- 1Faculty of Science, School of Psychology, University of Auckland, Auckland, New Zealand
- 2Centre for Brain Research, University of Auckland, Auckland, New Zealand
- 3Brain Research New Zealand, Auckland, New Zealand
- 4Psychedelic Research Group, Division of Brain Sciences, Centre for Psychiatry, Imperial College London, London, United Kingdom
- 5School of Psychology, University of Sydney, Sydney, NSW, Australia
- 6Department of Psychology, University of Trier, Trier, Germany
- 7Faculty of Medical and Health Sciences, School of Pharmacy, University of Auckland, Auckland, New Zealand
- 8Boston Scientific, Mascot, NSW, Australia
- 9Department of Obstetrics & Gynaecology, Faculty of Medical and Health Sciences, University of Auckland, Auckland, New Zealand
- 10Medical Science, University of Idaho, Moscow, ID, United States
- 11School of Pharmacy, University of Otago, Dunedin, New Zealand
Background: Long-term potentiation (LTP) is recognised as a core neuronal process underlying long-term memory. However, a direct relationship between LTP and human memory performance is yet to be demonstrated. The first aim of the current study was thus to assess the relationship between LTP and human long-term memory performance. With this also comes an opportunity to explore factors thought to mediate the relationship between LTP and long-term memory. The second aim of the current study was to explore the relationship between LTP and memory in groups differing with respect to brain-derived neurotrophic factor (BDNF) Val66Met; a single-nucleotide polymorphism (SNP) implicated in memory function.
Methods: Participants were split into three genotype groups (Val/Val, Val/Met, Met/Met) and were presented with both an EEG paradigm for inducing LTP-like enhancements of the visually-evoked response, and a test of visual memory.
Results: The magnitude of LTP 40 min after induction was predictive of long-term memory performance. Additionally, the BDNF Met allele was associated with both reduced LTP and reduced memory performance.
Conclusions: The current study not only presents the first evidence for a relationship between sensory LTP and human memory performance, but also demonstrates how targeting this relationship can provide insight into factors implicated in variation in human memory performance. It is anticipated that this will be of utility to future clinical studies of disrupted memory function.
Introduction
First demonstrated in vivo in 1973 (Bliss and Lømo, 1973), long-term potentiation (LTP) has since been widely recognised as the principal model for the neuronal basis of long-term memory. LTP is an enduring facilitation of synaptic transmission between neurons that follows repeated co-activation of the neurons in a network (Teyler and DiScenna, 1987; Martin et al., 2000; Cooke and Bliss, 2006). The cellular and molecular mechanisms of LTP have been studied extensively in vivo and in vitro in laboratory animals, which typically involves the application of direct neuronal electrical stimulation and results in an enhancement of the response in a neighbouring cell (Bliss and Lømo, 1973; Harris et al., 1984; Teyler and DiScenna, 1987; Kirkwood and Bear, 1994; Figurov et al., 1996). Such studies have demonstrated that, in its most widespread form, LTP is dependent on the influx of Ca2+ through N-methyl-D-aspartate (NMDA) receptors, leading to long-term alterations in cell structure and function, and an increase in synaptic efficacy. However, a direct relationship between LTP and memory performance has been notoriously difficult to demonstrate. In vivo rodent studies have typically focused on spatial memory as a measure of memory function (Lynch, 2004). While this is an accessible measure of memory, the disparate results from such studies indicate that it may not be the most appropriate index of LTP. As such, a definitive demonstration of the relationship between LTP and memory performance remains elusive.
Using similar induction protocols, the properties of human LTP have been shown to be consistent with those seen in animals (Chen et al., 1996; Beck et al., 2000). However, due to the invasive nature of these procedures, such studies have been limited to excised human tissue. The sensory LTP paradigm presents one of the first opportunities for the non-invasive in vivo study of an LTP-like shift in event related-potentials (ERPs) in humans. First presented by Teyler et al. (2005), the sensory LTP paradigm typically involves presenting participants with high-frequency (~9 Hz) visual stimulation, which leads to an enhancement of the visually-evoked potential (VEP) to subsequent low-frequency (~1 Hz) presentations of the same stimulus. This enhancement has been shown to conform to many of the Hebbian characteristics of LTP (Clapp et al., 2006; McNair et al., 2006; Ross et al., 2008), and is generated by a bottom-up modulation of connection strength between occipital and temporal regions (Spriggs et al., 2018). As such, this experience-dependent enhancement of the VEP is understood to represent the induction of an LTP-like form of neuroplasticity (Kirk et al., 2010; Clapp et al., 2012).
As the sensory LTP paradigm can be used non-invasively with humans, it provides a novel avenue for assessing the pivotal relationship between LTP and human long-term memory performance. The primary aim of the current study was thus to assess this relationship using the visual LTP paradigm and two subtests of the Wechsler Memory Scale-III (WMS-III; Wechsler et al., 2007) that are widely used in clinical assessments of delayed visual recognition memory (Conklin et al., 2002; Keilp et al., 2006; Seelye et al., 2009; Vann et al., 2009). It was hypothesised that individuals with greater LTP magnitude would also demonstrate greater memory performance.
With the ability to study LTP and memory performance comes an unique opportunity to also study the role that LTP alterations play in variations in human memory performance. Previous studies using the human sensory LTP paradigm have demonstrated modulated LTP in healthy populations differing in physical fitness (Smallwood et al., 2015), age (de Gobbi Porto et al., 2015; Spriggs et al., 2017a), and genetics (Spriggs et al., 2017b), as well as in clinical conditions such as depression (Normann et al., 2007) and schizophrenia (Çavuş et al., 2012). However, how these differences in LTP magnitude impact upon memory performance is yet to be assessed.
One factor implicated in healthy variations in human memory performance is the gene that controls the secretion of brain-derived neurotrophic factor (BDNF). BDNF regulates neuronal proliferation and differentiation in the developing brain, and is an important molecular mediator of synaptic plasticity in the mature brain (Tyler et al., 2002; Goldberg and Weinberger, 2004; Park and Poo, 2013). In humans, approximately 25%–50% of the population (Shimizu et al., 2004) carry a single nucleotide-polymorphism (SNP) on the BDNF gene, which substitutes valine to methionine at codon 66 (Val66Met; SNP rs6265). The Met allele of the polymorphism has been associated with reduced declarative memory performance (Kambeitz et al., 2012). However, it is unclear whether these genotype differences are due to the regulatory role of BDNF in brain development (Pezawas et al., 2004) or due to the modulation of synaptic plasticity (Egan et al., 2003; Hariri et al., 2003; Spriggs et al., 2018). The secondary aim of this study was thus to explore the effect of the polymorphism on both visual LTP and visual long-term memory. We hypothesised a consistent genotype difference across the two measures (LTP and memory), which would support the notion that the polymorphism mediates long-term memory performance through an effect on LTP.
