- 1Department of Speech and Language Therapy, School of Health Sciences, University of Ioannina, Ioannina, Greece
- 2Laboratory of Biology, Faculty of Medicine, School of Health Sciences, University of Ioannina, Ioannina, Greece
- 3Department of Biomedical Research, Foundation for Research and Technology-Hellas, Institute of Molecular Biology and Biotechnology, Ioannina, Greece
- 4Department of Biological Applications and Technologies, School of Health Sciences, University of Ioannina, Ioannina, Greece
- 5Faculty of Medicine, School of Health Sciences, University of Ioannina, Ioannina, Greece
- 6Neuropsychology Unit, Department of Psychology, IRCCS (National Institute for Research and Treatment) Fondazione Santa Lucia, Sapienza University of Rome, Rome, Italy
- 7First Department of Pediatrics, National and Kapodistrian University of Athens Medical School, “Aghia Sophia” Children’s Hospital, Athens, Greece
Developmental dyslexia (DD) is a multi-system disorder, combining influences of susceptibility genes and environmental factors. The causative interaction between specific genetic factors, brain regions, and personality/mental disorders, as well as specific learning disabilities, has been thoroughly investigated with regard to the approach of developing a multifaceted diagnostic procedure with an intervention strategy potential. In an attempt to add new translational evidence to the interconnection of the above factors in the occurrence of DD, we performed a combinatorial analysis of brain asymmetries, personality traits, cognitive and learning skills, and expression profiles of selected genes in an adult, early diagnosed with DD, and in his son of typical development. We focused on the expression of genes, based on the assumption that the regulation of transcription may be affected by genetic and epigenetic factors. The results highlighted a potential chain link between neuroplasticity-related as well as stress-related genes, such as BDNF, Sox4, mineralocorticoid receptor (MR), and GILZ, leftward asymmetries in the amygdala and selective cerebellum lobules, and tendencies for personality disorders and dyslexia. This correlation may reflect the presence of a specific neuro-epigenetic component of DD, ensuing from the continuous, multifaceted difficulties in the acquisition of cognitive and learning skills, which in turn may act as a fostering mechanism for the onset of long-term disorders. This is in line with recent findings demonstrating a dysfunction in processes supported by rapid neural adaptation in children and adults with dyslexia. Accordingly, the co-evaluation of all the above parameters may indicate a stress-related dyslexia endophenotype that should be carefully considered for a more integrated diagnosis and effective intervention.
Introduction
Developmental dyslexia (DD) is a multi-system neurodevelopmental disorder that affects the ability of individuals to acquire specific learning skills, such as reading, writing, and spelling, despite having normal intelligence, perception instruction, sensory abilities, motivation, and educational opportunities (Spironelli et al., 2008; Peterson and Pennington, 2012; Langer et al., 2017). In the DSM-5™ handbook of differential diagnosis, the term DD is incorporated in an extensive designation, i.e., that of Specific Learning Disorder (SLD; American Psychiatric Association, 2013). In this article, we opt for the term DD since both the diagnostic elements and the bibliographic data are documented according to this terminology.
Background
Personality and DD: Psycho-emotional and Behavioral Outcomes
As originally advocated by Pennington (2006), a new conceptualization of investigating the complex substrate of DD proposes that the association between genetic, neurological, cognitive, psycho-emotional, and behavioral factors as well as their potential involvement in the onset of the symptoms and their continuity during development could better clarify the underlying components of the disease (Zakopoulou et al., 2014; Perrachione et al., 2016; Zoccolotti et al., 2016). DD often shows co-occurrence [Kaplan et al., 2006; also named co-existence (Gillberg, 2010) or comorbidity (Ramus et al., 2013; Ashraf and Najam, 2017)] with other disturbances, and shares common genetic factors with other mental disorders (Grigorenko, 2001; Pennington, 2006). In the context of dyslexia research, the emerging relationship between continuous learning difficulties and personality traits (personality characteristics affecting a person’s behavior, thoughts, and feelings across situations, such as openness to experience, agreeableness, and neuroticism) is of increasing interest (Mason and Mason, 2005; Swanson and Hsieh, 2009; Tsitsas, 2017). Several independent studies support the notion that the frustration and difficulties caused by learning problems per se, both at home and in school, create continuous fear of failure or actual failure, sadness, inadequacy, reduced happiness and self-esteem, anxiety, emotional vulnerability, embarrassment, defensive behaviors, as well as withdrawal (Huc-Chabrolle et al., 2010; Zakopoulou et al., 2013; Bonifacci et al., 2016; Mammarella et al., 2016). Similarly, high levels of tension, anxiety, and depression in students and adolescents with learning disorders (LD) have been reported (Wilson et al., 2009; Panicker and Chelliah, 2016), while Lufi and Awwad (2013) have documented a high probability of test anxiety for adults with LD. Moreover, a significantly lower level of psychosocial health was reported recently for children with LD (Matteucci et al., 2019), and further, in addition to anxiety, such children may have attentional biases specific to reading (Haft et al., 2019), a situation that may, in turn, perpetuate anxiety (Bar-Haim et al., 2007), emphasizing the importance of individualized interventions, considering the psycho- and socio-emotional difficulties in this population.
Stress-HPA Axis, Neuroplasticity, and Epigenetic Reprogramming
Epigenetic mechanisms play a crucial role in the adaptive regulation of gene expression during postnatal life (Rutten and Mill, 2009; Szulwach et al., 2011). Numerous studies investigating the multidisciplinary phenotype of DD support the notion that early or longitudinal experiences of stress (prenatal and antenatal maternal stress, adult social stress) interact with neuroendocrine effectiveness in stressful social behavior and cognitive ability (Gudsnuk and Champagne, 2012; Li et al., 2013; Hostinar et al., 2015; D’Souza et al., 2016). Stress is capable of altering neurotransmission and synaptic plasticity in hypothalamic–pituitary–adrenal (HPA) axis-associated brain regions such as those of the prefrontal cortex (PFC), hippocampus, and amygdala (Gardner et al., 2009; van Bodegom et al., 2017). These regions are all targets of stress hormones known to be involved in dyslexia (Van den Bergh, 2011; Vogel and Schwabe, 2016). Stress exposure activates the HPA axis, resulting in elevation of blood glucocorticoid (GC) levels. The HPA axis is essential for successful adaptation to stress, and its dysfunction, combined with chronic stress exposure, especially early in life, may act as a triggering mechanism that could lead to the development of psychopathology (McEwen and Gianaros, 2011; Buschdorf and Meaney, 2015). GCs bind to two receptor types in the brain, mineralocorticoid receptor (MR) and glucocorticoid receptor (GR), both of which reside in the cytosol and upon GC-binding translocate to the nucleus where they regulate transcription by binding to GC-responsive elements of target genes (Pearce and Yamamoto, 1993). MR and GR mediate the initiation and termination of the HPA axis stress response and modulate acquisition, consolidation, storage, and retrieval of stressful experiences (Sapolsky et al., 2000; Kino and Chrousos, 2004; Montaron et al., 2006; Reul et al., 2015). GILZ has been used as an HPA axis activity measure. Its expression in peripheral blood decreases in chronic stress and social defeat and is correlated with smaller hippocampal volumes (Frodl et al., 2012). BDNF (brain-derived neurotrophic factor) is a key neurotrophic factor implicated in learning and memory, but also in neural plasticity in the amygdala (Rattiner et al., 2005; Cowansage et al., 2010). Mutations in its receptor have been shown to modulate acquisition and consolidation of fear learning (Musumeci et al., 2009). Adult neurogenesis, the birth of new neurons in the adult brain, is considered highly sensitive to environmental stressors (Karten et al., 2005) such as those specifically encountered in learning disabilities/dyslexia. The SoxC proteins play an important role in the genetic network controlling neuronal differentiation in adult neurogenesis, while Sox4 regulates the establishment of neuronal properties and specification of cell fate (Mu et al., 2012).
