- 1German Center for Vertigo and Balance Disorders, Munich, Germany
- 2Department of Neurology, Ludwig-Maximilians University, Munich, Germany
- 3Department of Psychiatry, Medical University Innsbruck, Innsbruck, Austria
- 4Department of Psychology, University of New Mexico, Albuquerque, NM, USA
- 5Institute for Diagnostic and Interventional Neuroradiology, University Hospital Carl Gustav Carus, Dresden, Germany
- 6Department of Acute Neurology, Schön Klinik Bad Aibling, Bad Aibling, Germany
- 7Institute for Clinical Neurosciences, Ludwig-Maximilians University, Munich, Germany
Bilateral vestibulopathy (BVP) is defined as the impairment or loss of function of either the labyrinths or the eighth nerves. Patients with total BVP due to bilateral vestibular nerve section exhibit difficulties in spatial memory and navigation and show a loss of hippocampal volume. In clinical practice, most patients do not have a complete loss of function but rather an asymmetrical residual functioning of the vestibular system. The purpose of the current study was to investigate navigational ability and hippocampal atrophy in BVP patients with residual vestibular function. Fifteen patients with BVP and a group of age- and gender- matched healthy controls were examined. Self-reported questionnaires on spatial anxiety and wayfinding were used to assess the applied strategy of wayfinding and quality of life. Spatial memory and navigation were tested directly using a virtual Morris Water Maze Task. The hippocampal volume of these two groups was evaluated by voxel-based morphometry. In the patients, the questionnaire showed a higher spatial anxiety and the Morris Water Maze Task a delayed spatial learning performance. MRI revealed a significant decrease in the gray matter mid-hippocampal volume (Left: p = 0.006, Z = 4.58, Right: p < 0.001, Z = 3.63) and posterior parahippocampal volume (Right: p = 0.005, Z = 4.65, Left: p < 0.001, Z = 3.87) compared to those of healthy controls. In addition, a decrease in hippocampal formation volume correlated with a more dominant route-finding strategy. Our current findings demonstrate that even partial bilateral vestibular loss leads to anatomical and functional changes in the hippocampal formation and objective and subjective behavioral deficits.
Introduction
Bilateralvestibulopathy (BVP) is characterized by impairment or complete loss of function of either the peripheral labyrinths or the eighth nerves (Baloh et al., 1989). The deficits can include both the semicircular canals and the otolith organs (Wiest et al., 2001). The most distressing symptom is unsteadiness of gait, which worsens in darkness and on uneven ground when vision and proprioception cannot substitute for the missing vestibular input (Crawford, 1952). About one half of patients also suffer from apparent motion of the visual scene (oscillopsia) during head movements or locomotion (Zingler et al., 2007), which is caused by involuntary retinal slip due to the deficient vestibulo-ocular reflex. With absence of motion, the patients are asymptomatic. BVP patients have a reduced quality of life with impaired social functioning (Guinand et al., 2012).
BVP is most commonly an adverse effect of aminoglycoside therapy or is caused by Meniere's disease or meningoencephalitis. However, in many patients, especially the elderly, the etiology remains unknown (Zingler et al., 2007). In the vast majority of these patients vestibular loss is incomplete and asymmetric. Electrophysiological testing, such as caloric irrigation and vestibular-evoked potentials, reveal that most patients have some residual vestibular function. For example, only 60% of the BVP patients with pathological canal function also had pathological otolith function (Agrawal et al., 2013). Total loss of peripheral vestibular function is very rare; it typically results from bilateral nerve surgical section in patients with Neurofibromatosis type II.
The human hippocampal formation (HF), i.e., the hippocampus (HC) and parahippocampus (PHC), is known to have functional importance in various aspects of memory (Scoville and Milner, 2000; Manns et al., 2003a,b) and to play an essential role in spatial memory and navigation (Maguire et al., 1996; Kessels et al., 2001; Spiers et al., 2001; Astur et al., 2002). Extensive navigational training (e.g., in taxi drivers) can lead to changes in HF volumes with smaller volumes in the anterior HF and larger volumes in the posterior HF (Maguire et al., 2000). Patients with vestibular nerve sections as well as those with extensive vestibular training display altered HF volumes (Brandt et al., 2005; Hufner et al., 2007, 2011a). Patients recovering from vestibular neuritis, which leads to an acute unilateral vestibular deficit, also show volume changes in the HF (zu Eulenburg et al., 2010).
In an earlier study in patients with bilateral vestibular nerve section we found deficits in spatial memory and navigation as well as hippocampal atrophy (Brandt et al., 2005). This suggests that a functioning vestibular system is important for spatial memory and navigation. A unilateral vestibular loss, however, did not greatly affect spatial memory or navigational performance, probably because sufficient information flows from the healthy side (Hufner et al., 2007). It remains to be seen if reduced and probably faulty input on both sides leads to such deficits, despite the same residual function.
In this study we assessed the navigational difficulties of patients with severe, but incomplete bilateral vestibulopathy, and possibly accompanying structural changes in the brain. In particular spatial memory and navigation performance were quantitatively evaluated with the virtual Morris water maze. Self-report questionnaires on spatial anxiety and spatial strategy were administered to investigate navigational problems in daily life. Finally, to examine structural changes in brain regions relating to navigation the hippocampal and parahippocampal gray matter volumes of patients and healthy controls were compared using voxel-based morphometry.
Our aim is to precisely characterize functional and structural changes in spatial memory and navigation in BVP in order to help physicians and physiotherapists recognize and treat these deficits.
Materials and Methods
Patient Characteristics
Fifteen patients with BVP (9 males, mean age 63.6 ± 11.5 years) with unsteadiness of gait were recruited from the German Centre for Vertigo and Balance Disorders, Munich. BVP was defined as (1) a bilateral pathological head-impulse test established by at least two experienced clinicians (O.K. and K.H.) and (2) a bilaterally reduced (mean peak slow phase velocity ≤6°/s) or absent responsiveness to bithermal (44 and 30°) caloric irrigation. Mean peak slow phase velocity (SPV) was calculated as the average of the peak slow velocities of the bithermal caloric-induced nystagmus on both sides. The cut-off of 6°/s refers to internal laboratory values, also in accordance with the literature (Vesterhauge and Kildegaard Larsen, 1977). Disease duration was 13.6 ± 17.4 years. Patients with clinical signs indicating dementia or mild cognitive impairment, cerebellar involvement or other neurological diseases were excluded from the study. Fifteen age- and sex-matched control subjects (CON) (mean age 63.6 ± 10.02 years), without a history of neurological diseases, vertigo or dizziness, and with a normal clinical head-impulse test and basic neurological clinical examination were also assessed. None of the subjects of the two groups were taking medication that directly affected cognitive function, e.g., antidepressants or antipsychotic drugs.
MRI scanning was not possible in two BVP patients and two CON due to contraindications for scanning. Handedness was determined according to the Edinburgh handedness inventory (Oldfield, 1971). Current clinical symptoms (only applicable to patients), including oscillopsia and ability to read signs while moving, as well as daily physical activities were assessed using a standardized questionnaire. The BVP and CON groups were also asked about the frequency of computer use (daily vs. 2–5 days/week vs. seldom/never). Questionnaire results were compared using Chi-Square (χ2) test.
The study was approved by the local ethics committee of the Ludwig-Maximilians University and conducted in accordance with the principles described in the Declaration of Helsinki. All subjects gave their written informed consent prior to the study.
