- 1Department of Communication Sciences and Disorders and the Institute for Stuttering Treatment and Research, Faculty of Rehabilitation Medicine, University of Alberta, Edmonton, AB, Canada
- 2Neuroscience and Mental Health Institute, University of Alberta, Edmonton, AB, Canada
- 3Program in Neuroscience and Mental Health, The Hospital for Sick Children, Toronto, ON, Canada
- 4Department of Medical Biophysics, University of Toronto, Toronto, ON, Canada
- 5Haskins Laboratories, New Haven, CT, USA
- 6Centre for Research on Brain, Language and Music, McGill University, Montreal, QC, Canada
- 7Department of Speech-Language Pathology, University of Toronto, Toronto, ON, Canada
The acquisition and mastery of speech-motor control requires years of practice spanning the course of development. People who stutter often perform poorly on speech-motor tasks thereby calling into question their ability to establish the stable neural motor programs required for masterful speech-motor control. There is evidence to support the assertion that these neural motor programs are represented in the posterior part of Broca’s area, specifically the left pars opercularis. Consequently, various theories of stuttering causation posit that the disorder is related to a breakdown in the formation of the neural motor programs for speech early in development and that this breakdown is maintained throughout life. To date, no study has examined the potential neurodevelopmental signatures of the disorder across pediatric and adult populations. The current study aimed to fill this gap in our knowledge. We hypothesized that the developmental trajectory of cortical thickness in people who stutter would differ across the lifespan in the left pars opercularis relative to a group of control participants. We collected structural magnetic resonance images from 116 males (55 people who stutter) ranging in age from 6 to 48 years old. Differences in cortical thickness across ages and between patients and controls were investigated in 30 brain regions previously implicated in speech-motor control. An interaction between age and group was found for the left pars opercularis only. In people who stutter, the pars opercularis did not demonstrate the typical maturational pattern of gradual gray matter thinning with age across the lifespan that we observed in control participants. In contrast, the developmental trajectory of gray matter thickness in other regions of interest within the neural network for speech-motor control was similar for both groups. Our findings indicate that the developmental trajectory of gray matter in left pars opercularis is abnormal in people who stutter.
Introduction
Stuttering is a complex developmental communication disorder affecting 1% of the population (Yairi and Ambrose, 1999; Boyle et al., 2011). Although there have been recent advances in our understanding of the genetic and neural influences on stuttering, the etiology and pathophysiology of the disorder remain poorly defined. The speech characteristics associated with the disorder are proposed to be the result of an aberrant brain mechanism caused by the interaction of genetic and environmental variables (De Nil, 1999; Ludlow and Loucks, 2003; Bohland et al., 2014). These characteristics, namely speech sound repetitions, prolongations and silent blocks, typically first present in affected children between the ages of 18 and 60 months (Yairi and Ambrose, 1999). Although 74% of children recover from stuttering within 4 years of onset, 26% persist to stutter over their lifespan and experience a resultant negative impact on their quality of life (Yairi and Ambrose, 1999; Craig et al., 2009).
Magnetic resonance imaging studies of brain structure in children and adults who stutter have begun to elucidate the role of neuroanatomical abnormalities in the disorder. Importantly, children and adults who stutter do not demonstrate gross abnormalities in total brain volume or overall gray matter, white matter or cerebral spinal fluid volumes (Beal et al., 2007, 2013; Chang and Zhu, 2013). Rather, the observed abnormalities in gray and white matter are regional in nature and, for the most part, localized to the parasylvian and subcortical regions known to be important for speech perception and production (Sommer et al., 2002; Jäncke et al., 2004; Beal et al., 2007, 2013; Chang et al., 2008; Watkins et al., 2008; Chang and Zhu, 2013).
A common finding that is emerging from the developmental stuttering literature is the repeated implication in the disorder of microstructural abnormalities in the gray and white matter regions that comprise Broca’s area. Broca’s area is defined, via cytoarchitectonic mapping, as Brodmann’s areas (BA) 44 and 45. BA44 and 45 are relatively synonymous with the pars opercularis and pars triangularis of the left hemisphere which are labeled via the cortical folding nomenclature (Amunts et al., 1999; Lindenberg et al., 2007; Keller et al., 2009; Amunts and Zilles, 2012; Lemaire et al., 2013). Modern models of the neural network for speech production posit that neurons in the left pars opercularis code for speech sounds and that the region is critically important for both speech acquisition and mature speech production (Guenther et al., 1998; Hickok et al., 2011; Guenther and Vladusich, 2012; Hickok, 2012). Neural representations of speech sounds and their sensorimotor processes are likely acquired via the support of a bilateral, but left hemisphere biased, neural network that is shared for speech perception and production (Guenther, 1995; Bailly, 1997; Guenther and Vladusich, 2012). The sensory component of the sensorimotor network is proposed to map acoustic speech signals to articulatory representations (Hickok and Poeppel, 2004, 2007; Guenther, 2006; Tourville et al., 2008). The major anatomical structures that comprise this afferent pathway are the primary auditory cortex, temporoparietal junction and pars opercularis of the inferior frontal gyrus. The motor component of the sensorimotor network is comprised of the pars opercularis, premotor and primary motor cortices and the subsequent cortico-spinal tracts. As such it is unsurprising that various studies have identified neuroanatomic abnormalities in these regions in people who stutter with the relatively consistent inclusion of the left pars opercularis of Broca’s area.
Of recent interest are the findings of neuroanatomic abnormalties in young children who stutter as they provide a window into brain structure much closer in time to the onset of the disorder than the studies focused on adults alone. Children who stutter have been shown to have less gray matter volume in the left and right inferior frontal gyri relative to controls (Chang et al., 2008; Beal et al., 2013). Specifically, in the left inferior frontal gyrus children who stutter are known to have less gray matter volume in the pars opercularis, pars triangularis and pars orbitalis. In the right inferior frontal gyrus children who stutter have less gray matter volume in the pars opercularis and pars triangularis. Beal et al. (2013) found that gray matter volume in the right pars triangularis and opercularis was negatively correlated with stuttering severity supporting the premise that more typical development in these regions aids compensation for stuttered speech. Children who stutter as young as 3 years of age have been shown to have less structural connectivity of white matter originating in the region of the left pars opercularis that link it to the left posterior temporal areas via the insula, putamen and extreme capsule as well as less connectivity between the right pars opercularis and the putamen (Chang and Zhu, 2013). The finding of less structural connectivity between the left pars opercularis and other regions of the neural network for speech production, including sensory regions, is consistent with previous studies of white matter properties of both children and adults who stutter that reported lesser fractional anisotropy (FA) values in white matter generally along the superior longitudinal fasciculus underlying the broader gray matter regions proximate to, and including, the left pars opercularis (Sommer et al., 2002; Chang et al., 2008; Watkins et al., 2008; Cykowski et al., 2010). Furthermore, the white matter underlying regions adjacent to the left pars opercularis, namely the left ventral premotor and middle primary motor cortices, has been noted to be less well connected to other speech relevant brain regions in adults who stutter vs. controls (Cai et al., 2014b; Connally et al., 2014).