The Neural-Systems Framework-Brain Asymmetries
Previous neuroimaging studies using functional MRI (fMRI; Shaywitz et al., 2002, 2004) and positron emission tomography (PET; Paulesu et al., 2014) when investigating both children and adults with dyslexia compared to control subjects reported hypo-activity in the left inferior parietal lobe and/or hyperactivity in the left inferior frontal gyrus during phonological awareness tasks such as rhyming judgments. Moreover, original voxel-based morphometry (VBM) studies unequivocally established the involvement of the posterior cerebellum in dyslexia, namely, lobules V, VI, and VII in reading difficulties (Carreiras et al., 2007), Crus I and II in semantic processing, and VIIB in cognitive tasks (Ruz et al., 2005).
Atypical abnormalities of the activity in the left temporal–occipital brain area may play a major role in the recognition of words and the accomplishment of phonological tasks (Kita et al., 2013). These atypical brain activities might underpin impaired phonological awareness in people with dyslexia. However, results from recent studies suggest that the differences found in multiple regions of the dyslexic brains indicate that cerebellar function is not the primary cause of dyslexia, but is rather a fundamental neurodevelopmental abnormality (Perrachione et al., 2016). Various investigations on animals and humans (Kim et al., 2011) testing the performance in cognitive or emotional tasks confirmed a structural and widespread reciprocal connectivity (Freese and Amaral, 2009) between the amygdala, hypothalamus, and ventromedial prefrontal cortex (vmPFC) in the regulation of emotions and social behavior (Arnsten, 2009; Schumann et al., 2009). During threat or uncertainty, the amygdala is activated under tonic inhibitory control from the PFC, having as a result the PFC to be hypoactive (Hänsel and von Känel, 2008; Thayer and Lane, 2009). Structural and functional brain asymmetries have been found in a number of prefrontal areas, mostly in adolescents and males, suggesting that these asymmetries may render them more vulnerable to certain disorders such as autism and dyslexia (Huster et al., 2007; Whittle et al., 2008).
Aim of the Present Study
In this study, we examined whether early stress and stressful learning experiences may constitute underlying predisposing components in the occurrence of DD (Eckert, 2004), in the case of an adult man (GA) diagnosed early with DD, with reported difficulties in manipulating strong emotional situations, and impulsive behavior, even though he presented an endearing personality.
To confirm that GA fulfilled the diagnostic criteria for DD, the DAST test (Fawcett and Nicholson, 1998) was implemented. An “at-risk” profile for dyslexia was highlighted, indicating weaknesses in specific areas related to learning (phonological processing; processing speed; language processing; visual-spatial processing). Moreover, the psychological test MMPI, assessing personality traits and psychopathology (Hathaway and McKinley, 1951), was conducted to check for any possible co-existing disorders. According to this analysis, the psychological profile of the patient showed the characteristics of fearful people with poor self-image and intense anxiety, who find it hard to relax and overcome their fears.
The psychological assessment was complemented with MRI and three-dimensional surface rendering techniques. Taking into account previous evidence showing a reduction of gray matter in specific cerebellar regions associated with DD or other types of dyslexia, such as inferior frontal gyrus, precentral gyrus, medial occipital gyri, frontal and occipital lobes, insula and basal ganglia (Brambati et al., 2004; Zadina et al., 2006), a VBM analysis was performed to investigate potential global volumetric changes in relevant brain regions. This analysis revealed anomalous leftward anatomical asymmetries, consistent with other studies in dyslexic brains (Stoodley and Stein, 2011).
Stressful conditions and experiences have been associated with alterations of HPA axis- and neuroplasticity-related genes, such as the nuclear receptors MR and GR, and the neurotrophin BDNF via epigenetic mechanisms (de Kloet et al., 2005; Unternaehrer et al., 2012). In our analysis, we also included the genes for Ube3A (Ubiquitin-protein ligase E3A), a transcriptional coactivator of steroid hormone receptors associated with neurodevelopmental syndromes and psychological conditions (LaSalle et al., 2015), and GILZ (GC-induced leucine-zipper), a GR/MR-responsive gene that contributes significantly to neural activation and transmitter release and has been linked to HPA axis dysfunction and psychosocial stress (Srinivasan and Lahiri, 2017). Finally, we also monitored genes that are important for neuroplasticity and neurogenesis such as the aforementioned BDNF and Sox4 (Bergsland et al., 2006; Mu et al., 2012). We compared the expression levels of the above genes using whole blood RNA from the patient and his 25-year-old son, a healthy individual, with normal reading ability. This test was repeated 1 year later. As detailed below, the results revealed significant and consistent differences in the mRNA levels of these genes between the two samples that could be related to potential pathological mechanisms underlying this disorder and may encourage the use of such genes as potential peripheral blood-based biomarkers.
GA Case History
GA is a 56-year-old man, right-handed, diagnosed early with DD. According to his medical history and physical examination, GA was physically healthy, without any history of neurologic, psychiatric, or otherwise chronic diseases. The developmental history provided information about GA’s significant, persistent difficulties in reading, writing, and spelling since school age, class repetition, and failure in the national, university entry, examinations. Despite his severe learning disabilities, GA managed to complete his studies and obtain a PhD, and today he is a well-known scientist. However, as became obvious from the clinical interview, his high academic performance was achieved as a result of persistent and intense effort.
GA was referred to the neurological clinic because of visual abnormalities without any coexistent pathology. The brain imaging data and his early life history suggested a complex profile, demanding a more detailed investigation.
An informed consent document was signed by GA and his son, in accordance with the principles of the Declaration of Helsinki.
Materials and Methods
See Supplementary File S1 for Materials and Methods.