Spatial Navigation and Spatial Anxiety
To quantify the patients' self-evaluation of their spatial performance two clinical scales were used: the Wayfinding Scale and the Spatial Anxiety Scale (Lawton, 1994). The Wayfinding Scale measures the degree to which participants use either a route (self-based position, Cronbach alpha = 0.73 for internal consistency) or an orientation strategy (environment-based, Cronbach alpha = 0.65) for navigation. The higher the value, the more the subjects employed the strategy being assessed. Persons with a high route strategy value tend to focus on specific instructions on how to get from place to place based on landmarks in the environment without a larger understanding of the environment (Lawton, 1994). A high score on the orientation strategy means that persons tend to develop a map-like or survey understanding of the environment and can update their own position within this environment (Lawton, 1994). Previous work showed that only a fraction of the population is able to develop this type of survey knowledge (Ishikawa and Montello, 2006), which is thought to depend on the hippocampus (Rodgers et al., 2012; Wiener et al., 2013). BVP patients were therefore expected to have a lower orientation score and higher route score, and the orientation strategy was expected to positively correlate with hippocampus gray matter volume, whereas the route strategy was expected to negatively correlate with the volume of the hippocampus.
The Spatial Anxiety Scale (Cronbach alpha = 0.80) is a 5-point Likert-type scale that assesses the anxiety participants have in eight daily life situations requiring spatial or navigational skills. The higher the value, the more strenuous the spatial tasks are for the subject. Both tests are indirect measures of spatial ability (Lawton, 1994). Results were weighted according to Lawton (1994), and group comparisons were performed using Student's t-test. The results of the Wayfinding scale and the Spatial Anxiety Scale were correlated using Pearson's correlation, according to Lawton (1994). P < 0.05 was considered significant for these analyses.
Memory Assessment
All tests were administered to all participants (BVP and CON). A German-language adaptation of the national adult reading test of Nelson was selected to estimate premorbid intelligence (MWI-B Test) (Lehrl, 2005). The following subtests of the Wechsler Memory Scale-Revised were administered to measure general memory performance (Härting et al., 2000): Mini Mental Test, Visual Reproduction I and II, Logical Memory I and II, Figural memory and Digit Span. Raw test values were transformed into percent ranges according to the age norms of the respective task (subjects >75 years were included in the eldest age group of 65–74). The Doors sub-test of the Doors and People Test (Baddeley et al., 1994) was used as a measure of long-term visual recognition memory. The normative results were compared using Student's t-test in all memory tests. P < 0.05 was considered significant for these analyses.
Virtual Morris Water Task (vMWT)
The Morris water task is considered the gold standard for testing spatial learning, spatial memory, and navigation in rodents (Morris, 1984). The virtual version of this test and its validation for determining human spatial learning, spatial memory, and navigation abilities are described in detail elsewhere (Hamilton et al., 2002; Driscoll et al., 2003). The test has been shown to effectively detect deficits in spatial memory in an elderly population (Driscoll et al., 2005) and in patients with bilateral vestibular failure (Brandt et al., 2005). Here a version of the virtual test adapted for elderly subjects was used: hidden and visible platform trials were applied before each testing phase. The subjects were given written instructions prior to testing, the testing procedures were explained in detail, and subjects had ample time to ask their questions of the investigator. A training phase with one or more test trials before the experimental trials allowed the subject to familiarize herself/himself with the required task. During the trial no assistance or verbal clues were given. Both groups received the same protocol.
In brief, the basic features of the environment consisted of a circular pool located in the center of a room with a square floor plan (Figure 1). Four conspicuous cues of equal size were placed around the distal walls. The cues were positioned so that one cue was on each of the four distal room walls, and the platform could not be encountered by simply moving toward a single cue from any release point. The platform was positioned in the center of one quadrant (N/E) and occupied 2% of the pool area. A first-person view of the virtual environment was displayed. The observer's position was always slightly above the surface of the water, and forward movement was controlled by the UP (:) arrow key on the keyboard. Rotation was controlled by the LEFT (/) and RIGHT (?) arrow keys. Backward navigation or up-down movement within the pool was not possible. A full 360° rotation in the absence of forward movement required 2.5 s to complete, and the direct path from a release point to the opposite side of the pool tool 4 s.
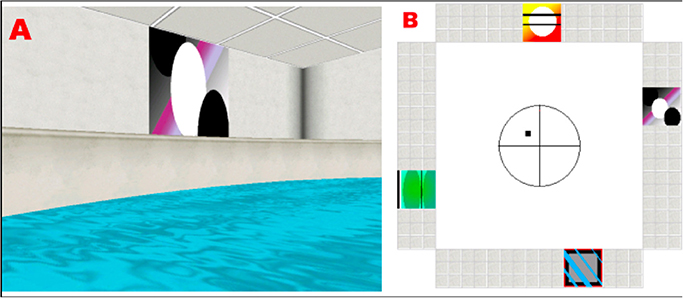
Figure 1. (A) Example of a visual cue as seen from the subject's perspective, while navigating in the platform. (B) Floor plan of the virtual room, indicating the position of the cues and the platform (black square).
Three phase trials were performed:
1) Phase I consisted of 10 blocks with 2 visible platform trials each (i.e., a total of 20 trials)
2) Phase II consisted of finding a hidden platform that was always in the same position starting from different points and
3) Phase III consisted of a single, 45-s-long probe trial during which the platform was removed from the environment.
The exact duration of the whole task depended on how fast the subjects found the platform. The time limit for trials I and II was 60 s.
Three measures were computed for each training trial: latency and path length to the platform in each trial and the heading error (deviation from a direct trajectory to the platform measured when the subject had traveled 25% of the pool diameter from the release point in degrees). Group comparisons (BVP and CON) were conducted with separate repeated measures analyses of covariance (RM ANCOVAs) with sex and group as between-subjects factors, trial block as a within-subjects factor and age as a covariate. To provide an additional qualitative assessment for hidden platform training trials three raters blind to group membership classified each participant as either (1) learning to execute direct trajectories to the platform from each release point or (2) using a strategy that did not result in execution of direct trajectories. These values were compared between BVP patients and CON using separate, repeated measures ANCOVA analyses of covariance with sex as between-subjects factors and with trial block as a within-subjects factor, and age as a covariate.
For the probe trial (Phase III) the time and distance spent in the platform quadrant were additionally assessed. A multivariate ANCOVA (MANCOVA) was performed, since the measures are all inherently correlated, with percent time and path length in the platform quadrant, latency and path length to enter the platform quadrant as dependent measures, and subject group and sex as between-subjects factors with age as a covariate.
Data of one BVP patient and one control could not be analyzed for technical reasons.
There were significant negative relationships between age and all dependent measures reported here (p < 0.001); therefore, age was included as a covariate for all analyses of the data from the vMWT.
Brain MRI Analysis
Acquisition protocol: Acquisitions were done with a 3-T GE Signa HDx scanner using an eight-channel head coil. A 3-D gradient-echo sequence (FSPGR fast spoiled gradient recalled), with a voxel size of 0.86 × 0.86 × 1.4 mm with 0.7 mm oversampling in the z direction was used to acquire T1-weighted brain images from all subjects (TE: 3.2 ms, TR: 7.9 ms, Ti: 500 ms, flip angle: 15°).
