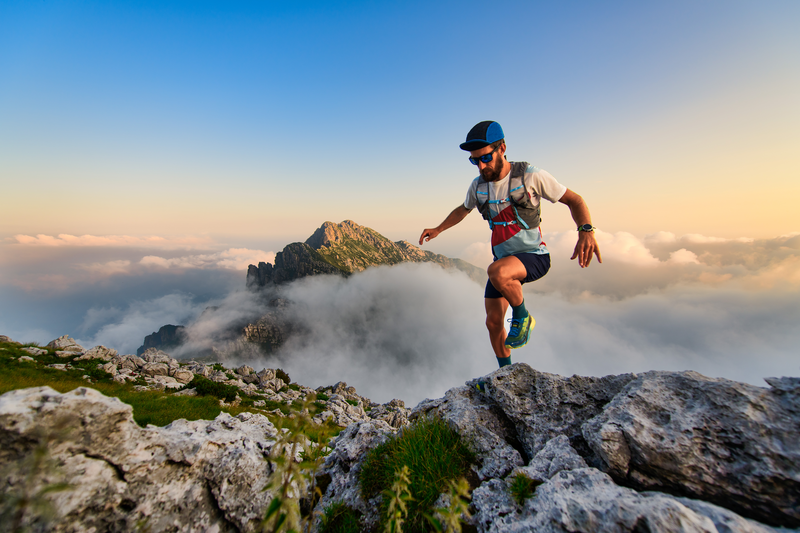
95% of researchers rate our articles as excellent or good
Learn more about the work of our research integrity team to safeguard the quality of each article we publish.
Find out more
BRIEF RESEARCH REPORT article
Front. Hum. Neurosci. , 21 April 2020
Sec. Cognitive Neuroscience
Volume 14 - 2020 | https://doi.org/10.3389/fnhum.2020.00131
This article is part of the Research Topic Wayfinding and Navigation: Strengths and Weaknesses in Atypical and Clinical Populations View all 16 articles
Path integration spatial navigation processes are emerging as promising cognitive markers for prodromal and clinical Alzheimer’s disease (AD). However, such path integration changes have been less explored in Vascular Cognitive Impairment (VCI), despite neurovascular change being a major contributing factor to dementia and potentially AD. In particular, the sensitivity and specificity of path integration impairments in VCI compared to AD is unclear. In the current pilot study, we explore path integration performance in early-stage AD and VCI patient groups and hypothesize that: (i) medial parietal mediated egocentric processes will be more affected in VCI; and (ii) medial temporal mediated allocentric processes will be more affected in AD. This cross-sectional study included early-stage VCI patients (n = 9), AD patients (n = 10) and healthy age-matched controls (n = 20). All participants underwent extensive neuropsychological testing, as well as spatial navigation testing. The spatial navigation tests included the virtual reality “Supermarket” task assessing egocentric (body-based) and allocentric (map-based) navigation as well as the “Clock Orientation” test assessing egocentric and path integration processes. Results showed that egocentric integration processes are only impaired in VCI, potentially distinguishing it from AD. However, in contrast to our prediction, allocentric integration was not more impaired in AD compared to VCI. These preliminary findings suggest limited specificity of allocentric integration deficits between VCI and AD. By contrast, egocentric path integration deficits emerge as more specific to VCI, potentially allowing for more specific diagnostic and treatment outcome measures for vascular impairment in dementia.
Vascular cognitive impairment (VCI) is the second most prevalent cause of cognitive decline after Alzheimer’s disease (AD) and is thought to account for ~20% of all dementias (Goodman et al., 2017; van der Flier et al., 2018). Although, individuals with mixed (AD and VCI) pathology are estimated to account for up to 70% of all dementia cases (Toledo et al., 2013). Despite the high prevalence of vascular impairment, its cognitive correlates are still being explored. Clinically, VCI is considered to involve a decline in executive function and higher-order cognition such as information processing, planning, set-shifting and working memory (Hachinski et al., 2006; Sachdev et al., 2014). These changes are mostly attributed to micro and macro infarcts in subcortical and cortical regions, as well as their connecting white matter tracts (Beason-Held et al., 2012; van der Flier et al., 2018), in particular affecting frontoparietal networks. Nevertheless, attributing such executive changes to VCI specifically has remained challenging, as deficits in executive function can also present as part of AD or related pathophysiology (Neufang et al., 2011; Girard et al., 2013; Guarino et al., 2018). However, the recent development of novel spatial navigation cognitive markers for AD show promise in being more specific to underlying disease pathophysiology (Coughlan et al., 2018b) and may help to identify cognitive decline specific to VCI. A clear distinction between VCI and AD is essential to both clinicians and patients as with appropriate intervention VCI can be slowed or halted, whereas AD has a fixed and terminal prognosis.
Spatial navigation is a fundamental cognitive skill that requires the integration of egocentric (body-based) and allocentric (map-based) frames of orientation. Both frames are required for everyday navigation with egocentric and allocentric processes shifting as a function of navigational demands (McNaughton et al., 2006). Path integration is integral to spatial navigation as it allows an individual to keep track of and return to their starting location based on visual, self-motion, vestibular and proprioceptive feedback which represent the current position and heading direction in references to a permanent location (Etienne and Jeffery, 2004: McNaughton et al., 2006; Knierim et al., 2014). This process involves translating distance traveled with changes in direction of movement either relative to our allocentric or egocentric orientation (Burgess, 2006). Multisensory (visual, self-motion, vestibular and proprioceptive) feedback combine egocentric and allocentric frames of reference, allowing path integration to continuously update this information, allowing one to keep track of one’s position in space (Rieser, 1989; Coughlan et al., 2018b).