Materials and Methods
Subjects
Fifteen females (all right-handed) and thirteen males (three left-handed) with a mean age of 24.2 years (range 21–35; SD = 3.3 years) took part in this experiment. All participants had normal or corrected-to-normal vision. All subjects gave written informed consent in accordance with the Declaration of Helsinki. All experimental procedures were approved by the University of Auckland Human Participants Ethics Committee.
BDNF Genotyping
DNA was extracted from blood samples using the method described in previous literature (Miller et al., 1988), and were analysed by the Auckland Sequenom Facility. Amplification was carried out on the 113 bp polymorphic BDNF fragment using a polymerase chain reaction (PCR), with Taq polymerase and the following primers: BDNF-F 5′-GAG GCT TGC CAT CAT TGG CT-3′ and BDNF-R 5′-CGT GTA CAA GTC TGC GTC CT-3′. PCR conditions were as follows: denaturation at 95°C for 15 min, 30 cycles on a thermocycler (denaturation at 94°C for 30 s annealing at 60°C for 30 s and extension at 72°C for 30 s with a final extension at 72°C. The PCR product (6.5 μL) was incubated with PmlI at 37°C overnight and digestion products were analysed using a High-res agarose gel (4%) with a Quick load 100 bp ladder (BioLabs, Ipswich, MA, USA) and a GelPilot Loading Dye (QIAGEN). Digestion resulted in a 113 bp fragment for the Met66 allele and this was cut into 78 and 35 bp fragments for the Val66 allele. Subjects were divided into three groups defined by Val66Met genotype (10 Val/Val, 10 Val/Met and 8 Met/Met).
Memory Performance
Memory performance was assessed using two subtests of the WMS-III (Wechsler et al., 2007): the Faces task, and the Family Pictures task.
The Faces task involved presenting participants with a set of 24 faces for 2 s each that they were asked to remember. After a 30 min delay, participants were tasked with identifying the original faces (make an “old/new” decision) from a selection of 48 faces which included the 24 original faces as well as 24 new faces.
For the Family Pictures task, participants were presented with pictures of a family in a variety of scenes for 10 s per scene. Again, after a 30 min delay, participants were asked to recall details about each scene.
For both tasks, performance was scored as percentage correct. An average score for the two tasks was then used as an index of visual-memory performance.
Apparatus
EEG data were recorded using a 128-channel Ag/AgCl electrode net (Electrode Geodesics Inc., Eugene, OR, USA) at a continuous sampling rate of 1,000 Hz (0.1–100 Hz analogue bandpass filter) with impedance kept below 40 kΩ. EEG data were recorded using a common vertex reference (Cz) and later re-referenced to average offline. Stimuli were presented on an SVGA computer monitor (1,024 × 768 pixel resolution; 60 Hz refresh rate).
Stimuli
Stimuli consisted of two circles containing black and white sine gratings of horizontal and vertical orientation with a spatial frequency of one cycle per degree (size: 9.6 × 9.6 cm, 272 × 272 pixels; Figure 1A). Stimuli were presented in full contrast against a grey background in the centre of the screen, subtending a diameter of 8° of visual angle. A red fixation dot was present throughout testing. Stimulus presentation was controlled using E-Prime v1.1 (Psychology Software Tools).
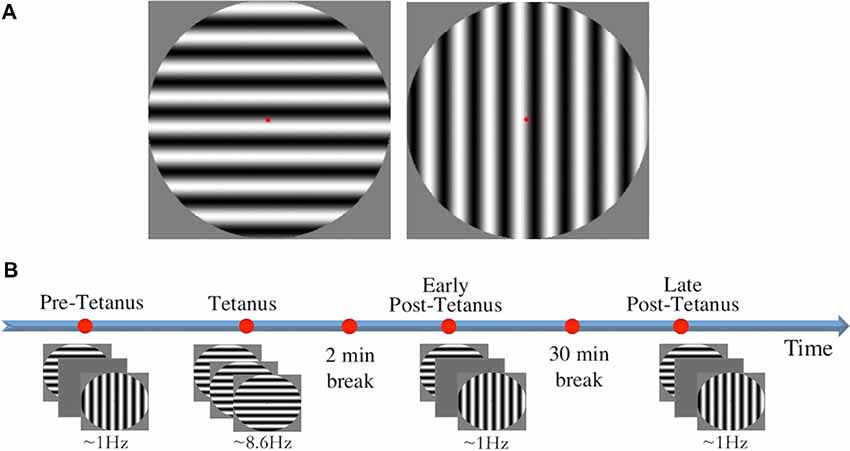
Figure 1. (A) The two circular sine gratings of vertical and horizontal orientation used for visual stimuli. (B) The experimental procedure consisted of four experimental blocks: three low-frequency blocks (pre-tetanus, early post-tetanus, late post-tetanus), and one high-frequency tetanus. Magnitude of long-term potentiation (LTP) was determined by subtracting pre-tetanus amplitude from each of the post-tetanus blocks. Rest periods were included after the tetanus (2 min), and between the two post-tetanus blocks (30 min).
LTP Procedure
LTP was assessed using our previously-established EEG paradigm (Teyler et al., 2005; McNair et al., 2006; Ross et al., 2008; Kirk et al., 2010; Smallwood et al., 2015; Figure 1B). Participants were first presented with a “pre-tetanus” baseline block, consisting of 240 presentations of each stimulus at a low temporal frequency of 0.67–1 Hz (33 ms presentation with a jittered inter-stimulus interval of 1,000–1,500 ms, ~8 min). Each participant was then presented with one of the stimulus orientations (counterbalanced) as an LTP-inducing stimulus, or “visual tetanus.” This consisted of 1,000 presentations, at a frequency of 8.6 Hz (jittered ISI of 67–100 ms, ~2 min). This was immediately followed by a 2 min rest period to allow retinal afterimages to dissipate. Participants were then presented with two more experimental blocks: an “early post-tetanus” block, and a “late post-tetanus” block. Both post-tetanus blocks had identical parameters to the pre-tetanus block (240 presentations of each stimulus at a temporal frequency of 0.67–1 Hz). The post-tetanus blocks were separated by a 30 min eyes-closed rest period.