Voxel-Based Morphometry (VBM)
VBM was used to analyze the 3-D FSPGR data sets. Data were processed using SPM8 (Wellcome Department of Cognitive Neurology, London, UK, http://www.fil.ion.ucl.ac.uk/spm) with the integrated VBM toolbox (VBM8, http://dbm.neuro.uni-jena.de/vbm/) and Matlab (MathWorks, Natick, MA, USA). Data sets of left-handed subjects (75–100% left handedness on the Edinburgh handedness inventory (Oldfield, 1971): three in the BVP group and one in the CON group) were flipped and then analyzed together with right-handed data sets. This step was applied since a lateralization of the cortical representation (right hemisphere dominance for right-handers and left hemisphere for left-handers) of the vestibular system was described previously (Dieterich et al., 2003). The following preprocessing steps of the VBM toolbox were applied: spatial normalization to the space defined by the MNI template, tissue classification, and registration using linear and non-linear transformations (warping) within the same generative model (Ashburner and Friston, 2005). Analysis was performed on modulated gray matter (GM) segments. They were multiplied by the non-linear components derived from the normalization matrix to preserve actual GM volumes locally, and then smoothed with an 8-mm isotropic Gaussian kernel. The confounding effects of individual differences in brain orientation, alignment, and different brain sizes were accounted for by applying modulation for non-linear warping only (Luders et al., 2009).
Voxel-wise GM differences between BVP patients and controls were compared using statistical parametric mapping software (SPM8) and a general linear model with one dependent variable (the voxel-wise GM volume changes) and multiple independent variables (univariate multiple linear regression, where all of the factors were considered simultaneously). The following independent variables were included in the analysis: results of the Doors Test, Wayfinding Score Route Strategy and Orientation Strategy, premorbid intelligence (MWT-B), Spatial Anxiety Scores, vMWT data “percent time in target quadrant” and “latency to target quadrant,” gender and age. Care was taken to select the independent variables, so as to exclude their correlation. Parameters were estimated using a least-squares approach; the t-statistic is a valid statistic for testing whether the slope of the relationship between the dependent variable and a linear combination of the independent variables is greater than zero. Grubb's test was used to identify potential outliers. Only clusters of 20 voxels or more were included in the analysis. Voxel-wise statistical parametric maps were created, which identified brain regions containing significant differences of local GM volume for the contrasts of interest; familywise error correction (FWE) was applied (Wright et al., 1995; Ashburner and Friston, 1997). A region of interest (ROI) was created for the hippocampus (HC), the parahippocampus (PHC), grouped together as the hippocampal formation (HF) and the caudate nucleus using the WFU-Pickatlas (ANSIR, Wake Forest University; Maldjian et al., 2003). Anatomical structures were named according to the Automated Anatomical Labeling Atlas (Tzourio-Mazoyer et al., 2002). The results for the hippocampus were described as located in the “anterior,” “middle,” or “posterior” hippocampus depending on the y coordinates obtained, since different hippocampal regions are implicated in distinct cognitive processes (Fanselow and Dong, 2010; Hufner et al., 2011a). This distinction was made by dividing the hippocampus into three regions along the Y-axis as described by Greicius et al. (2003). The MNI space was used in the current study.
Results
Patient Characteristics
The clinical characteristics and findings for the BVP patients are given in Table 1. All patients hat a clinically pathological head-impulse test (Halmagyi and Curthoys, 1988). BVP and CON did not differ in frequency of computer use and daily level of physical activity (Chi-Square, p > 0.05).
Neuropsychological Assessment
All patients and normal subjects completed all cognitive tasks and questionnaires (except for BVP 1 who did not complete the MWI-B Test and BVP 10 that left out a part of the Wayfinding scale). Subjects of the BVP group had significantly higher scores in the Spatial Anxiety Scale compared to controls [t-test, t(28) = 2.4, p = 0.023, r = 0.46; Table 2]. No significant group differences were observed in navigational strategies (orientation vs. route strategy) as measured by the Wayfinding Scale [Orientation strategy: t(27) = 1.7, p = 0.25, t(27) = 1.7, p = 0.15, Table 2]. No significant correlation between scores on the Wayfinding Scale and Spatial Anxiety Score was observed (Pearson correlation, p > 0.05). No differences in intelligence as measured by the reading test [MWI-B Test, t(27) = −0, 0.170, p = 0.87], in visual recognition memory as measured by the Doors test [t(28) = −0.43, p = 0.67] or in general memory as evaluated by the Wechsler Memory Scale Revised, [Mini Mental Test (t(28) = −0.46, p = 0.65), Visual Reproduction I (t(28) = −1.9, p = 0.7) and II (t(28) = −0.7, p = 0.49), Logical Memory I (t(28) = 0.1, p = 0.92) and II (t(28) = −1.05, p = 0.3), Figural memory (t(28) = −0.41, p = 0.69) and Digit Span (t(28) = 0.2, p = 0.67) were detected between groups].
Spatial Memory and Navigation
Visible Platform Navigation (Phase I)
No significant effects or interactions involving the group and trial block factors on latency, path length or heading error were found (ANCOVA for latency, path, length and heading error, p > 0.10 in all tests). Males were significantly faster than females [latency in males < females; F(1, 23) = 8.108, p = 0.009], and more precise [heading error males < females; F(1, 23) = 11.142, p = 0.003, r2 = 0.326], as shown in previous studies (Hufner et al., 2007); however, there were no other significant main effects or interactions involving gender (p > 0.08; Data not shown).
Hidden Platform Navigation (Phase II)
No significant effects or interactions involving the factors of interest were detected (ANCOVA; Figures 2A–C). Comparison of mean values for each dependent measure during the first 5 (1–5) and last 5 (6–10) trial blocks revealed that controls were finding the platform faster than the patients during the last 5 blocks of training [latency BVP > CTR, F(1, 23) = 4.46, p = 0.046, r2 = 0.163] [Figures 2D–F). All other effects and interactions were not significant (p > 0.05)].
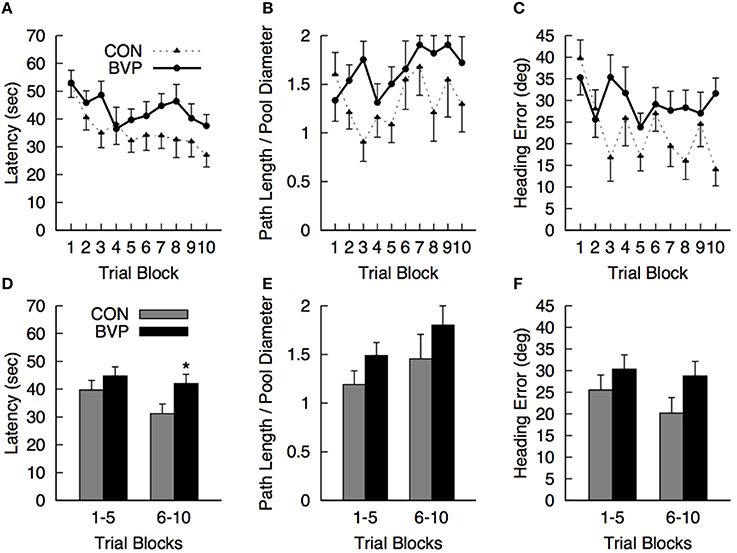
Figure 2. Mean (+SEM) measures during the hidden platform training (Phase II) for the control (CON) and bilateral vestibulopathy (BVP) groups; (A) Latency to navigate to the platform, (B) Path length to the platform, expressed as Path Length/Pool Diameter, (C) Heading error. (D–F)represent the same measures averaged over the first 5 and last 5 training blocks. *significant group effect at p < 0.05.
