- 1Department of Radiology, Nanjing First Hospital, Nanjing Medical University, Nanjing, China
- 2Department of Otolaryngology, Nanjing First Hospital, Nanjing Medical University, Nanjing, China
- 3Department of Endocrinology, Nanjing First Hospital, Nanjing Medical University, Nanjing, China
- 4Department of Vascular and Interventional Radiology, Nanjing First Hospital, Nanjing Medical University, Nanjing, China
Purpose: The neural mechanisms that give rise to the phantom sound of tinnitus have not been fully elucidated. Neuroimaging studies have revealed abnormalities in resting-state activity that could represent the neural signature of tinnitus, but there is considerable heterogeneity in the data. To address this issue, we conducted a meta-analysis of published neuroimaging studies aimed at identifying a common core of resting-state brain abnormalities in tinnitus patients.
Methods: A systematic search was conducted for whole-brain resting-state neuroimaging studies with SPECT, PET and functional MRI that compared chronic tinnitus patients with healthy controls. The authors searched PubMed, Science Direct, Web of Knowledge and Embase databases for neuroimaging studies on tinnitus published up to September 2016. From each study, coordinates were extracted from clusters with significant differences between tinnitus subjects and controls. Meta-analysis was performed using the activation likelihood estimation (ALE) method.
Results: Data were included from nine resting-state neuroimaging studies that reported a total of 51 distinct foci. The meta-analysis identified consistent regions of increased resting-state brain activity in tinnitus patients relative to controls that included, bilaterally, the insula, middle temporal gyrus (MTG), inferior frontal gyrus (IFG), parahippocampal gyrus, cerebellum posterior lobe and right superior frontal gyrus. Moreover, decreased brain activity was only observed in the left cuneus and right thalamus.
Conclusions: The current meta-analysis is, to our knowledge, the first to demonstrate a characteristic pattern of resting-state brain abnormalities that may serve as neuroimaging markers and contribute to the understanding of neuropathophysiological mechanisms for chronic tinnitus.
Introduction
Tinnitus is the conscious perception of sound in the absence of an internal or external acoustic signal (Jastreboff, 1990). In the United States, an estimated 50 million adults have experienced tinnitus occasionally, and 16 million adults are estimated to experience frequent tinnitus (Shargorodsky et al., 2010). The central nervous system is believed to play a major role in its development and maintenance of tinnitus (Rauschecker et al., 2010; Leaver et al., 2011; De Ridder et al., 2014b; Chen et al., 2015a). Previous electrophysiological and neuroimaging studies (Lockwood et al., 1998; Kaltenbach et al., 2005) suggest that tinnitus may arise from aberrant firing patterns or high levels of spontaneous neural activity in the central auditory pathway rather than the cochlea. However, evidences suggest that other brain regions outside the classical auditory pathway may involve attentional mechanisms that contribute to the persistent awareness of the phantom sound as well as the development of anxiety and distress leading to disabling features of chronic tinnitus (Mirz et al., 2000; Schmidt et al., 2013; Henry et al., 2014). Despite extensive research, the brain abnormalities and neuropathophysiological mechanisms underlying chronic tinnitus remain poorly understood. One of the major questions is what regions of the human brain are involved in tinnitus, a question that has been explored in numerous imaging studies (Jastreboff et al., 1994; Lockwood et al., 1998; Mirz et al., 2000; Rauschecker et al., 2010; De Ridder et al., 2014b).
A number of existing neurophysiological mechanisms and models have been proposed to account for the pathophysiology of tinnitus patients, such as central gain (Schaette and McAlpine, 2011), neural synchrony (Seki and Eggermont, 2003), frontostriatal gating (Rauschecker et al., 2015), thalamocortical dysrhythmia (Llinás et al., 1999), noise-canceling deficit (Rauschecker et al., 2010; Leaver et al., 2011), global workspace (De Ridder et al., 2014b), and precision/predictive coding models (Sedley et al., 2016). However, there is a lack of consensus as to which neural mechanism(s) and what regions of the central nervous system are common to the diverse population of tinnitus patients participating in the imaging studies. Human neuroimaging studies have revealed augmented activity in tinnitus patients in several brain regions within and/or beyond classical auditory pathways. According to the model of De Ridder et al. (2014b) tinnitus is underpinned by the integration of multiple nonspecific subnetworks of the brain involving general components of cognition, emotion and memory. Communication between these different subnetworks occurs at specific hubs, brain areas that are involved in multiple subnetworks simultaneously. These different subnetworks, which interact at different oscillatory frequencies, communicate with one another at partially overlapping hubs. Nonetheless, the role of the potential hubs involved in multiple subnetworks of tinnitus still remains unclear.
Since about 85% of chronic tinnitus patients perceived the phantom sound constantly (Schecklmann et al., 2014), the resting-state fMRI measurements seem well suited to identify the neural structures, subnetworks and hubs involved in tinnitus. A growing number of studies have used resting-state fMRI to investigate tinnitus (Husain and Schmidt, 2014) and multiple brain networks implicated in tinnitus have been identified, such as the auditory network (Burton et al., 2012; Kim et al., 2012; Maudoux et al., 2012a,b; Schmidt et al., 2013; Hinkley et al., 2015; Minami et al., 2015; Leaver et al., 2016b), default mode network (DMN; Schmidt et al., 2013; Chen et al., 2014, 2015d; Leaver et al., 2016b), dorsal attention network (DAN; Burton et al., 2012; Schmidt et al., 2013), ventral attention network (VAN; Burton et al., 2012), and visual network (Burton et al., 2012; Chen et al., 2014, 2015d). As such, tinnitus can be seen as the interaction of multiple brain subnetworks, each contributing to different aspects of tinnitus such as its acoustic features, emotional affect and awareness or attention. However, these studies reported relatively inconsistent results. For instance, most researches demonstrated the increased resting-state brain activity between tinnitus patients and healthy controls (Maudoux et al., 2012a; Chen et al., 2014, 2016; Laureano et al., 2014; Yang et al., 2014; Ueyama et al., 2015), while others failed to identify any regions of increased brain activity (Geven et al., 2014; Leaver et al., 2016b). Moreover, several studies have failed to detect any differences in network processing between tinnitus patients and controls (Wineland et al., 2012; Davies et al., 2014). These reported discrepancies can potentially be attributed to the limited sample size, variable clinical demographics and use of different methods. Furthermore, in many studies tinnitus groups were not compared to an appropriate control group (Leaver et al., 2011; Schecklmann et al., 2013; Lanting et al., 2014).
Tinnitus is a very heterogeneous condition with respect to the characteristics of the perceived sound, degree of associated awareness and distress, duration and comorbidities (Landgrebe et al., 2012). Given the high heterogeneity, it is not surprising that inconsistencies across studies are encountered. Nevertheless, there may be some commonalities across these diverse studies. One approach to identifying a common core is to perform a meta-analysis of the existing resting-state studies of patients with chronic tinnitus with the goal of identifying brain network hubs in tinnitus patients common to neuroimaging studies of tinnitus. Activation likelihood estimation (ALE) is the most common coordinate-based meta-analytic method used to analyze the neuroimaging literature; this approach seeks to identify brain locations with a consistent pattern of response across experiments. This approach is based on the collection of peak coordinates from each study included in the meta-analysis rather than the input of raw images (Turkeltaub et al., 2002; Wager et al., 2004; Laird et al., 2005a; Eickhoff et al., 2009). ALE technique has been successfully applied to neuroimaging studies of various neurological or psychiatric disorders, such as epilepsy (Li et al., 2012), Parkinson’s disease (Shao et al., 2015), schizophrenia (Ellison-Wright and Bullmore, 2010), and narcolepsy (Weng et al., 2015). Song et al. (2012) performed a meta-analysis exclusively of PET studies of tinnitus using the ALE method to retrieve the most consistent activation areas across different task dimensions. However, this meta-analysis did not include the large body of fMRI studies that might prove useful in detecting resting-state brain abnormalities in tinnitus patients. Therefore the goal of the current study was to conduct a quantitative meta-analysis of several different types of resting state neuroimaging data in the tinnitus literature that met our inclusion criterion. To accomplish this, we used the ALE algorithm to determine the resting-state brain abnormalities in tinnitus patients compared to healthy controls. Our working hypothesis was that the ALE analysis would identify a common core of brain regions linked to tinnitus generation despite the heterogeneity of the patients and experimental methods.