Egocentric orientation relies more on the prefrontal and parietal cortex to localize the position of objects relative to the body (Goodale and Milner, 1992; Arnold et al., 2014), the precuneus then uses these location cues to form the basis of an egocentric representation of the surrounding space, integrating self-motion cues with the egocentric reference frame (Wolbers and Wiener, 2014). While allocentric orientation is reliant on the formation of maps using place, grid and boundary vector cells situated mainly in the medial temporal lobe (Lester et al., 2017; Coughlan et al., 2018b). The integration of egocentric and allocentric frames occurs in the retrosplenial cortex (RSC), which is a critical interface between the medial temporal and medial parietal regions (Alexander and Nitz, 2015). Dorsal-medial regions of the RSC are thought to be implicated in orientating and recalling unseen locations from a current position in space, whilst ventrolateral portions were more linked to updating and integrating scene information (Burles et al., 2017).
Tasks that tap into path integration, therefore, provide a promising ecological, cognitive framework to detect medial temporal and medial parietal pathophysiology. Not surprisingly, path integration has been already explored in AD (Morganti et al., 2013; Serino et al., 2014; Vlček and Laczó, 2014; Ritchie et al., 2018) and the advent of VR based testing has allowed such tests to be clinically available (Plancher et al., 2012; Morganti et al., 2013; Parizkova et al., 2018). We have developed previously such a test, the Virtual Supermarket task, which is now used across many large cohorts and drug trials as it can reliably detect path integration differences in preclinical and clinical dementia populations (Tu et al., 2015, 2017). The VR task reliably measures spatial processes of: (i) egocentric self-reference navigation; (ii) allocentric map-based navigation; and (iii) heading direction. For example, we have previously shown that the test allows the distinction of behavioral variant fronto-temporal dementia (bvFTD) from AD, with AD showing particularly problems in switching between egocentric and allocentric frames during path integration (Tu et al., 2017). Importantly, these switching problems in AD were associated with grey matter atrophy in the RSC (Tu et al., 2015).
In contrast to the exciting findings in AD, less is known about path integration in VCI, despite path integration potentially allowing as well to tap into parietal deficits in VCI (Maguire et al., 1998; Wolbers et al., 2004; Papma et al., 2012; Haight et al., 2015). A previous case study by our group explored path integration in a 65-year-old male with VCI. The findings showed that the vascular patient had normal performance on allocentric orientation but a clear and isolated deficit in egocentric and heading direction sub-components of the path integration tasks (Coughlan et al., 2018a). These findings are consistent with frontoparietal network disruptions typically seen in vascular dementia patients (Beason-Held et al., 2012; Sachdev et al., 2014; van der Flier et al., 2018) and may suggest medial parietal changes imped the egocentric frame of reference and subsequent path integration.
The current study leads on from this case study by exploring path integration in a group of VCI patients and importantly comparing them against a group of AD patients and controls. Navigation will be tested using the Virtual Supermarket task where participants move through the virtual environment to a series of locations and are tested on their egocentric, allocentric and heading direction response. We hypothesize that: (i) medial parietal mediated egocentric processes will be more affected in VCI; and (ii) medial temporal mediated allocentric processes will be more affected in AD.
Nine early-stage vascular cognitive impairment and 10 early-stage Alzheimer’s disease patients along with 20 healthy controls were recruited from the community using “Join Dementia Research” to participate in the study at the University of East Anglia as part of the wider The Dementia Research and Care Clinic (TRACC) study. The research was approved by the Faculty of Medicine and Health Sciences Ethics Committee at the University of East Anglia (reference 16/LO/1366) and written informed consent was obtained from all participants. Clinical diagnosis (VCI or AD) was classified by a consultant at the Norfolk and Suffolk Foundation Trust by interviewing the patient, examining neuropsychological assessment scores, structural clinical MRI scans, and the patient’s medical history which met the diagnostic criteria for VCI (see Sachdev et al., 2014) or AD (see Dubois et al., 2007). For clarity, the structural MRI profile of VCI was indicated by subcortical infarcts and white matter hyperintensities, whilst volume loss focused on medial temporal lobes was associated with AD pathology. Disease duration was reported by the person’s study partner (a spouse or relative). Participants had no history of psychiatric or neurological disease, substance dependence disorder or traumatic brain injury and had normal or corrected-to-normal vision. None of the patient’s study partners in this experiment reported problems with spatial orientation before dementia onset or a history of developmental topographical disorientation (Iaria et al., 2009). All participants underwent neuropsychological screening, including cognitive screening, episodic memory and spatial memory tasks, Addenbrooke’s cognitive examination (ACE-III; Hsieh et al., 2013), Rey–Osterrieth Complex Figure Test (RCFT) copy and with 3-min delayed recall (Lezak, 1983), Cube Analysis, Dot Counting and Position Discrimination from the Visual Object and Space Perception Battery (VOSP; Warrington and James, 1991), Free and Cued Selective Reminding Test (FCSRT; Buschke, 1984).
