- 1Sackler School of Medicine, Tel-Aviv University, Tel-Aviv, Israel
- 2Radiology Department, Assaf Harofeh Medical Center, Zerifin, Israel
- 3Sagol Center for Hyperbaric Medicine and Research, Assaf Harofeh Medical Center, Zerifin, Israel
- 4Faculty of Medicine, Bar-Ilan University, Ramat Gan, Israel
- 5WiseImage, Hod Hasharon, Israel
- 6Research and Development Unit, Assaf Harofeh Medical Center, Zerifin, Israel
- 7Sagol School of Neuroscience, Tel-Aviv University, Tel-Aviv, Israel
Background: Recent clinical studies in stroke and traumatic brain injury (TBI) victims suffering chronic neurological injury present evidence that hyperbaric oxygen therapy (HBOT) can induce neuroplasticity.
Objective: To assess the neurotherapeutic effect of HBOT on prolonged post-concussion syndrome (PPCS) due to TBI, using brain microstructure imaging.
Methods: Fifteen patients afflicted with PPCS were treated with 60 daily HBOT sessions. Imaging evaluation was performed using Dynamic Susceptibility Contrast-Enhanced (DSC) and Diffusion Tensor Imaging (DTI) MR sequences. Cognitive evaluation was performed by an objective computerized battery (NeuroTrax).
Results: HBOT was initiated 6 months to 27 years (10.3 ± 3.2 years) from injury. After HBOT, DTI analysis showed significantly increased fractional anisotropy values and decreased mean diffusivity in both white and gray matter structures. In addition, the cerebral blood flow and volume were increased significantly. Clinically, HBOT induced significant improvement in the memory, executive functions, information processing speed and global cognitive scores.
Conclusions: The mechanisms by which HBOT induces brain neuroplasticity can be demonstrated by highly sensitive MRI techniques of DSC and DTI. HBOT can induce cerebral angiogenesis and improve both white and gray microstructures indicating regeneration of nerve fibers. The micro structural changes correlate with the neurocognitive improvements.
Introduction
Traumatic brain injury (TBI) is a significant public health concern in military and civilian populations (Chiu and LaPorte, 1993). The estimated number of TBI cases occurring each year is 10 million globally and 1.7–3.8 million in the United States alone. 75–90% of those are defined as mild TBI (mTBI) (Selassie et al., 2013; Marin et al., 2014).
The post-concussion syndrome (PCS) is a complex of symptoms consisting of headaches, dizziness, imbalance, vertigo, fatigue, changes in sleep pattern, neuropsychiatric symptoms (e.g., behavioral and mood changes, confusion), and cognitive impairments (in memory, attention, concentration and executive functions) (McCauley et al., 2005). PCS is most often described in the setting of mTBI, but may also occur after moderate and severe TBI. In Eight to Ninty percent of mTBI cases, the symptoms fade away in 7–10 days (McCrory et al., 2005; Hadanny and Efrati, 2016). Still, in 10–20 percent, PCS may persist for weeks or months due to structural and/or metabolic brain damage. Twenty-five to thirty-three percent of those retain a permanent brain injury and experience persistent PCS; the symptoms turn chronic and endure more than 6 months (Kashluba et al., 2004; Bazarian et al., 2005; Iverson, 2005; Sterr et al., 2006; King and Kirwilliam, 2011).
The sensitivity of classic anatomical brain imaging techniques, such as Computed Tomography (CT) and Magnetic Resonance Imaging (MRI), is generally not sufficient for detection of the pathophysiologic effects of mTBI. New techniques are increasingly utilized for objective evaluation of brain damage. Diffuse Tensor Imaging (DTI) can reveal the combination of axonal injury and secondary gliosis with local microvascular injury (Niogi and Mukherjee, 2010). Dynamic susceptibility contrast MR perfusion can demonstrate reduced cerebral blood flow (CBF), global and regional, as well as cerebral blood volume (CBV) (Tal et al., 2015).
The existing pharmacologic and non-pharmacologic treatments have mostly failed to elicit efficacious results in both the clinical symptoms and the pathophysiologic cascade leading to permanent brain injury (Hadanny and Efrati, 2016). In recent years, both basic (animal models) (Neubauer and James, 1998; Zhang et al., 2005; Vlodavsky et al., 2006; Chen et al., 2010; Huang and Obenaus, 2011; Lin et al., 2012; Efrati and Ben-Jacob, 2014) and clinical studies (Shi et al., 2003, 2006; Barrett et al., 2004; Golden et al., 2006; Wright et al., 2009; Harch et al., 2012; Boussi-Gross et al., 2013; Tal et al., 2015) have shown that hyperbaric oxygen therapy (HBOT) can improve PCS by targeting the basic pathological processes responsible for post-concussion symptoms (Hadanny and Efrati, 2016). In our previous study, it was evident that HBOT can induce brain angiogenesis, demonstrated by perfusion MRI with significant increase in CBF and CBV following HBOT along with significant cognitive improvement in patients post TBI (Tal et al., 2015).
The current study was aimed at evaluating the effects of HBOT on brain microstructure in chronic neurological deficiencies stemming from TBI. This has not been studied in humans so far.
Methods
A retrospective analysis of patients suffering from chronic neurocognitive impairment due to TBI, treated at Sagol Center for Hyperbaric Medicine and Research at Assaf Harofeh Medical Center, Israel, between September 2013 and December 2015. The study was approved by the Institutional Review Board of the hospital (0030-15-ASF) and registered in the US National Institute of Health Clinical Trials registry (NCT02452619).
Inclusion criteria: Patients with chronic neurocognitive impairment started only after TBI, persisting over 6 months who underwent two MRI brain imaging (including DTI and DSC sequences) and two neurocognitive tests, pre- and post- hyperbaric oxygen therapy (HBOT). All patients applied for HBOT of their own volition. Patients with other neurological conditions were excluded from the study's cohort.
The classification of TBI was based on the American Congress of Rehabilitation Medicine (ACRM) and the Centers of Disease Control (CDC), where mTBI is defined as altered brain function engendered by external forces with one or more of the following: loss of consciousness with duration of 0–30 min, post-traumatic amnesia with duration of less than 24 h, and Glasgow Coma Scale grade of 13–15 (Malec et al., 2007). GCS score of 3–8, or post-traumatic amnesia of more than 7 days, or loss of consciousness for more than 24 h is classified as severe TBI; GCS score of 9–12, or post-traumatic amnesia of 1–7 days, or loss of consciousness between 30 min and 24 h, is classified as moderate TBI (Malec et al., 2007).
Hyperbaric Oxygen Treatment
Patients were treated in a multiplace hyperbaric chamber (HAUX-Life-Support GmbH) for 60 daily hyperbaric sessions, 5 days a week. Each session consisted of 90 min of exposure to 100% oxygen at 2 ATA. Acceptable compression and decompression rates of 0.8 meter per minute were employed. Oxygen was delivered by tight masks.
MRI Scan Protocol
All patients had MRI scans 1–2 weeks before and after HBOT. Imaging was done with a 3 Tesla system (20 channels, MAGNETOM Skyra, Siemens Medical Solutions) with a multichannel head coil as a receiver coil. The MRI protocol included the following sequences: T2 weighted, T1 weighted, FLAIR, susceptibility weighted imaging (SWI), dynamic susceptibility contrast enhancement (DSC), and diffusion tensor imaging (DTI). The MRI, DTI, and DSC sequences' parameters are detailed in the supplementary material (SI-1). The injected gadolinium (0.5 mmol/ml) dosage was 0.2 ml/kg/patient.
MRI Analysis
MRI analysis was performed by WiseImage (Hod Hasharon, Israel, http://www.wise-image.com).
DSC Analysis
Images were corrected for motion using SPM software (version 12, UCL, London, UK). DSC analysis was performed as described in previous studies (Østergaard et al., 1996a,b part I and II) using in-house software written in Matlab R2011 (Mathworks, Natick, MA). Detailed description is found in the Supplementary Material SI-1. In short, MR signal intensity was converted to Gd concentration, fitted to the gamma variate function and deconvolved on a voxel-by-voxel basis to calculate the CBF, CBV, and MTT (Mean Transient Time) maps. Smoothing of 8 mm full width at half maxima (FWHM) was performed on the perfusion maps using the SPM software.