There is a relatively larger literature that has examined adults who stutter and found that they too present with neuroanatomic abnormalities in parasylvian regions as compared to controls with regards to regional gray matter volume as measured by voxel-based morphometry. However, the directionality of the results reported in the literature is inconsistent. Specifically, adults who stutter have been found to have more gray matter volume in the left inferior frontal gyrus as well as the bilateral pre and post central gyri, superior temporal gyri, middle temporal gyri, basal ganglia and cerebellum (Beal et al., 2007; Lu et al., 2010). Conversely, adults who stutter have also been found to have less gray matter volume in the left inferior frontal gyrus, left superior frontal gyrus as well as the bilateral middle frontal gyri, cerebellar posterior lobes, dorsal part of medulla and the cerebellar tonsil (Kell et al., 2009; Lu et al., 2010). Interestingly, not all studies have found differences in gray matter volume in adults who stutter (Jäncke et al., 2004). Further study of the neuroanatomy of adults who stutter is needed to clarify just how different the cortical structures pertinent to speech acquisition and production are relative to controls.
A number of parasylvian brain regions have been implicated in stuttering but it has been difficult to determine which regions are critical to the development of the disorder. Based on the common finding of gray and white matter structural abnormalities in and around the left pars opercularis, many authors have proposed that developmental stuttering is the result of a breakdown in motor control of articulation at the cortical level around this region (Sommer et al., 2002; Max et al., 2004; Brown et al., 2005; Watkins et al., 2008; Kell et al., 2009). Specifically, some researchers have proposed that stuttering may result from a failure to correctly form the neural representations of speech sounds during development due to difficulties with the integration of auditory or somatosensory information with said representations (Neilson and Neilson, 1987; Max et al., 2004; Corbera et al., 2005; Weber-Fox et al., 2008; Beal et al., 2013). In typically developing children between 5–12 years of age, the gray matter comprising the left inferior frontal gyrus and bilateral posterior superior temporal gyri continues to thicken over this short time span when the majority of other brain regions have started a processes of thinning (Sowell et al., 2004). This unique pattern of development suggests that there may be a special relationship between gray matter maturation and the mastery of speech-motor skills over this age span. Although the maturation of speech and language regions appears to be protracted relative to other brain regions, the overall pattern across adulthood is one of gradual gray matter thinning (Sowell et al., 2003) Despite the fact that stuttering is often a lifelong affliction, we are unaware of any study that has examined the potential neurodevelopmental signatures of the disorder across a pediatric and adult population of people who stutter. The current study aimed to fill this gap in the knowledge base and provide further insight into the regions that may be critically important for developmental stuttering by examining the trajectory of neurodevelopment in various speech relevant regions including Broca’s area as defined by the pars oeprcularis and pars triangularis.
Our investigation of the interaction of aging and group effects for cortical thickness aimed to identify regions that are problematic in their development over the lifespan in people who stutter. To describe the developmental differences in gray matter thickness in these regions we conducted a mega-analysis of a large cohort of children and adults who stutter utilizing magnetic resonance images of the brain collected across various smaller studies. If the onset and maintenance of stuttering is related to deficient neural resources for establishing the internal representations of speech-motor programs associated with the pars opercularis than neurodevelopment of this region should be implicated in the disorder. Based on our above review of the literature, we hypothesized that the developmental trajectory of cortical thickness in the left pars opercularis would be abnormal across the lifespan in people who stutter. To investigate whether or not development of this region played a key role in the disorder we tested the developmental trajectory of a total of 30 parasylvian regions known to contribute to the sensory-motor interactions that are associated with speech acquisition.
Materials and Methods
Participants
Participants were 116 right-handed males with English as their primary language and a normal developmental and medical history. The 55 boys and men with persistent developmental stuttering ranged in age from 7 to 47 years (mean = 26.07, median = 27, s.d. = 10.79) and the 61 matched control participants from 6 to 48 years (mean = 26.79, median = 27, s.d. = 10.10). The two groups did not differ in age (t(110) = 0.37 p = 0.71). The Stuttering Severity Index—3rd Edition was used to measure stuttering severity in the patient group. Stuttering severity ranged from very mild (5) to very severe (49) (mean = 21.80, median = 21, s.d. = 8.92). One patient’s stuttering severity score was not available due to technical difficulty.
MRI Acquisition
T1 images for 51 participants (23 people who stutter) were collected on a 1.5-T MRI system (GE Medical Systems, Milwaukee, Wisconsin, USA) using a standard quadrature head coil at the Toronto Western Hospital in Toronto, Ontario, Canada. A T1-weighted three-dimensional inversion recovery-prepared fast spoiled gradient echo (FSPGR) sequence (flip angle = 20 degrees, echo time (TE) = 5.2 ms, repetition time (TR) = 12 ms, preparation time = 300 ms) was used to generate 124 1.5 mm-thick sagittal slices (256 × 256 matrix). T1 images for 47 participants (23 people who stutter) were collected on a 1.5-T MRI system at the Hospital for Sick Children in Toronto, Ontario, Canada. A 1.5-T Signa Excite HD 12.0 MRI System (GE Medical Systems, Milwaukee, Wisconsin, USA) with an 8-channel head coil was used to obtain neuroantomic images. A T1-weighted 3D FSPGR sequence (flip angle = 9 degrees, TE = 4.2 ms, TR = 9 ms) was used to generate 114 1.5 mm-thick axial slices (256 × 192 matrix, 24 cm field of view). Pixels were squared post processing ultimately resulting in a 256 × 256 viewing matrix. The images for the remaining 18 participants (9 people who stutter) were collected on a 3-T MRI system (Siemens Medical Solutions) using an 8-channel head coil at the Magnetic Resonance Research Center at Yale University in New Haven, Connecticut, USA. A T1-weighted three-dimensional MPRAGE sequence (TE = 3.7 ms, TR = 2.5 ms) was used to generate 176 1 mm-thick sagittal slices (256 × 256 matrix).