Results and Discussion
According to the DAST analysis, GA’s profile indicates very severe difficulties (—) with Rapid Naming and Phonemic Segmentation together with serious problems (—) in One Minute Reading, One Minute Spelling, and Nonverbal Reasoning. Noticeable problems (-) were observed in Postural Stability, Backwards Span, Nonsense Passage, and Verbal Fluency. Relatively good performance (0) was recorded in Writing, while above average performance (+) was recorded in Semantic Fluency. The results (“At Risk” Quotient >1.0) indicate that GA is strongly at risk of dyslexia (Table 1), highlighting his impairments not only in reading and writing skills but also in other cognitive processes [(meta)phonological awareness, memory, reasoning; Callens et al., 2012; Tops et al., 2013]. Considering the MMPI profile, moderate and low T scores in specific scales revealed a personality profile, with mild symptoms of anxiety and tension [two-point code type: 9-7/7-9, corresponding to Mania scale (Ma) and Psychasthenia scale (Pt)], although without symptoms of hypo-mania (or mania), or other forms of psychopathology (Table 1). This is a relatively rare code type that describes people with phobias, who tend to be self-centered and often immature. They may have periods of impulsive-reckless behavior that are a frequent cause of difficulties in interpersonal relationships. These results were combined with the patient’s history information and clinical characteristics, obtained in the context of a clinical interview.
The VBM analysis (Supplementary Table S1) revealed a smaller left amygdala volume compared to age- and sex-matched controls (GA: 0.03 vs. normal range 0.05–0.07), whereas the overall volume of the amygdala was close to the lowest normal value (GA: 0.08 vs. normal range 0.09–0.14). Indeed, the asymmetry index (calculated as the difference between right and left volumes divided by their mean) revealed a significant amygdala asymmetry (GA: +39.59 vs. normal range −16.41/+18.62; Figure 1A and Supplementary Table S1). No other significant differences were found (Supplementary Table S1). With regard to the cerebellum, the VBM lobular analysis showed that each lobule V was increased compared to controls (total; GA: 0.62 vs. normal range 0.21–0.42, right; GA: 0.32 vs. normal range 0.10–0.21, and left; GA: 0.30 vs. normal range 0.10–0.21; Figure 1B and Supplementary File S2). There was also an asymmetry in the volume of lobule Crus II, with the left one being smaller than the right (GA: +22.03 vs. normal range −22.45/+17.66; Supplementary Figure S1 and Supplementary File S2). The left lobule VIIIB was increased and close to the upper limit compared to age- and sex-matched controls (GA: 0.39 vs. normal range 0.19–0.39), while there was an asymmetry between the right and left lobule VIIIB, with the right one being smaller than the left in this case (GA: −36.48 vs. normal range −25.39/+30.73). Both lobules X were decreased (total; GA: 0.08 vs. normal range 0.33–0.70, right; GA: 0.04 vs. normal range 0.16–0.34, and left; GA: 0.04 vs. normal range 0.17–0.35; Supplementary File S2).
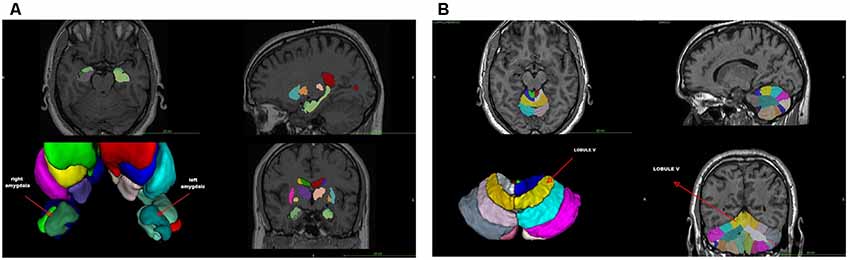
Figure 1. (A) Voxel-based morphometry (VMB) analysis of the cerebrum. (B) VBM lobular analysis of the cerebellum. The location of lobule V is shown (arrow).
The VBM findings reveal anomalous anatomical asymmetries, which support the argument that the cerebellum is one of the main regions associated with dyslexia with consistent differences between critical groups (Eckert, 2004; Stoodley and Stein, 2011; Vandermosten et al., 2016). More importantly, the results from the lobular analysis and the DAST are consistent with the findings of Stanberry et al. (2006), namely, that the asymmetries of lobules V, Crus II, VIIB, and X are involved in difficulties of postural stability, rapid naming, rapid reading, phonemic segmentation, and memory. They are also in line with other studies showing that working memory and fluid intelligence are associated with the dorsolateral prefrontal and anterior cingulate cortex, and that phonological processing, implicit learning, and rapid automatized naming are related to cerebellar asymmetries (Molinari et al., 2008; Stoodley and Stein, 2011, 2013).
Interestingly, although GA was diagnosed with dyslexia quite early, he achieved advanced degrees, indicating that the recorded brain asymmetries do not merely represent differences in reading experience, but rather they may actually contribute with a causative role in dyslexia (Vandermosten et al., 2016). Worthy of mention is that the emotional and/or behavioral characteristics of GA’s profile are not classified in the clinical spectrum, indicating that: (i) the cerebellum asymmetries may be specific to his learning difficulties (Stoodley and Stein, 2011); and (ii) the amygdala asymmetries might reflect alterations in the expression of neuroplasticity genes, which could be triggered by the frustration that the persistent learning difficulties evoke as it has been previously suggested (Blair, 2010), affecting his behavior and stimulus reinforcement learning. However, it would have been certainly interesting to have access to information concerning earlier life periods of the subject examined, as to the form and degree of his dyslexia, because the data presented here only refer to adult findings (Shaywitz et al., 2002). Therefore, whenever possible, a temporal approach should be employed to correlate the critical developmental stages with the natural history of these patients.
The investigation of the gene expression profile was performed by reverse transcriptase-polymerase chain reaction (RT-PCR) using self-designed specific primers (Supplementary Table S2). This analysis (Figure 2) showed that the mRNA levels of the genes tested differed remarkably between the two samples, with the exception of GR, which showed no difference between the patient and his healthy child. The mRNA levels of MR, UBE3A, GILZ, and Sox4 genes were lower in the father than in the son. In contrast, the expression of BDNF was significantly higher in the father. Several studies have recognized the role of epigenetic mechanisms in altering the expression of genes involved in HPA axis function and neuroplasticity after stressful conditions and experiences. Although the data have to be extended to include the analysis of more cases, the differences in the expression profiles of the genes observed here could reflect long-term adaptation and adjustments to changes in the environment. In other words, the father may have acquired epigenetic alterations in critical genes for brain function. One of the most remarkable differences was the very low expression of MR in the blood of the patient although the GR levels were similar. As MR and GR activities oppose each other, the ratio of MR to GR is considered as a marker for stress resilience and vulnerability (Almeida et al., 2000). Provided that this is reflected in relevant brain regions, it may denote an imbalance between GR- and MR-mediated actions in the limbic system, which may lead to inadequate response to stress (de Kloet, 2013). It has been suggested that, during stress, MR provides a negative feedback signal, and low MR functionality may predispose individuals to increased stress susceptibility for psychiatric disorders (Harris et al., 2013). In addition, reduced MR expression in the limbic system may lead to a less favorable strategy to respond properly to a novel, stressful situation (ter Horst et al., 2012). Thus, decreased MR levels may affect stress-related learning and modify the cognitive appraisal of stressful situations (ter Heegde et al., 2015). Another gene, the mRNA levels of which were significantly lower in the father compared to the son, was that of Sox4, which may reflect impaired neurogenesis, and therefore insufficient supply with new neurons that could become integrated into pre-existing neuronal networks. The levels of BDNF mRNA were notably higher in the patient, possibly indicating chronic compensatory mechanisms to cope with stressful events (Autry and Monteggia, 2012; Zaletel et al., 2017).