DTI Analysis
Motion and Echo planar imaging (EPI) correction and regularization of the DWI volumes as well as calculation of DTI maps (MD = mean diffusivity, FA = fractional anisotropy, AD = axial diffusivity, RD = radial diffusivity maps) were done using ExploreDTI software (Leemans et al., 2009). Two analysis types were performed: voxel-based analysis and fiber tracking. Detailed description is found in the Supplementary Material SI-1. In short, paired t-test was performed using voxel-based analysis, generating statistical parametric maps. Fiber tracking was applied using Explore DTI software in order to plot 8 fiber tracts for each patient: Uncinate fasciculus (UF), Cingulum, inferior longitudinal fasciculus (ILF), and Inferior fronto-occipital fasciculus (IFOF), in both hemispheres. After the tracking procedure, a mask was created from the tracts matrices of all subjects in order to create a tract mask. Overall, 8 masks were created for each subject: four fiber tracts (UF, fornix, cingulum, ILF), in both hemispheres. The tract masks of the different patients were registered to a tract mask of one patient.
Cognitive Assessment
The assessment of cognitive functions was done by NeuroTrax computerized cognitive tests (NeuroTrax Corp., TX) (Dwolatzky et al., 2003). The neurocognitive battery by Neurotrax is a validated computerized cognitive evaluation, which was specifically designed to TBI patients. These tests evaluate various aspects of brain function and incorporate Verbal Memory (immediate and delayed recognition), Non-Verbal Memory (immediate and delayed recognition), Go-No-Go Response Inhibition, Problem Solving, Stroop Interference, Finger Tapping, Catch Game, Staged Information Processing Speed (single digit, two-digit and three-digit arithmetic), Verbal Function, and Visual Spatial Processing. Cognitive index scores were computed from the normalized outcome parameters for the following domains: executive function, memory, attention, speed of information processing, visual spatial, verbal function and motor skills (Thaler et al., 2012; Achiron et al., 2013; Zur et al., 2015). The verbal domain score was excluded because only 8 patients (53%) had reliable calculated verbal domains. A global cognitive score was computed as the average of all index scores for each individual.
The NeuroTrax data were uploaded to the NeuroTrax central server, and outcome parameters were automatically calculated using a software blind to diagnosis or testing site. To account for the effects of age and education on cognitive performance, each outcome parameter was normalized and fit to an IQ-like scale (mean = 100, S.D. = 5) according to age and education. The normative data used by NeuroTrax consist of test data from cognitively healthy individuals in controlled research studies at more than 10 sites (Doniger, 2014).
Specifically, the patients received two different versions of the NeuroTrax test battery before and after HBOT so as to produce minimal learning effects upon repeated administration. Test-retest reliability for those versions was found to be high (Schweiger et al., 2003; Melton, 2005). Each cognitive domain score was calculated out of 3–5 different tests. It had 3 different forms of each test - out of which we used one for pre exam and another for the post test. This is a strong feature of these tests as it reduces the “learning effect” with a good test/retest validity. The fact that each index is referred to more than one test-score ensures that the index is associated with a cognitive domain score rather than with a test-dependent score.
Statistical Analysis
In addition to the MRI analysis described above, continuous data were expressed as means ± standard errors. The normal distribution for all variables was tested by means of the Kolmogorov-Smirnov test. The mean differences between cognitive index scores before and after HBOT were analyzed using two-tailed paired t-tests or a Wilcoxon signed-rank test. The alpha level was set to 0.05. Data were statistically analyzed using SPSS software (version 22.0).
Results
Patients
Fifteen patients with chronic cognitive impairment due to TBI who were treated at the Sagol Center for Hyperbaric Medicine and Research between September 2013 and December 2015 fulfilled the inclusion criteria.
The mean age was 35.8 ± 3.5 years (21–70), and 53% (8/15) were males. All patients had documented traumatic brain injury 6 months to 27 years (mean 6.7 ± 2.1 years) prior to HBOT. Seven patients (46.7%) suffered from moderate to severe TBI, and 8 (53.3%) suffered from PCS after mTBI. See patients' baseline characteristics in Table 1. Baseline standard MRI findings for each of the patients are summarized in SI-2.
Neurocognitive Function
HBOT induced a considerable improvement in the global cognitive score, with a mean change of 8.1 ± 1.5 and a relative change of 9.6 ± 1.9% (p = 0.0001). Memory, executive functions and information processing speed showed the most striking improvements (>15% relative change) with mean changes of 10.5 ± 2.4 (p = 0.001), 11.3 ± 2.7 (p = 0.0001) and 13.1 ± 2.7 (p = 0.0001), respectively. Attention increased by 16.1 ± 6.3% post HBOT but did not reach statistical significance (p = 0.06). There were no differences in neurocognitive scores (mean and relative changes) of patients taking SSRI/Opiates/Benzodiazpeines drugs compared to patients without them (p > 0.2).
The effect of HBOT on the patients' cognitive functions is summarized in Figure 1 and Table 2.
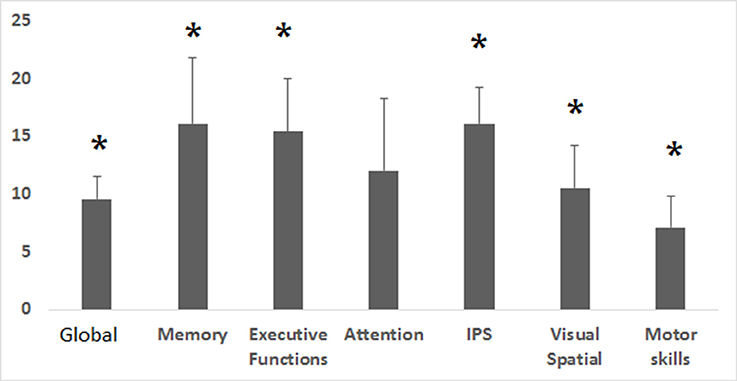
Figure 1. Cognitive indices relative changes post HBOT. Relative change in the corresponding cognitive indices after HBOT. Relative change was calculated by (post HBOT-pre HBOT)/Pre HBOT. IPS, Information processing speed. *p < 0.05.
Brain Microstructure Integrity Changes
Voxel-based DTI analysis was compared before and after HBOT using paired t-test. FA and MD whole brain maps are depicted in Figures 2, 3, and show the statistically significant increase in FA (yellow in Figure 2) and decrease in MD (blue in Figure 3) average values.
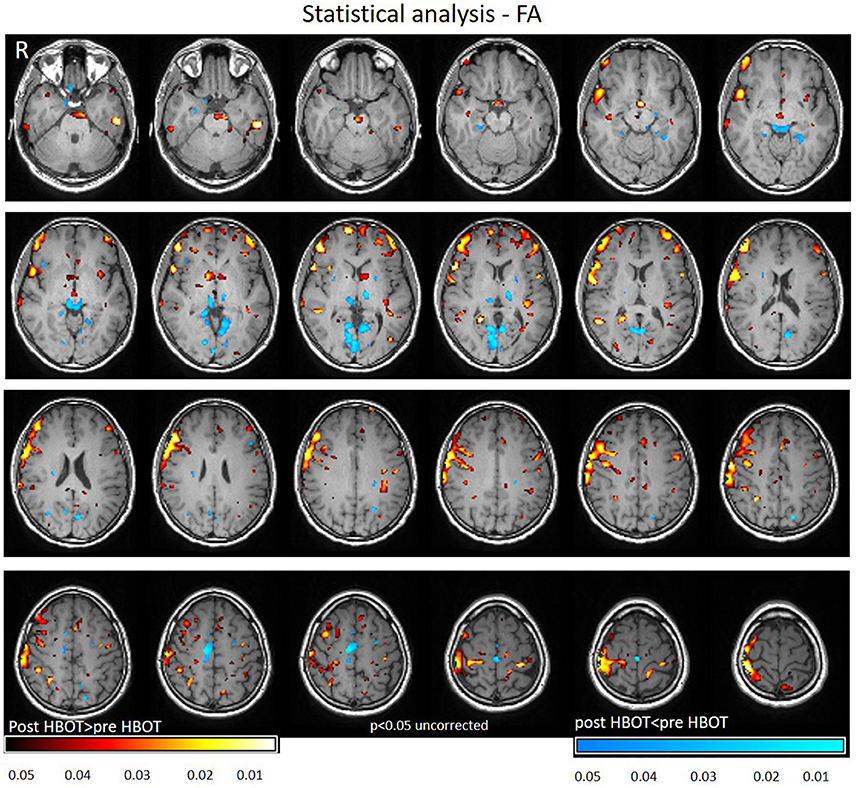
Figure 2. Average DTI normalized delta change in FA maps. Yellow and red areas show a statistically significant increase in FA (p < 0.05).