MRI Preprocessing
T1 images were registered to the ICBM 152 template with a 12-parameter linear transformation (Collins et al., 1994), RF inhomogeneity corrected (Sled and Pike, 1998), skull stripped (Smith, 2002), tissue classified (Zijdenbos et al., 2002) and tissue partial volume estimated (Tohka et al., 2004). Deformable models were used to fit the white matter surface for each hemisphere separately, followed by an expansion outward to find the gray matter/CSF intersection (MacDonald et al., 2000; Kim et al., 2005) resulting in 4 surfaces of 41,962 polygons each. From these surfaces the distance between the white and gray surfaces in native space was used to measure cortical thickness (Lerch and Evans, 2005). The individual surfaces were non-linearly aligned to the ICBM 152 template using surface-based registration techniques (Robbins et al., 2004). All analyses were performed using native cortical thickness. The preprocessing steps are depicted in Figure 1.
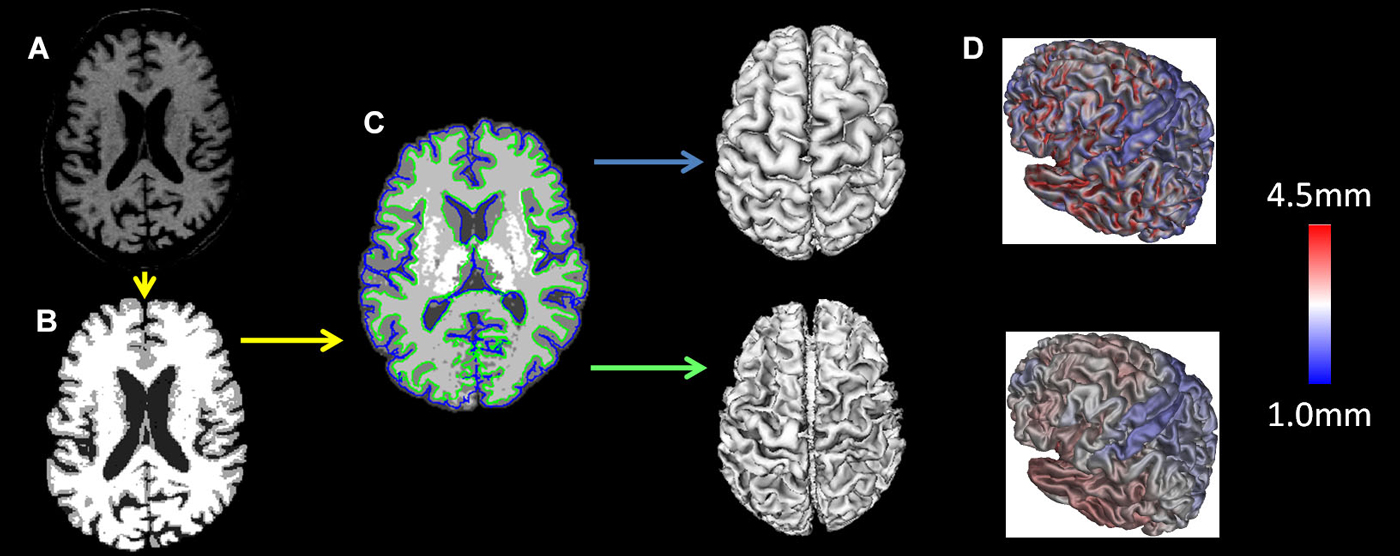
Figure 1. MRI preprocessing: the T1 images were (A) registered to the ICBM 152 template with a 12-parameter linear transformation, (B) RF inhomogeneity corrected and skull stripped, (C) tissue segmented and gray and white matter surfaces created and (D) tissue partial volumes estimated. Cortical thickness was measured as the distance between the white and gray surfaces in native space.
Region of Interest Analysis
Differences in cortical thickness across ages between people who stutter and controls were investigated in 30 specific brain regions of interest (ROI), separately for each hemisphere, based on our hypothesis. The ROIs are depicted in Figure 2 and consisted of the following in each hemisphere: pars opercularis (BA44), pars triangularis (BA45), pars orbitalis (BA47), ventral and dorsal premotor cortex, ventral and dorsal primary motor cortex, ventral and dorsal sensorimotor cortex, anterior and posterior angular gyrus, anterior and posterior superior temporal sulcus and anterior and posterior superior temporal gyrus. Using the average gray surface of all participants the ROI delineations were back projected onto the individual surfaces using the surface transformation and the average ROI thickness computed for each individual. For each ROI a linear mixed effect model was calculated with the intercept allowed to vary between scanners to correct for possible scanner effects and a False Discovery Rate was used (Genovese et al., 2002).
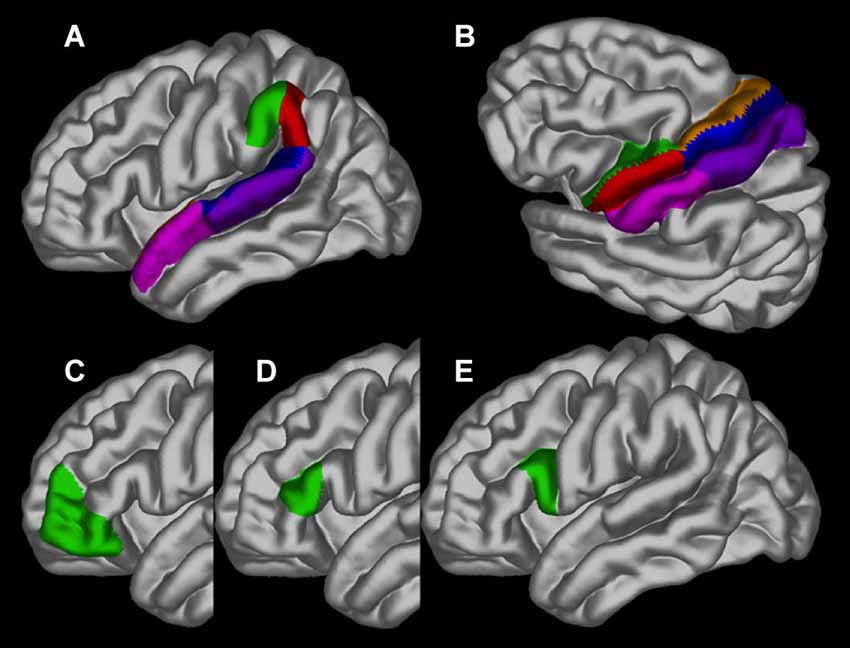
Figure 2. The regions of interest depicted on the gray matter surface of the left hemisphere: (A) the anterior (green) and posterior (red) angular gyrus, anterior (pink) and posterior (blue) superior temporal sulcus and anterior (dark pink) and posterior (blue) superior temporal gyrus; (B) ventral (green) and dorsal (brown) premotor cortex, ventral (red) and dorsal (blue) primary motor cortex and ventral (pink) and dorsal (purple) sensorimotor cortex; (C) pars orbitalis (BA47); (D) pars triangularis (BA45) and (E). pars opercularis (BA44).