Materials and Methods
Search Strategies and Study Selection
Our analysis was performed according to the Meta-Analysis of Observational Studies in Epidemiology (MOOSE) criteria (Stroup et al., 2000). A comprehensive literature search up to September, 2016 was conducted in PubMed, Science Direct, Web of Knowledge, and Embase using the following search terms: (1) “neuroimaging” <OR > “PET” <OR > “fMRI”; (2) “resting state”; and (3) “tinnitus”. Our search was restricted to humans. In addition, we manually reviewed the references cited in articles that were retrieved.
Studies were selected according to the following inclusion criteria: (1) published as an article (and not a letter or an abstract); (2) comparisons of tinnitus patients with healthy control groups on a whole-brain level; and (3) clearly reported Montreal Neurological Institute (MNI) or Talairach coordinates of the activation areas (x, y, z). Studies reporting only findings for specific ROIs were not included in the present meta-analysis. In accordance with many previous ALE meta-analyses (Laird et al., 2005b; Petacchi et al., 2005; Li et al., 2010; Kühn and Gallinat, 2013), we included coordinates resulting from fMRI as well as from PET data. We included data from PET and fMRI studies and other data analysis techniques despite the fact that they have different physiological bases and theoretical assumptions since both methods have been used to identify differences between the intrinsic brain function in patients compared with controls. The rationale was to provide an all-encompassing overview of attempts to identify resting-state abnormalities in tinnitus patients. Our search criteria yielded a total of 67 peer-reviewed published articles. Of the 67 studies, 26 studies were excluded as these studies were not about comparisons between tinnitus patients and healthy controls; nine articles were excluded since these were electroencephalography (EEG) or magnetoencephalography (MEG) studies; another four pulsatile tinnitus studies and four animal studies were excluded. Fifteen studies were further excluded since these did not report detailed peak coordinates over the whole brain. Finally, nine resting-state neuroimaging studies, six fMRI, two SPECT and one PET, were included in the ALE meta-analysis (Figure 1 and Table 1).
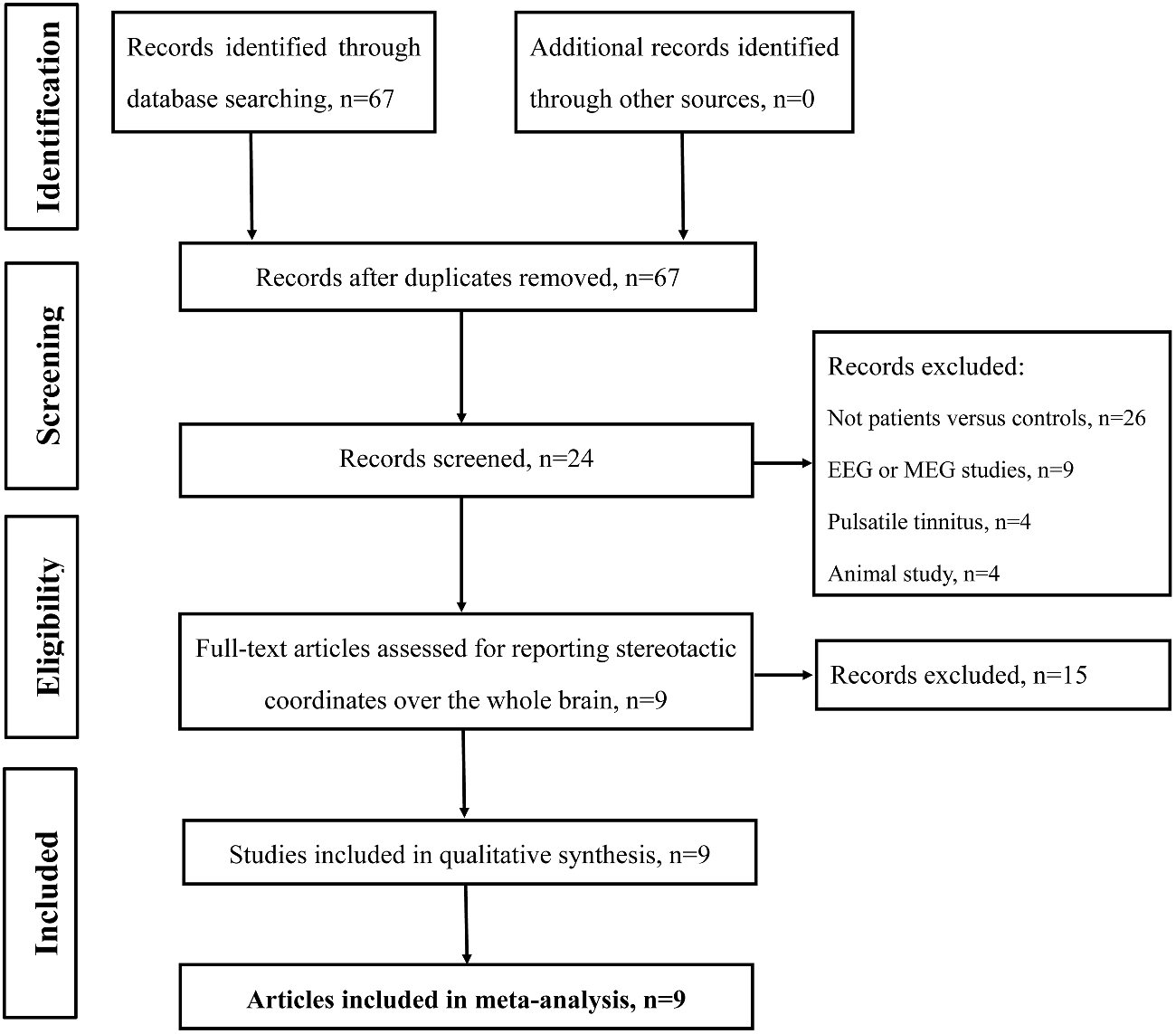
Figure 1. Flow diagram of the literature search. Flow diagram shows the results of the systematic search for the selected studies in this meta-analysis.
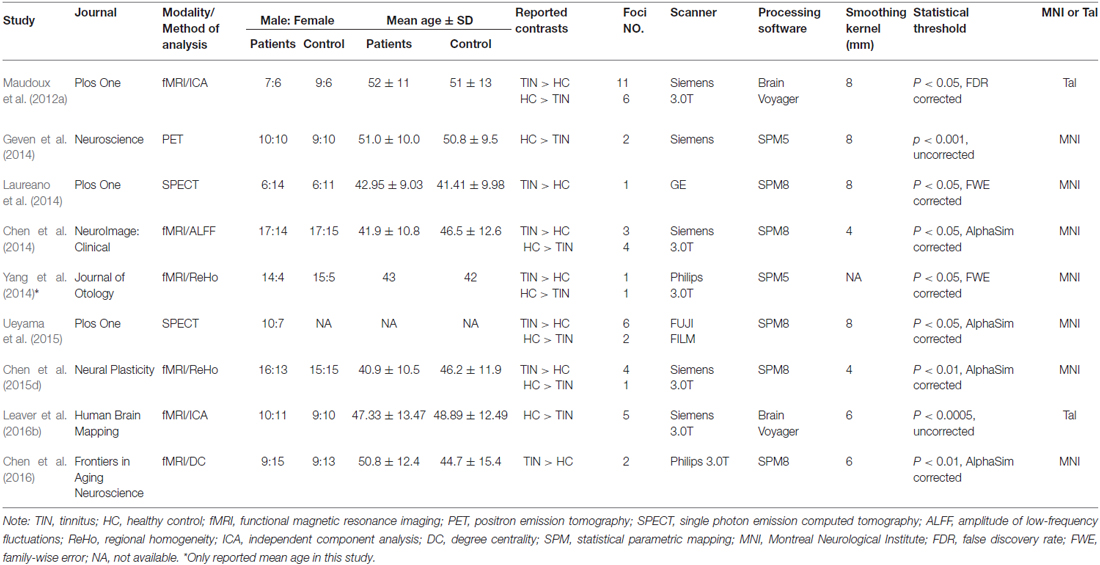
Table 1. List of all studies included in the meta-analysis: subjects’ demographic and clinical characteristics.