EEG Analysis
EEG data were processed using in-house software that has been employed in a number of previous studies of visual LTP (Smallwood et al., 2015; Spriggs et al., 2017a). First, the data were re-referenced to the common average and band-pass filtered [0.1–30 Hz, bidirectional three-pole Butterworth filter (Alarcon et al., 2000)]. The data were subsequently segmented into 600 ms epochs (100 ms prestimulus to 500 ms poststimulus onset) and baseline corrected to the pre-stimulus period. Epochs containing significant artefacts (e.g., eye-blinks) were corrected using the automatic methods developed by Jervis et al. (1985), and the remaining data were averaged according to block (pre-tetanus, early post-tetanus, and late post-tetanus) and stimulus condition (tetanized, non-tetanized). The magnitude of LTP was defined as the amplitude difference between the pre-tetanus block and the two post-tetanus blocks independently (referred to as early LTP and late LTP, respectively) within the N1b time window. In accordance with previous literature (McNair et al., 2006), the N1b was defined as the section of the VEP extending from the peak of the N170 to the midpoint between the peak of the N170 and the peak of the P2. The pre- and post-tetanus N1b components of the VEP were averaged across posterior clusters of electrodes that were determined from the topography of the mean visually-evoked potential across all conditions and were centred approximately around P7 and P8 for each participant.
Statistical Analyses
We used Bayesian hypothesis testing for all analyses. Because we understand readers may wish to compare these with frequentist analyses, we provide all the frequentist equivalents in the Supplementary Material. All analyses were performed in R (R Core Team, 2016), with the following packages: BayesFactor (Morey et al., 2015), dplyr (Wickham, 2011), ggplot2 (Wickham, 2009), rjags (Plummer et al., 2016).
For all Bayesian analyses, Markov chain Monte Carlo methods were used to generate posterior samples via the Metropolis-Hastings algorithm. All analyses were set at 10,000 iterations, with diagnostic checks for convergence. One chain per analysis was used for all analyses reported in the article, with a thinning interval of 1 (i.e., no iteration was discarded). All priors used in the reported analyses are default prior scales (Morey et al., 2015).
Results
Bayesian Analyses
Figure 2 depicts the grand-average waveform and bilateral topography of the N170 peak. A linear regression analysis with a default prior (r scale of 0.354) showed that late LTP was a reliable predictor of Memory performance (P(M|data) = 0.77, BFM = 3.27). The correlation between late LTP and Memory performance was robust (r = 0.44, BF10 = 3.32). The association between the two variables, with the breakdown into genotype groups, is shown in Figure 3A. In contrast, the data showed no evidence for early LTP being a reliable predictor of Memory performance (P(M|data) = 0.26, BFM = 0.35), substantiated by a null correlation (r = 0.02, BF10 = 0.24).
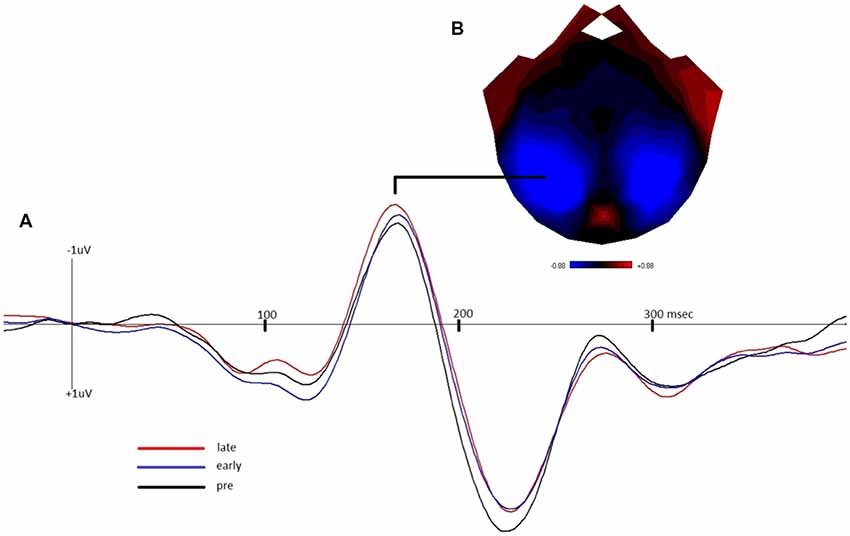
Figure 2. (A) Grand-average waveform demonstrating the negative shift of the N1b component of the visually-evoked potential in the early and late post-tetanus blocks, compared to pre-tetanus (negative plotted up). (B) Bilateral topography of the peak of the N170.
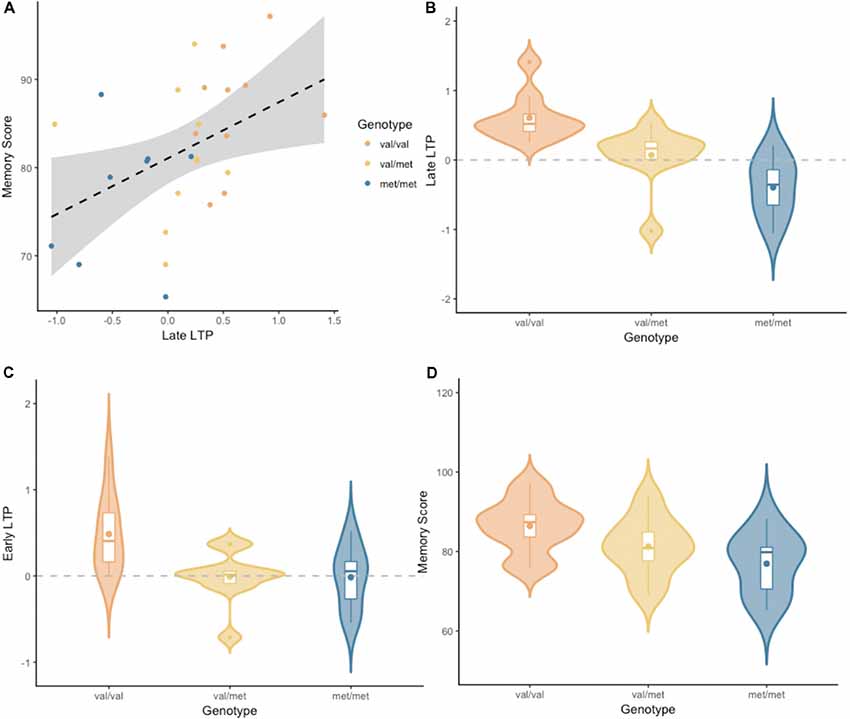
Figure 3. (A) Late LTP amplitude plotted against Wechsler Memory Scale-III (WMS-III) visual memory index (Memory Score), broken down by genotype. The dashed line is the regression line from the linear model, for all genotype groups combined. The shaded area around the dashed line represents the 95% confidence interval for predictions from the linear model fitted. (B) Late LTP amplitude as a function of brain-derived neurotrophic factor (BDNF) genotype. (C) Early LTP amplitude as a function of BDNF genotype. (D) Memory performance as a function of BDNF genotype. Subplots (B–D) depict the distribution of gain scores (violin) together with the mean (box central dot), median (box central line), first and third quartile (box edges), minimum and maximum (whiskers), and outliers (outside dots).