Probe Trial (Phase III)
As mentioned before, multiple ANCOVA was used for statistical analysis. Results are shown in Figure 3. There was a significant multivariate effect of group [Wilks' Λ: 0.485; F(4, 20) = 5.30, p = 0.004]. The main effect of gender and of group × gender interaction was not significant (p > 0.10). The standardized discriminate function coefficients for the multivariate group effect were ordered as follows: percent path length to platform quadrant (3.06), latency to enter the platform quadrant (−2.67), percent path length in the platform quadrant (1.48) and percent time in the platform quadrant (−0.56), indicating that the groups were best discriminated by variables related to navigating quickly and directly to the platform quadrant. Variables related to persistence in searching were somewhat less discriminating. It is important to note that the greater path length to navigate to the platform quadrant in controls compared to BVP patients was largely due to the two significant outliers (>2 standard deviations). When removed, the mean path length for group CON dropped significantly (26%) to 0.55 (SEM = 0.9). However, because of the high variability in the individual measures, none of the direct comparisons among groups for the individual dependent measures were significant (p > 0.30).
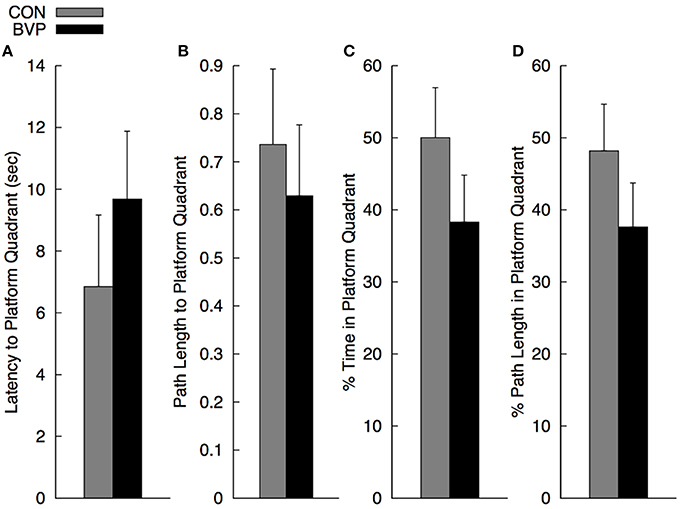
Figure 3. Mean (+SEM) measures during the no-platform probe trial (Phase III) for the control (CON) and bilateral vestibulopathy (BVP) groups; (A) Latency to enter the platform quadrant, (B) Path length to the platform quadrant, expressed as Path Length/Pool Diameter, (C) Percent time spent in the platform quadrant, and (D) Percent path length spent in the platform quadrant.
MRI Analysis
Voxel-Based Morphometry of Gray Matter Volumes
Gray matter brain volumes of BVP patients were compared to those of matched controls using voxel-based morphometry (Wright et al., 1995; Ashburner and Friston, 1997; Critchley et al., 2003). Whole brain analysis did not reveal any areas of gray matter change (p > 0.05, FWE corr.) for both contrasts (BVP > CON and CON>BVP). A ROI analysis of the HC and PHC detected no gray matter changes for the contrast BVP > CON (p > 0.05 FWE corr. or 0.001 uncorr.). For the contrast CON > BVP loss of gray matter was found in the HC and PHC bilaterally (Table 3, Figure 4). This included the mid-hippocampus bilaterally with a maximum at y = −22 right and at y = −25 left, reaching into the posterior HC on the left side, as well as the posterior PHC bilaterally (y = −37). No differences were observed in the caudate nucleus between the two groups.
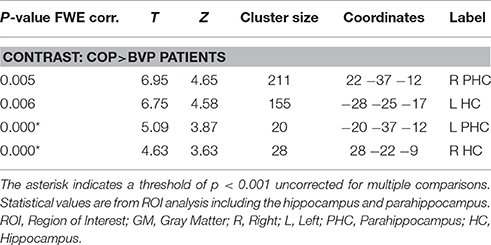
Table 3. GM coordinates and cluster sizes of areas of the hippocampal formation with larger GM volume in healthy controls compared to BVP patients.
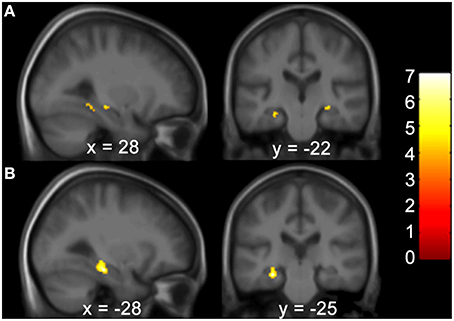
Figure 4. Areas of higher GM volume in healthy controls compared to BVP patients. (A) Clusters of GM differences between the two groups are shown on sagittal and coronal planes through the maximum cluster in the right hemisphere at 28 −22 −9 at p < 0.001 uncorr. and (B) left hemisphere at −28 −25 −17 p < 0.05 FWE corr. Clusters and significance values are from an ROI analysis of the HC and PHC bilaterally and are projected onto a mean image of the included subjects. Color bars indicate the range of t-values. The threshold for statistical significance was 3.69.
Relationship between HF Volume and Spatial Performance
Correlation analyses were performed to test for an effect of the covariates on regional GM brain volumes in the HF and caudate nucleus in patients and controls, separately and grouped together. For the results of the Doors Test, Wayfinding Score orientation strategy, premorbid intelligence (MWT-B), vMWT data “percent time in target quadrant” and “latency to target quadrant” and gender no significant effect was found (p > 0.001 uncorrected). However, results of the Wayfinding Score route strategy showed an inverse effect on HF volume: a maximum occurred at −28 −25 −17 (left middle HC reaching into the posterior HC), at 28 −24 −9 (right middle HC reaching into posterior HC), and at 22 −36 −11 (right posterior PHC) for the entire cohort (Table 4; Figure 5). The caudate nucleus data showed a positive correlation with the route strategy only at −6 10 −0 (T = 3.77, Z = 3.17, p = 0.001).
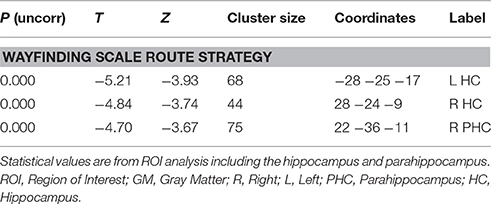
Table 4. GM coordinates and cluster sizes of areas that correlate negatively with the route strategy score of the Wayfinding scale.
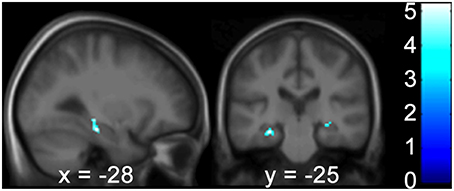
Figure 5. The Wayfinding Scale route strategy scores correlate negatively with GM hippocampal volume bilaterally. Results are shown in a sagittal and coronal plane through the peak voxel at −28 −25 −17 and with the PHC GM volume on the right (not visible here). (p < 0.001 uncorr., ROI analysis of the HC and PHC bilaterally). Color bars indicate the range of t-values. The threshold for statistical significance was 3.69. PHC, Parahippocampus; GM, Gray Matter; ROI, Region of Interest; HC, Hippocampus.
Discussion
In the present study we demonstrate subtle deficits in spatial memory and navigation as well as atrophy of the mid-hippocampus and the posterior parahippocampus and increased spatial anxiety, all of which were detected in an unselected patient population with severe but incomplete BVP. The patients did not differ from controls in general memory or whole brain gray matter volume. The specific impairment of spatial memory and navigation detected in these patients together with the increased spatial anxiety contributes to a reduced quality of life as described earlier (Guinand et al., 2012; Agrawal et al., 2013).