The Virtual Supermarket Task has been developed by our group previously and used in symptomatic mild cognitive impairment (MCI), AD, frontotemporal dementia (FTD) and VCI patients (Tu et al., 2015, 2017; Coughlan et al., 2018a). The VR task is an ecological test of spatial navigation abilities designed to simulate navigating through a real-world supermarket. An iPad 9.7 (Apple Inc.,) was used to show participants 20–40 s video clips of a moving shopping trolley in the virtual supermarket (Figures 1A–C). Videos were presented in a first-person perspective and participants are provided with optic flow cues from the moving shopping trolley and changing scenery as they followed different routes to reach a different endpoint in each trial. The task avoids the use of landmarks or salient features within the environment and limits the demand on episodic memory, reflecting similar tasks in the literature (see Cushman et al., 2008; Morganti et al., 2013; Wolbers et al., 2007) and taps into path integration processes via three core spatial processes: (i) egocentric self-reference navigation; (ii) allocentric map-based navigation; and (iii) heading direction. Once the video clip stops, participants indicate in real-life the direction of their starting point (egocentric orientation; Figure 1D). In a second step, participants indicate their finishing location on a birds-eye view map of the supermarket (allocentric orientation; Figure 1E), performance is calculated using the distance error (mm) between this and the coordinates of the actual finishing location. This map-based component provides an assessment of the geocentric encoding of the virtual environment. The participant then indicates their heading direction at the finishing point, which determines the ability to which heading direction was encoded and updated throughout the task. The tasks consist of 14 trials and takes approximately 15 min to complete.
Figure 1. Screenshots from the Virtual Supermarket task, showing (A) starting viewpoint, (B) movement during the example video clip, (C) end location of an example video clip, (D) onscreen instructions prompting the participant to indicate the direction of their starting point, (E) the supermarket map participants use to indicate their finishing location and their heading direction when the video clip ends.
The Clock Orientation test has also been developed by our lab (Coughlan et al., 2018a) as a bedside clinical test for egocentric orientation. It requires participants to imagine they are standing in the center of a large clock, facing a particular number, e.g., the number 3. Participants are then asked, “Which number is directly behind you?” (Answer: number 9). Next participants are asked to point, in real life, to the positions of different numbers on the clock face in relation to the number that they are currently facing. For example, “You are facing number 12, can you point to the number 3?” (Answer: pointing right). The questions increase in complexity across the test and require medial parietal mediated mental imagery, rotation, and egocentric processes, with no episodic memory demand. The test consists of 12 trials and takes 5–10 min to complete.
Participants completed a battery of neuropsychological assessments at their homes (see Table 1 for a list of tasks). In a second session held at the Norfolk and Suffolk Foundation Trust, participants undertook cognitive experimental tests (including the Virtual Supermarket task and Clock Orientation test) and completed a clinical interview with the Chief Investigator of the study.
Statistical analysis was performed using IBM SPSS (Version 25). Chi-square and two-tailed one-way univariate analysis of covariance (ANCOVA) with age and sex as covariates were used to test the significance of any demographic or neuropsychological differences between the clinical groups. When quantifying group differences, partial eta squared () was used as a measure of effect size. The Virtual Supermarket task has three measures -specifically egocentric response, allocentric response and heading direction. Each outcome measure was individually entered into a one-way ANCOVA with group as the independent variable and age and sex as covariates. Although groups were well matched for age and sex, these covariates were decided as evidence suggests they can affect navigational behavior (Coutrot et al., 2018). The Clock Orientation test was also analyzed using a one-way ANCOVA with group as the independent variable and age and sex as covariates. Post hoc pairwise comparisons were conducted using Bonferroni adjustment for multiple comparisons. The sensitivity and specificity of the egocentric supermarket task and clock orientation test performance in VCI and AD were compared using logistic regression and ROC curve analysis. A Z-score of AD performance was computed for seven missing values for one AD patient in the Virtual Supermarket task.
Participant groups were well matched and no significant differences in demographic measures were observed between the VCI, AD and control groups (all p-values > 0.1). ANOVA of participant groups showed both VCI and AD patients performed significantly lower on a general cognitive screening test (ACE-III) and the memory recall domain of RCFT compared to controls (all p-values < 0.01). Results showed no significant neuropsychological differences between the VCI and AD patients for the ACE-III, RCFT recall condition, VOSP dot counting, and cube analysis sub-sets (all p-values > 0.1. However, VCI patients were significantly more impaired than AD patients in the RCFT copy condition, FCSRT free recall condition and the VOSP position discrimination (all p-values < 0.1; see Table 1).
An ANCOVA with age and sex as covariates revealed a significant differences between egocentric responses on the supermarket task, F(2,34) = 8.14, p < 0.001, = 0.32. Post hoc comparisons revealed significantly greater egocentric impairment in VCI (M = 3.5, SD = 3.24) compared to AD (M = 10.01, SE = 1.11), p < 0.002, 95% CI (−10, −2.1) and control groups (M = 8.1, SD = 3.7), p < 0.009, 95% CI (−7.95, −1.1). No other significant group differences were observed (p > 0.1; see Figure 2A).
Figure 2. Spatial orientation performance between vascular cognitive impairment (VCI), Alzheimer’s disease (AD), and controls. **p < 0.01, ***p < 0.001, ns = non significant. Figures (A–C) show The Virtual Supermarket task performance; (A) egocentric response (correct), (B) allocentric response (error in mm) and (C) heading response (correct). Figure (D) displays The Clock Orientation test egocentric response (correct).