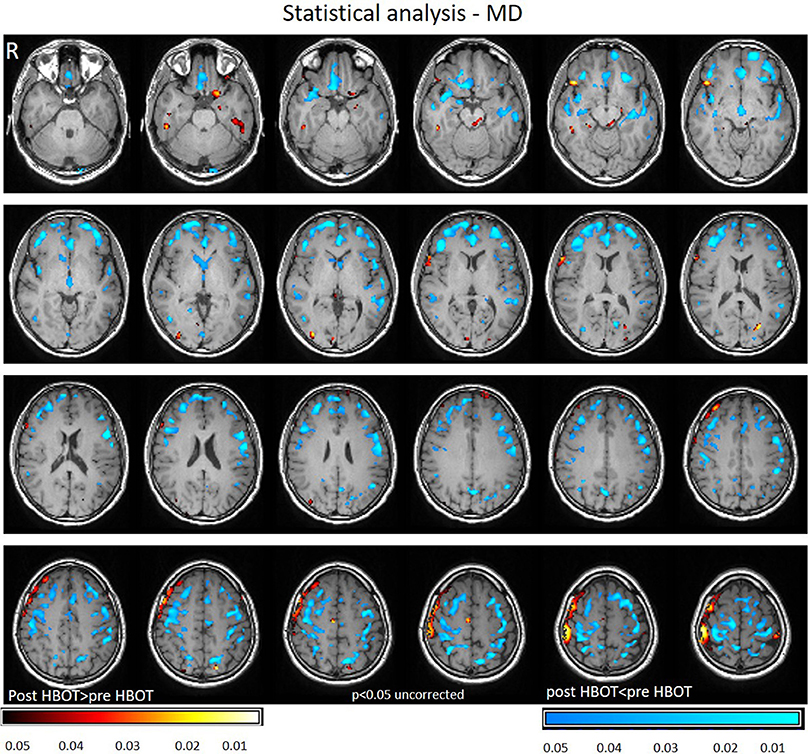
Figure 3. Average DTI normalized delta change in MD maps. Blue areas mark statistically significant decrease in MD (p < 0.05).
Regional Changes in Brain Microstructure Integrity
Statistically significant increase in FA was found in regions related to motor function (internal capsule, midbrain), association fiber tracts inferior fronto-occipial fasciculus (IFOF), inferior longitudinal fasciculus (ILF), superior longitudinal fasciculus (SLF), Cingulum and in the genu of the Corpus Callosum.
Decrease in FA was found in areas related to the visual system (superior colliculi, calcarine sulcus) and other cognitive areas (thalamus, and posterior cingulate gyrus). Graphs of FA in significant clusters are presented in Figures 4A,B.
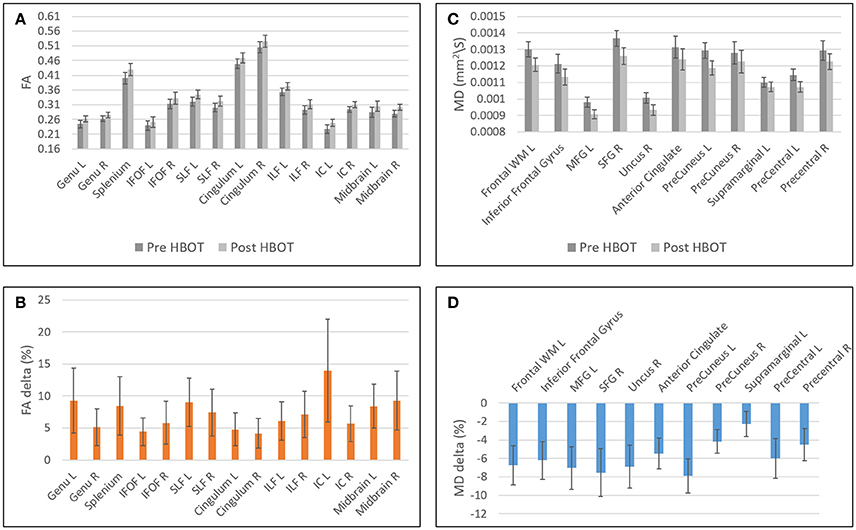
Figure 4. Graphs of FA and MD averages and standard error in statistically significant clusters. (A) Averages of FA before and after HBOT. (B) Normalized delta of FA maps. (C) Averages of MD before and after HBOT. (D) Normalized delta of MD maps.
Decrease in MD was found in the frontal lobe (anterior cingulate gyrus, posterior orbital gyrus, Precuneus, superior frontal gyrus, Uncinate fasciculus, and frontal lobe white matter, left middle frontal gyrus, precentral gyrus). Graphs of MD in significant clusters are presented in Figures 4C,D.
White Matter Tracts Integrity
Fiber tracking analysis revealed a statistically significant increase in number of fibers in the left cingulum (p = 0.03) (Figure 5) and in the right ILF following HBOT (p = 0.029) and in the right Uncinate fasciculus (p = 0.04) (Figure 5).
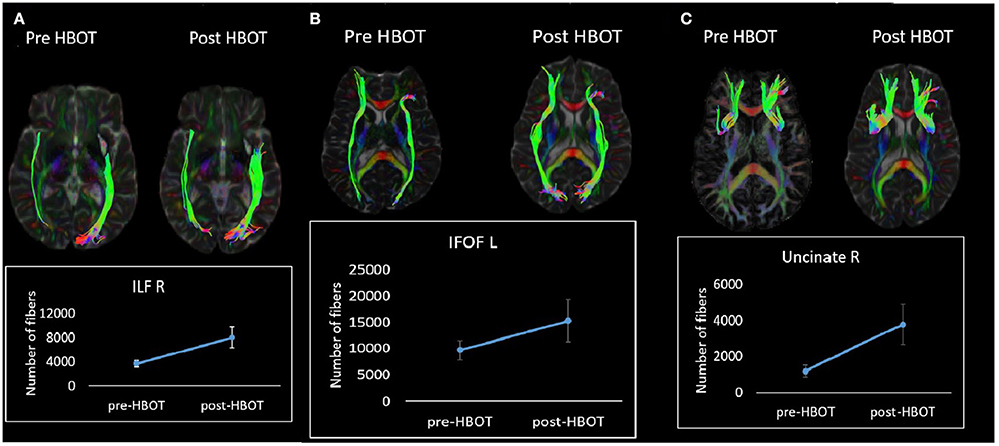
Figure 5. White matter tractography change in a single patient. (A) Fibers number increase in the right ILF tract. (B) Fibers number increase in the left IFOF tract. (C) Fibers increase in the right Uncinate tract.
Increased Brain Perfusion
Voxel-based DSC analysis was compared before and after HBOT using paired t-test. Average CBV and CBF and delta whole brain maps are depicted in Figure 5, and show the increase in both CBF and CBV post HBOT.
Regional Changes in Brain Perfusion
Statically significant increases in CBF involved frontal white matter (including corpus callosum), association fibers (SLF, IFOF), motor function-related structures (corona radiata, midbrain, and cerebellum) and structures related to memory function (temporal GM and fornix).