Two independent reviewers (CYC and FW) evaluated the methodology and the risk of bias of the eligible studies. First, they assessed the titles of the search results and retrieved the relevant articles. Second, the articles that remained eligible were assessed based on their abstract to determine whether any of the inclusion criteria were not met. The full text of all remaining articles was then assessed with a data extraction template, which was constructed for the purpose of organizing and extracting information from the included articles and excluding articles without peak values. The reviewers analyzed all articles in terms of patient selection and their comparable controls, blinding, diagnostic criteria and regression methods. Any disagreements were assessed by the third reviewer (FB). The demographic data were extracted from each article, including the first author’s name, year of publication, total patient number, sex distribution, mean patient age and range, statistic thresholds, hearing and psychological status.
Data Extraction
The x, y, and z peak activation coordinates of all eligible contrasts constituted the meta-analysis input. The data originally reported in Talairach spaces were converted to MNI coordinates (Lancaster et al., 2007). The data from MNI coordinates were texted and implemented in GingerALE 2.3.31, Research Imaging Institute of the University of Texas Health Science Center, San Antonio, TX, USA). Coordinates in each study were independently extracted by two authors (CYC and FW).
ALE Meta-Analysis
Ginger ALE software was used to analyze the resting-state brain activity between tinnitus patients and healthy controls. The reported loci of maximal anatomical differences were modeled as the peaks of three-dimensional Gaussian probability density functions defined by the full-width at half-maximum (FWHM), which was set according to a quantitative uncertainty model (Laird et al., 2005a; Eickhoff et al., 2009). ALE values were calculated on a voxel-by-voxel basis by measuring the union model activation maps modeled above. This revised analysis tested for convergence by studies (random effects) instead of foci (fixed effects). Following the method described by Turkeltaub et al. (2002, 2012), 1000 permutations were used to determine which tests were statistically significant and threshold determined for the resultant ALE map. A whole-brain histogram was computed in which the null hypothesis of uniformly distributed foci was rejected for voxels with an ALE value greater than the critical threshold, defined as the 100(1−α)th percentile of the permutation distribution, where α refers to the desired level of significance. The analyses were performed at a cluster forming threshold (reported with each p value and ALE thresholds in the results, ALE values greater than this threshold are statistically significant) computed using a p < 0.05, corrected for multiple comparisons using false-discovery rate (FDR; Genovese et al., 2002; Laird et al., 2005a). The volume of the minimum cluster threshold was set at 200 mm3. The coordinates of the weighted center were generated for each cluster. The resulting significant anatomical areas were labeled based on probabilistic cytoarchitectonic maps of the human brain using the SPM Anatomy Toolbox v2.1 (Eickhoff et al., 2005). Results were visualized with Mango software2, using the Colin brain template in the MNI space3.
Results
Using our inclusion/exclusion criteria, we identified nine eligible neuroimaging studies utilizing different methods, including SPECT (Laureano et al., 2014; Ueyama et al., 2015), PET (Geven et al., 2014), and fMRI (Maudoux et al., 2012a; Chen et al., 2014, 2015d, 2016; Leaver et al., 2016b). Figure 1 is a flow diagram showing the steps in the identification, exclusion and inclusion of the studies. The clinical and demographic data of the participants from all recruited studies are presented in Table 1. The subjects of patients and controls from each study are generally comparable by age, sex, and education. The hearing and psychological status are also shown in Table 2.
As illustrated in Figure 2, a total of 13 peak foci were reported in this meta-analysis. Compared with healthy controls, tinnitus patients showed increased resting-state neural activity bilaterally in the middle temporal gyrus (MTG), inferior frontal gyrus (IFG), parahippocampal gyrus, insula, cerebellar posterior lobe and right superior frontal gyrus (SFG). Moreover, decreased brain activity was also observed in the left cuneus and right thalamus. Table 3 displays the coordinates of cluster maxima.
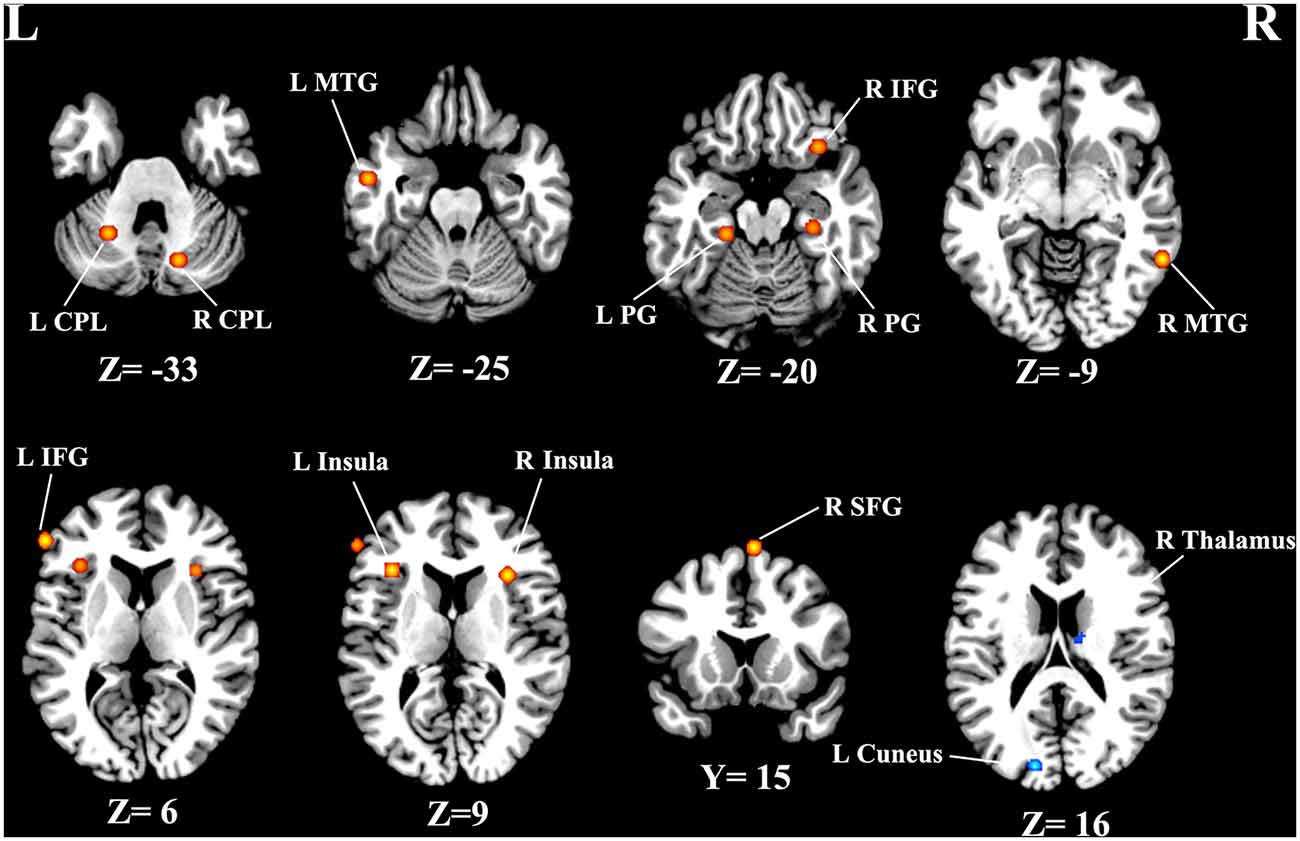
Figure 2. Resting-state brain activity alterations in chronic tinnitus patients compared with healthy controls. Results are from the activation likelihood estimation (ALE) software for meta-analyses. All activations are significant at p < 0.05 corrected for multiple comparisons using the false-discovery rate (FDR) correction.
Discussion
The current study is the first whole-brain meta-analysis exploring the resting-state brain abnormalities in chronic tinnitus patients compared to healthy controls. By analyzing nine neuroimaging studies, the meta-analysis identified consistent regions of aberrant neural activity mainly in the non-auditory brain regions, including the MTG, frontal cortex, parahippocampus, insula, cerebellum, cuneus, and thalamus, in different aspects of tinnitus. Surprisingly, the auditory cortex was not detected in this study. This may be due to the fact that most patients in these studies had little or mild hearing loss. These non-auditory areas are parts of separable subnetworks representing multiple clinical cognitive and emotional aspects of tinnitus; resting-state disruptions in these areas may provide new insights on the neuropathological mechanisms of this disorder.