Allocentric responses showed a significance difference between groups, controlled for age and sex F(2,34) = 10.1, p < 0.001, = 0.37. Post hoc comparisons showed significantly greater impairments in VCI patients (M = 68.33, SD = 38.1) compared to controls (M = 30.85, SD = 14.13), p < 0.001, 95% CI (16.02, 61.1) but impairments did not reach statistical significance in AD patients (M = 50.1, SD = 7), p = 0.09, 95% CI (−41.11, 2.1) compared to controls. However, there were no significant groups differences between VCI and AD (p > 0.1; see Figure 2B).
Heading direction (correct judgement of facing direction after travel period) did not reveal significant group differences when controlling for age and sex F(2,34) = 1.11, p > 0.1, = 0.06 (see Figure 2C).
An ANCOVA with age and sex as covariates revealed a significant difference between egocentric responses on the Clock Orientation task F(2,34) = 13.4, p < 0.001, = 0.44. Post hoc comparisons showed significantly greater egocentric deficits in VCI patients (M = 5.42, SD = 3.16) compared to AD (M = 10.1, SD = 1.21), p < 0.001, 95% CI (−7.2, −2) and control groups (M = 9.65, SD = 2.06), p < 0.001, 95% CI (−6.56, −7.1). No other significant group differences were observed (p > 0.1; see Figure 2D).
The sensitivity and specificity of egocentric Virtual Supermarket and Clock Orientation test performance in VCI and AD were explored using logistic regression and ROC curves. Logistic regression indicated that the regression model based on egocentric scores of Supermarket and Clock Orientation predictors was statistically significant, X(2)^2 = 16.36, p < 0.001. The model explained 77% (Nagelkerke R2) of variance in VCI and AD patients and correctly classified 84% of patients (7 out of 9 VCI; 9 out of 10 AD) into their respective cohorts. ROC curves were computed for the supermarket and clock test predictors in discerning VCI from AD patients. Similarly, Area Under the Curve (AUC) values indicated that egocentric orientation in the Supermarket [AUC = 0.8, SE = 0.12; 95% CI (0.56, 1)] and Clock test [AUC = 0.91, SE = 0.06, 95% CI (0.8, 1)] had strong diagnostic accuracy in distinguishing VCI from AD patients (see Figure 3).
Figure 3. ROC curves for Virtual Supermarket task (blue line) and Clock Orientation test (purple line) predicting correct diagnosis (VCI or AD).
Overall, our results indicate that medial parietal mediated egocentric path integration processes are a sensitive and specific cognitive marker selective for VCI. By contrast, allocentric orientation deficits were less sensitive, and not specific to distinguish between the underlying pathologies.
In more detail, the egocentric path integration measures of the Virtual Supermarket task and Clock Orientation test successfully detect vascular changes in patient populations. More importantly, the measures allowed to reliably distinguish vascular from AD pathophysiology in the patient populations. Notably, egocentric orientation was impaired in VCI, but relatively intact in AD patient groups when controlling for age and sex. This supports findings from our vascular patient case study (Coughlan et al., 2018a) and suggests egocentric impairments indicate a more medial parietal focused change (Weniger et al., 2009) in VCI. Furthermore, the AD patient’s egocentric ability remained intact which supports suggestions that MCI and earlier stage AD groups show an undisturbed egocentric orientation (Coughlan et al., 2019), which is consistent with our early-stage AD patient population (see total ACE-III score of 72.1). It would be interesting to explore whether more moderate to advanced AD patients might show problems using both allocentric and egocentric orientation, as it is known that medial parietal structures might be affected only later in the disease course (Braak and Del Tredici, 2015).
The egocentric demands in the virtual Supermarket requires the individual to form an accurate representation of the starting point by integrating virtual self-motion with heading direction to reach their end destination. Path integration plays an important role in updating spatial orientation during self-motion but this process is accumulative, therefore it can be liable to directional errors with respect to the original starting position (McNaughton et al., 2006), which may be responsible for problems observed across both egocentric tasks. The Clock Orientation test also demands path integration to configure the position of numbers on a clock face relative to the individual’s current position. Both tasks rely on accessing scene construction, mental rotation and imagery translated from an egocentric orientation. At the neural level, translation of these egocentric processes depend mainly on the medial parietal cortex (Goodale and Milner, 1992; Galati et al., 2000; Zaehle et al., 2007; Coughlan et al., 2018b) as well as the prefrontal cortex (Spiers, 2008; Bird et al., 2012; Spiers and Barry, 2015), indicating potential disruptions in frontoparietal structures typically seen in vascular patients (Beason-Held et al., 2012; Heiss et al., 2016; Vipin et al., 2018; van der Flier et al., 2018).
Medial parietal mediated egocentric deficits appear to characterize VCI patients. This is consistent with emerging evidence suggesting the earliest signs of dysfunction appear in medial frontal and anterior cingulate regions in at VCI-risk individuals (Papma et al., 2012; Haight et al., 2015), which is accompanied by a more typical vascular profile of reduced integrity of white matter in the bilateral superior longitudinal fasciculus (Beason-Held et al., 2012). Since egocentric orientation does not deteriorate in healthy aging and early-stage AD, compared to medial temporal based cognitive functions (for review, see Colombo et al., 2017) it emerges as a potential powerful cognitive marker to identify early vascular-related pathology. Given the prevalence of vascular-related dementia, it is surprising that investigation to isolate cognitive deficits unique to this pathology is so sparse. However, based on our findings, it appears that egocentric orientation may be a useful diagnostic tool to discriminate VCI from other neurodegenerative conditions.