Statistically significant increase in CBV was found in frontal white matter (including Uncinate fasiculus and Corpus Callosum), frontal gray matter (anterior cingulate), regions related to sensory-motor function and executive functions (including the thalamus and midbrain) association fiber tracts (SLF, ILF and cingulum) and regions related to memory function (hippocampus and fornix) (Figures 6, 7).
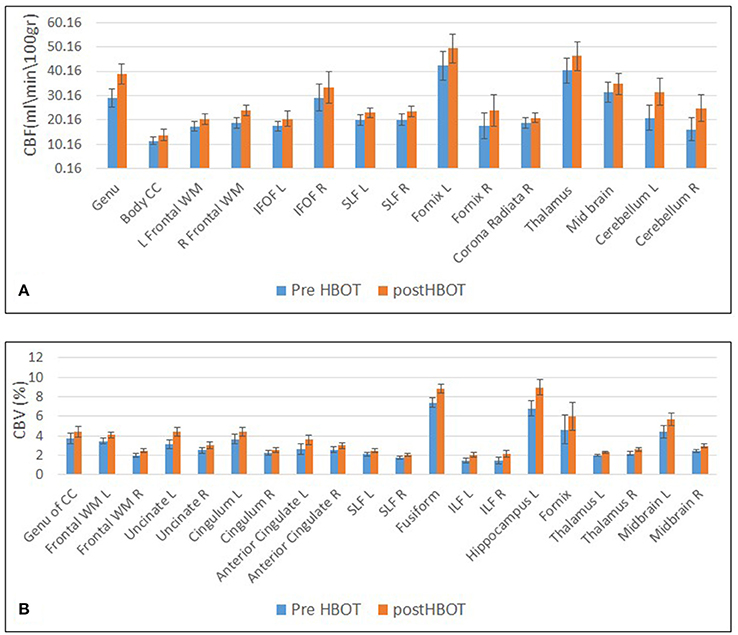
Figure 6. Graphs of CBF and CBV averages and standard error in statistically significant clusters. (A) Averages of CBF before and after HBOT. (B) Averages of CBV before and after HBOT.
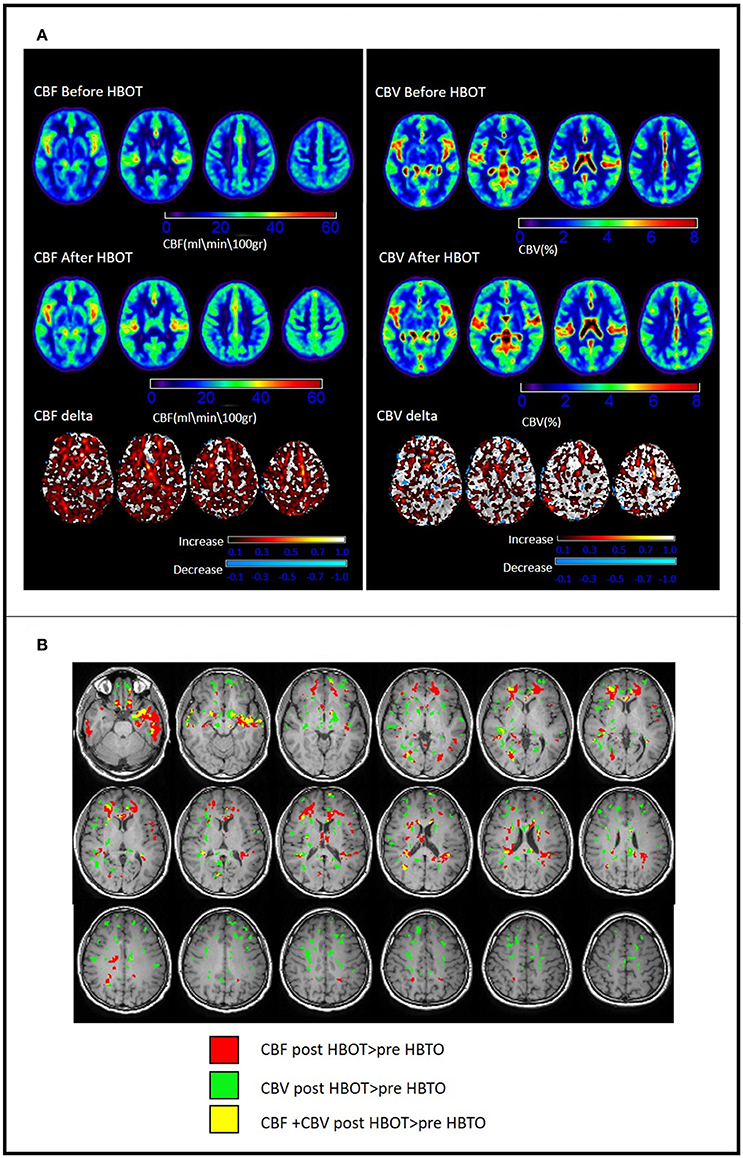
Figure 7. Changes in brain perfusion (CBF and CBV) post HBOT. (A) Average DSC maps pre and post HBOT and DSC normalized delta maps. Top row: CBF and CBV pre-HBOT. Middle row: CBF and CBV maps post-HBOT. Bottom row: normalized delta maps, showing diffuse increases in CBF and CBV post-HBOT. (B) Significant CBF and CBV normalized delta changes post HBOT. Areas of maximal statistically significant increase in perfusion.
Most of the anatomic structures that presented a significant increase in CBF also exhibited an increase in CBV (Figure 6).
Discussion
The current study shows, for the first time in humans, that HBOT can induce brain microstructure recovery in TBI patients. Brain recovery encompassed gray and white matter areas, white matter tracts and angiogenesis. Post HBOT, FA increased and MD decreased in the DTI sequence, CBV and CBF increased in the DSC (perfusion) sequence, along with improved cognitive functions. Recovery was induced in the late chronic stage of TBI (6.7 ± 2.1 years post injury).
Previous studies using DTI have reported that patients suffering from TBI may still have microstructural damage months to years after the initial injury (Kraus et al., 2007; Yuan et al., 2007; Lipton et al., 2008; Sugiyama et al., 2009; Hartikainen et al., 2010; Niogi and Mukherjee, 2010; Murugavel et al., 2014; Perez et al., 2014; Li et al., 2016). DTI characterizes the diffusion of water in the tissues, thus it indicates microstructural density, spacing, and orientational organization of cell membranes, including myelin (Assaf and Pasternak, 2008; Alexander et al., 2011). Animal studies of brain plasticity, revealed that decrease in MD and increase in FA correlates with synaptophysin (a marker of synaptic vesicles), glial fibrillary acidic protein (GFAP; a marker of astrocyte activation), and brain-derived neurotrophic factor (BDNF; a marker of neuronal growth that facilitates learning) (Sagi et al., 2012). With regards to white matter, it was found that myelin density estimated by DTI can accurately predict the actual myelin density seen/measured by electron microscopy (Sepehrband et al., 2015). The injury can be demonstrated by increased water diffusion as measured by MD and reduced directionality of diffusion as measured by FA, suggesting that either axonal injury or disruption of myelination could have altered brain connectivity (Kraus et al., 2007; Yuan et al., 2007; Lipton et al., 2008; Sugiyama et al., 2009; Hartikainen et al., 2010; Niogi and Mukherjee, 2010; Murugavel et al., 2014; Perez et al., 2014; Li et al., 2016). Moreover, FA changes appear to correlate with the severity of the clinical presentation (Benson et al., 2007; Yuan et al., 2007). The observed decrease in FA values may reflect the barriers to axoplasmic transport, the local accumulation of apoptosis in organelles, and secondary Wallerian degeneration in the white matter, while the increased MD values may be the result of vasogenic cerebral edema.