An analyses of variance (ANOVA) with a default prior (r scale of 0.5) on late LTP with BDNF genotype as a fixed factor, showed overwhelming evidence for the alternative model over the null model (P(M|data) > 0.99, BFM = 338.1, see Figure 3B). Pairwise comparisons with default priors (r scale of 0.701) showed support, although of different magnitudes, for all possible comparisons. There was overwhelming evidence for the difference between Val/Val and Met/Met (BF10 = 407.56), strong evidence for the difference between Val/Met and Val/Val (BF10 = 8.06), and very moderate evidence for the difference between Val/Met and Met/Met (BF10 = 2.26).
An ANOVA with a default prior (r scale of 0.5) on early LTP with BDNF genotype as a fixed factor showed evidence for the alternative model over the null model (P(M|data) = 0.86, BFM = 6.13, see Figure 3C). Pairwise comparisons with default prior (r scale of 0.701) showed that the effect differed based on the specific comparison. There was moderate evidence for the difference between Val/Val and Met/Met (BF10 = 3.18), somewhat stronger evidence for the difference between Val/Met and Val/Val (BF10 = 5.46), and no evidence for the difference between Val/Met and Met/Met (BF10 = 0.41).
An ANOVA with a default prior (r scale of 0.5) on Memory performance with BDNF genotype as a fixed factor, showed moderate evidence for the alternative model over the null model (P(M|data) = 0.69, BFM = 2.19, see Figure 3D). Pairwise comparisons with default prior (r scale of 0.701) showed support only for the difference between Val/Val and Met/Met (BF10 = 4.31). There was no evidence for a difference between Val/Met and Val/Val, or between Val/Met and Met/Met (BF10 = 0.99 and BF10 = 0.67, respectively).
Discussion
The current study provides the first evidence that the degree of visually-induced LTP is a significant predictor of human visual memory performance. While LTP is at the core of our understanding of long-term memory formation, a direct relationship between LTP magnitude and memory task performance has been notoriously difficult to demonstrate. However, the visual LTP paradigm presents the unique opportunity to study LTP in humans non-invasively, thus allowing for a direct assessment of this important relationship.
The current results demonstrate that LTP magnitude in the late post-tetanus block is a reliable predictor of long-term memory performance. This late post-tetanus block is run approximately 40 min after the LTP-inducing visual tetanus, and thus indexes enduring changes in neuronal activation. Conversely, there was no relationship between memory performance and early LTP, which indexes immediate post-tetanus modulations in neuronal response. Although these results may appear somewhat contradictory, this suggests that the two post-tetanus blocks may index different phases of LTP: LTP induction and LTP maintenance. LTP induction and maintenance are dependent on different cellular processes (Abraham and Williams, 2003; Cooke and Bliss, 2006), and therefore it is unsurprising that they have distinct relationships to long-term memory performance. Importantly, the current results demonstrate that it is the late post-tetanus block, and thus LTP maintenance, which is key to memory performance, with greater LTP magnitude predicting better memory performance.
The two subtests of the WMS-III assessed here are widely used to evaluate delayed visual recognition memory across diverse clinical populations (Conklin et al., 2002; Keilp et al., 2006; Seelye et al., 2009; Vann et al., 2009). Although delayed recognition memory is traditionally thought of as dependent on a network within the medial temporal lobe and frontal cortex (Aggleton and Brown, 2006), the current results indicate that LTP measured over the visual cortex is predictive of performance on this task. One explanation is that this represents a general propensity for an individual to exhibit LTP, perhaps across the brain as a whole. However, previous studies of the BDNF polymorphism indicate that there is divergence of the effect of the polymorphism between the motor cortex (Kleim et al., 2006; Antal et al., 2010; Cirillo et al., 2012; Teo et al., 2014) and auditory cortex (Teo et al., 2014). This suggests that the propensity for plasticity is not homogeneous across the brain, and therefore, sensory LTP is not a global index.
An alternative, and perhaps more likely, explanation for the correlation between visual cortical plasticity and memory performance is the integral involvement of visual system circuitry in networks specifically sub-serving visual memory formation. Experience-dependent plasticity within the visual network is understood to be fundamental in the mnemonic processing of visual information (Fahle, 2004; Kourtzi and DiCarlo, 2006; Ji and Wilson, 2007; Tsanov and Manahan-Vaughan, 2008), and plastic processes in the visual system influence subsequent processing in hippocampus (Tsanov and Manahan-Vaughan, 2008). In support of this, Spriggs et al. (2018) recently demonstrated that LTP induction using the visual paradigm modulates connections between the occipital and temporal cortices. It therefore appears that visually-induced LTP may represent an early, yet integral stage in visual memory processing, and that the magnitude of LTP can act as a “window” into the efficacy of the visual memory network.
There was a robust effect of the BDNF Val66Met polymorphism on memory performance. Individuals homozygous for the BDNF Met allele demonstrated poorer performance on tests of visual memory relative to those homozygous for the BDNF Val allele. This is consistent with the pattern of previous work that has demonstrated memory decrements in BDNF Met carriers across behavioral (Beste et al., 2011) and electrophysiological measures (Di Lorenzo et al., 2012). Importantly, this was also consistent with the current results of the impact of the BDNF polymorphism on visual LTP. LTP magnitude decreased with the increasing number of Met alleles an individual carried. This effect was seen in the comparisons between all three groups in the late post-tetanus block, and between Val homozygotes and Met carriers in the early post-tetanus block. Spriggs et al. (2017b)1 also found that the Val66Met polymorphism impacted the magnitude of visual LTP, with genotype differences in the P2 component of the VEP (greater shift in Met carriers)2. It will therefore be important for further studies to characterise the complex relationship between the BDNF Val66Met polymorphism and visual LTP.