Middle Temporal Gyrus
The MTG has been suggested to be involved in cognitive processes including language, semantic memory and multimodal sensory integration (Cabeza and Nyberg, 2000). A quantitative EEG study has demonstrated higher α2-band activity in the MTG in tinnitus patients with higher distress (Vanneste et al., 2010a). Voxel-based morphometry (VBM) analyses revealed that the gray matter increases in the MTG in tinnitus patients with hearing impairment (Boyen et al., 2013). Using resting-state fMRI, significantly increased spontaneous neural activity was observed in the right MTG in tinnitus patients (Chen et al., 2014). Moreover, Zhang et al. (2015) showed decreased functional connectivity between right MTG and left thalamus, which was negatively correlated with tinnitus severity. In addition, the MTG has been regarded as a key region of the DMN (Raichle et al., 2001). The DMN, consisting of nodes in the MTG, posterior cingulate/precuneus, angular gyrus and medial frontal gyrus, is most active at rest and shows reduced activity when a subject enters a task-based state involving attention or goal-directed behavior (Raichle et al., 2001; Mantini et al., 2007). As a condition involving the perception of a phantom auditory sensation, tinnitus might lead to dysfunction of the DMN. Previous fMRI studies found abnormal functional connectivity within the DMN associated with tinnitus distress (Burton et al., 2012; Maudoux et al., 2012b; Schmidt et al., 2013). Nevertheless, the source or type of aberrant neural activity within DMN regions in tinnitus remains unclear. Our results indicate that increased neuronal activity in the MTG may be responsible for disrupting the DMN in tinnitus patients.
Frontal Cortex
The frontal cortex, including the SFG and IFG, exhibited increased neural activity in tinnitus patients in the current study. Rauschecker et al. (2010) developed a model to demonstrate structural and functional differences in ventromedial prefrontal cortex that were associated with tinnitus subjective loudness, indicating that frontal cortex may contribute to certain perceptual features of tinnitus. Resting-state fMRI studies have pointed out that the abnormalities of the frontal cortex could act as a direct mechanism of tinnitus chronification (Burton et al., 2012; Schmidt et al., 2013; Chen et al., 2014, 2015c,d, 2016), which are confirmed by the current meta-analysis. Based on the previous fMRI studies, the SFG has been regarded as a major integrative hub of the tinnitus network architecture (Chen et al., 2016), which can receive and integrate all kinds of information from different parts of the brain from inside and outside the body. Besides, it can also timely organize efferent impulses to ensure the coordination of the central nervous system as a whole (Mathiak et al., 2007; Melloni et al., 2007). A possible explanation for our result was that the increased activity of the SFG might be due to feedback inhibition of an over active auditory network (Downar et al., 2000). Furthermore, the IFG serves as the core region of response inhibition and IFG activity might mirror the attempt to control the bottom-up attention allocation to the tinnitus percept in a top-down manner (Aron et al., 2014). In one hypothetical model, the fronto-insular cortex is part of a salience network that drives switching by a central executive control network important to maintaining and adjusting attention (Sridharan et al., 2008). In another model, the IFG and the insula act as executive control components in the attention system that regulates dorsal and VANs, which lack direct interconnections (Shulman et al., 2009). Taken together, we suggest that tinnitus distress, salience, or attentional focus is associated with increased resting-state activity in these brain subnetworks.
Parahippocampus
The parahippocampal area has been hypothesized to play a central role in memory recollection and transferring information from the hippocampus to the association areas, which might explain its involvement in the generation of simple auditory phantom percepts such as tinnitus (De Ridder et al., 2014a; Vanneste and De Ridder, 2016). EEG study suggested that tinnitus patients differed from healthy controls by increased delta and theta activity in the parahippocampus (Moazami-Goudarzi et al., 2010). Prior resting-state fMRI studies also provided further support linking tinnitus physiopathology with parahippocampal region involved in mnemonic network (Maudoux et al., 2012a; Chen et al., 2015b; Leaver et al., 2016b).
Insula
The increased response in the insula, mainly the anterior part, may be an indication of successful adaption to the tinnitus perception (van der Loo et al., 2011). On the basis of resting-state quantitative EEG, the insula has been implicated in tinnitus and specific tinnitus characteristics (van der Loo et al., 2011; Vanneste et al., 2011a,b). Greater synchrony of alpha activity was observed bilaterally in the anterior insula of patients with more severe tinnitus-related distress (Vanneste et al., 2010a). In addition, chronic tinnitus patients showed enhanced spontaneous neuronal activity and functional connectivity in bilateral anterior insula were revealed by resting-state fMRI (Burton et al., 2012; Chen et al., 2015d).
Furthermore, the insula is one of key nodes in the common brain circuit of both tinnitus and chronic pain (Rauschecker et al., 2015). Although there also exist important differences between the two disorders, the striking similarities and associations indicate that tinnitus and chronic pain share common neuropathological mechanisms. Now advances in neuroimaging have demonstrated that similar structures and functional systems are involved and probably play a central role in both disorders (Rauschecker et al., 2015). Significant loss of gray matter and compromised circuit function are detected in several specific regions, such as the ventromedial prefrontal cortex, nucleus accumbens and insula (De Ridder et al., 2011; Rauschecker et al., 2015). These areas act as a central gatekeeping system for perceptual sensations, which determines the affective value of sensory stimuli and modulates information flow in the brain (Rauschecker et al., 2015). Tinnitus and chronic pain occur when this system is compromised.
Although the EEG has been used to determine resting-state long-range functional coupling in tinnitus, it has many differences from the resting-state fMRI (Vanneste et al., 2010a,b). EEG does not offer the spatial resolution of fMRI, but offer the advantage of being quiet and not influencing the resting-state network of tinnitus. Thus these two techniques may measure different aspects of spontaneous neuronal activity (Tagliazucchi et al., 2012). Britz et al. (2010) extracted four resting-state networks from the EEG data that were the equivalent of stereotypical fMRI networks dedicated to auditory, attetional, visual and self-referential processing, but DMN was not detected by EEG in Britz’s study. Other studies have correlated the DMN with beta-2 (Laufs et al., 2003) or with delta (Mantini et al., 2007) spectral bands of EEG. Therefore, a direct comparison of EEG and resting-state fMRI is complicated by the fact that similar EEG power bands may be correlated with varying fMRI-generated spatial maps and a single resting-state network may be linked with different EEG spectral patterns (Laufs et al., 2008; Musso et al., 2010).
Cerebellum
Furthermore, although the cerebellum is primarily involved in motor actions and control, several cerebellar regions such as the paraflocculus and vermis receive inputs from auditory centers (Petacchi et al., 2005). Brozoski et al. (2007) observed increased activity in the parafloccular lobe of the cerebellum in animals with tinnitus confirmed and suggested that the cerebellum acts as a gating control mechanism comparing the afferent input from the cochlea with descending signals from the auditory cortex (Bauer et al., 2013; Chen et al., 2015b). Consistent with this view, hyperactivity in the auditory cortex and increased functional connectivity between the auditory cortex and cerebellum were observed in rats with salicylate-induced tinnitus (Chen et al., 2015a). If this cerebellar-tinnitus gating hypothesis is correct, then inactivating the cerebellum could possibly suppress the sound perception of tinnitus. Based on these findings, we suggest that cerebellum may play a pivotal role of gating control in tinnitus. Furthermore, using resting-state fMRI, chronic tinnitus patients exhibited enhanced functional connectivity in the cerebellar hemisphere that was associated with tinnitus distress (Maudoux et al., 2012a; Ueyama et al., 2013), indicating the involvement of cerebellum in auditory system.
Cuneus
Our meta-analysis found decreased brain activity in the cuneus of tinnitus patients. We speculate that the connections between auditory and visual regions make it possible to alter the brain activity in the visual areas (Kaltenbach et al., 2004; Cate et al., 2009). This is consistent with prior fMRI studies showing negative correlations of functional connectivity between auditory and visual resting-state subnetworks in tinnitus patients (Burton et al., 2012; Maudoux et al., 2012b). One possibility is that the phantom sounds might act to decrease spontaneous activity in visual areas because of the salience of the tinnitus perception (Chen et al., 2014, 2015d). Thus, tinnitus may be regarded as the consequence of multisensory interactions between auditory and visual regions.