Significant injury foci were reported in dedicated pathways involved in the transmission of efferent and afferent information, such as the corpus callosum, internal capsule, SLF, ILF, SFO, superior frontal gyrus, insula, and fornix (Yuan et al., 2007; Caeyenberghs et al., 2010). Importantly, the microstructure injuries depicted by the DTI imaging markers, FA and MD, correlated with objective measures of general and cognitive functioning (Benson et al., 2007; Assaf and Pasternak, 2008; Sugiyama et al., 2009; Caeyenberghs et al., 2010; Hartikainen et al., 2010; Alexander et al., 2011; Sagi et al., 2012; Wada et al., 2012; Arenth et al., 2014; Haberg et al., 2015; Sepehrband et al., 2015; Li et al., 2016). Disorders in reaction time, executive functions, information processing speed, attention and memory were correlated with axonal lesions in different areas. Moreover, in two recent longitudinal studies, FA values increased in patients with favorable outcome within 6–12 months, while no DTI changes registered in patients with unfavorable outcome (Sidaros et al., 2008; Hartikainen et al., 2010).
In the current study, for the first time in humans, DTI changes of chronic TBI were evaluated before and after HBOT. The increase in FA and decrease in MD post HBOT, together with cognitive function improvement of patients in the late chronic stage of TBI, suggest that brain microstructure recovery can be induced by HBOT.
DTI values, FA and MD, were found in our study to correlate with the improvements in cognitive functions in concordance with previous studies (Sugiyama et al., 2009; Wada et al., 2012; Arenth et al., 2014; Haberg et al., 2015). Memory, executive function and information processing speed were all significantly improved. In correlation with these cognitive improvements, MD decreased in most of the frontal lobe white matter, such as the prefrontal cortex that enables executive control (Miller and Cohen, 2001) and the anterior cingulate gyrus involved in error detection, especially in a Stroop task (Bush et al., 2000). Also, FA increased in most of the long association fibers critical for proper cognitive function:
• SLF–Bi-directional connection of the hemispheric frontal, parietal, temporal and occipital lobes. The SLF plays an important role in high brain functions, particularly language, reflected in information processing speed and executive function tasks (Heilman et al., 1970; Rocha et al., 2005; Sasson et al., 2013). In correlation with those changes, there was a significant improvement in neurocognitive test results in both information processing speed (IPS) and executive functions (EF) (i.e., IPS: 13.1 ± 2.7, p < 0.0001; EF: 11.3 ± 2.7, p < 0.001).
• ILF–Connection between the temporal and occipital lobes on the same hemisphere. The ILF is known to play an important role in visual memory (Bauer and Trobe, 1984; Shinoura et al., 2007). In correlation with those changes, there was a significant improvement in the memory index, which includes a visual memory task (i.e., Memory: 10.5 ± 2.4, p < 0.001).
• Cingulum – A cluster of white matter fibers projecting from the cingulate gyrus in the frontal lobe to the entorhinal cortex in the temporal lobe. The cingulum has been tightly associated with memory disorders (Charlton et al., 2006; Sepulcre et al., 2009). The memory correlates also with the changes in the cingulum.
• Genu of the Corpus Callosum-The largest white matter structure in the brain. It connects the left and right cerebral hemispheres and facilitates interhemispheric communication. Integrity of the corpus callosum is linked to information processing speed and episodic memory (Bucur et al., 2008; Lockhart and DeCarli, 2014). The improvement in information processing speed and memory indices may also correlate with the improvement in the genu of the corpus callosum.
Mechanisms of neuroplasticity and cellular repair by HBOT have been suggested in many animal studies (Hadanny and Efrati, 2016). These include enhanced mitochondrial function and cellular metabolism, improved blood brain barrier and inflammatory reactions, reduced apoptosis, alleviation of oxidative stress, increased levels of neurotrophins and nitric oxide, and up-regulation of axonal guidance agents (Efrati et al., 2013; Efrati and Ben-Jacob, 2014). Moreover, the effects of HBOT on neurons may be mediated indirectly by glial cells. HBOT may also promote neurogenesis of endogenous neural stem cells (Efrati et al., 2013; Efrati and Ben-Jacob, 2014). HBOT may enable the metabolic change simply by supplying the missing oxygen/energy needed for these regeneration processes (Efrati et al., 2013; Efrati and Ben-Jacob, 2014). The ability of HBOT to induce angiogenesis was demonstrated in several different pre-clinical studies (Mu et al., 2011; Lin et al., 2012; Lee et al., 2013; Hu et al., 2014). Hu et al. have demonstrated that HBOT-induced neurogenesis is mediated by ROS/HIF-1α/β-catenin pathway (Hu et al., 2014). In the current study, it is demonstrated that HBOT can induce neuroplasticity in humans even years after the acute insult.
Along with the structural changes, HBOT induces angiogenesis, as shown by the increase of CBF and CBV in this study as well as in our previous study (Tal et al., 2015). The injured areas in the brains post TBI experience hypoxia and hypoperfusion, which serve as a rate-limiting factor for any regenerative process (Graham and Adams, 1971; Graham et al., 1978; Stein et al., 2004; Kim et al., 2010; Ostergaard et al., 2014). HBOT-induced angiogenesis has been amply confirmed in pre-clinical models and can be deduced from brain SPECTs of patients post stroke and post TBI even years after the acute insult (Lin et al., 2012; Boussi-Gross et al., 2013; Efrati et al., 2013; Peng et al., 2014; Duan et al., 2015). The generation of new microvessels renders the local environment non-hypoxic, thus able to induce brain plasticity, enhance neurogenesis and synaptogenesis and foster functional recovery (Chen et al., 2003; Jiang et al., 2005). Unsurprisingly, CBV and CBF increased in the long association fiber tracts discussed above, including corpus callosum, association fibers (SLF, IFOF) and cingulum. Angiogenesis and increased perfusion to the malfunctioning tissue, seen in DSC, serve as infrastructure for the regenerative process and the preservation of newly generated metabolic functioning of the axonal microstructure seen in DTI.
Our study has several limitations. The major one is related to lack of control group. However, one can hardly expect any significant changes in DSC and DTI values or neurocognitive improvement to occur spontaneously years after the acute insult. The cognitive improvement seen here is in line with our earlier randomized controlled trial on patients suffering from mild TBI. In our previous randomized control study it was clearly demonstrated that the control group had no neurocognitive improvement (same cognitive tests used in the current study) or significant change of in brain perfusion measured by SPECT 1-5 years after the acute insult (Boussi-Gross et al., 2013). Nevertheless, one can hardly expect any significant changes in DSC and DTI values or neurocognitive improvement occurring spontaneously years after the acute insult. In addition, a previous randomized controlled trial with a control group showed neurocognitive effects and brain perfusion improvement using SPECT (Boussi-Gross et al., 2013).
Conclusion
HBOT can induce cerebral angiogenesis and recovery of brain microstructure in patients with chronic cognitive impairments due to TBI months to years after the acute injury. The increased integrity of brain fibers correlates with the functional cognitive improvement. The mechanism by which HBOT can induce brain neuroplasticity can be demonstrated by highly sensitive perfusion MRI and DTI. Further studies, using DTI - MRI, are needed in order to gain better understanding of the neuroplasticity effect of HBOT in a larger cohort of patients with different types of brain injuries.
Ethics Statement
This study was carried out in accordance with the recommendations of Assaf Harfoeh Medical Center Institutional Review Board (0030-15-ASF) with written informed consent from all subjects. All subjects gave written informed consent in accordance with the Declaration of Helsinki. The protocol was approved by the Assaf Harfoeh Medical Center Institutional Review Board (0030-15-ASF).
Author Contributions
Conceived and designed the experiments: AH, ST, and SE. Performed the experiments: AH, ST, GS, and SE. Analyzed the data: AH, ES, and SE. Contributed analysis tools: AH, ST, ES, and SE. Wrote the paper: AH, ST, GS, ES, and SE.
Conflict of Interest Statement
The authors declare that the research was conducted in the absence of any commercial or financial relationships that could be construed as a potential conflict of interest.
Acknowledgments
Special thanks to Ms. Michal Ben-Jacob and Mr. Raz Tuval for reviewing and proofing the manuscript.