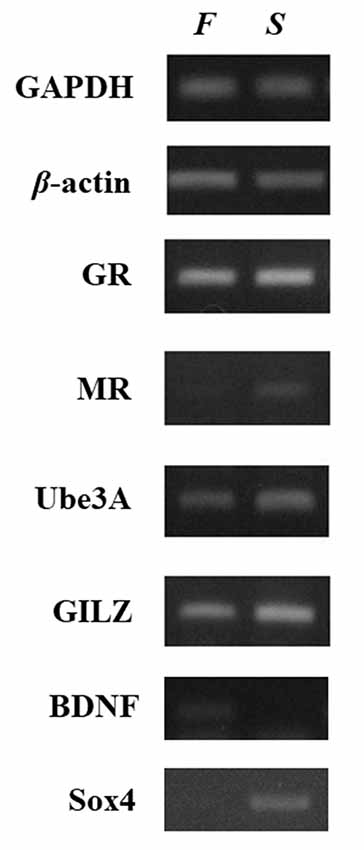
Figure 2. Relative expression of selected neuroplasticity and stress-related genes between father and son. Gene expression was compared in whole blood RNA samples collected from the patient and his son. Relative gene expression levels were determined by semiquantitative reverse transcriptase-polymerase chain reaction (RT-PCR). The levels of GAPDH and β-actin were used as controls for cDNA normalization. Photographs provide one representative example of at least three different experiments that yielded consistent results. The mRNA levels of the genes encoding MR, Ube3A, GILZ, and Sox4 were lower in the sample from the father (F) compared to the son (S). In contrast, the expression of BDNF was significantly higher in the father whereas glucocorticoid receptor (GR) showed no difference.
The analysis of peripheral blood is emerging as a pertinent route for studying relevant gene expression changes in brain disease. For example, chromatin extracted from peripheral blood carries epigenetic marks that reflect individual life experiences. Such epigenetic biomarkers have been associated with various brain disorders, including schizophrenia (Gavin and Sharma, 2009), depression, aggressive behavior, or post-traumatic stress disorder (Rusiecki et al., 2012). Moreover, GILZ mRNA levels in peripheral blood mononuclear cells have been correlated with hippocampal volumes in patients with depression (Frodl et al., 2012). In addition, the methylation of the GR promoter in blood leukocytes has been associated with the history of various childhood adversities (McGowan et al., 2009; Tyrka et al., 2012), while acute psychosocial stress has been shown to alter the DNA methylation status of the BDNF gene in peripheral blood cells (Unternaehrer et al., 2012).
Concluding Remarks
The data analyses on GA point to a dyslexic profile or endophenotype, characterized by altered stress response, MRI findings (asymmetries in the amygdala and specific cerebellar regions), and difficulties in coping with strong emotional and behavioral states. The case findings can be taken to indicate that the cerebellum asymmetries may negatively affect the skills of rapid reading and writing, phonological segmentation and sensorimotor functionality, possibly resulting in a blunted stress response. The asymmetries of the amygdala could indicate an impaired regulation system of the response to threatening stimuli (reading difficulties), which may result in reduced GR-mediated negative feedback on the HPA axis and allow reactive aggression. Given that the long-standing reading difficulties mirror threatening of frustrating conditions, they are considered as stress stimuli; as a result, the limited amygdala activity unable to manipulate negative emotions, supported by a corticosteroid receptor imbalance, might compromise adaptation and activate reactive aggression. In addition, a potential interplay between the dysfunction of particular lobules and amygdala should be taken into account, considering the dynamic changes in gene expression that may occur in the amygdala under threatening conditions, such as the learning difficulties. The significant differences in the expression profiles of the HPA axis and the neuroplasticity genes tested here may reflect long-term adjustments of transcriptional programs to the “threatening” learning environment (Figure 3). Thus, stress might be an important environmental factor that could act in concordance with genetic mutations or alone (epigenetically), resulting in DD endophenotypes and a variation of clinical phenotypes.
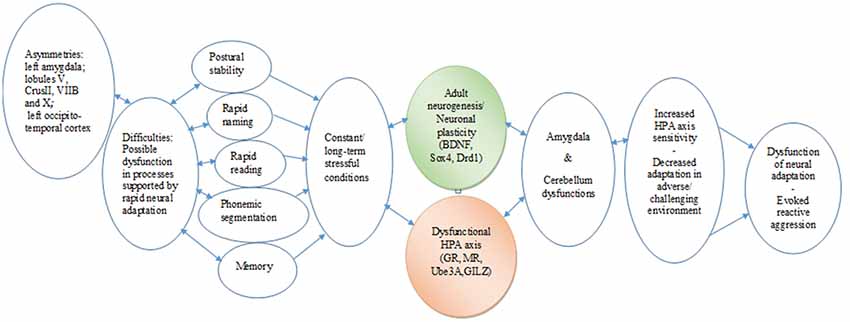
Figure 3. Chain link of brain asymmetries, specific learning disabilities, neurogenesis, hypothalamic–pituitary–adrenal (HPA) axis, and psycho-emotional disorders. The proposed translational mechanism highlights the complex roles that specific brain and genetic asymmetries in constant interaction with the HPA axis may play in the expression and the management of learning disabilities. It also indicates the longitudinal potential consequences in cognitive and emotional development and in the behavioral adaptation of the individual from early childhood to adulthood (Hoeft et al., 2011). In essence, this chain link underscores the core roles of multifaceted associations between neurophysiological and epigenetic development, as well as the adaptation of cognitive and learning mechanisms.
Thus, we propose that the present single case report suggests that, for a more integrated diagnosis and effective treatment, stress-related phenotypes should be carefully considered; in this direction, one should also bear in mind that epigenetic changes induced by environmental factors are dynamic and could be reversed with appropriate intervention processes. This interpretation is in line with current conceptualizations that, in the analysis of complex behaviors (for example, learning disabilities), different components such as cognitive processes, neural systems, and genetic and epigenetic factors should be co-estimated. While the in-depth description of a single case may be highly suggestive, future research involving a sizeable sample of informative individuals will be definitely required in order to reach firm conclusions on this interpretation.
Data Availability Statement
All datasets analyzed for this study are included in the manuscript/Supplementary files.
Ethics Statement
The patient and his son provided us with written informed consent in accordance with the Declaration of Helsinki. They also gave their consent for the analysis, processing, and publication of the data. Since all described interventions were part of clinical practice, we did not consult the ethics committee for this study.