Our study suggests allocentric orientation deficits were not statistically present in AD, only VCI showed significant impairments compared to healthy controls. This does not support our prediction that allocentric deficits would be more profound in AD. The literature suggests allocentric deficits are more prominent in preclinical AD (Coughlan et al., 2019) with a loss in selectivity as the disease stage progresses and deficits become more widespread (Braak and Del Tredici, 2015). Yet, for the early-stage AD patients in our study results were not significant. A post hoc power analysis was employed using G*Power3 (Faul et al., 2007) and results indicate power at Cohen’s d = 0.32 would have been sufficient to yield significant results between AD and VCI allocentric performance. The actual power yielded between groups was reported at Cohen’s d = 0.71. Therefore, group sizes should have been large enough to yield significant effects. Indeed, as evident from Figure 2, it is clear that AD patients perform differently from controls but this did not reach statistical significance.
One potential explanation for the results observed may be provided by the large range in allocentric scores across the VCI group (see Table 1). VCI is a highly heterogeneous disorder in terms of disease pathology and subsequent cognitive impairments which may account for this variation, compared to AD pathology and symptoms that are more uniform. As VCI patients revealed both egocentric and allocentric orientation problems this is likely to represent a disruption to translational and integration processes where both frames are combined to produce effective navigation. This view also explains the reduced visuospatial performance exhibited by the VCI patients during neuropsychological testing across RCFT copy and position discrimination tasks.
It is also important to consider the domain of memory when interpreting our findings. Results from the FCSRT suggest VCI patients had significantly worse memory than the AD and control groups, sub-score results indicate this is driven by reduced performance during free recall. This is likely due to the retrieval demands on prefrontal and parietal structures (Staresina and Davachi, 2006) which are typically disrupted in VCI. However, when cued VCI patients outperform AD patients. This finding is consistent with evidence that suggests providing a cue has little bearing on improved memory recall in AD (Sarazin et al., 2007; Wagner et al., 2012). This finding may be relevant to the poor allocentric results observed for VCI patients, as reduced retrieval mechanisms may have disrupted their task performance as opposed to pure allocentric (medial temporal) mapping problems, which we would expect to see in the AD patients.
Despite these exciting findings, our study is not without limitations. First and foremost, replication in larger patient cohorts is important. Further, clinical characterization of VCI subtypes (Skrobot et al., 2017) would help to better classify vascular pathology and determine accompanying cognitive symptoms, this may also help inform the variation of results seen in allocentric performance for the VCI patients. Future studies may also wish to examine the relationship between spatial navigation performance and the patient’s perceived navigational abilities. Findings suggest perceived spatial ability assessed by the self-report Santa Barbara Sense of Direction Scale (Hegarty et al., 2002) is correlated with spatial accuracy and hippocampal volume (Burte et al., 2018). Therefore, the assessment of perceived spatial abilities may help inform spatial navigation as a marker of pathological aging. Finally, as the study did not access the patient’s clinical MRI scans, confirmation of vascular lesions and their locations, as well as AD specific biomarkers would be important in the future to corroborate our cognitive findings.
Nevertheless, to our knowledge this in the first study to isolate a selective navigational deficit in VCI. This showcases the important role of virtual navigation and spatial tests in the future development of sensitive and specific diagnostic tests for VCI. Further investigation into the cognitive symptoms selective to VCI as well as longitudinal cohort studies in at VCI-risk individuals is critical to identify the emergence of the disease and intervene with therapeutic strategies as early as possible.
In conclusion, our findings show a distinct egocentric orientation deficit that is specific for VCI relative to AD. This is critical given the lack of specificity in current diagnostic tests and the indistinct diagnostic criteria for cognitive symptoms in VCI. In turn, this will inform diagnostic work-ups and aid personalized treatment pathways to treat underlying vascular changes in patients.
The datasets generated for this study are available on request to the corresponding author.
The studies involving human participants were reviewed and approved by Faculty of Medicine and Health Sciences Research Ethics Committee, University of East Anglia. The patients/participants provided their written informed consent to participate in this study.
EL and MH contributed to the conception and design of the study, statistical analysis and intellectual contribution to the writing of the manuscript. VP, GC, and SJ contributed to the data collection and intellectual contribution to the manuscript.
This study was funded by the University of East Anglia.
The authors declare that the research was conducted in the absence of any commercial or financial relationships that could be construed as a potential conflict of interest.
We would like to thank the Norfolk and Suffolk Foundation Trust and the patients and families involved in the TRACC study.
VCI, vascular cognitive impairment; AD, Alzheimer’s disease.