Results
The results of the ROI statistical analyses are shown in Table 1. As expected, there was a main effect for age in nearly every ROI. Only the left anterior superior temporal gyrus and right anterior superior temporal sulcus did not demonstrate an effect for age. As can be seen in Figure 3, gray matter cortex was thickest in childhood and then thinned with age in nearly every ROI. There was no main effect for group in any of the ROIs. A significant interaction between age and group was found for the left pars opercularis (F = 10.63, p = 0.001, q = 0.04). Gray matter in this region was thinner in the younger people who stutter relative to their same aged peers but this association inversed with age such that gray matter was thicker in older people who stutter relative to their same age peers. By contrast, the developmental trajectory of thickness for the other ROIs was similar for both groups and no statistically significant interactions were identified.
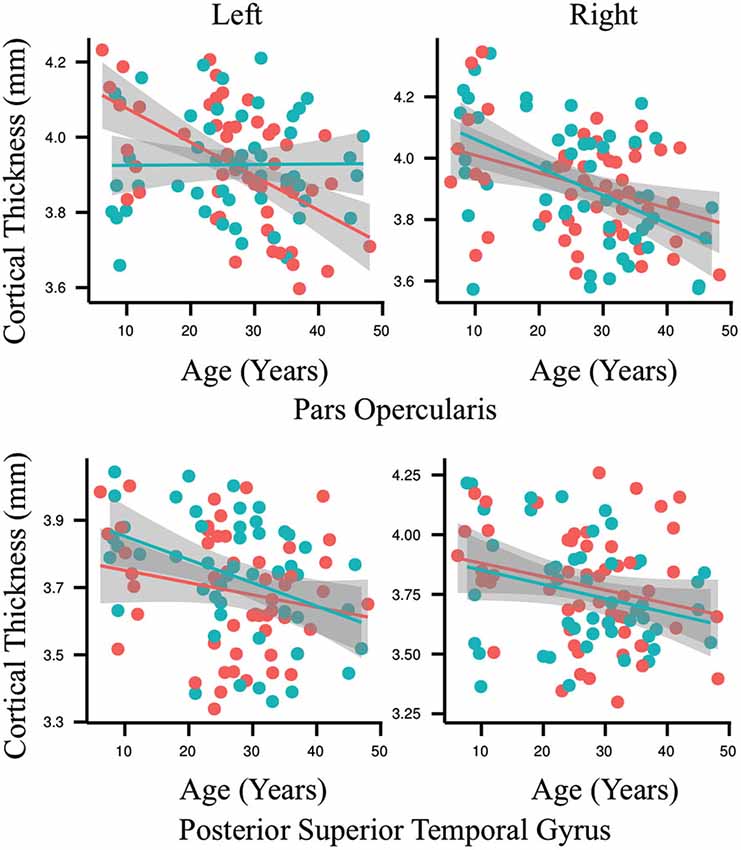
Figure 3. Each graph depicts the association between age (6–49 years old) and cortical thickness (millimeters) for an exemplary region interest. Results for the people who stutter are shown in red and for the control group in blue. Regression lines are included and circles indicate individual measurements. The only significant interaction between age and group for cortical thickness was found in the left pars opercularis (p = 0.001, q = 0.04). By contrast, the developmental trajectory of thickness for all of the other ROIs was similar for both groups and no statistically significant differences were identified.
Discussion
Our cross-sectional study is the first to examine the maturational time course of cortical gray matter thickness associated with persistent developmental stuttering across the lifespan in a large cohort of patients. Our data indicate that the developmental trajectory of gray matter thickness in Broca’s area, specifically the left pars opercularis, is abnormal in people who stutter. Within the group of people who stutter, the gray matter in the left pars opercularis did not demonstrate the typical linear maturational pattern of gradual life-long thinning associated with the controls but rather was unchanging across the lifespan. No other regional group differences were identified. Our findings suggest that the neuroanatomic microstructure of the left pars opercularis is crucially important for the development of fluent speech-motor control and that deviations in the development of this region are associated with the onset and persistence of stuttered speech.
The acquisition of speech-motor control spans the course of infant, child and adolescent development (Green et al., 2002; Walsh and Smith, 2002; Smith and Zelaznik, 2004; Nip et al., 2009). Modern models of speech production assert that the pars opercularis is a critical node in the neural network for speech learning (Guenther et al., 1998; Bohland and Guenther, 2006; Hickok and Poeppel, 2007; Hickok et al., 2011; Guenther and Vladusich, 2012; Hickok, 2012). The neurons within the left pars opercularis are active when listening to and producing speech sounds suggesting that they both are crucial to acquiring and maintaining the neural representations of speech-motor programs associated with this region (Devlin and Watkins, 2007; Möttönen et al., 2013). This assertion is supported by a recent meta-analysis of functional neuroimaging experiments that found that imitation and speech have a common neural basis within Broca’s area with an emphasis on the pars opercularis (Kühn et al., 2013). The flat and unchanging linear pattern of gray matter maturation in the left pars opercularis in people who stutter may be reflective of a failure of neural proliferation and dendritic expansion that is critical to support the acquisition and mastery of speech-motor control at a crucial period of childhood speech development. This appears to be followed by a failure, in adolescence and adulthood, of the synaptic pruning process that results in an inefficient neural organization of the resources in this region that are necessary for masterful and fluent speech production. It is particularly interesting that natural recovery from stuttering is very rare once adolescence is reached and that the closing of a window for natural recovery overlaps with this key period where pruning of cortical gray matter, in typically developing adolescents and adults, leads to improved efficiency of networks of neural regions for various tasks.
Our observed abnormal developmental pattern may contribute to the chronic nature of stuttering, as adult-like efficiency of neural processing in the pars opercularis is not attained with maturation. There is an emerging literature of behavioral evidence to support this assertion. Children who stutter as young as 4-years-old are known to have abnormal speech-planning and execution skills as evidenced by higher variability in oral-motor coordination relative to typically developing peers (Smith et al., 2012). People who stutter continue to show higher variability in speech-motor movements than controls in adulthood (Kleinow and Smith, 2000; Smith and Kleinow, 2000). Adults who stutter also have difficulty learning speech-motor, as well as non-speech motor, sequences compared to controls (Smits-Bandstra et al., 2006) and adapt poorly to perturbations in auditory and tactile sensory feedback important for online speech-motor control (Neilson and Neilson, 1987; Loucks and De Nil, 2006a,b; Loucks et al., 2007; Namasivayam and van Lieshout, 2008; Cai et al., 2012, 2014a).