Increased Spatial Anxiety in Patients with BVP
The Spatial Anxiety Scale was developed to quantify anxiety about environmental navigation (Lawton, 1994). This scale provides an objective measure of the difficulties that these patients face and takes into account daily situations requiring spatial navigation abilities, such as finding one's car in a parking lot or finding one's way in a supermarket. Patients showed on average higher scores than their matched controls. This result provides evidence that the spatial deficits in BVP, as shown in the current and previous studies (Brandt et al., 2005; Hufner et al., 2007) are not limited to laboratory test conditions. These deficits have been neglected so far in the clinical routine, partly because they are not captured by the standard Dizziness Handicap Inventory (DHI) score (Jacobson and Newman, 1990; Guinand et al., 2012). They are, however, clinically relevant since increased levels of anxiety can lead to an increase in secondary somatoform vertigo (Tschan et al., 2011) and further reduce the quality of life.
Spatial Memory and Navigation Are Impaired in BVP
The increased spatial anxiety in BVP was associated with an objectively impaired spatial memory and navigation (measured by the virtual version of the Morris water task). The patients' impairment was most evident in the parameters measuring direct and rapid navigation to the platform quadrant in the probe trial, particularly during the late phase of learning. Furthermore, patients required more time for the less direct trajectories to the platform during the training phase than controls. These impairments were not as pronounced as those observed in patients with complete bilateral vestibular deafferentation (Brandt et al., 2005), most likely due to our patients' residual vestibular input. Such a pattern in the vMWT suggests that the degree of vestibular damage and the degree of impairment in place learning and memory are related. Mild impairments indicated in the vWMT were accordingly detected in patients with only right vestibular lesions (Hufner et al., 2007).
Impairments of performance in the vMWT have been reported for elderly subjects. They were particularly evident in subjects within the age ranges used in the present study (Driscoll et al., 2005). However, all patients and controls sampled here were matched for age. Because there were significant negative relationships between age and all dependent measures reported, age was included as a covariate for all analyses. Therefore, the observed impairments in spatial learning memory in the VMWT related to vestibular input represent effects above and beyond those attributable to normal age-related decline.
BVP Leads to Atrophy of the Hippocampal Formation
It is well recognized that the vestibular system is relevant for human spatial memory and navigation as well as for the integrity of the hippocampal formation (HF), i.e., the parahippocampal region and the hippocampus proper. Several studies in humans have shown that there is an intimate interaction between the vestibular system and the HF, for example, the use of vestibular stimulation provided evidence of HF activation with functional imaging (Vitte et al., 1996; Suzuki et al., 2001; Dieterich et al., 2003) as well as neuropsychological changes in spatial memory (Bachtold et al., 2001). Human lesion studies showed that the abolition of vestibular input causes significant deficits in spatial memory as well as hippocampal volume changes (Schautzer et al., 2003; Brandt et al., 2005; zu Eulenburg et al., 2010; Alessandrini et al., 2013).
The present VBM analysis showed atrophy of the mid-hippocampus and the posterior parahippocampus bilaterally in patients with chronic, bilaterally reduced vestibular function. On the other hand, neither generalized brain atrophy nor atrophy of the caudate nucleus was noted. Patients with unilateral vestibular deafferentation after acoustic neurinoma removal did not show significant atrophy of the HF, probably because the unilateral vestibular input was preserved in the HF (Hufner et al., 2007). An fMRI study of subjects with bilateral vestibular deafferentation and blind subjects proposed that vestibular input enters the hippocampal formation at its anterior aspect, i.e., the entorhinal cortex, while the processing of visual information takes place in the more posterior aspects (Jahn et al., 2009). A meta-analysis supports this view of a spatial visual-vestibular separation of information processing in the hippocampal formation (Hufner et al., 2011b). In our meta-analysis the maxima of vestibular signals were located in the anterior and middle HC.
The maximum of HC atrophy was also located within this range but extended slightly more posteriorly on the left side (maxima at y = −25 left and −22 right). This is similar to the region (y = −23) found in a recent study on patients after unilateral vestibular neuritis; atrophy was only found on the left side of the HC irrespective of the side of vestibular failure (zu Eulenburg et al., 2010).
In the PHC, vestibular-related information was likely to be located more anteriorly and visual information more posteriorly, although this separation was less evident than in the HC (Hufner et al., 2011b). In the current analysis the volume changes in the PHC were located in the posterior aspects bilaterally, which, according to the model described, cannot directly be attributed to the loss of vestibular input. This suggests that a complex network for multisensory information processing is present in the HF.
Measures of Hippocampal Volume and Spatial Performance
The relevance of the HF, the right side in particular, for spatial orientation and navigation has been revealed in both animal and human studies (Moser et al., 1993; Ghaem et al., 1997; Maguire et al., 1997; Gron et al., 2000; Astur et al., 2002; Hartley et al., 2003; Zhang et al., 2004). The patients in the present study did not differ from controls in their self-reported navigation strategies (route vs. orientation strategy), both of which were measured by the Wayfinding Scale; however, their performance in the vMWT was reduced. The GM volume of the HF did not correlate with any quantitative performance scores. Thus, there is no proof of a direct relationship between disease-related hippocampal volume changes and spatial navigation deficits. The only correlation between performance and GM volume was found for the middle-to-posterior hippocampus, including the right posterior parahippocampus. This volume also correlated negatively with the route strategy in our entire subject cohort. Therefore, with smaller the hippocampal size, the route strategy became more dominant.
The Wayfinding Scale consists of a set of questions weighted according to two different navigation strategies: orientation and route. The orientation strategy can be thought of as monitoring self-position information rather than external environmental cues (Lawton, 1994). Questions that are weighted toward this strategy include, but are not limited to, orientation or place strategies and the development of a cognitive map, which are known to be dependent on the hippocampus (Tolman, 1948; Cheung et al., 2012), particularly the posterior hippocampus (Janzen and van Turennout, 2004). It is strange that the orientation strategy showed no positive correlation with the hippocampus. However, previous studies showed that values from the route strategy are more sensitive to differences of gender (Lawton, 1994) and culture (Lawton and Kallai, 2002). Questions that are weighted toward the route strategy primarily rely on directions (Lawton, 1994), e.g., turn right at the next intersection. The elderly (Rodgers et al., 2012; Wiener et al., 2013) and women (Lawton, 1994) prefer such route-, response-based strategies. The negative correlation between route-based navigation and posterior hippocampal volume could reflect this higher sensitivity.
On the other hand, caudate nucleus showed only a weak correlation at −6 10 −0 with the route strategy, which on the whole is consistent with navigation strategies that do not depend on environmental clues (Bohbot et al., 2007). Nevertheless, it is difficult to draw any further conclusions based on this result.
Although the orientation strategy may be based more on self-position monitoring, both strategies have sensorimotor components that require accurate vestibular information for successful navigation. Indeed this may partially explain why no behavioral differences were found between our groups. However, it is still possible that given lower between-subject variability and a larger sample size, differences in behavior and the resulting reduction in hippocampal volume may become apparent.