Author Contributions
VZ, MS and TM conceived and designed the experiments and contributed reagents, materials and analysis tools. VZ, A-MV, MD, ZP, DT, KP, GA, HB, VS, MS and TM performed the experiments. VZ, GA, HB, VS, PZ, GC, MS and TM analyzed the data. VZ, A-MV, MD, ZP, DT, GA, HB, VS, PZ, GC, MS and TM wrote the article.
Funding
This work was supported by the 7th Framework Collaborative Program “ILearnRW” of the EU (FP7-504 ICT-2011-8/318803 to VZ) and in part by the Greek national funds through the Operational Program “Education and Lifelong Learning” of the National Strategic Reference Framework (NRSF)–Research Funding Program: “Thalis” (MIS380247-MIREG to TM) and “The National Research Infrastructures on Integrated Structural Biology, Drug Screening Efforts and Drug target functional characterization-Inspired” (MIS5002550 to MS and TM).
Conflict of Interest
The authors declare that the research was conducted in the absence of any commercial or financial relationships that could be construed as a potential conflict of interest.
Acknowledgments
We thank Dr. Theodora Tseligka for editing the manuscript.
Supplementary Material
The Supplementary Material for this article can be found online at: https://www.frontiersin.org/articles/10.3389/fnhum.2019.00327/full#supplementary-material
SUPPLEMENTARY FILE S1 | Materials and Methods.
SUPPLEMENTARY FILE S2 | Volumetry report: the raw data are shown, including a screenshot of the results.
FIGURE S1 | VBM lobular analysis of the cerebellum: the location of lobule Crus II is shown (arrow).
TABLE S1 | Volumetric brain analysis.
TABLE S2 | Primer sequences used for reverse transcription-PCR.
References
Almeida, O. F., Condé, G. L., Crochemore, C., Demeneix, B. A., Fischer, D., Hassan, A. H., et al. (2000). Subtle shifts in the ratio between pro- and antiapoptotic molecules after activation of corticosteroid receptors decide neuronal fate. FASEB J. 14, 779–790. doi: 10.1096/fasebj.14.5.779
American Psychiatric Association. (2013). Diagnostic and Statistical Manual of Mental Disorders (DSM-5®). Arlington, VA: American Psychiatric Publishing.
Arnsten, A. F. (2009). Stress signalling pathways that impair prefrontal cortex structure and function. Nat. Rev. Neurosci. 10, 410–422. doi: 10.1038/nrn2648
Ashraf, F., and Najam, N. (2017). Co-morbidity of specific learning disabilities and depressive symptoms in non-clinical sample of adolescents. J. Postgrad. Med. Inst. 31, 105–109.
Autry, A. E., and Monteggia, L. M. (2012). Brain-derived neurotrophic factor and neuropsychiatric disorders. Pharmacol. Rev. 64, 238–258. doi: 10.1124/pr.111.005108
Bar-Haim, Y., Lamy, D., Pergamin, L., Bakermans-Kranenburg, M. J., and van IJzendoorn, M. H. (2007). Threat-related attentional bias in anxious and nonanxious individuals: a meta-analytic study. Psychol. Bull. 133, 1–24. doi: 10.1037/0033-2909.133.1.1
Bergsland, M., Werme, M., Malewicz, M., Perlmann, T., and Muhr, J. (2006). The establishment of neuronal properties is controlled by Sox4 and Sox11. Genes Dev. 20, 3475–3486. doi: 10.1101/gad.403406
Blair, R. J. (2010). Psychopathy, frustration, and reactive aggression: the role of ventromedial prefrontal cortex. Br. J. Psychol. 101, 383–399. doi: 10.1348/000712609x418480
Bonifacci, P., Storti, M., Tobia, V., and Suardi, A. (2016). Specific learning disorders: a look inside children’s and parents’ psychological well-being and relationships. J. Learn. Disabil. 49, 532–545. doi: 10.1177/0022219414566681
Brambati, S. M., Termine, C., Ruffino, M., Stella, G., Fazio, F., Cappa, S. F., et al. (2004). Regional reductions of gray matter volume in familial dyslexia. Neurology 63, 742–745. doi: 10.1212/01.wnl.0000134673.95020.ee
Buschdorf, J. P., and Meaney, M. J. (2015). Epigenetics/programming in the HPA axis. Compr. Physiol. 6, 87–110. doi: 10.1002/cphy.c140027
Callens, M., Tops, W., and Brysbaert, M. (2012). Cognitive profile of students who enter higher education with an indication of dyslexia. PLoS One 7:e38081. doi: 10.1371/journal.pone.0038081
Carreiras, M., Mechelli, A., Estevez, A., and Price, C. J. (2007). Brain activation for lexical decision and reading aloud: two sides of the same coin? J. Cogn. Neurosci. 19, 433–444. doi: 10.1162/jocn.2007.19.3.433
Cowansage, K. K., LeDoux, J. E., and Monfils, M. H. (2010). Brain-derived neurotrophic factor: a dynamic gatekeeper of neural plasticity. Curr. Mol. Pharmacol. 3, 12–29. doi: 10.2174/1874467211003010012
de Kloet, E. R. (2013). Functional profile of the binary brain corticosteroid receptor system: mediating, multitasking, coordinating, integrating. Eur. J. Pharmacol. 719, 53–62. doi: 10.1016/j.ejphar.2013.04.053
de Kloet, E. R., Joëls, M., and Holsboer, F. (2005). Stress and the brain: from adaptation to disease. Nat. Rev. Neurosci. 6, 463–475. doi: 10.1038/nrn1683
D’Souza, S., Backhouse-Smith, A., Thompson, J. M., Slykerman, R., Marlow, G., Wall, C., et al. (2016). Associations between the KIAA0319 dyslexia susceptibility gene variants, antenatal maternal stress, and reading ability in a longitudinal birth cohort. Dyslexia 22, 379–393. doi: 10.1002/dys.1534
Eckert, M. (2004). Neuroanatomical markers for dyslexia: a review of dyslexia structural imaging studies. Neuroscientist 10, 362–371. doi: 10.1177/1073858404263596
Freese, J. L., and Amaral, D. G. (2009). “Neuroanatomy of the primate amygdala,” in The Human Amygdale, eds P. J. Whalen and E. A. Phelps (New York, NY: Guilford Press), 3–42.
