Mov. Disord. 18, 303–312. doi: 10.1002/mds.10377
Coubes, P., Roubertie, A., Vayssiere, N., Hemm, S., and Echenne, B. (2000). Treatment of DYT1-generalised dystonia by stimulation of the internal globus pallidus. Lancet 355, 2220–2221. doi: 10.1016/s0140-6736(00)02410-7
Fasano, A., Nardocci, N., Elia, A. E., Zorzi, G., Bentivoglio, A. R., and Albanese, A. (2006). Non-DYT1 early-onset primary torsion dystonia: comparison with DYT1 phenotype and review of the literature. Mov. Disord. 21, 1411–1418. doi: 10.1002/mds.21000
FitzGerald, J. J., Rosendal, F., de Pennington, N., Joint, C., Forrow, B., Fletcher, C., et al. (2014). Long-term outcome of deep brain stimulation in generalised dystonia: a series of 60 cases. J. Neurol. Neurosurg. Psychiatry 85, 1371–1376. doi: 10.1136/jnnp-2013-306833
Isaias, I. U., Alterman, R. L., and Tagliati, M. (2008). Outcome predictors of pallidal stimulation in patients with primary dystonia: the role of disease duration. Brain 131, 1895–1902. doi: 10.1093/brain/awn120
Isaias, I. U., Volkmann, J., Kupsch, A., Burgunder, J.-M., Ostrem, J. L., Alterman, R. L., et al. (2011). Factors predicting protracted improvement after pallidal DBS for primary dystonia: the role of age and disease duration. J. Neurol. 258, 1469–1476. doi: 10.1007/s00415-011-5961-9
Koy, A., Cirak, S., Gonzalez, V., Becker, K., Roujeau, T., Milesi, C., et al. (2018). Deep brain stimulation is effective in pediatric patients with GNAO1 associated severe hyperkinesia. J. Neurol. Sci. 391, 31–39. doi: 10.1016/j.jns.2018.05.018
Krause, M., Fogel, W., Kloss, M., Rasche, D., Volkmann, J., and Tronnier, V. (2004). Pallidal stimulation for dystonia. Neurosurgery 55, 1361–1368; discussion 1368–1370. doi: 10.1227/01.neu.0000143331.86101.5e
Krause, P., Lauritsch, K., Lipp, A., Horn, A., Weschke, B., Kupsch, A., et al. (2016). Long-term results of deep brain stimulation in a cohort of eight children with isolated dystonia. J. Neurol. 263, 2319–2326. doi: 10.1007/s00415-016-8253-6
Krauss, J. K., Loher, T. J., Pohle, T., Weber, S., Taub, E., Bärlocher, C. B., et al. (2002). Pallidal deep brain stimulation in patients with cervical dystonia and severe cervical dyskinesias with cervical myelopathy. J. Neurol. Neurosurg. Psychiatry 72, 249–256. doi: 10.1136/jnnp.72.2.249
Kupsch, A., Tagliati, M., Vidailhet, M., Aziz, T., Krack, P., Moro, E., et al. (2011). Early postoperative management of DBS in dystonia: programming, response to stimulation, adverse events, medication changes, evaluations and troubleshooting. Mov. Disord. 26, S37–S53. doi: 10.1002/mds.23624
Lee, W.-W., Ahn, T.-B., Chung, S. J., and Jeon, B. S. (2012). Phenotypic differences in Dyt1 between ethnic groups. Curr. Neurol. Neurosci. Rep. 12, 341–347. doi: 10.1007/s11910-012-0285-4
Lumsden, D. E., Kaminska, M., Gimeno, H., Tustin, K., Baker, L., Perides, S., et al. (2013). Proportion of life lived with dystonia inversely correlates with response to pallidal deep brain stimulation in both primary and secondary childhood dystonia. Dev. Med. Child Neurol. 55, 567–574. doi: 10.1111/dmcn.12117
Markun, L. C., Starr, P. A., Air, E. L., Marks, W. J., Volz, M. M., and Ostrem, J. L. (2012). Shorter disease duration correlates with improved long-term deep brain stimulation outcomes in young-onset DYT1 dystonia. Neurosurgery 71, 325–330. doi: 10.1227/NEU.0b013e318258e21b
Martino, D., Gajos, A., Gallo, V., Cif, L., Coubes, P., Tinazzi, M., et al. (2013). Extragenetic factors and clinical penetrance of DYT1 dystonia: an exploratory study. J. Neurol. 260, 1081–1086. doi: 10.1007/s00415-012-6765-2
Mehrkens, J. H., Bötzel, K., Steude, U., Zeitler, K., Schnitzler, A., Sturm, V., et al. (2009). Long-term efficacy and safety of chronic globus pallidus internus stimulation in different types of primary dystonia. Stereotact. Funct. Neurosurg. 87, 8–17. doi: 10.1159/000177623
Miyagi, Y., and Koike, Y. (2013). Tolerance of early pallidal stimulation in pediatric generalized dystonia. J. Neurosurg. Pediatr. 12, 476–482. doi: 10.3171/2013.8.PEDS12578
Morishita, T., Hilliard, J. D., Okun, M. S., Neal, D., Nestor, K. A., Peace, D., et al. (2017). Postoperative lead migration in deep brain stimulation surgery: incidence, risk factors and clinical impact. PLoS One 12:e0183711. doi: 10.1371/journal.pone.0183711
Moro, E., LeReun, C., Krauss, J. K., Albanese, A., Lin, J.-P., Walleser Autiero, S., et al. (2017). Efficacy of pallidal stimulation in isolated dystonia: a systematic review and meta-analysis. Eur. J. Neurol. 24, 552–560. doi: 10.1111/ene.13255