Supplementary Material
The Supplementary Material for this article can be found online at: https://www.frontiersin.org/articles/10.3389/.2017.00508/full#supplementary-material
Abbreviations
CBF, cerebral blood flow; CBV, cerebral blood volume.
References
Achiron, A., Chapman, J., Magalashvili, D., Dolev, M., Lavie, M., Bercovich, E., et al. (2013). Modeling of cognitive impairment by disease duration in multiple sclerosis: a cross-sectional study. PLoS ONE 8:e71058. doi: 10.1371/journal.pone.0071058
Alexander, A. L., Hurley, S. A., Samsonov, A. A., Adluru, N., Hosseinbor, A. P., Mossahebi, P., et al. (2011). Characterization of cerebral white matter properties using quantitative magnetic resonance imaging stains. Brain Connect. 1, 423–446. doi: 10.1089/brain.2011.0071
Arenth, P. M., Russell, K. C., Scanlon, J. M., Kessler, L. J., and Ricker, J. H. (2014). Corpus callosum integrity and neuropsychological performance after traumatic brain injury: a diffusion tensor imaging study. J. Head Trauma Rehabil. 29, E1–E10. doi: 10.1097/HTR.0b013e318289ede5
Assaf, Y., and Pasternak, O. (2008). Diffusion tensor imaging (DTI)-based white matter mapping in brain research: a review. J. Mol. Neurosci. 34, 51–61. doi: 10.1007/s12031-007-0029-0
Barrett, K. F., Masel, B., Patterson, J., Scheibel, R. S., Corson, K. P., and Mader, J. T. (2004). Regional CBF in chronic stable TBI treated with hyperbaric oxygen. Undersea Hyperb. Med. 31, 395–406.
Bauer, R. M., and Trobe, J. D. (1984). Visual memory and perceptual impairments in prosopagnosia. J. Clin. Neuroophthalmol. 4, 39–46.
Bazarian, J. J., McClung, J., Shah, M. N., Cheng, Y. T., Flesher, W., and Kraus, J. (2005). Mild traumatic brain injury in the United States, 1998–2000. Brain Inj. 19, 85–91.
Benson, R. R., Meda, S. A., Vasudevan, S., Kou, Z., Govindarajan, K. A., Hanks, R. A., et al. (2007). Global white matter analysis of diffusion tensor images is predictive of injury severity in traumatic brain injury. J. Neurotrauma 24, 446–459. doi: 10.1089/neu.2006.0153
Boussi-Gross, R., Golan, H., Fishlev, G., Bechor, Y., Volkov, O., Bergan, J., et al. (2013). Hyperbaric oxygen therapy can improve post concussion syndrome years after mild traumatic brain injury - randomized prospective trial. PLoS ONE 8:e79995. doi: 10.1371/journal.pone.0079995
Bucur, B., Madden, D. J., Spaniol, J., Provenzale, J. M., Cabeza, R., White, L. E., et al. (2008). Age-related slowing of memory retrieval: contributions of perceptual speed and cerebral white matter integrity. Neurobiol. Aging 29, 1070–1079. doi: 10.1016/j.neurobiolaging.2007.02.008
Bush, G., Luu, P., and Posner, M. I. (2000). Cognitive and emotional influences in anterior cingulate cortex. Trends Cogn. Sci. 4, 215–222. doi: 10.1016/S1364-6613(00)01483-2
Caeyenberghs, K., Leemans, A., Geurts, M., Taymans, T., Vander Linden, C., Smits-Engelsman, B. C., et al. (2010). Brain-behavior relationships in young traumatic brain injury patients: fractional anisotropy measures are highly correlated with dynamic visuomotor tracking performance. Neuropsychologia 48, 1472–1482. doi: 10.1016/j.neuropsychologia.2010.01.017
Charlton, R. A., Barrick, T. R., McIntyre, D. J., Shen, Y., O'Sullivan, M., Howe, F. A., et al. (2006). White matter damage on diffusion tensor imaging correlates with age-related cognitive decline. Neurology 66, 217–222. doi: 10.1212/01.wnl.0000194256.15247.83
Chen, J., Zhang, Z. G., Li, Y., Wang, L., Xu, Y. X., Gautam, S. C., et al. (2003). Intravenous administration of human bone marrow stromal cells induces angiogenesis in the ischemic boundary zone after stroke in rats. Circ. Res. 92, 692–699. doi: 10.1161/01.RES.0000063425.51108.8D
Chen, Z., Ni, P., Lin, Y., Xiao, H., Chen, J., Qian, G., et al. (2010). Visual pathway lesion and its development during hyperbaric oxygen treatment: a bold- fMRI and DTI study. J. Magn. Reson. Imaging 31, 1054–1060. doi: 10.1002/jmri.22142
Chiu, W. T., and LaPorte, R. E. (1993). Global spine and head injury prevention (SHIP) project. J. Trauma 35, 969–970. doi: 10.1097/00005373-199312000-00034
Duan, S., Shao, G., Yu, L., and Ren, C. (2015). Angiogenesis contributes to the neuroprotection induced by hyperbaric oxygen preconditioning against focal cerebral ischemia in rats. Int. J. Neurosci. 125, 625–634. doi: 10.3109/00207454.2014.956101
Dwolatzky, T., Whitehead, V., Doniger, G. M., Simon, E. S., Schweiger, A., Jaffe, D., et al. (2003). Validity of a novel computerized cognitive battery for mild cognitive impairment. BMC Geriatr. 3:4. doi: 10.1186/1471-2318-3-4
Efrati, S., and Ben-Jacob, E. (2014). Reflections on the neurotherapeutic effects of hyperbaric oxygen. Expert Rev. Neurother. 14, 233–236. doi: 10.1586/14737175.2014.884928
Efrati, S., Fishlev, G., Bechor, Y., Volkov, O., Bergan, J., Kliakhandler, K., et al. (2013). Hyperbaric oxygen induces late neuroplasticity in post stroke patients–randomized, prospective trial. PLoS ONE 8:e53716. doi: 10.1371/journal.pone.0053716
Golden, Z., Golden, C. J., and Neubauer, R. A. (2006). Improving neuropsychological function after chronic brain injury with hyperbaric oxygen. Disabil. Rehabil. 28, 1379–1386. doi: 10.1080/09638280600638364
Graham, D. I., Adams, J. H., and Doyle, D. (1978). Ischaemic brain damage in fatal non-missile head injuries. J. Neurol. Sci. 39, 213–234. doi: 10.1016/0022-510X(78)90124-7
Graham, D. I., and Adams, J. H. (1971). Ischaemic brain damage in fatal head injuries. Lancet 1, 265–266. doi: 10.1016/S0140-6736(71)91003-8
Haberg, A. K., Olsen, A., Moen, K. G., Schirmer-Mikalsen, K., Visser, E., Finnanger, T. G., et al. (2015). White matter microstructure in chronic moderate-to-severe traumatic brain injury: impact of acute-phase injury-related variables and associations with outcome measures. J. Neurosci. Res. 93, 1109–1126. doi: 10.1002/jnr.23534
Hadanny, A., and Efrati, S. (2016). Treatment of persistent post-concussion syndrome due to mild traumatic brain injury: current status and future directions. Expert Rev. Neurother. 16, 875–887. doi: 10.1080/14737175.2016.1205487
Harch, P. G., Andrews, S. R., Fogarty, E. F., Amen, D., Pezzullo, J. C., Lucarini, J., et al. (2012). A phase I study of low-pressure hyperbaric oxygen therapy for blast-induced post-concussion syndrome and post-traumatic stress disorder. J. Neurotrauma 29, 168–185. doi: 10.1089/neu.2011.1895
Hartikainen, K. M., Waljas, M., Isoviita, T., Dastidar, P., Liimatainen, S., Solbakk, A. K., et al. (2010). Persistent symptoms in mild to moderate traumatic brain injury associated with executive dysfunction. J. Clin. Exp. Neuropsychol. 32, 767–774. doi: 10.1080/13803390903521000
Heilman, K. M., Pandya, D. N., and Geschwind, N. (1970). Trimodal inattention following parietal lobe ablations. Trans. Am. Neurol. Assoc. 95, 259–261.