Nevertheless, these data provide compelling support for the hypothesis that differences in memory task performance between BDNF genotypes are, at least to a considerable extent, due to differences in acute or rapid LTP-like changes in synaptic transmission in mnemonic networks (Egan et al., 2003; Hariri et al., 2003; Spriggs et al., 2018). While there may be chronic developmental differences in brain structure resulting from the BDNF polymorphism (Pezawas et al., 2004), here we demonstrate a genetic difference in experience-dependent plasticity that is over and above any developmental differences. It is interesting to note that, in light of relatively high memory scores, the Val/Met group showed little LTP and the Met/Met homozygotes showed on average the inverse of LTP [long-term depression (LTD)]. We have previously noted that the baseline stimulation used in the current paradigm can induce LTD in specific groups (Teyler et al., 2005; Ross et al., 2008; Spriggs et al., 2017a), which may be due to modulatory or metaplastic processes [for review, see (Abraham, 2008)]. It will therefore be important for future studies to examine the influence of genotype on LTP/LTD thresholds.
It should be noted here that the sample size in this study is small relative to the sample sizes considered “adequate” to yield sufficient power in most behavioural genetics studies. However, as highlighted by Rasch et al. (2010), sample sizes as low as 20 participants have previously been sufficient to identify genetic effects in imaging studies due to the increased proximity, and thus sensitivity, of neuronal phenotypes to the effect of the polymorphism (Hariri et al., 2003; Mattay et al., 2008; Rasch et al., 2010; Thompson et al., 2010). The current results support this hypothesis. Specifically, while there was moderate evidence for an effect of the BDNF Val66Met polymorphism on memory performance, the evidence was overwhelming for the effect of the polymorphism on LTP magnitude. Additionally, there was a dosage effect of genotype on LTP that was only trending for memory scores. The current results do indicate that the effect size of genetic variations on brain activity is much larger than on behavioural measures, rendering a small sample size less pertinent. It is nevertheless important to stress that replication with larger sample sizes will be a critical step in corroborating the current findings. It should also be noted here that, while we did not explicitly test for any effect of age on the current results, all participants were between 21 and 35 years of age. This is consistent with the age bracket for a young control group used in a previous study that did specifically assess age (Spriggs et al., 2017a). Additionally, the cohort consisted of an almost equal split between males (N = 13) and females (N = 15). Using a similar visual LTP paradigm, Sumner et al. (2018) recently found no reliable differences in the magnitude of induced visual LTP in the follicular and mid-luteal phases of the female mensural cycle in a cohort of young, healthy females. There is nevertheless evidence for an interaction between BDNF and sex in human motor control tasks (Smolders et al., 2012), and it may be important for future studies to assess this in the memory domain with a larger cohort.
Aberrant plasticity is implicated in a number of psychological and neurological conditions, including schizophrenia (Friston and Frith, 1995) and Alzheimer’s disease (Klein, 2006). Previous studies using the sensory LTP paradigm have demonstrated reduced LTP magnitude in both major depression (Normann et al., 2007) and schizophrenia (Çavuş et al., 2012). As previously mentioned, the memory tasks employed in the current study have also been used widely in clinical assessments of memory function (Conklin et al., 2002; Keilp et al., 2006; Seelye et al., 2009; Vann et al., 2009). We have demonstrated for the first time that the combination of these neurophysiological and behavioural measures provides a level of insight beyond the use of these measures in isolation. With a focus on the BDNF Val66Met polymorphism, the current study demonstrates how this can be used to explore the neural-basis for group differences in memory performance. It is hoped that this will be of utility to future studies assessing memory decline in neuropsychological and neurodegenerative disorders.
Here, we provide the first evidence for the relationship between visually-induced LTP and visual memory performance. This not only bridges the gap between LTP and memory performance, but provides further evidence for the visual-LTP paradigm as an effective index of memory related neuroplasticity in the human neocortex. Additionally, the current study demonstrates that both memory performance and LTP magnitude are influenced by the BDNF Val66Met polymorphism, thus supporting the role of the polymorphism exerting its influence over memory performance through the modulation of experience-dependent plasticity. Finally, the current study demonstrates the unique insight offered through the combination of these neurophysiological and behavioural measures of memory function. It is anticipated that this will have clinical applications in studying the variety of cognitive and affective disorders.
Author Contributions
MS: writing and manuscript preparation. CT: data collection and analysis. DM: data analysis and writing. NMcN: EEG data collection and design input. CW and NMcK: EEG data collection. YL: genetic data collection. RK: editing. UA, AS and BR: genetic analysis. JH: design input and analysis. TT: design input. KW: data analysis. IK: overall experimental design, data analysis and writing.
Funding
This work was supported by a grant from the NZ Royal Society (Marsden Grant #06-UoA-077; IK, KW). MS is supported by a Brain Research New Zealand Doctoral Scholarship.
Conflict of Interest Statement
The authors declare that the research was conducted in the absence of any commercial or financial relationships that could be construed as a potential conflict of interest.
Acknowledgments
We would like to thank the Auckland Sequenom Facility for genetic analysis. Subsets of these data have been presented at conferences, and have thus been described previously in published abstracts (e.g., Thompson et al., 2010, 2011, 2013).
Footnotes
- ^ This is the same study as Spriggs et al. (2018), with an added comparison between BDNF genotype groups (only BiorXiv preprint available).
- ^ While the results of these two studies may seem contradictory, there are significant differences in the approaches taken between them. First, Spriggs et al. (2017b) grouped Met homozygotes and heterozygotes together into a “Met Carrier” group, thus overshadowing the dosage effect seen in the late post-tetanus block of the current study. Additionally, the current study employed previously established analysis methods and specifically focused on the N1b. Spriggs et al. (2018) analysed the entire time window from 0 to 250 ms as this was better suited for the use of Dynamic Causal Modelling in this study, but also may have washed out the genotype effect on the N1b.