Panov, F., Gologorsky, Y., Connors, G., Tagliati, M., Miravite, J., and Alterman, R. L. (2013). Deep brain stimulation in DYT1 dystonia: a 10-year experience. Neurosurgery 73, 86–93. doi: 10.1227/01.neu.0000429841.84083.c8
Panov, F., Tagliati, M., Ozelius, L. J., Fuchs, T., Gologorsky, Y., Cheung, T., et al. (2012). Pallidal deep brain stimulation for DYT6 dystonia. J. Neurol. Neurosurg. Psychiatry 83, 182–187. doi: 10.1136/jnnp-2011-300979
Pauls, K. A. M., Krauss, J. K., Kämpfer, C. E., Kühn, A. A., Schrader, C., Südmeyer, M., et al. (2017). Causes of failure of pallidal deep brain stimulation in cases with pre-operative diagnosis of isolated dystonia. Parkinsonism Relat. Disord. 43, 38–48. doi: 10.1016/j.parkreldis.2017.06.023
Picillo, M., Lozano, A. M., Kou, N., Munhoz, R. P., and Fasano, A. (2016). Programming deep brain stimulation for tremor and dystonia: the toronto western hospital algorithms. Brain Stimul. 9, 438–452. doi: 10.1016/j.brs.2016.02.003
Risch, N. J., Bressman, S. B., Senthil, G., and Ozelius, L. J. (2007). Intragenic Cis and trans modification of genetic susceptibility in DYT1 torsion dystonia. Am. J. Hum. Genet. 80, 1188–1193. doi: 10.1086/518427
Siokas, V., Aloizou, A., Tsouris, Z., Michalopoulou, A., Mentis, A. A., and Dardiotis, E. (2019). Risk factor genes in patients with dystonia: a comprehensive review. Tremor Other Hyperkinet. Mov. 8:559. doi: 10.7916/D8H438GS
Starr, P. A., Turner, R. S., Rau, G., Lindsey, N., Heath, S., Volz, M., et al. (2006). Microelectrode-guided implantation of deep brain stimulators into the globus pallidus internus for dystonia: techniques, electrode locations and outcomes. J. Neurosurg. 104, 488–501. doi: 10.3171/jns.2006.104.4.488
Susatia, F., Malaty, I. A., Foote, K. D., Wu, S. S., Zeilman, P. R., Mishra, M., et al. (2010). An evaluation of rating scales utilized for deep brain stimulation for dystonia. J. Neurol. 257, 44–58. doi: 10.1007/s00415-009-5261-9
Tsuboi, T., Jabarkheel, Z., Foote, K. D., Okun, M. S., and Wagle Shukla, A. (2019). Importance of the initial response to GPi deep brain stimulation in dystonia: a nine year quality of life study. Parkinsonism Relat. Disord. 64, 249–255. doi: 10.1016/j.parkreldis.2019.04.024
Vasques, X., Cif, L., Gonzalez, V., Nicholson, C., and Coubes, P. (2009). Factors predicting improvement in primary generalized dystonia treated by pallidal deep brain stimulation. Mov. Disord. 24, 846–853. doi: 10.1002/mds.22433
Vidailhet, M., Vercueil, L., Houeto, J. L., Krystkowiak, P., Benabid, A. L., Cornu, P., et al. (2005). Bilateral deep-brain stimulation of the globus pallidus in primary generalized dystonia. N. Engl. J. Med. 352, 459–467. doi: 10.1056/NEJMoa042187
Volkmann, J., Wolters, A., Kupsch, A., Müller, J., Kühn, A. A., Schneider, G.-H., et al. (2012). Pallidal deep brain stimulation in patients with primary generalised or segmental dystonia: 5-year follow-up of a randomised trial. Lancet Neurol. 11, 1029–1038. doi: 10.1016/S1474-4422(12)70257-0
Keywords: DYT1, dystonia, deep brain stimulation, globus pallidus internus, pallidum
Citation: Tsuboi T, Cif L, Coubes P, Ostrem JL, Romero DA, Miyagi Y, Lozano AM, De Vloo P, Haq I, Meng F, Sharma N, Ozelius LJ, Wagle Shukla A, Cauraugh JH, Foote KD and Okun MS (2020) Secondary Worsening Following DYT1 Dystonia Deep Brain Stimulation: A Multi-country Cohort. Front. Hum. Neurosci. 14:242. doi: 10.3389/fnhum.2020.00242
Received: 21 February 2020; Accepted: 02 June 2020;
Published: 25 June 2020.
Edited by:
Yusuf Ozgur Cakmak, University of Otago, New ZealandReviewed by:
Stephen Tisch, St Vincent’s Hospital Sydney, AustraliaElena Moro, Université Grenoble Alpes, France
Marie Vidailhet, Université Pierre et Marie Curie, France
Copyright © 2020 Tsuboi, Cif, Coubes, Ostrem, Romero, Miyagi, Lozano, De Vloo, Haq, Meng, Sharma, Ozelius, Wagle Shukla, Cauraugh, Foote and Okun. This is an open-access article distributed under the terms of the Creative Commons Attribution License (CC BY). The use, distribution or reproduction in other forums is permitted, provided the original author(s) and the copyright owner(s) are credited and that the original publication in this journal is cited, in accordance with accepted academic practice. No use, distribution or reproduction is permitted which does not comply with these terms.
*Correspondence: Takashi Tsuboi, takashi80@gmail.com; takashi.tsuboi@neurology.ufl.edu
†ORCID: Takashi Tsuboi orcid.org/0000-0001-5042-3181