Frodl, T., Carballedo, A., Hughes, M. M., Saleh, K., Fagan, A., Skokauskas, N., et al. (2012). Reduced expression of glucocorticoid-inducible genes GILZ and SGK-1: high IL-6 levels are associated with reduced hippocampal volumes in major depressive disorder. Transl. Psychiatry 2:e88. doi: 10.1038/tp.2012.14
Gardner, K. L., Hale, M. W., Lightman, S. L., Plotsky, P. M., and Lowry, C. A. (2009). Adverse early life experience and social stress during adulthood interact to increase serotonin transporter mRNA expression. Brain Res. 1305, 47–63. doi: 10.1016/j.brainres.2009.09.065
Gavin, D. P., and Sharma, R. P. (2009). Chromatin from peripheral blood mononuclear cells as biomarkers for epigenetic abnormalities in schizophrenia. Cardiovasc. Psychiatry Neurol. 2009:409562. doi: 10.1155/2009/409562
Gillberg, C. (2010). The ESSENCE in child psychiatry: early symptomatic syndromes eliciting neurodevelopmental clinical examinations. Res. Dev. Disabil. 31, 1543–1551. doi: 10.1016/j.ridd.2010.06.002
Grigorenko, E. L. (2001). Developmental dyslexia: an update on genes, brains, and environments. J. Child Psychol. Psychiatry 42, 91–125. doi: 10.1111/1469-7610.00704
Groth-Marnat, G. (2003). Handbook of Psychological Assessment. Hoboken, NJ: John Wiley and Sons, 270–294.
Gudsnuk, K., and Champagne, F. A. (2012). Epigenetic influence of stress and the social environment. ILAR J. 53, 279–288. doi: 10.1093/ilar.53.3-4.279
Haft, S. L., Duong, P. H., Ho, T. C., Hendren, R. L., and Hoeft, F. (2019). Anxiety and attentional bias in children with specific learning disorders. J. Abnorm. Child Psychol. 47, 487–497. doi: 10.1007/s10802-018-0458-y
Hänsel, A., and von Känel, R. (2008). The ventro-medial prefrontal cortex: a major link between the autonomic nervous system, regulation of emotion, and stress reactivity? Biopsychosoc. Med. 2:21. doi: 10.1186/1751-0759-2-21
Harris, A. P., Holmes, M. C., de Kloet, E. R., Chapman, K. E., and Seckl, J. R. (2013). Mineralocorticoid and glucocorticoid receptor balance in control of HPA axis and behaviour. Psychoneuroendocrinology 38, 648–658. doi: 10.1016/j.psyneuen.2012.08.007
Hathaway, S. R., and McKinley, J. C. (1951). Minnesota Multiphasic Personality Inventory; Manual, revised. San Antonio, TX: Psychological Corporation.
Hoeft, F., McCandliss, B. D., Black, J. M., Gantman, A., Zakerani, N., Hulme, C., et al. (2011). Neural systems predicting long-term outcome in dyslexia. Proc. Natl. Acad. Sci. U S A 108, 361–366. doi: 10.1073/pnas.1008950108
Hostinar, C. E., Johnson, A. E., and Gunnar, M. R. (2015). Early social deprivation and the social buffering of cortisol stress responses in late childhood: an experimental study. Dev. Psychol. 51, 1597–1608. doi: 10.1037/dev0000029
Huc-Chabrolle, M., Barthez, M. A., Tripi, G., Barthelemy, C., and Bonnet-Brilhault, F. (2010). Psychocognitive and psychiatric disorders associated with developmental dyslexia: a clinical and scientific issue. Encephale 36, 172–179. doi: 10.1016/j.encep.2009.02.005
Huster, R. J., Westerhausen, R., Kreuder, F., Schweiger, E., and Wittling, W. (2007). Morphologic asymmetry of the human anterior cingulate cortex. Neuroimage 34, 888–895. doi: 10.1016/j.neuroimage.2006.10.023
Kaplan, B., Crawford, S., Cantell, M., Kooistra, L., and Dewey, D. (2006). Comorbidity, co-occurrence, continuum: what’s in a name? Child Care Health Dev. 32, 723–731. doi: 10.1111/j.1365-2214.2006.00689.x
Karten, Y. J., Olariu, A., and Cameron, H. A. (2005). Stress in early life inhibits neurogenesis in adulthood. Trends Neurosci. 28, 171–172. doi: 10.1016/j.tins.2005.01.009
Kim, M. J., Loucks, R. A., Palmer, A. L., Brown, A. C., Solomon, K. M., Marchante, A. N., et al. (2011). The structural and functional connectivity of the amygdala: from normal emotion to pathological anxiety. Behav. Brain Res. 223, 403–410. doi: 10.1016/j.bbr.2011.04.025
Kino, T., and Chrousos, G. P. (2004). Glucocorticoid and mineralocorticoid receptors and associated diseases. Essays Biochem. 40, 137–155. doi: 10.1042/bse0400137
Kita, Y., Yamamoto, H., Oba, K., Terasawa, Y., Moriguchi, Y., Uchiyama, H., et al. (2013). Altered brain activity for phonological manipulation in dyslexic Japanese children. Brain 136, 3696–3708. doi: 10.1093/brain/awt248
Langer, N., Peysakhovich, B., Zuk, J., Drottar, M., Sliva, D. D., Smith, S., et al. (2017). White matter alterations in infants at risk for developmental dyslexia. Cereb. Cortex 27, 1027–1036. doi: 10.1093/cercor/bhv281
LaSalle, J. M., Reiter, L. T., and Chamberlain, S. J. (2015). Epigenetic regulation of UBE3A and roles in human neurodevelopmental disorders. Epigenomics 7, 1213–1228. doi: 10.2217/epi.15.70
Li, J., Robinson, M., Malacova, E., Jacoby, P., Foster, J., and van Eekelen, A. (2013). Maternal life stress events in pregnancy link to children’s school achievement at age 10 years. J. Pediatr. 162, 483–489. doi: 10.1016/j.jpeds.2012.09.007
Lufi, D., and Awwad, A. (2013). Using the minnesota multiphasic personality inventory-2 to develop a scale to identify test anxiety among students with learning disabilities. Learn. Disabil. Q. 36, 242–249. doi: 10.1177/0731948712471199
Mammarella, I. C., Ghisi, M., Bomba, M., Bottesi, G., Caviola, S., Broggi, F., et al. (2016). Anxiety and depression in children with nonverbal learning disabilities, reading disabilities, or typical development. J. Learn. Disabil. 49, 130–139. doi: 10.1177/0022219414529336
Manjón, J. V., and Coupé, P. (2016). volBrain: an online MRI brain volumetry system. Front. Neuroinform. 10:30. doi: 10.3389/fninf.2016.00030
Mason, A., and Mason, M. (2005). Understanding college students with learning disabilities. Pediatr. Clin. North Am. 52, 61–70. doi: 10.1016/j.pcl.2004.11.001
Matteucci, M. C., Scalone, L., Tomasetto, C., Cavrini, G., and Selleri, P. (2019). Health-related quality of life and psychological wellbeing of children with specific learning disorders and their mothers. Res. Dev. Disabil. 87, 43–53. doi: 10.1016/j.ridd.2019.02.003
McEwen, B. S., and Gianaros, P. J. (2011). Stress- and allostasis-induced brain plasticity. Annu. Rev. Med. 62, 431–445. doi: 10.1146/annurev-med-052209-100430
McGowan, P. O., Sasaki, A., D’Alessio, A. C., Dymov, S., Labonte, B., Szyf, M., et al. (2009). Epigenetic regulation of the glucocorticoid receptor in human brain associates with childhood abuse. Nat. Neurosci. 12, 342–348. doi: 10.1038/nn.2270
Molinari, M., Chiricozzi, F. R., Clausi, S., Tedesco, A. M., De Lisa, M., and Leggio, M. G. (2008). Cerebellum and detection of sequences, from perception to cognition. Cerebellum 7, 611–615. doi: 10.1007/s12311-008-0060-x
Montaron, M. F., Drapeau, E., Dupret, D., Kitchener, P., Aurousseau, C., Le Moal, M., et al. (2006). Lifelong corticosterone level determines age-related decline in neurogenesis and memory. Neurobiol. Aging 27, 645–654. doi: 10.1016/j.neurobiolaging.2005.02.014
Mu, L., Berti, L., Masserdotti, G., Covic, M., Michaelidis, T. M., Doberauer, K., et al. (2012). SoxC transcription factors are required for neuronal differentiation in adult hippocampal neurogenesis. J. Neurosci. 32, 3067–3080. doi: 10.1523/jneurosci.4679-11.2012
Musumeci, G., Sciarretta, C., Rodriguez-Moreno, A., Al Banchaabouchi, M., Negrete-Diaz, V., Costanzi, M., et al. (2009). TrkB modulates fear learning and amygdalar synaptic plasticity by specific docking sites. J. Neurosci. 29, 10131–10143. doi: 10.1523/JNEUROSCI.1707-09.2009
Panicker, A. S., and Chelliah, A. (2016). Resilience and stress in children and adolescents with specific learning disability. J. Can. Acad. Child Adolesc. Psychiatry 25, 17–23.