Importantly, there is a precedent for associating changes in cortical gray matter development with speech-motor and language related behaviors. The pars opercularis and posterior superior temporal gyrus show protracted development relative to other brain regions over the school-age years suggesting the importance of continued cortical maturation in these areas for speech-motor and language development during this period (Sowell et al., 2004). The thinning of frontal gray matter during childhood is correlated with improved verbal learning between the ages of 7–16 years (Sowell et al., 2001). Furthermore, reductions of gray matter density in the left dorsolateral frontal and lateral parietal regions during adolescence are correlated with improved vocabulary scores and better performance during verbal learning tasks (Lee et al., 2007). Improved motor skills are also associated with thinning in more dorsal regions of frontal cortex (Lu et al., 2007). Preschool children with apraxia of speech demonstrated thicker left supramarginal gyri than controls and a thinning of the left posterior superior temporal gyrus over a short course of speech therapy (Kadis et al., 2014). As such, gray matter maturation is important for normal speech, language and motor skill development.
Our control data demonstrate the typical pattern of gray matter development defined by thicker gray matter cortex in pre-adolescent followed by a steady and consistent post-adolescent decrease across the majority of neural regions for the remainder of life (Figure 3, red; Sowell et al., 2003, 2004). Early in childhood, progressive gray matter growth observed via MRI is attributed to neural proliferation and dendritic expansion. As the brain matures new connections are made between neurons to facilitate development, for example, the acquisition of motor skills (Draganski et al., 2004, 2006). In contrast to the rapid cortical thickening that occurs in childhood, starting in early adolescence maturation leads to thinning of gray matter by way of synaptic pruning (Sowell et al., 2001). Unlike dendritic expansion, synaptic pruning is a regressive process that creates a more efficient neural structure through the reduction or elimination of potentially unnecessary neural connections and cells. This removal of weaker or neglected cells and connections is thought to improve the efficiency of the remaining neuronal signals. Thinning of the cortex continues to some degree for the remainder of the lifespan and this pattern is observable in our control data (Figure 3, red). The people who stutter also demonstrate this pattern in all of the speech ROI examined except for the left pars opercularis (Figure 3, blue).
The identification of a developmental pathology within the left pars opercularis in people who stutter is consistent with the existing structural and functional neuroimaging literature and modern hypotheses of stuttering onset and persistence. At this time it is impossible to determine if the deficient development of gray matter within the left pars opercularis is causal or the result of the repeatedly documented limited white matter connectivity that this region has with critical nodes within the neural network for speech production in both children and adults who persistent phenotypes of the disorder (Sommer et al., 2002; Chang et al., 2008, 2011; Cykowski et al., 2010; Chang and Zhu, 2013). It is reasonable to suggest that these structural abnormalities in Broca’s area are related to the deficient neural activity observed during speech in both children and adults who stutter. Lesser brain activity has been noted in the left pars opercularis and overlapping the white matter pathways found to be deficient in adults who stutter (Watkins et al., 2008). Furthermore, children and adults who stutter have been noted to have neural timing and sequencing errors directly related to functioning of the left pars opercularis and regions known to be closely connected to it, such as the superior temporal gyrus, relative to controls during speech processing tasks as measured by magnetoencephalography (Salmelin et al., 2000; Beal et al., 2010, 2011). Various functional magnetic resonance and positron emission studies have shown that adults who stutter have increased neural activity in the right hemisphere homologues of parasylvian regions during speech relative to controls (Fox et al., 1996; Brown et al., 2005; De Nil et al., 2008) and that this activity is normalized with treatment and recovery (De Nil et al., 2003; Neumann et al., 2005; Kell et al., 2009). Many authors have attributed these observations to neural compensation for a left inferior frontal deficit (De Nil, 1999; Ludlow and Loucks, 2003; Brown et al., 2005; Kell et al., 2009) that results in faulty neural motor commands for speech production (Max et al., 2004; Civier et al., 2010). Our data demonstrate, for the first time, a lifelong microstructural deficit in the left pars opercularis in a large sample of people with persistent developmental stuttering thereby lending credence to the notion that it plays a role in the cause of the disorder and that right hemisphere biased brain activity noted in adults who stutter is indeed compensatory. Future studies examining both gray and white matter development in childhood stuttering should employ a large cohort followed longitudinally over the seemingly critical age range for speech-motor development.
Conflict of Interest Statement
The authors declare that the research was conducted in the absence of any commercial or financial relationships that could be construed as a potential conflict of interest.
Acknowledgments
Financial support for this project was provided by the Canadian Institutes of Health Research Operating Grant MOP-68969 and the National Institute on Deafness and Other Communication Disorders Grant R01-DC-007603. Financial support to the first author was provided by the Clinician-Scientist Training Program at the Hospital for Sick Children Training Centre. The authors wish to thank Jurgen Germann for his assistance with preliminary data analyses, Adrian Crawley for his assistance with data acquisition and Robert Kroll for his help with participant recruitment.