Supplementary Material
The Supplementary Material for this article can be found online at: https://www.frontiersin.org/articles/10.3389/fnhum.2019.00022/full#supplementary-material
References
Abraham, W. C. (2008). Metaplasticity: tuning synapses and networks for plasticity. Nat. Rev. Neurosci. 9, 387–399. doi: 10.1038/nrn2356
Abraham, W. C., and Williams, J. M. (2003). Properties and mechanisms of LTP maintenance. Neuroscientist 9, 463–474. doi: 10.1177/1073858403259119
Aggleton, J. P., and Brown, M. W. (2006). Interleaving brain systems for episodic and recognition memory. Trends Cogn. Sci. 10, 455–463. doi: 10.1016/j.tics.2006.08.003
Alarcon, G., Guy, C. N., and Binnie, C. D. (2000). A simple algorithm for a digital three-pole Butterworth filter of arbitrary cut-off frequency: application to digital electroencephalography. J. Neurosci. Methods 104, 35–44. doi: 10.1016/s0165-0270(00)00324-1
Antal, A., Chaieb, L., Moliadze, V., Monte-Silva, K., Poreisz, C., Thirugnanasambandam, N., et al. (2010). Brain-derived neurotrophic factor (BDNF) gene polymorphisms shape cortical plasticity in humans. Brain Stimul. 3, 230–237. doi: 10.1016/j.brs.2009.12.003
Beck, H., Goussakov, I. V., Lie, A., Helmstaedter, C., and Elger, C. E. (2000). Synaptic plasticity in the human dentate gyrus. J. Neurosci. 20, 7080–7086. doi: 10.1523/JNEUROSCI.20-18-07080.2000
Beste, C., Schneider, D., Epplen, J. T., and Arning, L. (2011). The functional BDNF Val66Met polymorphism affects functions of pre-attentive visual sensory memory processes. Neuropharmacology 60, 467–471. doi: 10.1016/j.neuropharm.2010.10.028
Bliss, T. V. P., and Lømo, T. (1973). Long-lasting potentiation of synaptic transmission in the dentate area of the anaesthetized rabbit following stimulation of the perforant path. J. Physiol. 232, 331–356. doi: 10.1113/jphysiol.1973.sp010273
Çavuş, I., Reinhart, R. M., Roach, B. J., Gueorguieva, R., Teyler, T. J., Clapp, W. C., et al. (2012). Impaired visual cortical plasticity in schizophrenia. Biol. Psychiatry 71, 512–520. doi: 10.1016/j.biopsych.2012.01.013
Chen, W. R., Lee, S., Kato, K., Spencer, D. D., Shepherd, G. M., and Williamson, A. (1996). Long-term modifications of synaptic efficacy in the human inferior and middle temporal cortex. Proc. Natl. Acad. Sci. U S A 93, 8011–8015. doi: 10.1073/pnas.93.15.8011
Cirillo, J., Hughes, J., Ridding, M., Thomas, P. Q., and Semmler, J. G. (2012). Differential modulation of motor cortex excitability in BDNF Met allele carriers following experimentally induced and use-dependent plasticity. Eur. J. Neurosci. 36, 2640–2649. doi: 10.1111/j.1460-9568.2012.08177.x
Clapp, W. C., Eckert, M. J., Teyler, T. J., and Abraham, W. C. (2006). Rapid visual stimulation induces N-methyl-D-aspartate receptor-dependent sensory long-term potentiation in the rat cortex. Neuroreport 17, 511–515. doi: 10.1097/01.wnr.0000209004.63352.10
Clapp, W. C., Hamm, J. P., Kirk, I. J., and Teyler, T. J. (2012). Translating long-term potentiation from animals to humans: a novel method for noninvasive assessment of cortical plasticity. Biol. Psychiatry 71, 496–502. doi: 10.1016/j.biopsych.2011.08.021
Conklin, H. M., Calkins, M. E., Anderson, C. W. III., Dinzeo, T. J., and Iacono, W. G. (2002). Recognition memory for faces in schizophrenia patients and their first-degree relatives. Neuropsychologia 40, 2314–2324. doi: 10.1016/s0028-3932(02)00091-x
Cooke, S. F., and Bliss, T. V. P. (2006). Plasticity in the human central nervous system. Brain 129, 1659–1673. doi: 10.1093/brain/awl082
de Gobbi Porto, F. H., Fox, A. M., Tusch, E. S., Sorond, F., Mohammed, A. H., and Daffner, K. R. (2015). In vivo evidence for neuroplasticity in older adults. Brain Res. Bull. 114, 56–61. doi: 10.1016/j.brainresbull.2015.03.004
Di Lorenzo, C., Di Lorenzo, G., Daverio, A., Pasqualetti, P., Coppola, G., Giannoudas, I., et al. (2012). The Val66Met polymorphism of the BDNF gene influences trigeminal pain-related evoked responses. J. Pain 13, 866–873. doi: 10.1016/j.jpain.2012.05.014
Egan, M. F., Kojima, M., Callicott, J. H., Goldberg, T. E., Kolachana, B. S., Bertolino, A., et al. (2003). The BDNF Val66Met polymorphism affects activity-dependent secretion of BDNF and human memory and hippocampal function. Cell 112, 257–269. doi: 10.1016/s0092-8674(03)00035-7
Fahle, M. (2004). Perceptual learning: a case for early selection. J. Vis. 4, 879–890. doi: 10.1167/4.10.4
Figurov, A., Pozzo-Miller, L. D., Olafsson, P., Wang, T., and Lu, B. (1996). Regulation of synaptic responses to high-frequency stimulation and LTP by neurotrophins in the hippocampus. Nature 381, 706–709. doi: 10.1038/381706a0
Friston, K. J., and Frith, C. D. (1995). Schizophrenia: a disconnection syndrome. Clin. Neurosci. 3, 89–97.