Paulesu, E., Danelli, L., and Berlingeri, M. (2014). Reading the dyslexic brain: multiple dysfunctional routes revealed by a new meta-analysis of PET and fMRI activation studies. Front. Hum. Neurosci. 8:830. doi: 10.3389/fnhum.2014.00830
Pearce, D., and Yamamoto, K. R. (1993). Mineralocorticoid and glucocorticoid receptor activities distinguished by nonreceptor factors at a composite response element. Science 259, 1161–1165. doi: 10.1126/science.8382376
Pennington, B. F. (2006). From single to multiple deficit models of developmental disorders. Cognition 101, 385–413. doi: 10.1016/j.cognition.2006.04.008
Perrachione, T. K., Del Tufo, S. N., Winter, R., Murtagh, J., Cyr, A., Chang, P., et al. (2016). Dysfunction of rapid neural adaptation in dyslexia. Neuron 92, 1383–1397. doi: 10.1016/j.neuron.2016.11.020
Peterson, R. L., and Pennington, B. F. (2012). Developmental dyslexia. Lancet 379, 1997–2007. doi: 10.1016/S0140-6736(12)60198-6
Ramus, F., Marshall, C. R., Rosen, S., and van der Lely, H. K. (2013). Phonological deficits in specific language impairment and developmental dyslexia: towards a multidimensional model. Brain 136, 630–645. doi: 10.1093/brain/aws356
Rattiner, L. M., Davis, M., and Ressler, K. J. (2005). Brain-derived neurotrophic factor in amygdala-dependent learning. Neuroscientist 11, 323–333. doi: 10.1177/1073858404272255
Reul, J. M., Collins, A., Saliba, R. S., Mifsud, K. R., Carter, S. D., Gutierrez-Mecinas, M., et al. (2015). Glucocorticoids, epigenetic control and stress resilience. Neurobiol. Stress 1, 44–59. doi: 10.1016/j.ynstr.2014.10.001
Rusiecki, J. A., Chen, L., Srikantan, V., Zhang, L., Yan, L., Polin, M. L., et al. (2012). DNA methylation in repetitive elements and post-traumatic stress disorder: a case-control study of US military service members. Epigenomics 4, 29–40. doi: 10.2217/epi.11.116
Rutten, B. P., and Mill, J. (2009). Epigenetic mediation of environmental influences in major psychotic disorders. Schizophr. Bull. 35, 1045–1056. doi: 10.1093/schbul/sbp104
Ruz, M., Wolmetz, M. E., Tudela, P., and McCandliss, B. D. (2005). Two brain pathways for attended and ignored words. Neuroimage 27, 852–861. doi: 10.1016/j.neuroimage.2005.05.031
Sapolsky, R. M., Romero, L. M., and Munck, A. U. (2000). How do glucocorticoids influence stress responses? Integrating permissive, suppressive, stimulatory, and preparative actions. Endocr. Rev. 21, 55–89. doi: 10.1210/er.21.1.55
Schumann, C. M., Barnes, C. C., Lord, C., and Courchesne, E. (2009). Amygdala enlargement in toddlers with autism related to severity of social and communication impairments. Biol. Psychiatry 66, 942–949. doi: 10.1016/j.biopsych.2009.07.007
Shaywitz, B. A., Shaywitz, S. E., Blachman, B. A., Pugh, K. R., Fulbright, R. K., Skudlarski, P., et al. (2004). Development of left occipitotemporal systems for skilled reading in children after a phonologically-based intervention. Biol. Psychiatry 55, 926–933. doi: 10.1016/j.biopsych.2003.12.019
Shaywitz, B. A., Shaywitz, S. E., Pugh, K. R., Mencl, W. E., Fulbright, R. K., Skudlarski, P., et al. (2002). Disruption of posterior brain systems for reading in children with developmental dyslexia. Biol. Psychiatry 52, 101–110. doi: 10.1016/s0006-3223(02)01365-3
Spironelli, C., Penolazzi, B., and Angrilli, A. (2008). Dysfunctional hemispheric asymmetry of theta and beta EEG activity during linguistic tasks in developmental dyslexia. Biol. Psychol. 77, 123–131. doi: 10.1016/j.biopsycho.2007.09.009
Srinivasan, M., and Lahiri, D. K. (2017). Glucocorticoid-induced leucine zipper in central nervous system health and disease. Mol. Neurobiol. 54, 8063–8070. doi: 10.1007/s12035-016-0277-5
Stanberry, L. I., Richards, T. L., Berninger, V. W., Nandy, R. R., Aylward, E. H., Maravilla, K. R., et al. (2006). Low-frequency signal changes reflect differences in functional connectivity between good readers and dyslexics during continuous phoneme mapping. Magn. Reson. Imaging 24, 217–229. doi: 10.1016/j.mri.2005.12.006
Stoodley, C. J., and Stein, J. F. (2011). The cerebellum and dyslexia. Cortex 47, 101–116. doi: 10.1016/j.cortex.2009.10.005
Stoodley, C. J., and Stein, J. F. (2013). Cerebellar function in developmental dyslexia. Cerebellum 12, 267–276. doi: 10.1007/s12311-012-0407-1
Swanson, H. L., and Hsieh, C. J. (2009). Reading disabilities in adults: a selective meta-analysis of the literature. Rev. Educ. Res. 79, 1362–1390. doi: 10.3102/0034654309350931
Szulwach, K. E., Li, X., Li, Y., Song, C. X., Wu, H., Dai, Q., et al. (2011). 5-hmC-mediated epigenetic dynamics during postnatal neurodevelopment and aging. Nat. Neurosci. 14, 1607–1616. doi: 10.1038/nn.2959
ter Heegde, F., De Rijk, R. H., and Vinkers, C. H. (2015). The brain mineralocorticoid receptor and stress resilience. Psychoneuroendocrinology 52, 92–110. doi: 10.1016/j.psyneuen.2014.10.022
ter Horst, J. P., Carobrez, A. P., van der Mark, M. H., de Kloet, E. R., and Oitzl, M. S. (2012). Sex differences in fear memory and extinction of mice with forebrain-specific disruption of the mineralocorticoid receptor. Eur. J. Neurosci. 36, 3096–3102. doi: 10.1111/j.1460-9568.2012.08237.x
Thayer, J. F., and Lane, R. D. (2009). Claude Bernard and the heart-brain connection: further elaboration of a model of neurovisceral integration. Neurosci. Biobehav. Rev. 33, 81–88. doi: 10.1016/j.neubiorev.2008.08.004
Tops, W., Verguts, E., Callens, M., and Brysbaert, M. (2013). Do students with dyslexia have a different personality profile as measured with the big five? PLoS One 8:e64484. doi: 10.1371/journal.pone.0064484
Tsitsas, G. (2017). How personality traits relate to the self-esteem of Greek children and adolescents with dyslexia. J. Pedagog. Dev. 7, 1–14.