References
Amunts, K., Schleicher, A., Bürgel, U., Mohlberg, H., Uylings, H. B., and Zilles, K. (1999). Broca’s region revisited: cytoarchitecture and intersubject variability. J. Comp. Neurol. 412, 319–341. doi: 10.1002/(sici)1096-9861(19990920)412:2<319::aid-cne10>3.0.co;2-7
Pubmed Abstract | Pubmed Full Text | CrossRef Full Text | Google Scholar
Amunts, K., and Zilles, K. (2012). Architecture and organizational principles of Broca’s region. Trends Cogn. Sci. 16, 418–426. doi: 10.1016/j.tics.2012.06.005
Pubmed Abstract | Pubmed Full Text | CrossRef Full Text | Google Scholar
Bailly, G. (1997). Learning to speak. Sensori-motor control of speech movements. Speech Commun. 22, 251–267. doi: 10.1016/s0167-6393(97)00025-3
Beal, D. S., Cheyne, D. O., Gracco, V. L., Quraan, M. A., Taylor, M. J., and De Nil, L. F. (2010). Auditory evoked fields to vocalization during passive listening and active generation in adults who stutter. Neuroimage 52, 1645–1653. doi: 10.1016/j.neuroimage.2010.04.277
Pubmed Abstract | Pubmed Full Text | CrossRef Full Text | Google Scholar
Beal, D. S., Gracco, V. L., Brettschneider, J., Kroll, R. M., and De Nil, L. F. (2013). A voxel-based morphometry (VBM) analysis of regional grey and white matter volume abnormalities within the speech production network of children who stutter. Cortex 49, 2151–2161. doi: 10.1016/j.cortex.2012.08.013
Pubmed Abstract | Pubmed Full Text | CrossRef Full Text | Google Scholar
Beal, D. S., Gracco, V. L., Lafaille, S. J., and De Nil, L. F. (2007). Voxel-based morphometry of auditory and speech-related cortex in stutterers. Neuroreport 18, 1257–1260. doi: 10.1097/wnr.0b013e3282202c4d
Pubmed Abstract | Pubmed Full Text | CrossRef Full Text | Google Scholar
Beal, D. S., Quraan, M. A., Cheyne, D. O., Taylor, M. J., Gracco, V. L., and De Nil, L. F. (2011). Speech-induced suppression of evoked auditory fields in children who stutter. Neuroimage 54, 2994–3003. doi: 10.1016/j.neuroimage.2010.11.026
Pubmed Abstract | Pubmed Full Text | CrossRef Full Text | Google Scholar
Bohland, J. W., and Guenther, F. H. (2006). An fMRI investigation of syllable sequence production. Neuroimage 32, 821–841. doi: 10.1016/j.neuroimage.2006.04.173
Pubmed Abstract | Pubmed Full Text | CrossRef Full Text | Google Scholar
Bohland, J. W., Myers, E. M., and Kim, E. (2014). An informatics approach to integrating genetic and neurological data in speech and language neuroscience. Neuroinformatics 12, 39–62. doi: 10.1007/s12021-013-9201-6
Pubmed Abstract | Pubmed Full Text | CrossRef Full Text | Google Scholar
Boyle, C. A., Boulet, S., Schieve, L. A., Cohen, R. A., Blumberg, S. J., Yeargin-Allsopp, M., et al. (2011). Trends in the prevalence of developmental disabilities in US children, 1997–2008. Pediatrics 127, 1034–1042. doi: 10.1542/peds.2010-2989
Pubmed Abstract | Pubmed Full Text | CrossRef Full Text | Google Scholar
Brown, S., Ingham, R. J., Ingham, J. C., Laird, A. R., and Fox, P. T. (2005). Stuttered and fluent speech production: an ALE meta-analysis of functional neuroimaging studies. Hum. Brain Mapp. 25, 105–117. doi: 10.1002/hbm.20140
Pubmed Abstract | Pubmed Full Text | CrossRef Full Text | Google Scholar
Cai, S., Beal, D. S., Ghosh, S. S., Guenther, F. H., and Perkell, J. S. (2014a). Impaired timing adjustments in response to time-varying auditory perturbation during connected speech production in persons who stutter. Brain Lang. 129, 24–29. doi: 10.1016/j.bandl.2014.01.002
Pubmed Abstract | Pubmed Full Text | CrossRef Full Text | Google Scholar
Cai, S., Beal, D. S., Ghosh, S. S., Tiede, M. K., Guenther, F. H., and Perkell, J. S. (2012). Weak responses to auditory feedback perturbation during articulation in persons who stutter: evidence for abnormal auditory-motor transformation. PLoS One 7:e41830. doi: 10.1371/journal.pone.0041830
Pubmed Abstract | Pubmed Full Text | CrossRef Full Text | Google Scholar
Cai, S., Tourville, J. A., Beal, D. S., Perkell, J. S., Guenther, F. H., and Ghosh, S. S. (2014b). Diffusion imaging of cerebral white matter in persons who stutter: evidence for network-level anomalies. Front. Hum. Neurosci. 8:54. doi: 10.3389/fnhum.2014.00054
Pubmed Abstract | Pubmed Full Text | CrossRef Full Text | Google Scholar
Chang, S. E., Erickson, K. I., Ambrose, N. G., Hasegawa-Johnson, M. A., and Ludlow, C. L. (2008). Brain anatomy differences in childhood stuttering. Neuroimage 39, 1333–1344. doi: 10.1016/j.neuroimage.2007.09.067
Pubmed Abstract | Pubmed Full Text | CrossRef Full Text | Google Scholar
Chang, S. E., Horwitz, B., Ostuni, J., Reynolds, R., and Ludlow, C. L. (2011). Evidence of left inferior frontal-premotor structural and functional connectivity deficits in adults who stutter. Cereb. Cortex 21, 2507–2518. doi: 10.1093/cercor/bhr028
Pubmed Abstract | Pubmed Full Text | CrossRef Full Text | Google Scholar
Chang, S. E., and Zhu, D. C. (2013). Neural network connectivity differences in children who stutter. Brain 136, 3709–3726. doi: 10.1093/brain/awt275
Pubmed Abstract | Pubmed Full Text | CrossRef Full Text | Google Scholar
Civier, O., Tasko, S. M., and Guenther, F. H. (2010). Overreliance on auditory feedback may lead to sound/syllable repetitions: simulations of stuttering and fluency-inducing conditions with a neural model of speech production. J. Fluency Disord. 35, 246–279. doi: 10.1016/j.jfludis.2010.05.002
Pubmed Abstract | Pubmed Full Text | CrossRef Full Text | Google Scholar
Collins, D. L., Neelin, P., Peters, T. M., and Evans, A. C. (1994). Automatic 3D intersubject registration of MR volumetric data in standardized Talairach space. J. Comput. Assist. Tomogr. 18, 192–205. doi: 10.1097/00004728-199403000-00005
Pubmed Abstract | Pubmed Full Text | CrossRef Full Text | Google Scholar
Connally, E. L., Ward, D., Howell, P., and Watkins, K. E. (2014). Disrupted white matter in language and motor tracts in developmental stuttering. Brain Lang. 131, 25–35. doi: 10.1016/j.bandl.2013.05.013
Pubmed Abstract | Pubmed Full Text | CrossRef Full Text | Google Scholar
Corbera, S., Corral, M. J., Escera, C., and Idiazábal, M. A. (2005). Abnormal speech sound representation in persistent developmental stuttering. Neurology 65, 1246–1252. doi: 10.1212/01.wnl.0000180969.03719.81
Pubmed Abstract | Pubmed Full Text | CrossRef Full Text | Google Scholar
Craig, A., Blumgart, E., and Tran, Y. (2009). The impact of stuttering on the quality of life in adults who stutter. J. Fluency Disord. 34, 61–71. doi: 10.1016/j.jfludis.2009.05.002
Pubmed Abstract | Pubmed Full Text | CrossRef Full Text | Google Scholar
Cykowski, M. D., Fox, P. T., Ingham, R. J., Ingham, J. C., and Robin, D. A. (2010). A study of the reproducibility and etiology of diffusion anisotropy differences in developmental stuttering: a potential role for impaired myelination. Neuroimage 52, 1495–1504. doi: 10.1016/j.neuroimage.2010.05.011
Pubmed Abstract | Pubmed Full Text | CrossRef Full Text | Google Scholar
De Nil, L. (1999). “Stuttering: a neurophysiological perspective,” in Stuttering Research and Practice: Bridging the Gap (Mahwah, NJ: Erlbaum), 85–102.