Thalamus
The thalamus, which regulates the flow of sensory information to and from the auditory cortex, has been thought to play a key role in tinnitus (Richardson et al., 2012). Llinás et al. (1999) hypothesized that tinnitus results from thalamocortical dysrhythmias triggered by peripheral damage. Prior MRI studies have identified structural and functional abnormalities involved in thalamocortical network of tinnitus (Mühlau et al., 2006; Rauschecker et al., 2010; Benson et al., 2014; Chen et al., 2014; Lanting et al., 2014; Zhang et al., 2015). Consistent with our results, Zhang et al. (2015) showed decreased functional connectivity between the thalamus and auditory cortical areas in tinnitus, which may be a reflection of disrupted thalamic gating mechanism (Rauschecker et al., 2010). The thalamus is regarded as the center of ascending noise canceling system, and thus if it is dysfunctional, noise canceling is no longer possible and the subject may perceive tinnitus (Rauschecker et al., 2010). However, the mechanisms responsible for these functional changes are unknown, but could involve aberrant inhibition (Richardson et al., 2012).
Limitations
Several inevitable limitations should be noted in our study. First, the heterogeneity of the included studies could affect the current results. In particular, hearing loss is always a complicating factor in structural and functional studies of tinnitus. Melcher et al. (2013) compared tinnitus patients with controls who were matched for hearing thresholds in the standard clinical frequencies and found that gray matter changes were not related to tinnitus but instead negatively correlated with hearing thresholds at frequencies above 8 kHz. Therefore, a more complete characterization of hearing loss is desirable. Moreover, tinnitus can become a significant psychological problem and comorbid symptoms such as depression and anxiety are potential confounds on the observed brain activity in tinnitus patients (Leaver et al., 2016a). It is necessary to characterize the psychological health problems as covariates in the analyses. The problems of differential tinnitus variables on structural and functional changes in neural activity can be solved using correlational analyses of large samples (Schecklmann et al., 2013) and comparisons of different tinnitus subtypes (Vanneste and De Ridder, 2012). Second, voxel-wise meta-analytic approach like ALE provides excellent control of false positive results, but it is more difficult to avoid false negatives (Radua et al., 2012). Voxel-wise meta-analysis is based on the pooling of peak stereotactic coordinates rather than on raw statistical brain maps from the original studies, which could give rise to less accurate results (Radua et al., 2012). Furthermore, fMRI produces aversive scanner noise which has been shown to interfere with auditory processing in the human brain at the physiological and psychological level (Perrachione and Ghosh, 2013). The concept of resting state is somewhat problematic in tinnitus studies because the auditory pathway is likely to be activated by scanner noise which is nearly impossible to completely eliminate even with ear plugs or active noise reduction (Logothetis et al., 2009). Therefore, this limitation should be taken into consideration when interpreting the resting-state fMRI data in auditory system-related researches. Finally, the number of included neuroimaging studies is relatively small. Further explorations are needed to perform the subgroup analyses or meta-regression analyses for the control of confounding factors that might influence resting-state brain function in tinnitus, such as the imaging methodology and data analysis method. Most importantly, we should establish standard protocols for resting-state fMRI researches to minimize heterogeneity on the experimental side.
Conclusion
Using the ALE-based meta-analysis, our study demonstrated abnormal resting-state neural activity mainly in the non-auditory brain areas, including the MTG, frontal cortex, parahippocampus, insula, cerebellum, cuneus and thalamus. In accordance to the hypothesis, these results show that there exist several important brain hubs in specific tinnitus network encompassing DMN, attentional, mnemonic, salience, visual and thalamocortical subnetworks. Aberrant resting-state brain patterns in tinnitus-related networks may help enhance our understanding of the neuropathological mechanisms underlying chronic subjective tinnitus. Nonetheless, a theoretical pathophysiological framework capable of explaining all these aspects in one model is still highly required. There is thus hope that a single cure can be found that would target a common mechanism.
Author Contributions
Y-CC and FW designed the experiment, reviewed the literatures, performed the analysis and wrote the manuscript. JW, FB and WX reviewed the literatures. J-PG and XY contributed to the discussion and manuscript revision.
Conflict of Interest Statement
The authors declare that the research was conducted in the absence of any commercial or financial relationships that could be construed as a potential conflict of interest.
The reviewer MM and handling Editor declared their shared affiliation, and the handling Editor states that the process nevertheless met the standards of a fair and objective review.
Acknowledgments
The authors thank Prof. Richard Salvi, Center for Hearing and Deafness, University at Buffalo, Buffalo, NY, USA, for his contributions to the revision of the manuscript. This work was supported by a grant from the Natural Science Foundation of Jiangsu Higher Education Institutions (No. 16KJB320001) and National Natural Science Foundation of China (Nos. 81601477, 81600638).
Footnotes
References
Aron, A. R., Robbins, T. W., and Poldrack, R. A. (2014). Inhibition and the right inferior frontal cortex: one decade on. Trends Cogn. Sci. 18, 177–185. doi: 10.1016/j.tics.2013.12.003
Bauer, C. A., Kurt, W., Sybert, L. T., and Brozoski, T. J. (2013). The cerebellum as a novel tinnitus generator. Hear. Res. 295, 130–139. doi: 10.1016/j.heares.2012.03.009
Benson, R. R., Gattu, R., and Cacace, A. T. (2014). Left hemisphere fractional anisotropy increase in noise-induced tinnitus: a diffusion tensor imaging (DTI) study of white matter tracts in the brain. Hear. Res. 309, 8–16. doi: 10.1016/j.heares.2013.10.005
Boyen, K., Langers, D. R., de Kleine, E., and van Dijk, P. (2013). Gray matter in the brain: differences associated with tinnitus and hearing loss. Hear. Res. 295, 67–78. doi: 10.1016/j.heares.2012.02.010
Britz, J., Van De Ville, D., and Michel, C. M. (2010). BOLD correlates of EEG topography reveal rapid resting-state network dynamics. Neuroimage 52, 1162–1170. doi: 10.1016/j.neuroimage.2010.02.052
Brozoski, T. J., Ciobanu, L., and Bauer, C. A. (2007). Central neural activity in rats with tinnitus evaluated with manganese-enhanced magnetic resonance imaging (MEMRI). Hear. Res. 228, 168–179. doi: 10.1016/j.heares.2007.02.003
Burton, H., Wineland, A., Bhattacharya, M., Nicklaus, J., Garcia, K. S., and Piccirillo, J. F. (2012). Altered networks in bothersome tinnitus: a functional connectivity study. BMC Neurosci. 13:3. doi: 10.1186/1471-2202-13-3