Effects of Stress on Hippocampal Volumes
In light of the increased spatial anxiety scores of BVP patients, it is of interest that deficits of spatial memory and navigation have also been reported to occur in children with anxiety disorders (Mueller et al., 2009). Furthermore, adults with social phobias show higher cerebral blood flow in the anterior and middle hippocampal regions (y = −13) when anticipating speaking in public (Tillfors et al., 2002); this area includes the region showing GM atrophy in our patients. These findings point to a two-way interaction between anxiety and hippocampal volume which involves spatial memory and navigation. Fanselow and Dong (2010) proposed that the dorsal HC is involved in information processing (spatial orientation predominantly on the right side), and the ventral HC correlates with emotion and stress. This theory derived from evaluation of the expression of genetic markers as well as functional lesion studies. Their theory also supports the notion of an interaction between spatial memory and anxiety within the hippocampus.
A stress effect could be responsible for the discrepancies between human and animal studies. Animal studies have not so far shown hippocampal atrophy after bilateral vestibular damage (Besnard et al., 2012; Zheng et al., 2012). One contributing factor that should be taken into consideration (besides loss of vestibular input) is the higher anxiety levels described in patients with BVP (Guinand et al., 2012; Saman et al., 2012), partly due to social difficulties (Agrawal et al., 2013). Laboratory animals, on the other hand, are rather hyperactive after labyrinthectomy, which may help maintain hippocampal volumes (Zheng et al., 2012) and contrary to humans (Horii et al., 2007), they do not show higher levels of circulating corticosteroids after vestibular deafferentation (Lindsay et al., 2005; Russell et al., 2006).
Conclusions
Our current findings demonstrate that partial bilateral vestibular loss also leads to anatomical and functional changes in the hippocampal formation, which are reflected in subjective and objective behavioral deficits. These deficits should be directly addressed by the attending physicians, when evaluating daily life challenges in those patients.
Author Contributions
OK designed the experiment, recruited subjects, acquired, analyzed and interpreted the data and drafted the manuscript. KH designed the experiment, acquired, analyzed and interpreted the data and drafted the manuscript. VF designed the experiment, acquired analyzed and interpreted the data and drafted part or the manuscript. DH designed the experiment, analyzed and interpreted the data and drafted part of the manuscript. JL substantially contributed to the conception of the experiment and acquisition and interpretation of the data and critically revised the manuscript. MS substantially contributed to the conception of the experiment, interpretation of the data and critically revised the manuscript. KJ substantially contributed to the conception of the experiment, interpretation of the data and critically revised the manuscript. TB substantially contributed to the conception and design of the experiment, interpretation of the data and critically revised the manuscript. All authors gave final approval for publication and agreed to be accountable for all aspects of the work in ensuring that questions related to the accuracy or integrity of any part of the work are appropriately investigated and resolved.
Conflict of Interest Statement
The authors declare that the research was conducted in the absence of any commercial or financial relationships that could be construed as a potential conflict of interest.
Acknowledgments
This work was supported by the Hertie Foundation and the German Federal Ministry of Education and Research (German Vertigo and Dizziness Center, Grant Number: 01 EO 0901). The authors would like to thank Ms. Judy Benson for copyediting the manuscript.
Abbreviations
ANOVA, analysis of variance; BVP, bilateral vestibulopathy patients; CON, control patients; GM, gray matter; HC, hippocampus; HF, hippocampal formation; PHC, parahippocampus; ROI, region of interest; vMWT, virtual Morris water task.
References
Agrawal, Y., Bremova, T., Kremmyda, O., and Strupp, M. (2013). Semicircular canal, saccular and utricular function in patients with bilateral vestibulopathy: analysis based on etiology. J. Neurol. 260, 876–883. doi: 10.1007/s00415-012-6724-y
Alessandrini, M., Pagani, M., Napolitano, B., Micarelli, A., Candidi, M., Bruno, E., et al. (2013). Early and phasic cortical metabolic changes in vestibular neuritis onset. PLoS ONE 8:e57596. doi: 10.1371/journal.pone.0057596
Ashburner, J., and Friston, K. (1997). Multimodal image coregistration and partitioning–a unified framework. Neuroimage 6, 209–217. doi: 10.1006/nimg.1997.0290
Ashburner, J., and Friston, K. J. (2005). Unified segmentation. Neuroimage 26, 839–851. doi: 10.1016/j.neuroimage.2005.02.018
Astur, R. S., Taylor, L. B., Mamelak, A. N., Philpott, L., and Sutherland, R. J. (2002). Humans with hippocampus damage display severe spatial memory impairments in a virtual Morris water task. Behav. Brain Res. 132, 77–84. doi: 10.1016/S0166-4328(01)00399-0
Bachtold, D., Baumann, T., Sandor, P. S., Kritos, M., Regard, M., and Brugger, P. (2001). Spatial- and verbal-memory improvement by cold-water caloric stimulation in healthy subjects. Exp. Brain Res. 136, 128–132. doi: 10.1007/s002210000588
Baddeley, A., Emslie, H., and Nimmo-Smith, I. (1994). Doors and People Test. Bury St. Edmunds: Thames Valley Test Company.
Baloh, R. W., Jacobson, K., and Honrubia, V. (1989). Idiopathic bilateral vestibulopathy. Neurology 39(2 Pt 1), 272–275. doi: 10.1212/WNL.39.2.272
Besnard, S., Machado, M. L., Vignaux, G., Boulouard, M., Coquerel, A., Bouet, V., et al. (2012). Influence of vestibular input on spatial and nonspatial memory and on hippocampal NMDA receptors. Hippocampus 22, 814–826. doi: 10.1002/hipo.20942
Bohbot, V. D., Lerch, J., Thorndycraft, B., Iaria, G., and Zijdenbos, A. P. (2007). Gray matter differences correlate with spontaneous strategies in a human virtual navigation task. J. Neurosci. 27, 10078–10083. doi: 10.1523/JNEUROSCI.1763-07.2007
Brandt, T., Schautzer, F., Hamilton, D. A., Bruning, R., Markowitsch, H. J., Kalla, R., et al. (2005). Vestibular loss causes hippocampal atrophy and impaired spatial memory in humans. Brain 128(Pt 11), 2732–2741. doi: 10.1093/brain/awh617
Cheung, A., Ball, D., Milford, M., Wyeth, G., and Wiles, J. (2012). Maintaining a cognitive map in darkness: the need to fuse boundary knowledge with path integration. PLoS Comput. Biol. 8:e1002651. doi: 10.1371/journal.pcbi.1002651
Crawford, J. (1952). Living without a balancing mechanism. N. Engl. J. Med. 246, 458–460. doi: 10.1056/NEJM195203202461207
Critchley, H. D., Mathias, C. J., Josephs, O., O'Doherty, J., Zanini, S., Dewar, B. K., et al. (2003). Human cingulate cortex and autonomic control: converging neuroimaging and clinical evidence. Brain 126(Pt 10), 2139–2152. doi: 10.1093/brain/awg216
Dieterich, M., Bense, S., Lutz, S., Drzezga, A., Stephan, T., Bartenstein, P., et al. (2003). Dominance for vestibular cortical function in the non-dominant hemisphere. Cereb. Cortex 13, 994–1007. doi: 10.1093/cercor/13.9.994
Driscoll, I., Hamilton, D. A., Petropoulos, H., Yeo, R. A., Brooks, W. M., Baumgartner, R. N., et al. (2003). The aging hippocampus: cognitive, biochemical and structural findings. Cereb. Cortex 13, 1344–1351. doi: 10.1093/cercor/bhg081
Driscoll, I., Hamilton, D. A., Yeo, R. A., Brooks, W. M., and Sutherland, R. J. (2005). Virtual navigation in humans: the impact of age, sex, and hormones on place learning. Horm. Behav. 47, 326–335. doi: 10.1016/j.yhbeh.2004.11.013
Fanselow, M. S., and Dong, H. W. (2010). Are the dorsal and ventral hippocampus functionally distinct structures? Neuron 65, 7–19. doi: 10.1016/j.neuron.2009.11.031
Ghaem, O., Mellet, E., Crivello, F., Tzourio, N., Mazoyer, B., Berthoz, A., et al. (1997). Mental navigation along memorized routes activates the hippocampus, precuneus, and insula. Neuroreport 8, 739–744. doi: 10.1097/00001756-199702100-00032
Greicius, M. D., Krasnow, B., Boyett-Anderson, J. M., Eliez, S., Schatzberg, A. F., Reiss, A. L., et al. (2003). Regional analysis of hippocampal activation during memory encoding and retrieval: fMRI study. Hippocampus 13, 164–174. doi: 10.1002/hipo.10064
Gron, G., Wunderlich, A. P., Spitzer, M., Tomczak, R., and Riepe, M. W. (2000). Brain activation during human navigation: gender-different neural networks as substrate of performance. Nat. Neurosci. 3, 404–408. doi: 10.1038/73980
Guinand, N., Boselie, F., Guyot, J. P., and Kingma, H. (2012). Quality of life of patients with bilateral vestibulopathy. Ann. Otol. Rhinol. Laryngol. 121, 471–477. doi: 10.1177/000348941212100708
Halmagyi, G. M., and Curthoys, I. S. (1988). A clinical sign of canal paresis. Arch. Neurol. 45, 737–739. doi: 10.1001/archneur.1988.00520310043015
Hamilton, D. A., Driscoll, I., and Sutherland, R. J. (2002). Human place learning in a virtual Morris water task: some important constraints on the flexibility of place navigation. Behav. Brain Res. 129, 159–170. doi: 10.1016/S0166-4328(01)00343-6
Härting, C., Markowitsch, H.-J., Neufeld, H., Calabrese, P., Deisinger, K., and Kessler, J. (2000). Wechsler Memory Scale - Revised Edition. Bern: Hans Huber Verlag.