Tyrka, A. R., Price, L. H., Marsit, C., Walters, O. C., and Carpenter, L. L. (2012). Childhood adversity and epigenetic modulation of the leukocyte glucocorticoid receptor: preliminary findings in healthy adults. PLoS One 7:e30148. doi: 10.1371/journal.pone.0030148
Unternaehrer, E., Luers, P., Mill, J., Dempster, E., Meyer, A. H., Staehli, S., et al. (2012). Dynamic changes in DNA methylation of stress-associated genes (OXTR, BDNF) after acute psychosocial stress. Transl. Psychiatry 2:e150. doi: 10.1038/tp.2012.77
van Bodegom, M., Homberg, J. R., and Henckens, M. (2017). Modulation of the hypothalamic-pituitary-adrenal axis by early life stress exposure. Front. Cell. Neurosci. 11:87. doi: 10.3389/fncel.2017.00087
Van den Bergh, B. R. (2011). Developmental programming of early brain and behaviour development and mental health: a conceptual framework. Dev. Med. Child Neurol. 53, 19–23. doi: 10.1111/j.1469-8749.2011.04057.x
Vandermosten, M., Hoeft, F., and Norton, E. S. (2016). Integrating MRI brain imaging studies of pre-reading children with current theories of developmental dyslexia: a review and quantitative meta-analysis. Curr. Opin. Behav. Sci. 10, 155–161. doi: 10.1016/j.cobeha.2016.06.007
Vogel, S., and Schwabe, L. (2016). Stress in the zoo: tracking the impact of stress on memory formation over time. Psychoneuroendocrinology 71, 64–72. doi: 10.1016/j.psyneuen.2016.04.027
Whittle, S., Yap, M. B., Yucel, M., Fornito, A., Simmons, J. G., Barrett, A., et al. (2008). Prefrontal and amygdala volumes are related to adolescents’ affective behaviors during parent-adolescent interactions. Proc. Natl. Acad. Sci. U S A 105, 3652–3657. doi: 10.1073/pnas.0709815105
Wilson, A. M., Armstrong, C. D., Furrie, A., and Walcot, E. (2009). The mental health of Canadians with self-reported learning disabilities. J. Learn. Disabil. 42, 24–40. doi: 10.1177/0022219408326216
Zadina, J. N., Corey, D. M., Casbergue, R. M., Lemen, L. C., Rouse, J. C., Knaus, T. A., et al. (2006). Lobar asymmetries in subtypes of dyslexic and control subjects. J. Child Neurol. 21, 922–931. doi: 10.1177/08830738060210110201
Zakopoulou, V., Mavreas, V., Christodoulides, P., Lavidas, A., Fili, E., Georgiou, G., et al. (2014). Specific learning difficulties: a retrospective study of their co morbidity and continuity as early indicators of mental disorders. Res. Dev. Disabil. 35, 3496–3507. doi: 10.1016/j.ridd.2014.07.040
Zakopoulou, V., Pashou, T., Tzavelas, P., Christodoulides, P., Anna, M., and Iliana, K. (2013). Learning difficulties: a retrospective study of their co morbidity and continuity as indicators of adult criminal behaviour in 18–70-year-old prisoners. Res. Dev. Disabil. 34, 3660–3671. doi: 10.1016/j.ridd.2013.08.033
Zaletel, I., Filipovic, D., and Puskas, N. (2017). Hippocampal BDNF in physiological conditions and social isolation. Rev. Neurosci. 28, 675–692. doi: 10.1515/revneuro-2016-0072
Keywords: dyslexia, brain asymmetries, stress, hypothalamic–pituitary–adrenal (HPA) axis, neuroplasticity genes, BDNF, MR
Citation: Zakopoulou V, Vlaikou A-M, Darsinou M, Papadopoulou Z, Theodoridou D, Papageorgiou K, Alexiou GA, Bougias H, Siafaka V, Zoccolotti P, Chroussos GP, Syrrou M and Michaelidis TM (2019) Linking Early Life Hypothalamic–Pituitary–Adrenal Axis Functioning, Brain Asymmetries, and Personality Traits in Dyslexia: An Informative Case Study. Front. Hum. Neurosci. 13:327. doi: 10.3389/fnhum.2019.00327
Received: 19 February 2019; Accepted: 05 September 2019;
Published: 01 October 2019.
Edited by:
Guadalupe Dávila, University of Málaga, SpainReviewed by:
Maria E. Grigoriou, Democritus University of Thrace, GreeceJohn Frederick Stein, University of Oxford, United Kingdom
Copyright © 2019 Zakopoulou, Vlaikou, Darsinou, Papadopoulou, Theodoridou, Papageorgiou, Alexiou, Bougias, Siafaka, Zoccolotti, Chroussos, Syrrou and Michaelidis. This is an open-access article distributed under the terms of the Creative Commons Attribution License (CC BY). The use, distribution or reproduction in other forums is permitted, provided the original author(s) and the copyright owner(s) are credited and that the original publication in this journal is cited, in accordance with accepted academic practice. No use, distribution or reproduction is permitted which does not comply with these terms.
*Correspondence: Victoria Zakopoulou, dnpha29wQHVvaS5ncg==; Maria Syrrou, bXN5cnJvdUB1b2kuZ3I=; Theologos M. Michaelidis, dGhlb2xvZ29zX21pY2hhaWxpZGlzQGltYmIuZm9ydGguZ3I=; dG1pY2hhZWxAdW9pLmdy