Goldberg, T. E., and Weinberger, D. R. (2004). Genes and the parsing of cognitive processes. Trends Cogn. Sci. 8, 325–335. doi: 10.1016/j.tics.2004.05.011
Hariri, A. R., Goldberg, T. E., Mattay, V. S., Kolachana, B. S., Callicott, J. H., Egan, M. F., et al. (2003). Brain-derived neurotrophic factor Val66Met polymorphism affects human memory-related hippocampal activity and predicts memory performance. J. Neurosci. 23, 6690–6694. doi: 10.1523/JNEUROSCI.23-17-06690.2003
Harris, E. W., Ganong, A. H., and Cotman, C. W. (1984). Long-term potentiation in the hippocampus involves activation of N-methyl-D-aspartate receptors. Brain Res. 323, 132–137. doi: 10.1016/0006-8993(84)90275-0
Jervis, B. W., Nichols, M. J., Allen, E. M., Hudson, N. R., and Johnson, T. E. (1985). The assessment of two methods for removing eye movement artefact from the EEG. Electroencephalogr. Clin. Neurophysiol. 61, 444–452. doi: 10.1016/0013-4694(85)91037-5
Ji, D., and Wilson, M. A. (2007). Coordinated memory replay in the visual cortex and hippocampus during sleep. Nat. Neurosci. 10, 100–107. doi: 10.1038/nn1825
Kambeitz, J. P., Bhattacharyya, S., Kambeitz-Ilankovic, L. M., Valli, I., Collier, D. A., and McGuire, P. (2012). Effect of BDNF Val66Met polymorphism on declarative memory and its neural substrate: a meta-analysis. Neurosci. Biobehav. Rev. 36, 2165–2177. doi: 10.1016/j.neubiorev.2012.07.002
Keilp, J. G., Corbera, K., Slavov, I., Taylor, M. J., Sackeim, H. A., and Fallon, B. A. (2006). WAIS-III and WMS-III performance in chronic Lyme disease. J. Int. Neuropsychol. Soc. 12, 119–129. doi: 10.1017/S1355617706060231
Kirk, I. J., McNair, N. A., Hamm, J. P., Clapp, W. C., Mathalon, D. H., Cavus, I., et al. (2010). Long-term potentiation (LTP) of human sensory-evoked potentials. Wiley Interdiscip. Rev. Cogn. Sci. 1, 766–773. doi: 10.1002/wcs.62
Kirkwood, A., and Bear, M. F. (1994). Hebbian synapses in visual cortex. J. Neurosci. 14, 1634–1645. doi: 10.1523/JNEUROSCI.14-03-01634.1994
Kleim, J. A., Chan, S., Pringle, E., Schallert, K., Procaccio, V., Jimenez, R., et al. (2006). BDNF Val66Met polymorphism is associated with modified experience-dependent plasticity in human motor cortex. Nat. Neurosci. 9, 735–737. doi: 10.1038/nn1699
Klein, W. L. (2006). Synaptic targeting by Aβ oligomers (ADDLS) as a basis for memory loss in early Alzheimer’s disease. Alzheimers Dement. 2, 43–55. doi: 10.1016/j.jalz.2005.11.003
Kourtzi, Z., and DiCarlo, J. J. (2006). Learning and neural plasticity in visual object recognition. Curr. Opin. Neurobiol. 16, 152–158. doi: 10.1016/j.conb.2006.03.012
Lynch, M. A. (2004). Long-term potentiation and memory. Physiol. Rev. 84, 87–136. doi: 10.1152/physrev.00014.2003
Martin, S. J., Grimwood, P. D., and Morris, R. G. (2000). Synaptic plasticity and memory: an evaluation of the hypothesis. Annu. Rev. Neurosci. 23, 649–711. doi: 10.1146/annurev.neuro.23.1.649
Mattay, V. S., Goldberg, T. E., Sambataro, F., and Weinberger, D. R. (2008). Neurobiology of cognitive aging: insights from imaging genetics. Biol. Psychol. 79, 9–22. doi: 10.1016/j.biopsycho.2008.03.015
McNair, N. A., Clapp, W. C., Hamm, J. P., Teyler, T. J., Corballis, M. C., and Kirk, I. J. (2006). Spatial frequency-specific potentiation of human visual-evoked potentials. Neuroreport 17, 739–741. doi: 10.1097/01.wnr.0000215775.53732.9f
Miller, S. A., Dykes, D. D., and Polesky, H. (1988). A simple salting out procedure for extracting DNA from human nucleated cells. Nucleic Acids Res. 16:1215. doi: 10.1093/nar/16.3.1215
Morey, R. D., Rouder, J. N., and Jamil, T. (2015). BayesFactor: computation of Bayes factors for common designs. R Package Version 0.9 Available online at: http://CRAN.R-project.org/package=BayesFactor
Normann, C., Schmitz, D., Fürmaier, A., Döing, C., and Bach, M. (2007). Long-term plasticity of visually evoked potentials in humans is altered in major depression. Biol. Psychiatry 62, 373–380. doi: 10.1016/j.biopsych.2006.10.006
Park, H., and Poo, M. (2013). Neurotrophin regulation of neural circuit development and function. Nat. Rev. Neurosci. 14, 7–23. doi: 10.1038/nrn3379
Pezawas, L., Verchinski, B. A., Mattay, V. S., Callicott, J. H., Kolachana, B. S., Straub, R. E., et al. (2004). The brain-derived neurotrophic factor Val66Met polymorphism and variation in human cortical morphology. J. Neurosci. 24, 10099–10102. doi: 10.1523/JNEUROSCI.2680-04.2004
Plummer, M., Stukalov, A., and Denwood, M. (2016). rjags: bayesian graphical models using MCMC (Version 4-6). Available online at: https://cran.r-project.org/web/packages/rjags/index.html
Rasch, B., Papassotiropoulos, A., and de Quervain, D.-F. (2010). Imaging genetics of cognitive functions: focus on episodic memory. Neuroimage 53, 870–877. doi: 10.1016/j.neuroimage.2010.01.001
R Core Team. (2016). R: A Language and Environment for Statistical Computing. R Foundation for Statistical Computing. Vienna, Austria. Available online at: http://www.R-project.org
Ross, R. M., McNair, N. A., Fairhall, S. L., Clapp, W. C., Hamm, J. P., Teyler, T. J., et al. (2008). Induction of orientation-specific LTP-like changes in human visual evoked potentials by rapid sensory stimulation. Brain Res. Bull. 76, 97–101. doi: 10.1016/j.brainresbull.2008.01.021
Seelye, A. M., Howieson, D. B., Wild, K. V., Moore, M. M., and Kaye, J. A. (2009). Wechsler Memory Scale-III Faces test performance in patients with mild cognitive impairment and mild Alzheimer’s disease. J. Clin. Exp. Neuropsychol. 31, 682–688. doi: 10.1080/13803390802484763