Hartley, T., Maguire, E. A., Spiers, H. J., and Burgess, N. (2003). The well-worn route and the path less traveled: distinct neural bases of route following and wayfinding in humans. Neuron 37, 877–888. doi: 10.1016/S0896-6273(03)00095-3
Horii, A., Uno, A., Kitahara, T., Mitani, K., Masumura, C., Kizawa, K., et al. (2007). Effects of fluvoxamine on anxiety, depression, and subjective handicaps of chronic dizziness patients with or without neuro-otologic diseases. J. Vestib. Res. 17, 1–8.
Hufner, K., Binetti, C., Hamilton, D. A., Stephan, T., Flanagin, V. L., Linn, J., et al. (2011a). Structural and functional plasticity of the hippocampal formation in professional dancers and slackliners. Hippocampus 21, 855–865. doi: 10.1002/hipo.20801
Hufner, K., Hamilton, D. A., Kalla, R., Stephan, T., Glasauer, S., Ma, J., et al. (2007). Spatial memory and hippocampal volume in humans with unilateral vestibular deafferentation. Hippocampus 17, 471–485. doi: 10.1002/hipo.20283
Hufner, K., Strupp, M., Smith, P., Brandt, T., and Jahn, K. (2011b). Spatial separation of visual and vestibular processing in the human hippocampal formation. Ann. N. Y. Acad. Sci. 1233, 177–186. doi: 10.1111/j.1749-6632.2011.06115.x
Ishikawa, T., and Montello, D. R. (2006). Spatial knowledge acquisition from direct experience in the environment: individual differences in the development of metric knowledge and the integration of separately learned places. Cogn. Psychol. 52, 93–129. doi: 10.1016/j.cogpsych.2005.08.003
Jacobson, G. P., and Newman, C. W. (1990). The development of the Dizziness Handicap Inventory. Arch. Otolaryngol. Head Neck Surg. 116, 424–427. doi: 10.1001/archotol.1990.01870040046011
Jahn, K., Wagner, J., Deutschlander, A., Kalla, R., Hufner, K., Stephan, T., et al. (2009). Human hippocampal activation during stance and locomotion: fMRI study on healthy, blind, and vestibular-loss subjects. Ann. N. Y. Acad. Sci. 1164, 229–235. doi: 10.1111/j.1749-6632.2009.03770.x
Janzen, G., and van Turennout, M. (2004). Selective neural representation of objects relevant for navigation. Nat. Neurosci. 7, 673–677. doi: 10.1038/nn1257
Kessels, R. P., de Haan, E. H., Kappelle, L. J., and Postma, A. (2001). Varieties of human spatial memory: a meta-analysis on the effects of hippocampal lesions. Brain Res. Brain Res. Rev. 35, 295–303. doi: 10.1016/S0165-0173(01)00058-3
Lawton, C. A. (1994). Gender differences in way-finding strategies-relationship to spatial ability and spatial anxiety. Sex Roles 30, 765–779. doi: 10.1007/BF01544230
Lawton, C. A., and Kallai, J. (2002). Gender differences in wayfinding strategies and anxiety about wayfinding: a cross-cultural comparison. Sex Roles 47, 389–401. doi: 10.1023/A:1021668724970
Lindsay, L., Liu, P., Gliddon, C., Zheng, Y., Smith, P. F., and Darlington, C. L. (2005). Cytosolic glucocorticoid receptor expression in the rat vestibular nucleus and hippocampus following unilateral vestibular deafferentation. Exp. Brain Res. 162, 309–314. doi: 10.1007/s00221-004-2168-7
Luders, E., Toga, A. W., Lepore, N., and Gaser, C. (2009). The underlying anatomical correlates of long-term meditation: larger hippocampal and frontal volumes of gray matter. Neuroimage 45, 672–678. doi: 10.1016/j.neuroimage.2008.12.061
Maguire, E. A., Frackowiak, R. S., and Frith, C. D. (1996). Learning to find your way: a role for the human hippocampal formation. Proc. Biol. Sci. R. Soc. 263, 1745–1750. doi: 10.1098/rspb.1996.0255
Maguire, E. A., Frackowiak, R. S., and Frith, C. D. (1997). Recalling routes around london: activation of the right hippocampus in taxi drivers. J. Neurosci. 17, 7103–7110.
Maguire, E. A., Gadian, D. G., Johnsrude, I. S., Good, C. D., Ashburner, J., Frackowiak, R. S., et al. (2000). Navigation-related structural change in the hippocampi of taxi drivers. Proc. Natl. Acad. Sci. U.S.A. 97, 4398–4403. doi: 10.1073/pnas.070039597
Maldjian, J. A., Laurienti, P. J., Kraft, R. A., and Burdette, J. H. (2003). An automated method for neuroanatomic and cytoarchitectonic atlas-based interrogation of fMRI data sets. Neuroimage 19, 1233–1239. doi: 10.1016/S1053-8119(03)00169-1
Manns, J. R., Hopkins, R. O., Reed, J. M., Kitchener, E. G., and Squire, L. R. (2003a). Recognition memory and the human hippocampus. Neuron 37, 171–180. doi: 10.1016/S0896-6273(02)01147-9
Manns, J. R., Hopkins, R. O., and Squire, L. R. (2003b). Semantic memory and the human hippocampus. Neuron 38, 127–133. doi: 10.1016/S0896-6273(03)00146-6
Morris, R. (1984). Developments of a water-maze procedure for studying spatial learning in the rat. J. Neurosci. Methods 11, 47–60. doi: 10.1016/0165-0270(84)90007-4
Moser, E., Moser, M. B., and Andersen, P. (1993). Spatial learning impairment parallels the magnitude of dorsal hippocampal lesions, but is hardly present following ventral lesions. J. Neurosci. 13, 3916–3925.