De Nil, L. F., Beal, D. S., Lafaille, S. J., Kroll, R. M., Crawley, A. P., and Gracco, V. L. (2008). The effects of simulated stuttering and prolonged speech on the neural activation patterns of stuttering and nonstuttering adults. Brain Lang. 107, 114–123. doi: 10.1016/j.bandl.2008.07.003
Pubmed Abstract | Pubmed Full Text | CrossRef Full Text | Google Scholar
De Nil, L. F., Kroll, R. M., Lafaille, S. J., and Houle, S. (2003). A positron emission tomography study of short- and long-term treatment effects on functional brain activation in adults who stutter. J. Fluency Disord. 28, 357–380. doi: 10.1016/j.jfludis.2003.07.002
Pubmed Abstract | Pubmed Full Text | CrossRef Full Text | Google Scholar
Devlin, J. T., and Watkins, K. E. (2007). Stimulating language: insights from TMS. Brain 130, 610–622. doi: 10.1093/brain/awl331
Pubmed Abstract | Pubmed Full Text | CrossRef Full Text | Google Scholar
Draganski, B., Gaser, C., Busch, V., Schuierer, G., Bogdahn, U., and May, A. (2004). Neuroplasticity: changes in grey matter induced by training. Nature 427, 311–312. doi: 10.1038/427311a
Pubmed Abstract | Pubmed Full Text | CrossRef Full Text | Google Scholar
Draganski, B., Gaser, C., Kempermann, G., Kuhn, H. G., Winkler, J., Büchel, C., et al. (2006). Temporal and spatial dynamics of brain structure changes during extensive learning. J. Neurosci. 26, 6314–6317. doi: 10.1523/jneurosci.4628-05.2006
Pubmed Abstract | Pubmed Full Text | CrossRef Full Text | Google Scholar
Fox, P. T., Ingham, R. J., Ingham, J. C., Hirsch, T. B., Downs, J. H., Martin, C., et al. (1996). A PET study of the neural systems of stuttering. Nature 382, 158–161. doi: 10.1038/382158a0
Pubmed Abstract | Pubmed Full Text | CrossRef Full Text | Google Scholar
Genovese, C. R., Lazar, N. A., and Nichols, T. (2002). Thresholding of statistical maps in functional neuroimaging using the false discovery rate. Neuroimage 15, 870–878. doi: 10.1006/nimg.2001.1037
Pubmed Abstract | Pubmed Full Text | CrossRef Full Text | Google Scholar
Green, J. R., Moore, C. A., and Reilly, K. J. (2002). The sequential development of jaw and lip control for speech. J. Speech Lang. Hear. Res. 45, 66–79. doi: 10.1044/1092-4388(2002/005)
Pubmed Abstract | Pubmed Full Text | CrossRef Full Text | Google Scholar
Guenther, F. H. (1995). Speech sound acquisition, coarticulation, and rate effects in a neural network model of speech production. Psychol. Rev. 102, 594–621. doi: 10.1037/0033-295x.102.3.594
Pubmed Abstract | Pubmed Full Text | CrossRef Full Text | Google Scholar
Guenther, F. H. (2006). Cortical interactions underlying the production of speech sounds. J. Commun. Disord. 39, 350–365. doi: 10.1016/j.jcomdis.2006.06.013
Pubmed Abstract | Pubmed Full Text | CrossRef Full Text | Google Scholar
Guenther, F. H., Hampson, M., and Johnson, D. (1998). A theoretical investigation of reference frames for the planning of speech movements. Psychol. Rev. 105, 611–633. doi: 10.1037/0033-295x.105.4.611-633
Pubmed Abstract | Pubmed Full Text | CrossRef Full Text | Google Scholar
Guenther, F. H., and Vladusich, T. (2012). A neural theory of speech acquisition and production. J. Neurolinguistics 25, 408–422. doi: 10.1016/j.jneuroling.2009.08.006
Pubmed Abstract | Pubmed Full Text | CrossRef Full Text | Google Scholar
Hickok, G. (2012). Computational neuroanatomy of speech production. Nat. Rev. Neurosci. 13, 135–145. doi: 10.1038/nrn3158
Pubmed Abstract | Pubmed Full Text | CrossRef Full Text | Google Scholar
Hickok, G., Houde, J., and Rong, F. (2011). Sensorimotor integration in speech processing: computational basis and neural organization. Neuron 69, 407–422. doi: 10.1016/j.neuron.2011.01.019
Pubmed Abstract | Pubmed Full Text | CrossRef Full Text | Google Scholar
Hickok, G., and Poeppel, D. (2004). Dorsal and ventral streams: a framework for understanding aspects of the functional anatomy of language. Cognition 92, 67–99. doi: 10.1016/j.cognition.2003.10.011
Pubmed Abstract | Pubmed Full Text | CrossRef Full Text | Google Scholar
Hickok, G., and Poeppel, D. (2007). The cortical organization of speech processing. Nat. Rev. Neurosci. 8, 393–402. doi: 10.1038/nrn2113
Pubmed Abstract | Pubmed Full Text | CrossRef Full Text | Google Scholar
Jäncke, L., Hänggi, J., and Steinmetz, H. (2004). Morphological brain differences between adult stutterers and non-stutterers. BMC Neurol. 4:23. doi: 10.1186/1471-2377-4-23
Pubmed Abstract | Pubmed Full Text | CrossRef Full Text | Google Scholar
Kadis, D. S., Goshulak, D., Namasivayam, A., Pukonen, M., Kroll, R., De Nil, L. F., et al. (2014). Cortical thickness in children receiving intensive therapy for idiopathic apraxia of speech. Brain Topogr. 27, 240–247. doi: 10.1007/s10548-013-0308-8
Pubmed Abstract | Pubmed Full Text | CrossRef Full Text | Google Scholar
Kell, C. A., Neumann, K., von Kriegstein, K., Posenenske, C., von Gudenberg, A. W., Euler, H., et al. (2009). How the brain repairs stuttering. Brain 132, 2747–2760. doi: 10.1093/brain/awp185
Pubmed Abstract | Pubmed Full Text | CrossRef Full Text | Google Scholar
Keller, S. S., Crow, T., Foundas, A., Amunts, K., and Roberts, N. (2009). Broca’s area: nomenclature, anatomy, typology and asymmetry. Brain Lang. 109, 29–48. doi: 10.1016/j.bandl.2008.11.005
Pubmed Abstract | Pubmed Full Text | CrossRef Full Text | Google Scholar
Kim, J. S., Singh, V., Lee, J. K., Lerch, J., Ad-Dab’bagh, Y., MacDonald, D., et al. (2005). Automated 3-D extraction and evaluation of the inner and outer cortical surfaces using a Laplacian map and partial volume effect classification. Neuroimage 27, 210–221. doi: 10.1016/j.neuroimage.2005.03.036