Shimizu, E., Hashimoto, K., and Iyo, M. (2004). Ethnic difference of the BDNF 196G/A (Val66Met) polymorphism frequencies: the possibility to explain ethnic mental traits. Am. J. Med. Genet. B Neuropsychiatr. Genet. 126, 122–123. doi: 10.1002/ajmg.b.20118
Smallwood, N., Spriggs, M. J., Thompson, C. S., Wu, C. C., Hamm, J. P., Moreau, D., et al. (2015). Influence of physical activity on human sensory long-term potentiation. J. Int. Neuropsychol. Soc. 21, 831–840. doi: 10.1017/s1355617715001095
Smolders, R., Rijpkema, M., Franke, B., and Fernandez, G. (2012). BDNF Val66Met polymorphism interacts with sex to influence bimanual motor control in healthy humans. Brain Behav. 2, 726–731. doi: 10.1002/brb3.83
Spriggs, M. J., Cadwallader, C. J., Hamm, J. P., Tippett, L. J., and Kirk, I. J. (2017a). Age-related alterations in human neocortical plasticity. Brain Res. Bull. 130, 53–59. doi: 10.1016/j.brainresbull.2016.12.015
Spriggs, M. J., Sumner, R. L., McMillan, R. L., Moran, R. J., Kirk, I. J., and Muthukumaraswamy, S. D. (2017b). Indexing sensory plasticity: evidence for distinct Predictive Coding and Hebbian Learning mechanisms in the cerebral cortex. BioRxiv 189944. doi: 10.1101/189944
Spriggs, M. J., Sumner, R. L., McMillan, R. L., Moran, R. J., Kirk, I. J., and Muthukumaraswamy, S. D. (2018). Indexing sensory plasticity: evidence for distinct Predictive Coding and Hebbian Learning mechanisms in the cerebral cortex. Neuroimage 176, 290–300. doi: 10.1016/j.neuroimage.2018.04.060
Sumner, R. L., Spriggs, M. J., McMillan, R. L., Sundram, F., Kirk, I. J., and Muthukumaraswamy, S. D. (2018). Neural plasticity is modified over the human menstrual cycle: combined insight from sensory evoked potential LTP and repetition suppression. Neurobiol. Learn. Mem. 30, 422–434. doi: 10.1016/j.nlm.2018.08.016
Teo, J. T., Bentley, G., Lawrence, P., Soltesz, F., Miller, S., Willé, D., et al. (2014). Late cortical plasticity in motor and auditory cortex: role of met-allele in BDNF Val66Met polymorphism. Int. J. Neuropsychopharmacol. 17, 705–713. doi: 10.1017/s1461145713001636
Teyler, T. J., and DiScenna, P. (1987). Long-term potentiation. Annu. Rev. Neurosci. 10, 131–161. doi: 10.1146/annurev.ne.10.030187.001023
Teyler, T. J., Hamm, J. P., Clapp, W. C., Johnson, B. W., Corballis, M. C., and Kirk, I. J. (2005). Long-term potentiation of human visual evoked responses. Eur. J. Neurosci. 21, 2045–2050. doi: 10.1111/j.1460-9568.2005.04007.x
Thompson, P. M., Martin, N. G., and Wright, M. J. (2010). Imaging genomics. Curr. Opin. Neurol. 23, 368–373. doi: 10.1097/WCO.0b013e32833b764c
Thompson, C. S., McNair, N. A., Antia, U., Kennedy, K., Shelling, A. N., Russell, B. R., et al. (2013). Brain-derived neurotrophic factor val66met polymorphism influences the magnitude of human long-term potentiation which predicts memory performance. J. Cog. Neurosci. 113:2013.
Thompson, C. S., McNair, N. A., Kennedy, K., Antia, U., Wannenburg, S., Shelling, A. et al. (2011). Brain-derived neurotrophic factor Val66Met polymorphism influences the magnitude of human long-term potentiation which predicts memory performance. J. Int. Neuropsychol. Soc. 17:S2. doi: 10.1017/S1355617711001184
Tsanov, M., and Manahan-Vaughan, D. (2008). Synaptic plasticity from visual cortex to hippocampus: systems integration in spatial information processing. Neuroscientist 14, 584–597. doi: 10.1177/1073858408315655
Tyler, W. J., Alonso, M., Bramham, C. R., and Pozzo-Miller, L. D. (2002). From acquisition to consolidation: on the role of brain-derived neurotrophic factor signaling in hippocampal-dependent learning. Learn. Mem. 9, 224–237. doi: 10.1101/lm.51202
Vann, S. D., Tsivilis, D., Denby, C. E., Quamme, J. R., Yonelinas, A. P., Aggleton, J. P., et al. (2009). Impaired recollection but spared familiarity in patients with extended hippocampal system damage revealed by 3 convergent methods. Proc. Natl. Acad. Sci. U S A 106, 5442–5447. doi: 10.1073/pnas.0812097106
Wechsler, D., Padovani, F., Orsini, A., and Laicardi, C. (2007). WAIS-R: Wechsler Adult Intelligence Scale-Revised: Manuale. New York, NY: Psychological Corporation.
Wickham, H. (2009). ggplot2: Elegant Graphics for Data Analysis Springer-Verlag. New York, NY: Springer.
Keywords: ERP, neuroplasticity, neurogenetics, visual memory, single-nucleotide polymorphism, long-term potentiation, VEP, brain-derived neurotrophic factor
Citation: Spriggs MJ, Thompson CS, Moreau D, McNair NA, Wu CC, Lamb YN, McKay NS, King ROC, Antia U, Shelling AN, Hamm JP, Teyler TJ, Russell BR, Waldie KE and Kirk IJ (2019) Human Sensory LTP Predicts Memory Performance and Is Modulated by the BDNF Val66Met Polymorphism. Front. Hum. Neurosci. 13:22. doi: 10.3389/fnhum.2019.00022
Received: 11 July 2018; Accepted: 18 January 2019;
Published: 15 February 2019.
Edited by:
Matthias Kliegel, Université de Genève, SwitzerlandReviewed by:
Giorgio Di Lorenzo, University of Rome Tor Vergata, ItalyMario Tombini, Campus Bio-Medico University, Italy
Copyright © 2019 Spriggs, Thompson, Moreau, McNair, Wu, Lamb, McKay, King, Antia, Shelling, Hamm, Teyler, Russell, Waldie and Kirk. This is an open-access article distributed under the terms of the Creative Commons Attribution License (CC BY). The use, distribution or reproduction in other forums is permitted, provided the original author(s) and the copyright owner(s) are credited and that the original publication in this journal is cited, in accordance with accepted academic practice. No use, distribution or reproduction is permitted which does not comply with these terms.
*Correspondence: Meg J. Spriggs, bS5zcHJpZ2dzQGltcGVyaWFsLmFjLnVr
† These authors have contributed equally to this work