Hu, Q., Liang, X., Chen, D., Chen, Y., Doycheva, D., Tang, J., et al. (2014). Delayed hyperbaric oxygen therapy promotes neurogenesis through reactive oxygen species/hypoxia-inducible factor-1alpha/beta-catenin pathway in middle cerebral artery occlusion rats. Stroke 45, 1807–1814. doi: 10.1161/STROKEAHA.114.005116
Huang, L., and Obenaus, A. (2011). Hyperbaric oxygen therapy for traumatic brain injury. Med. Gas Res. 1:21. doi: 10.1186/2045-9912-1-21
Iverson, G. L. (2005). Outcome from mild traumatic brain injury. Curr. Opin. Psychiatry 18, 301–317. doi: 10.1097/01.yco.0000165601.29047.ae
Jiang, Q., Zhang, Z. G., Ding, G. L., Zhang, L., Ewing, J. R., Wang, L., et al. (2005). Investigation of neural progenitor cell induced angiogenesis after embolic stroke in rat using MRI. Neuroimage 28, 698–707. doi: 10.1016/j.neuroimage.2005.06.063
Kashluba, S., Paniak, C., Blake, T., Reynolds, S., Toller-Lobe, G., and Nagy, J. (2004). A longitudinal, controlled study of patient complaints following treated mild traumatic brain injury. Arch. Clin. Neuropsychol. 19, 805–816. doi: 10.1016/j.acn.2003.09.005
Kim, J., Whyte, J., Patel, S., Avants, B., Europa, E., Wang, J., et al. (2010). Resting cerebral blood flow alterations in chronic traumatic brain injury: an arterial spin labeling perfusion FMRI study. J. Neurotrauma 27, 1399–1411. doi: 10.1089/neu.2009.1215
King, N. S., and Kirwilliam, S. (2011). Permanent post-concussion symptoms after mild head injury. Brain Inj. 25, 462–470. doi: 10.3109/02699052.2011.558042
Kraus, M. F., Susmaras, T., Caughlin, B. P., Walker, C. J., Sweeney, J. A., and Little, D. M. (2007). White matter integrity and cognition in chronic traumatic brain injury: a diffusion tensor imaging study. Brain 130, 2508–2519. doi: 10.1093/brain/awm216
Lee, Y. S., Chio, C. C., Chang, C. P., Wang, L. C., Chiang, P. M., Niu, K. C., et al. (2013). Long course hyperbaric oxygen stimulates neurogenesis and attenuates inflammation after ischemic stroke. Mediators Inflamm. 2013:512978. doi: 10.1155/2013/512978
Leemans, A., Jeurissen, B., Sijbers, J., and Jones, D. K. (2009). “ExploreDTI: a graphical toolbox for processing, analyzing, and visualizing diffusion MR data,” in Proceedings of the 17th Annual Meeting of the International Society for Magnetic Resonance in Medicine (Hawaii), 3537. Available online at: http://www.exploredti.com/ref/ExploreDTI_ISMRM_2009.pdf
Li, L., Sun, G., Liu, K., Li, M., Li, B., Qian, S. W., et al. (2016). White matter changes in posttraumatic stress disorder following mild traumatic brain injury: a prospective longitudinal diffusion tensor imaging study. Chin. Med. J. 129, 1091–1099. doi: 10.4103/0366-6999.180518
Lin, K. C., Niu, K. C., Tsai, K. J., Kuo, J. R., Wang, L. C., Chio, C. C., et al. (2012). Attenuating inflammation but stimulating both angiogenesis and neurogenesis using hyperbaric oxygen in rats with traumatic brain injury. J. Trauma Acute Care Surg. 72, 650–659. doi: 10.1097/TA.0b013e31823c575f
Lipton, M. L., Gellella, E., Lo, C., Gold, T., Ardekani, B. A., Shifteh, K., et al. (2008). Multifocal white matter ultrastructural abnormalities in mild traumatic brain injury with cognitive disability: a voxel-wise analysis of diffusion tensor imaging. J. Neurotrauma 25, 1335–1342. doi: 10.1089/neu.2008.0547
Lockhart, S. N., and DeCarli, C. (2014). Structural imaging measures of brain aging. Neuropsychol. Rev. 24, 271–289. doi: 10.1007/s11065-014-9268-3
Malec, J. F., Brown, A. W., Leibson, C. L., Flaada, J. T., Mandrekar, J. N., Diehl, N. N., et al. (2007). The mayo classification system for traumatic brain injury severity. J. Neurotrauma 24, 1417–1424. doi: 10.1089/neu.2006.0245
Marin, J. R., Weaver, M. D., Yealy, D. M., and Mannix, R. C. (2014). Trends in visits for traumatic brain injury to emergency departments in the United States. JAMA 311, 1917–1919. doi: 10.1001/jama.2014.3979
McCauley, S. R., Boake, C., Pedroza, C., Brown, S. A., Levin, H. S., Goodman, H. S., et al. (2005). Postconcussional disorder: are the DSM-IV criteria an improvement over the ICD-10? J. Nerv. Ment. Dis. 193, 540–550. doi: 10.1097/01.nmd.0000172592.05801.71
McCrory, P., Johnston, K., Meeuwisse, W., Aubry, M., Cantu, R., Dvorak, J., et al. (2005). Summary and agreement statement of the 2nd International Conference on Concussion in Sport, Prague 2004. Br. J. Sports Med. 39, 196–204. doi: 10.1136/bjsm.2005.018614
Melton, J. L. (2005). Psychometric Evaluation of the Mindstreams Neuropsychological Screening Tool Panama City, FL: Navy Experimental Diving Unit (US).
Miller, E. K., and Cohen, J. D. (2001). An integrative theory of prefrontal cortex function. Annu. Rev. Neurosci. 24, 167–202. doi: 10.1146/annurev.neuro.24.1.167
Mu, J., Krafft, P. R., and Zhang, J. H. (2011). Hyperbaric oxygen therapy promotes neurogenesis: where do we stand? Med. Gas Res. 1:14. doi: 10.1186/2045-9912-1-14
Murugavel, M., Cubon, V., Putukian, M., Echemendia, R., Cabrera, J., Osherson, D., et al. (2014). A longitudinal diffusion tensor imaging study assessing white matter fiber tracts after sports-related concussion. J. Neurotrauma 31, 1860–1871. doi: 10.1089/neu.2014.3368
Neubauer, R. A., and James, P. (1998). Cerebral oxygenation and the recoverable brain. Neurol. Res. 20(Suppl. 1), S33–S36. doi: 10.1080/01616412.1998.11740606
Niogi, S. N., and Mukherjee, P. (2010). Diffusion tensor imaging of mild traumatic brain injury. J. Head Trauma Rehabil. 25, 241–255. doi: 10.1097/HTR.0b013e3181e52c2a
Ostergaard, L., Engedal, T. S., Aamand, R., Mikkelsen, R., Iversen, N. K., Anzabi, M., et al. (2014). Capillary transit time heterogeneity and flow-metabolism coupling after traumatic brain injury. J. Cereb. Blood Flow Metab. 34, 1585–1598. doi: 10.1038/jcbfm.2014.131
Østergaard, L., Sorensen, A. G., Kwong, K. K., Weisskoff, R. M., Gyldensted, C., and Rosen, B. R. (1996a). High resolution measurement of cerebral blood flow using intravascular tracer bolus passages. Part II: experimental comparison and preliminary results. Magn. Reson. Med. 36, 726–736.
Østergaard, L., Weisskoff, R. M., Chesler, D. A., Gyldensted, C., and Rosen, B. R. (1996b). High resolution measurement of cerebral blood flow using intravascular tracer bolus passages. Part I: mathematical approach and statistical analysis. Magn. Reson. Med. 36, 715–725.