Alexander, A., and Nitz, D. (2015). Retrosplenial cortex maps the conjunction of internal and external spaces. Nat. Neurosci. 18, 1143–1151. doi: 10.1038/nn.4058
Arnold, A. E., Burles, F., Bray, S., Levy, R. M., and Iaria, G. (2014). Differential neural network configuration during human path integration. Front. Hum. Neurosci. 8:263. doi: 10.3389/fnhum.2014.00263
Beason-Held, L., Thambisetty, M., Deib, G., Sojkova, J., Landman, B., Zonderman, A., et al. (2012). Baseline cardiovascular risk predicts subsequent changes in resting brain function. Stroke 43, 1542–1547. doi: 10.1161/strokeaha.111.638437
Bird, C. M., Bisby, J. A., and Burgess, N. (2012). The hippocampus and spatial constraints on mental imagery. Front. Hum. Neurosci. 6:142. doi: 10.3389/fnhum.2012.00142
Braak, H., and Del Tredici, K. (2015). The preclinical phase of the pathological process underlying sporadic Alzheimer’s disease. Brain 138, 2814–2833. doi: 10.1093/brain/awv236
Burgess, N. (2006). Spatial memory: how egocentric and allocentric combine. Trends Cogn. Sci. 10, 551–557. doi: 10.1016/j.tics.2006.10.005
Burles, F., Slone, E., and Iaria, G. (2017). Dorso-medial and ventro-lateral functional specialization of the human retrosplenial complex in spatial updating and orienting. Brain Struct. Funct. 222, 1481–1493. doi: 10.1007/s00429-016-1288-8
Burte, H., Turner, B. O., Miller, M. B., and Hegarty, M. (2018). The neural basis of individual differences in directional sense. Front. Hum. Neurosci. 12:410. doi: 10.3389/fnhum.2018.00410
Buschke, H. (1984). Cued recall in amnesia. J. Clin. Exp. Neuropsychol. 6, 433–440. doi: 10.1080/01688638408401233
Colombo, D., Serino, S., Tuena, C., Pedroli, E., Dakanalis, A., Cipresso, P., et al. (2017). Egocentric and allocentric spatial reference frames in aging: a systematic review. Neurosci. Biobehav. Rev. 80, 605–621. doi: 10.1016/j.neubiorev.2017.07.012
Coughlan, G., Coutrot, A., Khondoker, M., Minihane, A. M., Spiers, H., and Hornberger, M. (2019). Toward personalized cognitive diagnostics of at-genetic-risk Alzheimer’s disease. Proc. Natl. Acad. Sci. U S A 116, 9285–9292. doi: 10.1073/pnas.1901600116
Coughlan, G., Flanagan, E., Jeffs, S., Bertoux, M., Spiers, H., Mioshi, E., et al. (2018a). Diagnostic relevance of spatial orientation for vascular dementia: a case study. Dement. Neuropsychol. 12, 85–91. doi: 10.1590/1980-57642018dn12-010013
Coughlan, G., Laczó, J., Hort, J., Minihane, A. M., and Hornberger, M. (2018b). Spatial navigation deficits—overlooked cognitive marker for preclinical Alzheimer disease? Nat. Rev. Neurol. 14, 496–506. doi: 10.1038/s41582-018-0031-x
Coutrot, A., Silva, R., Manley, E., de Cothi, W., Sami, S., Bohbot, V. D., et al. (2018). Global determinants of navigation ability. Curr. Biol. 28, 2861–2866. doi: 10.1016/j.cub.2018.06.009
Cushman, L. A., Stein, K., and Duffy, C. J. (2008). Detecting navigational deficits in cognitive aging and Alzheimer disease using virtual reality. Neurology 71, 888–895. doi: 10.1212/01.wnl.0000326262.67613.fe
Dubois, B., Feldman, H. H., Jacova, C., DeKosky, S. T., Barberger-Gateau, P., Cummings, J., et al. (2007). Research criteria for the diagnosis of Alzheimer’s disease: revising the NINCDS-ADRDA criteria. Lancet Neurol. 6, 734–746. doi: 10.1016/S1474-4422(07)70178-3
Etienne, A. S., and Jeffery, K. J. (2004). Path integration in mammals. Hippocampus 14, 180–192. doi: 10.1002/hipo.10173
Faul, F., Erdfelder, E., Lang, A.-G., and Buchner, A. (2007). G*Power 3: a flexible statistical power analysis program for the social, behavioral, and biomedical sciences. Behav. Res. Methods 39, 175–191. doi: 10.3758/bf03193146
Galati, G., Lobel, E., Vallar, G., Berthoz, A., Pizzamiglio, L., and Le Bihan, D. (2000). The neural basis of egocentric and allocentric coding of space in humans: a functional magnetic resonance study. Exp. Brain Res. 133, 156–164. doi: 10.1007/s002210000375
Girard, S., Baranger, K., Gauthier, C., Jacquet, M., Bernard, A., Escoffier, G., et al. (2013). Evidence for early cognitive impairment related to frontal cortex in the 5XFAD mouse model of Alzheimer’s disease. J. Alzheimers Dis. 33, 781–796. doi: 10.3233/jad-2012-120982
Goodale, M., and Milner, A. (1992). Separate visual pathways for perception and action. Trends Neurosci. 15, 20–25. doi: 10.1016/0166-2236(92)90344-8
Goodman, R., Lochner, K., Thambisetty, M., Wingo, T., Posner, S., and Ling, S. (2017). Prevalence of dementia subtypes in united states medicare fee-for-service beneficiaries, 2011–2013. Alzheimers Dement. 13, 28–37. doi: 10.1016/j.jalz.2016.04.002
Guarino, A., Favieri, F., Boncompagni, I., Agostini, F., Cantone, M., and Casagrande, M. (2018). Executive functions in Alzheimer disease: a systematic review. Front. Aging Neurosci. 10:437. doi: 10.3389/fnagi.2018.00437