Cabeza, R., and Nyberg, L. (2000). Imaging cognition II: an empirical review of 275 PET and fMRI studies. J. Cogn. Neurosci. 12, 1–47. doi: 10.1162/08989290051137585
Cate, A. D., Herron, T. J., Yund, E. W., Stecker, G. C., Rinne, T., Kang, X., et al. (2009). Auditory attention activates peripheral visual cortex. PLoS One 4:e4645. doi: 10.1371/journal.pone.0004645
Chen, Y.-C., Feng, Y., Xu, J.-J., Mao, C.-N., Xia, W., Ren, J., et al. (2016). Disrupted brain functional network architecture in chronic tinnitus patients. Front. Aging Neurosci. 8:174. doi: 10.3389/fnagi.2016.00174
Chen, Y.-C., Li, X., Liu, L., Wang, J., Lu, C.-Q., Yang, M., et al. (2015a). Tinnitus and hyperacusis involve hyperactivity and enhanced connectivity in auditory-limbic-arousal-cerebellar network. Elife 4:e06576. doi: 10.7554/eLife.06576
Chen, Y.-C., Xia, W., Feng, Y., Li, X., Zhang, J., Feng, X., et al. (2015b). Altered interhemispheric functional coordination in chronic tinnitus patients. Biomed Res. Int. 2015:345647. doi: 10.1155/2015/345647
Chen, Y.-C., Xia, W., Luo, B., Muthaiah, V. P., Xiong, Z., Zhang, J., et al. (2015c). Frequency-specific alternations in the amplitude of low-frequency fluctuations in chronic tinnitus. Front. Neural Circuits 9:67. doi: 10.3389/fncir.2015.00067
Chen, Y.-C., Zhang, J., Li, X.-W., Xia, W., Feng, X., Qian, C., et al. (2015d). Altered intra-and interregional synchronization in resting-state cerebral networks associated with chronic tinnitus. Neural Plast. 2015:475382. doi: 10.1155/2015/475382
Chen, Y.-C., Zhang, J., Li, X.-W., Xia, W., Feng, X., Gao, B., et al. (2014). Aberrant spontaneous brain activity in chronic tinnitus patients revealed by resting-state functional MRI. Neuroimage Clin. 6, 222–228. doi: 10.1016/j.nicl.2014.09.011
Davies, J., Gander, P., Andrews, M., and Hall, D. (2014). Auditory network connectivity in tinnitus patients: a resting-state fMRI study. Int. J. Audiol. 53, 192–198. doi: 10.3109/14992027.2013.846482
Downar, J., Crawley, A. P., Mikulis, D. J., and Davis, K. D. (2000). A multimodal cortical network for the detection of changes in the sensory environment. Nat. Neurosci. 3, 277–283. doi: 10.1038/72991
Eickhoff, S. B., Laird, A. R., Grefkes, C., Wang, L. E., Zilles, K., and Fox, P. T. (2009). Coordinate–based activation likelihood estimation meta–analysis of neuroimaging data: a random–effects approach based on empirical estimates of spatial uncertainty. Hum. Brain Mapp. 30, 2907–2926. doi: 10.1002/hbm.20718
Eickhoff, S. B., Stephan, K. E., Mohlberg, H., Grefkes, C., Fink, G. R., Amunts, K., et al. (2005). A new SPM toolbox for combining probabilistic cytoarchitectonic maps and functional imaging data. Neuroimage 25, 1325–1335. doi: 10.1016/j.neuroimage.2004.12.034
Ellison-Wright, I., and Bullmore, E. (2010). Anatomy of bipolar disorder and schizophrenia: a meta-analysis. Schizophr. Res. 117, 1–12. doi: 10.1016/j.schres.2009.12.022
Genovese, C. R., Lazar, N. A., and Nichols, T. (2002). Thresholding of statistical maps in functional neuroimaging using the false discovery rate. Neuroimage 15, 870–878. doi: 10.1006/nimg.2001.1037
Geven, L., de Kleine, E., Willemsen, A., and van Dijk, P. (2014). Asymmetry in primary auditory cortex activity in tinnitus patients and controls. Neuroscience 256, 117–125. doi: 10.1016/j.neuroscience.2013.10.015
Henry, J. A., Roberts, L. E., Caspary, D. M., Theodoroff, S. M., and Salvi, R. J. (2014). Underlying mechanisms of tinnitus: review and clinical implications. J. Am. Acad. Audiol. 25, 5–22; quiz 126. doi: 10.3766/jaaa.25.1.2
Hinkley, L. B., Mizuiri, D., Hong, O., Nagarajan, S. S., and Cheung, S. W. (2015). Increased striatal functional connectivity with auditory cortex in tinnitus. Front. Hum. Neurosci. 9:568. doi: 10.3389/fnhum.2015.00568
Husain, F. T., and Schmidt, S. A. (2014). Using resting state functional connectivity to unravel networks of tinnitus. Hear. Res. 307, 153–162. doi: 10.1016/j.heares.2013.07.010
Jastreboff, P. J. (1990). Phantom auditory perception (tinnitus): mechanisms of generation and perception. Neurosci. Res. 8, 221–254. doi: 10.1016/0168-0102(90)90031-9
Jastreboff, P. J., Hazell, J. W., and Graham, R. L. (1994). Neurophysiological model of tinnitus: dependence of the minimal masking level on treatment outcome. Hear. Res. 80, 216–232. doi: 10.1016/0378-5955(94)90113-9
Kaltenbach, J. A., Zacharek, M. A., Zhang, J., and Frederick, S. (2004). Activity in the dorsal cochlear nucleus of hamsters previously tested for tinnitus following intense tone exposure. Neurosci. Lett. 355, 121–125. doi: 10.1016/j.neulet.2003.10.038
Kaltenbach, J. A., Zhang, J., and Finlayson, P. (2005). Tinnitus as a plastic phenomenon and its possible neural underpinnings in the dorsal cochlear nucleus. Hear. Res. 206, 200–226. doi: 10.1016/j.heares.2005.02.013
Kim, J.-Y., Kim, Y.-H., Lee, S., Seo, J.-H., Song, H.-J., Cho, J. H., et al. (2012). Alteration of functional connectivity in tinnitus brain revealed by resting-state fMRI?: a pilot study. Int. J. Audiol. 51, 413–417. doi: 10.3109/14992027.2011.652677
Kühn, S., and Gallinat, J. (2013). Resting-state brain activity in schizophrenia and major depression: a quantitative meta-analysis. Schizophr. Bull. 39, 358–365. doi: 10.1093/schbul/sbr151
Laird, A. R., Fox, P. M., Price, C. J., Glahn, D. C., Uecker, A. M., Lancaster, J. L., et al. (2005a). ALE meta-analysis: controlling the false discovery rate and performing statistical contrasts. Hum. Brain Mapp. 25, 155–164. doi: 10.1002/hbm.20136
Laird, A. R., McMillan, K. M., Lancaster, J. L., Kochunov, P., Turkeltaub, P. E., Pardo, J. V., et al. (2005b). A comparison of label-based review and ALE meta-analysis in the Stroop task. Hum. Brain Mapp. 25, 6–21. doi: 10.1002/hbm.20129
Lancaster, J. L., Tordesillas-Gutiérrez, D., Martinez, M., Salinas, F., Evans, A., Zilles, K., et al. (2007). Bias between MNI and Talairach coordinates analyzed using the ICBM-152 brain template. Hum. Brain Mapp. 28, 1194–1205. doi: 10.1002/hbm.20345
Landgrebe, M., Azevedo, A., Baguley, D., Bauer, C., Cacace, A., Coelho, C., et al. (2012). Methodological aspects of clinical trials in tinnitus: a proposal for an international standard. J. Psychosom. Res. 73, 112–121. doi: 10.1016/j.jpsychores.2012.05.002
Lanting, C. P., de Kleine, E., Langers, D. R., and van Dijk, P. (2014). Unilateral tinnitus: changes in connectivity and response lateralization measured with FMRI. PLoS One 9:e110704. doi: 10.1371/journal.pone.0110704
Laufs, H., Daunizeau, J., Carmichael, D. W., and Kleinschmidt, A. (2008). Recent advances in recording electrophysiological data simultaneously with magnetic resonance imaging. Neuroimage 40, 515–528. doi: 10.1016/j.neuroimage.2007.11.039
Laufs, H., Krakow, K., Sterzer, P., Eger, E., Beyerle, A., Salek-Haddadi, A., et al. (2003). Electroencephalographic signatures of attentional and cognitive default modes in spontaneous brain activity fluctuations at rest. Proc. Natl. Acad. Sci. U S A 100, 11053–11058. doi: 10.1073/pnas.1831638100