Peng, Z. R., Yang, A. L., and Yang, Q. D. (2014). The effect of hyperbaric oxygen on intracephalic angiogenesis in rats with intracerebral hemorrhage. J. Neurol. Sci. 342, 114–123. doi: 10.1016/j.jns.2014.04.037
Perez, A. M., Adler, J., Kulkarni, N., Strain, J. F., Womack, K. B., and Diaz-Arrastia, R. (2014). Longitudinal white matter changes after traumatic axonal injury. J. Neurotrauma 31, 1478–1485. doi: 10.1089/neu.2013.3216
Rocha, F. T., Rocha, A. F., Massad, E., and Menezes, R. (2005). Brain mappings of the arithmetic processing in children and adults. Brain Res. Cogn. Brain Res. 22, 359–372. doi: 10.1016/j.cogbrainres.2004.09.008
Sagi, Y., Tavor, I., Hofstetter, S., Tzur-Moryosef, S., Blumenfeld-Katzir, T., and Assaf, Y. (2012). Learning in the fast lane: new insights into neuroplasticity. Neuron 73, 1195–1203. doi: 10.1016/j.neuron.2012.01.025
Sasson, E., Doniger, G. M., Pasternak, O., Tarrasch, R., and Assaf, Y. (2013). White matter correlates of cognitive domains in normal aging with diffusion tensor imaging. Front. Neurosci. 7:32. doi: 10.3389/fnins.2013.00032
Schweiger, A., Doniger, G. M., Dwolatzky, T., Jaffe, D., and Simon, E. S. (2003). Reliability of a novel computerized neuropsychological battery for mild cognitive impairment. Acta Neuropsychol. 1, 407–413.
Selassie, A. W., Wilson, D. A., Pickelsimer, E. E., Voronca, D. C., Williams, N. R., and Edwards, J. C. (2013). Incidence of sport-related traumatic brain injury and risk factors of severity: a population-based epidemiologic study. Ann. Epidemiol. 23, 750–756. doi: 10.1016/j.annepidem.2013.07.022
Sepehrband, F., Clark, K. A., Ullmann, J. F., Kurniawan, N. D., Leanage, G., Reutens, D. C., et al. (2015). Brain tissue compartment density estimated using diffusion-weighted MRI yields tissue parameters consistent with histology. Hum. Brain Mapp. 36, 3687–3702. doi: 10.1002/hbm.22872
Sepulcre, J., Masdeu, J. C., Pastor, M. A., Goni, J., Barbosa, C., Bejarano, B., et al. (2009). Brain pathways of verbal working memory: a lesion-function correlation study. Neuroimage 47, 773–778. doi: 10.1016/j.neuroimage.2009.04.054
Shi, X. Y., Tang, Z. Q., Sun, D., and He, X. J. (2006). Evaluation of hyperbaric oxygen treatment of neuropsychiatric disorders following traumatic brain injury. Chin. Med. J. 119, 1978–1982.
Shi, X. Y., Tang, Z. Q., Xiong, B., Bao, J. X., Sun, D., Zhang, Y. Q., et al. (2003). Cerebral perfusion SPECT imaging for assessment of the effect of hyperbaric oxygen therapy on patients with postbrain injury neural status. Chin. J. Traumatol. 6, 346–349.
Shinoura, N., Suzuki, Y., Tsukada, M., Katsuki, S., Yamada, R., Tabei, Y., et al. (2007). Impairment of inferior longitudinal fasciculus plays a role in visual memory disturbance. Neurocase 13, 127–130. doi: 10.1080/13554790701399254
Sidaros, A., Engberg, A. W., Sidaros, K., Liptrot, M. G., Herning, M., Petersen, P., et al. (2008). Diffusion tensor imaging during recovery from severe traumatic brain injury and relation to clinical outcome: a longitudinal study. Brain 131, 559–572. doi: 10.1093/brain/awm294
Stein, S. C., Graham, D. I., Chen, X. H., and Smith, D. H. (2004). Association between intravascular microthrombosis and cerebral ischemia in traumatic brain injury. Neurosurgery 54, 687–691; discussion 691. doi: 10.1227/01.NEU.0000108641.98845.88
Sterr, A., Herron, K. A., Hayward, C., and Montaldi, D. (2006). Are mild head injuries as mild as we think? Neurobehavioral concomitants of chronic post-concussion syndrome. BMC Neurol. 6:7. doi: 10.1186/1471-2377-6-7
Sugiyama, K., Kondo, T., Oouchida, Y., Suzukamo, Y., Higano, S., Endo, M., et al. (2009). Clinical utility of diffusion tensor imaging for evaluating patients with diffuse axonal injury and cognitive disorders in the chronic stage. J. Neurotrauma 26, 1879–1890. doi: 10.1089/neu.2008.0839
Tal, S., Hadanny, A., Berkovitz, N., Sasson, E., Ben-Jacob, E., and Efrati, S. (2015). Hyperbaric oxygen may induce angiogenesis in patients suffering from prolonged post-concussion syndrome due to traumatic brain injury. Restor. Neurol. Neurosci. 33, 943–951. doi: 10.3233/RNN-150585
Thaler, A., Mirelman, A., Gurevich, T., Simon, E., Orr-Urtreger, A., Marder, K., onsortium, A. J., et al. (2012). Lower cognitive performance in healthy G2019S LRRK2 mutation carriers. Neurology 79, 1027–1032. doi: 10.1212/WNL.0b013e3182684646
Vlodavsky, E., Palzur, E., and Soustiel, J. F. (2006). Hyperbaric oxygen therapy reduces neuroinflammation and expression of matrix metalloproteinase-9 in the rat model of traumatic brain injury. Neuropathol. Appl. Neurobiol. 32, 40–50. doi: 10.1111/j.1365-2990.2005.00698.x
Wada, T., Asano, Y., and Shinoda, J. (2012). Decreased fractional anisotropy evaluated using tract-based spatial statistics and correlated with cognitive dysfunction in patients with mild traumatic brain injury in the chronic stage. AJNR Am. J. Neuroradiol. 33, 2117–2122. doi: 10.3174/ajnr.A3141
Wright, J. K., Zant, E., Groom, K., Schlegel, R. E., and Gilliland, K. (2009). Case report: treatment of mild traumatic brain injury with hyperbaric oxygen. Undersea Hyperb. Med. 36, 391–399.
Yuan, W., Holland, S. K., Schmithorst, V. J., Walz, N. C., Cecil, K. M., Jones, B. V., et al. (2007). Diffusion tensor MR imaging reveals persistent white matter alteration after traumatic brain injury experienced during early childhood. AJNR Am. J. Neuroradiol. 28, 1919–1925. doi: 10.3174/ajnr.A0698
Zhang, J. H., Lo, T., Mychaskiw, G., and Colohan, A. (2005). Mechanisms of hyperbaric oxygen and neuroprotection in stroke. Pathophysiology 12, 63–77. doi: 10.1016/j.pathophys.2005.01.003
Keywords: hyperbaric oxygen, DTI, tractography, angiogenesis, MRI, perfusion, TBI, post-concussion
Citation: Tal S, Hadanny A, Sasson E, Suzin G and Efrati S (2017) Hyperbaric Oxygen Therapy Can Induce Angiogenesis and Regeneration of Nerve Fibers in Traumatic Brain Injury Patients. Front. Hum. Neurosci. 11:508. doi: 10.3389/fnhum.2017.00508
Received: 30 March 2017; Accepted: 06 October 2017;
Published: 19 October 2017.
Edited by:
Manousos A. Klados, Aston University, Birmingham, United KingdomReviewed by:
Bariş Saylam, Ankara Numune Training Hospital, TurkeyJacek Kot, Gdańsk Medical University, Poland
Copyright © 2017 Tal, Hadanny, Sasson, Suzin and Efrati. This is an open-access article distributed under the terms of the Creative Commons Attribution License (CC BY). The use, distribution or reproduction in other forums is permitted, provided the original author(s) or licensor are credited and that the original publication in this journal is cited, in accordance with accepted academic practice. No use, distribution or reproduction is permitted which does not comply with these terms.
*Correspondence: Amir Hadanny, YW1pci5oYWRAZ21haWwuY29t
Shai Efrati, ZWZyYXRpc2hhaUAwMTMubmV0
†These authors have contributed equally to this work.