Hachinski, V., Iadecola, C., Petersen, R., Breteler, M., Nyenhuis, D., Black, S., et al. (2006). National institute of neurological disorders and stroke-canadian stroke network vascular cognitive impairment harmonization standards. Stroke 37, 2220–2241. doi: 10.1161/01.STR.0000237236.88823.47
Haight, T., Bryan, R., Erus, G., Davatzikos, C., Jacobs, D., D’Esposito, M., et al. (2015). Vascular risk factors, cerebrovascular reactivity, and the default-mode brain network. NeuroImage 115, 7–16. doi: 10.1016/j.neuroimage.2015.04.039
Hegarty, M., Richardson, A. E., Montello, D. R., Lovelace, K., and Subbiah, I. (2002). Development of a self-report measure of environmental spatial ability. Intelligence 30, 425–447. doi: 10.1016/s0160-2896(02)00116-2
Heiss, W., Rosenberg, G., Thiel, A., Berlot, R., and de Reuck, J. (2016). Neuroimaging in vascular cognitive impairment: a state-of-the-art review. BMC Med. 14:174. doi: 10.1186/s12916-016-0725-0
Hsieh, S., Schubert, S., Hoon, C., Mioshi, E., and Hodges, J. R. (2013). Validation of the addenbrooke’s cognitive examination III in frontotemporal dementia and Alzheimer’s disease. Dement. Geriatr. Cogn. Disord. 36, 242–250. doi: 10.1159/000351671
Iaria, G., Bogod, N., Fox, C. J., and Barton, J. J. (2009). Developmental topographical disorientation: case one. Neuropsychologia 47, 30–40. doi: 10.1016/j.neuropsychologia.2008.08.021
Knierim, J. J., Neunuebel, J. P., and Deshmukh, S. S. (2014). Functional correlates of the lateral and medial entorhinal cortex: objects, path integration and local-global reference frames. Philos. Trans. R. Soc. Lond. B Biol. Sci. 369:20130369. doi: 10.1098/rstb.2013.0369
Lester, A. W., Moffat, S. D., Wiener, J. M., Barnes, C. A., and Wolbers, T. (2017). The aging navigational system. Neuron 95, 1019–1035. doi: 10.1016/j.neuron.2017.06.037
Maguire, E., Burgess, N., Donnett, J., Frackowiak, R., Frith, C., and O’Keefe, J. (1998). Knowing where and getting there: a human navigation network. Science 280, 921–924. doi: 10.1126/science.280.5365.921
McNaughton, B., Battaglia, F., Jensen, O., Moser, E., and Moser, M. (2006). Path integration and the neural basis of the ‘cognitive map’. Nat. Rev. Neurosci. 7, 663–678. doi: 10.1038/nrn1932
Morganti, F., Stefanini, S., and Riva, G. (2013). From allo- to egocentric spatial ability in early Alzheimer’s disease: a study with virtual reality spatial tasks. Cogn. Neurosci. 4, 171–180. doi: 10.1080/17588928.2013.854762
Neufang, S., Akhrif, A., Riedl, V., Förstl, H., Kurz, A., Zimmer, C., et al. (2011). Disconnection of frontal and parietal areas contributes to impaired attention in very early Alzheimer’s disease. J. Alzheimers Dis. 25, 309–321. doi: 10.3233/jad-2011-102154
Papma, J., den Heijer, T., de Koning, I., Mattace-Raso, F., van der Lugt, A., van der Lijn, F., et al. (2012). The influence of cerebral small vessel disease on default mode network deactivation in mild cognitive impairment. Neuroimage Clin. 2, 33–42. doi: 10.1016/j.nicl.2012.11.005
Parizkova, M., Lerch, O., Moffat, S., Andel, R., Mazancova, A. F., Nedelska, Z., et al. (2018). The effect of Alzheimer’s disease on spatial navigation strategies. Neurobiol. Aging 64, 107–115. doi: 10.1016/j.neurobiolaging.2017.12.019
Plancher, G., Tirard, A., Gyselinck, V., Nicolas, S., and Piolino, P. (2012). Using virtual reality to characterize episodic memory profiles in amnestic mild cognitive impairment and Alzheimer’s disease: influence of active and passive encoding. Neuropsychologia 50, 592–602. doi: 10.1016/j.neuropsychologia.2011.12.013
Rieser, J. (1989). Access to knowledge of spatial structure at novel points of observation. J. Exp. Psychol. Learn. Mem. Cogn. 15, 1157–1165. doi: 10.1037/0278-7393.15.6.1157
Ritchie, K., Carrière, I., Howett, D., Su, L., and Hornberger, M. (2018). Allocentric and egocentric spatial processing in middle-aged adults at high risk of late-onset Alzheimer’s disease: the PREVENT Dementia study. J. Alzheimers Dis. 65, 885–896. doi: 10.3233/jad-180432
Sachdev, P., Kalaria, R., O’Brien, J., Skoog, I., Alladi, S., Black, S., et al. (2014). Diagnostic criteria for vascular cognitive disorders: a VASCOG statement. Alzheimer Dis. Assoc. Disord. 28, 206–218. doi: 10.1097/wad.0000000000000034
Sarazin, M., Berr, C., De Rotrou, J., Fabrigoule, C., Pasquier, F., Legrain, S., et al. (2007). Amnestic syndrome of the medial temporal type identifies prodromal AD: a longitudinal study. Neurology 69, 1859–1867. doi: 10.1212/01.wnl.0000279336.36610.f7
Serino, S., Cipresso, P., Morganti, F., and Riva, G. (2014). The role of egocentric and allocentric abilities in Alzheimer’s disease: a systematic review. Ageing Res. Rev. 16, 32–44. doi: 10.1016/j.arr.2014.04.004
Skrobot, O., O’Brien, J., Black, S., Chen, C., DeCarli, C., Erkinjuntti, T., et al. (2017). The vascular impairment of cognition classification consensus study. Alzheimers Dement. 13, 624–633. doi: 10.1016/j.jalz.2016.10.007