Laureano, M. R., Onishi, E. T., Bressan, R. A., Castiglioni, M. L. V., Batista, I. R., Reis, M. A., et al. (2014). Memory networks in tinnitus: a functional brain image study. PLoS One 9:e87839. doi: 10.1371/journal.pone.0087839
Leaver, A. M., Renier, L., Chevillet, M. A., Morgan, S., Kim, H. J., and Rauschecker, J. P. (2011). Dysregulation of limbic and auditory networks in tinnitus. Neuron 69, 33–43. doi: 10.1016/j.neuron.2010.12.002
Leaver, A. M., Seydell-Greenwald, A., and Rauschecker, J. P. (2016a). Auditory-limbic interactions in chronic tinnitus: challenges for neuroimaging research. Hear. Res. 334, 49–57. doi: 10.1016/j.heares.2015.08.005
Leaver, A. M., Turesky, T. K., Seydell-Greenwald, A., Morgan, S., Kim, H. J., and Rauschecker, J. P. (2016b). Intrinsic network activity in tinnitus investigated using functional MRI. Hum. Brain Mapp. 37, 2717–2735. doi: 10.1002/hbm.23204
Li, H., Chan, R. C., McAlonan, G. M., and Gong, Q. Y. (2010). Facial emotion processing in schizophrenia: a meta-analysis of functional neuroimaging data. Schizophr. Bull. 36, 1029–1039. doi: 10.1093/schbul/sbn190
Li, J., Zhang, Z., and Shang, H. (2012). A meta-analysis of voxel-based morphometry studies on unilateral refractory temporal lobe epilepsy. Epilepsy Res. 98, 97–103. doi: 10.1016/j.eplepsyres.2011.10.002
Llinás, R. R., Ribary, U., Jeanmonod, D., Kronberg, E., and Mitra, P. P. (1999). Thalamocortical dysrhythmia: a neurological and neuropsychiatric syndrome characterized by magnetoencephalography. Proc. Natl. Acad. Sci. U S A 96, 15222–15227. doi: 10.1073/pnas.96.26.15222
Lockwood, A. H., Salvi, R. J., Coad, M. L., Towsley, M. L., Wack, D. S., and Murphy, B. W. (1998). The functional neuroanatomy of tinnitus: evidence for limbic system links and neural plasticity. Neurology 50, 114–120. doi: 10.1212/WNL.50.1.114
Logothetis, N. K., Murayama, Y., Augath, M., Steffen, T., Werner, J., and Oeltermann, A. (2009). How not to study spontaneous activity. Neuroimage 45, 1080–1089. doi: 10.1016/j.neuroimage.2009.01.010
van der Loo, E., Congedo, M., Vanneste, S., Van De Heyning, P., and De Ridder, D. (2011). Insular lateralization in tinnitus distress. Auton. Neurosci. 165, 191–194. doi: 10.1016/j.autneu.2011.06.007
Mantini, D., Perrucci, M. G., Del Gratta, C., Romani, G. L., and Corbetta, M. (2007). Electrophysiological signatures of resting state networks in the human brain. Proc. Natl. Acad. Sci. U S A 104, 13170–13175. doi: 10.1073/pnas.0700668104
Mathiak, K., Menning, H., Hertrich, I., Mathiak, K. A., Zvyagintsev, M., and Ackermann, H. (2007). Who is telling what from where? A functional magnetic resonance imaging study. Neuroreport 18, 405–409. doi: 10.1097/WNR.0b013e328013cec4
Maudoux, A., Lefebvre, P., Cabay, J.-E., Demertzi, A., Vanhaudenhuyse, A., Laureys, S., et al. (2012a). Auditory resting-state network connectivity in tinnitus: a functional MRI study. PLoS One 7:e36222. doi: 10.1371/journal.pone.0036222
Maudoux, A., Lefebvre, P., Cabay, J. E., Demertzi, A., Vanhaudenhuyse, A., Laureys, S., et al. (2012b). Connectivity graph analysis of the auditory resting state network in tinnitus. Brain Res. 1485, 10–21. doi: 10.1016/j.brainres.2012.05.006
Melcher, J. R., Knudson, I. M., and Levine, R. A. (2013). Subcallosal brain structure: correlation with hearing threshold at supra-clinical frequencies ( >8 kHz), but not with tinnitus. Hear. Res. 295, 79–86. doi: 10.1016/j.heares.2012.03.013
Melloni, L., Molina, C., Pena, M., Torres, D., Singer, W., and Rodriguez, E. (2007). Synchronization of neural activity across cortical areas correlates with conscious perception. J. Neurosci. 27, 2858–2865. doi: 10.1523/JNEUROSCI.4623-06.2007
Minami, S. B., Oishi, N., Watabe, T., Uno, K., Kaga, K., and Ogawa, K. (2015). Auditory resting-state functional connectivity in tinnitus and modulation with transcranial direct current stimulation. Acta Otolaryngol. 135, 1286–1292. doi: 10.3109/00016489.2015.1068952
Mirz, F., Gjedde, A., Ishizu, K., and Pedersen, C. B. (2000). Cortical networks subserving the perception of tinnitus–a PET study. Acta Otolaryngol. Suppl. 543, 241–243. doi: 10.1080/000164800454503
Moazami-Goudarzi, M., Michels, L., Weisz, N., and Jeanmonod, D. (2010). Temporo-insular enhancement of EEG low and high frequencies in patients with chronic tinnitus. QEEG study of chronic tinnitus patients. BMC Neurosci. 11:40. doi: 10.1186/1471-2202-11-40
Mühlau, M., Rauschecker, J. P., Oestreicher, E., Gaser, C., Röttinger, M., Wohlschlager, A. M., et al. (2006). Structural brain changes in tinnitus. Cereb. Cortex 16, 1283–1288. doi: 10.1093/cercor/bhj070
Musso, F., Brinkmeyer, J., Mobascher, A., Warbrick, T., and Winterer, G. (2010). Spontaneous brain activity and EEG microstates. A novel EEG/fMRI analysis approach to explore resting-state networks. Neuroimage 52, 1149–1161. doi: 10.1016/j.neuroimage.2010.01.093
Perrachione, T. K., and Ghosh, S. S. (2013). Optimized design and analysis of sparse-sampling FMRI experiments. Front. Neurosci. 7:55. doi: 10.3389/fnins.2013.00055
Petacchi, A., Laird, A. R., Fox, P. T., and Bower, J. M. (2005). Cerebellum and auditory function: an ALE meta-analysis of functional neuroimaging studies. Hum. Brain Mapp. 25, 118–128. doi: 10.1002/hbm.20137
Radua, J., Mataix-Cols, D., Phillips, M., El-Hage, W., Kronhaus, D., Cardoner, N., et al. (2012). A new meta-analytic method for neuroimaging studies that combines reported peak coordinates and statistical parametric maps. Eur. Psychiatry 27, 605–611. doi: 10.1016/j.eurpsy.2011.04.001
Raichle, M. E., MacLeod, A. M., Snyder, A. Z., Powers, W. J., Gusnard, D. A., and Shulman, G. L. (2001). A default mode of brain function. Proc. Natl. Acad. Sci. U S A 98, 676–682. doi: 10.1073/pnas.98.2.676
Rauschecker, J. P., Leaver, A. M., and Mühlau, M. (2010). Tuning out the noise: limbic-auditory interactions in tinnitus. Neuron 66, 819–826. doi: 10.1016/j.neuron.2010.04.032
Rauschecker, J. P., May, E. S., Maudoux, A., and Ploner, M. (2015). Frontostriatal gating of tinnitus and chronic pain. Trends Cogn. Sci. 19, 567–578. doi: 10.1016/j.tics.2015.08.002
Richardson, B. D., Brozoski, T. J., Ling, L. L., and Caspary, D. M. (2012). Targeting inhibitory neurotransmission in tinnitus. Brain Res. 1485, 77–87. doi: 10.1016/j.brainres.2012.02.014
De Ridder, D., Elgoyhen, A. B., Romo, R., and Langguth, B. (2011). Phantom percepts: tinnitus and pain as persisting aversive memory networks. Proc. Natl. Acad. Sci. U S A 108, 8075–8080. doi: 10.1073/pnas.1018466108
De Ridder, D., Vanneste, S., and Freeman, W. (2014a). The Bayesian brain: phantom percepts resolve sensory uncertainty. Neurosci. Biobehav. Rev. 44, 4–15. doi: 10.1016/j.neubiorev.2012.04.001
De Ridder, D., Vanneste, S., Weisz, N., Londero, A., Schlee, W., Elgoyhen, A. B., et al. (2014b). An integrative model of auditory phantom perception: tinnitus as a unified percept of interacting separable subnetworks. Neurosci. Biobehav. Rev. 44, 16–32. doi: 10.1016/j.neubiorev.2013.03.021
Schaette, R., and McAlpine, D. (2011). Tinnitus with a normal audiogram: physiological evidence for hidden hearing loss and computational model. J. Neurosci. 31, 13452–13457. doi: 10.1523/JNEUROSCI.2156-11.2011