Mueller, S. C., Temple, V., Cornwell, B., Grillon, C., Pine, D. S., and Ernst, M. (2009). Impaired spatial navigation in pediatric anxiety. J. Child Psychol. Psychiatry 50, 1227–1234. doi: 10.1111/j.1469-7610.2009.02112.x
Oldfield, R. C. (1971). The assessment and analysis of handedness: the Edinburgh inventory. Neuropsychologia 9, 97–113. doi: 10.1016/0028-3932(71)90067-4
Rodgers, M. K., Sindone, J. A. III, and Moffat, S. D. (2012). Effects of age on navigation strategy. Neurobiol. Aging 33, 202 e15–202 e22. doi: 10.1016/j.neurobiolaging.2010.07.021
Russell, N. A., Horii, A., Smith, P. F., Darlington, C. L., and Bilkey, D. K. (2006). Lesions of the vestibular system disrupt hippocampal theta rhythm in the rat. J. Neurophysiol. 96, 4–14. doi: 10.1152/jn.00953.2005
Saman, Y., Bamiou, D. E., Gleeson, M., and Dutia, M. B. (2012). Interactions between stress and vestibular compensation - a review. Front. Neurol. 3:116. doi: 10.3389/fneur.2012.00116
Schautzer, F., Hamilton, D., Kalla, R., Strupp, M., and Brandt, T. (2003). Spatial memory deficits in patients with chronic bilateral vestibular failure. Ann. N. Y. Acad. Sci. 1004, 316–324. doi: 10.1196/annals.1303.029
Scoville, W. B., and Milner, B. (2000). Loss of recent memory after bilateral hippocampal lesions. 1957. J. Neuropsychiatry Clin. Neurosci. 12, 103–113. doi: 10.1176/jnp.12.1.103-a
Spiers, H. J., Burgess, N., Maguire, E. A., Baxendale, S. A., Hartley, T., Thompson, P. J., et al. (2001). Unilateral temporal lobectomy patients show lateralized topographical and episodic memory deficits in a virtual town. Brain 124(Pt 12), 2476–2489. doi: 10.1093/brain/124.12.2476
Suzuki, M., Kitano, H., Ito, R., Kitanishi, T., Yazawa, Y., Ogawa, T., et al. (2001). Cortical and subcortical vestibular response to caloric stimulation detected by functional magnetic resonance imaging. Brain Res. Cogn. Brain Res. 12, 441–449. doi: 10.1016/S0926-6410(01)00080-5
Tillfors, M., Furmark, T., Marteinsdottir, I., and Fredrikson, M. (2002). Cerebral blood flow during anticipation of public speaking in social phobia: a PET study. Biol. Psychiatry 52, 1113–1119. doi: 10.1016/S0006-3223(02)01396-3
Tolman, E. C. (1948). Cognitive maps in rats and men. Psychol. Rev. 55, 189–208. doi: 10.1037/h0061626
Tschan, R., Best, C., Beutel, M. E., Knebel, A., Wiltink, J., Dieterich, M., et al. (2011). Patients' psychological well-being and resilient coping protect from secondary somatoform vertigo and dizziness (SVD) 1 year after vestibular disease. J. Neurol. 258, 104–112. doi: 10.1007/s00415-010-5697-y
Tzourio-Mazoyer, N., Landeau, B., Papathanassiou, D., Crivello, F., Etard, O., Delcroix, N., et al. (2002). Automated anatomical labeling of activations in SPM using a macroscopic anatomical parcellation of the MNI MRI single-subject brain. Neuroimage 15, 273–289. doi: 10.1006/nimg.2001.0978
Vesterhauge, S., and Kildegaard Larsen, P. (1977). Normal values in a routine ENG test. Acta Otolaryngol. 84, 91–97. doi: 10.3109/00016487709123946
Vitte, E., Derosier, C., Caritu, Y., Berthoz, A., Hasboun, D., and Soulie, D. (1996). Activation of the hippocampal formation by vestibular stimulation: a functional magnetic resonance imaging study. Exp. Brain Res. 112, 523–526. doi: 10.1007/BF00227958
Wiener, J. M., de Condappa, O., Harris, M. A., and Wolbers, T. (2013). Maladaptive bias for extrahippocampal navigation strategies in aging humans. J. Neurosci. 33, 6012–6017. doi: 10.1523/JNEUROSCI.0717-12.2013
Wiest, G., Demer, J. L., Tian, J., Crane, B. T., and Baloh, R. W. (2001). Vestibular function in severe bilateral vestibulopathy. J. Neurol. Neurosurg. Psychiatr. 71, 53–57. doi: 10.1136/jnnp.71.1.53
Wright, I. C., McGuire, P. K., Poline, J. B., Travere, J. M., Murray, R. M., Frith, C. D., et al. (1995). A voxel-based method for the statistical analysis of gray and white matter density applied to schizophrenia. Neuroimage 2, 244–252. doi: 10.1006/nimg.1995.1032
Zhang, W. N., Pothuizen, H. H., Feldon, J., and Rawlins, J. N. (2004). Dissociation of function within the hippocampus: effects of dorsal, ventral and complete excitotoxic hippocampal lesions on spatial navigation. Neuroscience 127, 289–300. doi: 10.1016/j.neuroscience.2004.05.007
Zheng, Y., Balabhadrapatruni, S., Baek, J. H., Chung, P., Gliddon, C., Zhang, M., et al. (2012). The effects of bilateral vestibular loss on hippocampal volume, neuronal number, and cell proliferation in rats. Front. Neurol. 3:20. doi: 10.3389/fneur.2012.00020
Zingler, V. C., Cnyrim, C., Jahn, K., Weintz, E., Fernbacher, J., Frenzel, C., et al. (2007). Causative factors and epidemiology of bilateral vestibulopathy in 255 patients. Ann. Neurol. 61, 524–532. doi: 10.1002/ana.21105
Keywords: hippocampal atrophy, loss of vestibular function, navigation strategies, spatial anxiety, spatial orientation
Citation: Kremmyda O, Hüfner K, Flanagin VL, Hamilton DA, Linn J, Strupp M, Jahn K and Brandt T (2016) Beyond Dizziness: Virtual Navigation, Spatial Anxiety and Hippocampal Volume in Bilateral Vestibulopathy. Front. Hum. Neurosci. 10:139. doi: 10.3389/fnhum.2016.00139
Received: 04 January 2016; Accepted: 15 March 2016;
Published: 31 March 2016.
Edited by:
Klaus Gramann, Berlin Institute of Technology, GermanyReviewed by:
Sean Commins, National University of Ireland Maynooth, IrelandClayton Ford Burles, University of Calgary, Canada
Copyright © 2016 Kremmyda, Hüfner, Flanagin, Hamilton, Linn, Strupp, Jahn and Brandt. This is an open-access article distributed under the terms of the Creative Commons Attribution License (CC BY). The use, distribution or reproduction in other forums is permitted, provided the original author(s) or licensor are credited and that the original publication in this journal is cited, in accordance with accepted academic practice. No use, distribution or reproduction is permitted which does not comply with these terms.
*Correspondence: Olympia Kremmyda, olympia.kremmyda@med.uni-muenchen.de