Spiers, H. J. (2008). Keeping the goal in mind: prefrontal contributions to spatial navigation. Neuropsychologia 46, 2106–2108. doi: 10.1016/j.neuropsychologia.2008.01.028
Spiers, H., and Barry, C. (2015). Neural systems supporting navigation. Curr. Opin. Behav. Sci. 1, 47–55. doi: 10.1016/j.cobeha.2014.08.005
Staresina, B. P., and Davachi, L. (2006). Differential encoding mechanisms for subsequent associative recognition and free recall. J. Neurosci. 26, 9162–9172. doi: 10.1523/jneurosci.2877-06.2006
Toledo, J., Arnold, S., Raible, K., Brettschneider, J., Xie, S. X., Grossman, M., et al. (2013). Contribution of cerebrovascular disease in autopsy confirmed neurodegenerative disease cases in the National Alzheimer’s Coordinating Centre. Brain 136, 2697–2706. doi: 10.1093/brain/awt188
Tu, S., Spiers, H. J., Hodges, J. R., Piguet, O., and Hornberger, M. (2017). Egocentric versus allocentric spatial memory in behavioral variant frontotemporal dementia and Alzheimer’s disease. J. Alzheimers Dis. 59, 883–892. doi: 10.3233/jad-160592
Tu, S., Wong, S., Hodges, J., Irish, M., Piguet, O., and Hornberger, M. (2015). Lost in spatial translation—a novel tool to objectively assess spatial disorientation in Alzheimer’s disease and frontotemporal dementia. Cortex 67, 83–94. doi: 10.1016/j.cortex.2015.03.016
van der Flier, W., Skoog, I., Schneider, J., Pantoni, L., Mok, V., Chen, C., et al. (2018). Vascular cognitive impairment. Nat. Rev. Dis. Primers 4:18003. doi: 10.1038/nrdp.2018.3
Vipin, A., Loke, Y., Liu, S., Hilal, S., Shim, H., Xu, X., et al. (2018). Cerebrovascular disease influences functional and structural network connectivity in patients with amnestic mild cognitive impairment and Alzheimer’s disease. Alzheimers Res. Ther. 10:82. doi: 10.1186/s13195-018-0413-8
Vlček, K., and Laczó, J. (2014). Neural correlates of spatial navigation changes in mild cognitive impairment and Alzheimer’s disease. Front. Behav. Neurosci. 8:89. doi: 10.3389/fnbeh.2014.00089
Wagner, M., Wolf, S., Reischies, F. M., Daerr, M., Wolfsgruber, S., Jessen, F., et al. (2012). Biomarker validation of a cued recall memory deficit in prodromal Alzheimer disease. Neurology 78, 379–386. doi: 10.1212/WNL.0b013e318245f447
Warrington, E. K., and James, M. (1991). The Visual Object and Space Battery Perception. Bury St Edmunds: Thames Valley Company.
Weniger, G., Ruhleder, M., Wolf, S., Lange, C., and Irle, E. (2009). Egocentric memory impaired and allocentric memory intact as assessed by virtual reality in subjects with unilateral parietal cortex lesions. Neuropsychologia 47, 59–69. doi: 10.1016/j.neuropsychologia.2008.08.018
Wolbers, T., Weiller, C., and Büchel, C. (2004). Neural foundations of emerging route knowledge in complex spatial environments. Cogn. Brain Res. 21, 401–411. doi: 10.1016/j.cogbrainres.2004.06.013
Wolbers, T., and Wiener, J. M. (2014). Challenges for identifying the neural mechanisms that support spatial navigation: the impact of spatial scale. Front. Hum. Neurosci. 8:571. doi: 10.3389/fnhum.2014.00571
Wolbers, T., Wiener, J. M., Mallot, H. A., and Büchel, C. (2007). Differential recruitment of the hippocampus, medial prefrontal cortex and the human motion complex during path integration in humans. J. Neurosci. 27, 9408–9416. doi: 10.1523/jneurosci.2146-07.2007
Keywords: navigation, egocentric, virtual-reality, dementia, VCI, vascular cognitive impairment, vascular-dementia
Citation: Lowry E, Puthusseryppady V, Coughlan G, Jeffs S and Hornberger M (2020) Path Integration Changes as a Cognitive Marker for Vascular Cognitive Impairment?—A Pilot Study. Front. Hum. Neurosci. 14:131. doi: 10.3389/fnhum.2020.00131
Received: 18 November 2019; Accepted: 20 March 2020;
Published: 21 April 2020.
Edited by:
Ineke Van Der Ham, Leiden University, NetherlandsReviewed by:
Silvia Serino, CHU de Lausanne (CHUV), SwitzerlandCopyright © 2020 Lowry, Puthusseryppady, Coughlan, Jeffs and Hornberger. This is an open-access article distributed under the terms of the Creative Commons Attribution License (CC BY). The use, distribution or reproduction in other forums is permitted, provided the original author(s) and the copyright owner(s) are credited and that the original publication in this journal is cited, in accordance with accepted academic practice. No use, distribution or reproduction is permitted which does not comply with these terms.
*Correspondence: Michael Hornberger, bS5ob3JuYmVyZ2VyQHVlYS5hYy51aw==
Disclaimer: All claims expressed in this article are solely those of the authors and do not necessarily represent those of their affiliated organizations, or those of the publisher, the editors and the reviewers. Any product that may be evaluated in this article or claim that may be made by its manufacturer is not guaranteed or endorsed by the publisher.
Research integrity at Frontiers
Learn more about the work of our research integrity team to safeguard the quality of each article we publish.