Schecklmann, M., Landgrebe, M., Langguth, B., and TRI Database Study Group. (2014). Phenotypic characteristics of hyperacusis in tinnitus. PLoS One 9:e86944. doi: 10.1371/journal.pone.0086944
Schecklmann, M., Landgrebe, M., Poeppl, T. B., Kreuzer, P., Männer, P., Marienhagen, J., et al. (2013). Neural correlates of tinnitus duration and distress: a positron emission tomography study. Hum. Brain Mapp. 34, 233–240. doi: 10.1002/hbm.21426
Schmidt, S. A., Akrofi, K., Carpenter-Thompson, J. R., and Husain, F. T. (2013). Default mode, dorsal attention and auditory resting state networks exhibit differential functional connectivity in tinnitus and hearing loss. PLoS One 8:e76488. doi: 10.1371/journal.pone.0076488
Sedley, W., Friston, K. J., Gander, P. E., Kumar, S., and Griffiths, T. D. (2016). An integrative tinnitus model based on sensory precision. Trends Neurosci. 39, 799–812. doi: 10.1016/j.tins.2016.10.004
Seki, S., and Eggermont, J. J. (2003). Changes in spontaneous firing rate and neural synchrony in cat primary auditory cortex after localized tone-induced hearing loss. Hear. Res. 180, 28–38. doi: 10.1016/s0378-5955(03)00074-1
Shao, N., Yang, J., and Shang, H. (2015). Voxelwise meta-analysis of gray matter anomalies in Parkinson variant of multiple system atrophy and Parkinson’s disease using anatomic likelihood estimation. Neurosci. Lett. 587, 79–86. doi: 10.1016/j.neulet.2014.12.007
Shargorodsky, J., Curhan, G. C., and Farwell, W. R. (2010). Prevalence and characteristics of tinnitus among US adults. Am. J. Med. 123, 711–718. doi: 10.1016/j.amjmed.2010.02.015
Shulman, G. L., Astafiev, S. V., Franke, D., Pope, D. L., Snyder, A. Z., McAvoy, M. P., et al. (2009). Interaction of stimulus-driven reorienting and expectation in ventral and dorsal frontoparietal and basal ganglia-cortical networks. J. Neurosci. 29, 4392–4407. doi: 10.1523/JNEUROSCI.5609-08.2009
Song, J. J., De Ridder, D., Van de Heyning, P., and Vanneste, S. (2012). Mapping tinnitus-related brain activation: an activation-likelihood estimation metaanalysis of PET studies. J. Nucl. Med. 53, 1550–1557. doi: 10.2967/jnumed.112.102939
Sridharan, D., Levitin, D. J., and Menon, V. (2008). A critical role for the right fronto-insular cortex in switching between central-executive and default-mode networks. Proc. Natl. Acad. Sci. U S A 105, 12569–12574. doi: 10.1073/pnas.0800005105
Stroup, D. F., Berlin, J. A., Morton, S. C., Olkin, I., Williamson, G. D., Rennie, D., et al. (2000). Meta-analysis of observational studies in epidemiology: a proposal for reporting. JAMA 283, 2008–2012. doi: 10.1001/jama.283.15.2008
Tagliazucchi, E., von Wegner, F., Morzelewski, A., Brodbeck, V., and Laufs, H. (2012). Dynamic BOLD functional connectivity in humans and its electrophysiological correlates. Front. Hum. Neurosci. 6:339. doi: 10.3389/fnhum.2012.00339
Turkeltaub, P. E., Eden, G. F., Jones, K. M., and Zeffiro, T. A. (2002). Meta-analysis of the functional neuroanatomy of single-word reading: method and validation. Neuroimage 16, 765–780. doi: 10.1006/nimg.2002.1131
Turkeltaub, P. E., Eickhoff, S. B., Laird, A. R., Fox, M., Wiener, M., and Fox, P. (2012). Minimizing within-experiment and within-group effects in Activation Likelihood Estimation meta-analyses. Hum. Brain Mapp. 33, 1–13. doi: 10.1002/hbm.21186
Ueyama, T., Donishi, T., Ukai, S., Ikeda, Y., Hotomi, M., Yamanaka, N., et al. (2013). Brain regions responsible for tinnitus distress and loudness: a resting-state fMRI study. PLoS One 8:e67778. doi: 10.1371/journal.pone.0067778
Ueyama, T., Donishi, T., Ukai, S., Yamamoto, Y., Ishida, T., Tamagawa, S., et al. (2015). Alterations of regional cerebral blood flow in tinnitus patients as assessed using single-photon emission computed tomography. PLoS One 10:e0137291. doi: 10.1371/journal.pone.0137291
Vanneste, S., and De Ridder, D. (2012). The auditory and non-auditory brain areas involved in tinnitus. An emergent property of multiple parallel overlapping subnetworks. Front. Syst. Neurosci. 6:31. doi: 10.3389/fnsys.2012.00031
Vanneste, S., and De Ridder, D. (2016). Deafferentation-based pathophysiological differences in phantom sound: tinnitus with and without hearing loss. Neuroimage 129, 80–94. doi: 10.1016/j.neuroimage.2015.12.002
Vanneste, S., Plazier, M., der Loo, E., de Heyning, P. V., Congedo, M., and De Ridder, D. (2010a). The neural correlates of tinnitus-related distress. Neuroimage 52, 470–480. doi: 10.1016/j.neuroimage.2010.04.029
Vanneste, S., Plazier, M., van der Loo, E., Van de Heyning, P., and De Ridder, D. (2010b). The differences in brain activity between narrow band noise and pure tone tinnitus. PLoS One 5:e13618. doi: 10.1371/journal.pone.0013618
Vanneste, S., Plazier, M., van der Loo, E., Van de Heyning, P., and De Ridder, D. (2011a). The difference between uni- and bilateral auditory phantom percept. Clin. Neurophysiol. 122, 578–587. doi: 10.1016/j.clinph.2010.07.022
Vanneste, S., van de Heyning, P., and De Ridder, D. (2011b). The neural network of phantom sound changes over time: a comparison between recent-onset and chronic tinnitus patients. Eur. J. Neurosci. 34, 718–731. doi: 10.1111/j.1460-9568.2011.07793.x
Wager, T. D., Jonides, J., and Reading, S. (2004). Neuroimaging studies of shifting attention: a meta-analysis. Neuroimage 22, 1679–1693. doi: 10.1016/j.neuroimage.2004.03.052
Weng, H.-H., Chen, C.-F., Tsai, Y.-H., Wu, C.-Y., Lee, M., Lin, Y.-C., et al. (2015). Gray matter atrophy in narcolepsy: an activation likelihood estimation meta-analysis. Neurosci. Biobehav. Rev. 59, 53–63. doi: 10.1016/j.neubiorev.2015.03.009
Wineland, A. M., Burton, H., and Piccirillo, J. (2012). Functional connectivity networks in nonbothersome tinnitus. Otolaryngol. Head Neck Surg. 147, 900–906. doi: 10.1177/0194599812451414
Yang, H., Zheng, Y., Ou, Y., and Huang, X. (2014). Regional homogeneity on resting state fMRI in patients with tinnitus. J. Otol. 9, 173–178. doi: 10.1016/j.joto.2014.10.001
Keywords: tinnitus, neuroimaging, meta-analysis, resting-state fMRI, brain networks
Citation: Chen Y-C, Wang F, Wang J, Bo F, Xia W, Gu J-P and Yin X (2017) Resting-State Brain Abnormalities in Chronic Subjective Tinnitus: A Meta-Analysis. Front. Hum. Neurosci. 11:22. doi: 10.3389/fnhum.2017.00022
Received: 02 September 2016; Accepted: 11 January 2017;
Published: 24 January 2017.
Edited by:
Tobias Kleinjung, University of Zurich, SwitzerlandReviewed by:
Martin Meyer, University of Zurich, SwitzerlandJae-Jin Song, Seoul National University Bundang Hospital, South Korea
Derek James Hoare, University of Nottingham, UK
Copyright © 2017 Chen, Wang, Wang, Bo, Xia, Gu and Yin. This is an open-access article distributed under the terms of the Creative Commons Attribution License (CC BY). The use, distribution and reproduction in other forums is permitted, provided the original author(s) or licensor are credited and that the original publication in this journal is cited, in accordance with accepted academic practice. No use, distribution or reproduction is permitted which does not comply with these terms.
*Correspondence: Yu-Chen Chen, chenyuchen1989@126.com
Xindao Yin, y.163yy@163.com
† These authors have contributed equally to this work.