Pubmed Abstract | Pubmed Full Text | CrossRef Full Text | Google Scholar
Kleinow, J., and Smith, A. (2000). Influences of length and syntactic complexity on the speech motor stability of the fluent speech of adults who stutter. J. Speech Lang. Hear. Res. 43, 548–559. doi: 10.1044/jslhr.4302.548
Pubmed Abstract | Pubmed Full Text | CrossRef Full Text | Google Scholar
Kühn, S., Brass, M., and Gallinat, J. (2013). Imitation and speech: commonalities within Broca’s area. Brain Struct. Funct. 218, 1419–1427. doi: 10.1007/s00429-012-0467-5
Pubmed Abstract | Pubmed Full Text | CrossRef Full Text | Google Scholar
Lee, H., Devlin, J. T., Shakeshaft, C., Stewart, L. H., Brennan, A., Glensman, J., et al. (2007). Anatomical traces of vocabulary acquisition in the adolescent brain. J. Neurosci. 27, 1184–1189. doi: 10.1523/jneurosci.4442-06.2007
Pubmed Abstract | Pubmed Full Text | CrossRef Full Text | Google Scholar
Lemaire, J. J., Golby, A., Wells, W. M., Pujol, S., Tie, Y., Rigolo, L., et al. (2013). Extended Broca’s area in the functional connectome of language in adults: combined cortical and subcortical single-subject analysis using fMRI and DTI tractography. Brain Topogr. 26, 428–441. doi: 10.1007/s10548-012-0257-7
Pubmed Abstract | Pubmed Full Text | CrossRef Full Text | Google Scholar
Lerch, J. P., and Evans, A. C. (2005). Cortical thickness analysis examined through power analysis and a population simulation. Neuroimage 24, 163–173. doi: 10.1016/j.neuroimage.2004.07.045
Pubmed Abstract | Pubmed Full Text | CrossRef Full Text | Google Scholar
Lindenberg, R., Fangerau, H., and Seitz, R. J. (2007). “Broca’s area” as a collective term? Brain Lang. 102, 22–29. doi: 10.1016/j.bandl.2006.11.012
Pubmed Abstract | Pubmed Full Text | CrossRef Full Text | Google Scholar
Loucks, T. M., and De Nil, L. F. (2006a). Anomalous sensorimotor integration in adults who stutter: a tendon vibration study. Neurosci. Lett. 402, 195–200. doi: 10.1016/j.neulet.2006.04.002
Pubmed Abstract | Pubmed Full Text | CrossRef Full Text | Google Scholar
Loucks, T. M., and De Nil, L. F. (2006b). Oral kinesthetic deficit in adults who stutter: a target-accuracy study. J. Mot. Behav. 38, 238–246. doi: 10.3200/jmbr.38.3.238-247
Pubmed Abstract | Pubmed Full Text | CrossRef Full Text | Google Scholar
Loucks, T. M., De Nil, L. F., and Sasisekaran, J. (2007). Jaw-phonatory coordination in chronic developmental stuttering. J. Commun. Disord. 40, 257–272. doi: 10.1016/j.jcomdis.2006.06.016
Pubmed Abstract | Pubmed Full Text | CrossRef Full Text | Google Scholar
Lu, C., Chen, C., Ning, N., Ding, G., Guo, T., Peng, D., et al. (2010). The neural substrates for atypical planning and execution of word production in stuttering. Exp. Neurol. 221, 146–156. doi: 10.1016/j.expneurol.2009.10.016
Pubmed Abstract | Pubmed Full Text | CrossRef Full Text | Google Scholar
Lu, L., Leonard, C., Thompson, P., Kan, E., Jolley, J., Welcome, S., et al. (2007). Normal developmental changes in inferior frontal gray matter are associated with improvement in phonological processing: a longitudinal MRI analysis. Cereb. Cortex 17, 1092–1099. doi: 10.1093/cercor/bhl019
Pubmed Abstract | Pubmed Full Text | CrossRef Full Text | Google Scholar
Ludlow, C. L., and Loucks, T. (2003). Stuttering: a dynamic motor control disorder. J. Fluency Disord. 28, 273–295. doi: 10.1016/j.jfludis.2003.07.001
Pubmed Abstract | Pubmed Full Text | CrossRef Full Text | Google Scholar
MacDonald, D., Kabani, N., Avis, D., and Evans, A. C. (2000). Automated 3-D extraction of inner and outer surfaces of cerebral cortex from MRI. Neuroimage 12, 340–356. doi: 10.1006/nimg.1999.0534
Pubmed Abstract | Pubmed Full Text | CrossRef Full Text | Google Scholar
Max, L., Guenther, F. H., Gracco, V. L., Ghosh, S. S., and Wallace, M. E. (2004). Unstable or insufficiently activated internal models and feedback-biased motor control as sources of dysfluency: a theoretical model of stuttering. Contemp. Issues Commun. Sci. Disord. 31, 105–122.
Möttönen, R., Dutton, R., and Watkins, K. E. (2013). Auditory-motor processing of speech sounds. Cereb. Cortex 23, 1190–1197. doi: 10.1093/cercor/bhs110
Pubmed Abstract | Pubmed Full Text | CrossRef Full Text | Google Scholar
Namasivayam, A. K., and van Lieshout, P. (2008). Investigating speech motor practice and learning in people who stutter. J. Fluency Disord. 33, 32–51. doi: 10.1016/j.jfludis.2007.11.005
Pubmed Abstract | Pubmed Full Text | CrossRef Full Text | Google Scholar
Neilson, M. D., and Neilson, P. D. (1987). Speech motor control and stuttering: a computational model of adaptive sensory-motor processing. Speech Commun. 6, 325–333. doi: 10.1016/0167-6393(87)90007-0
Neumann, K., Preibisch, C., Euler, H. A., von Gudenberg, A. W., Lanfermann, H., Gall, V., et al. (2005). Cortical plasticity associated with stuttering therapy. J. Fluency Disord. 30, 23–39. doi: 10.1016/j.jfludis.2004.12.002
Pubmed Abstract | Pubmed Full Text | CrossRef Full Text | Google Scholar
Nip, I. S., Green, J. R., and Marx, D. B. (2009). Early speech motor development: cognitive and linguistic considerations. J. Commun. Disord. 42, 286–298. doi: 10.1016/j.jcomdis.2009.03.008
Pubmed Abstract | Pubmed Full Text | CrossRef Full Text | Google Scholar
Robbins, S., Evans, A. C., Collins, D. L., and Whitesides, S. (2004). Tuning and comparing spatial normalization methods. Med. Image Anal. 8, 311–323. doi: 10.1016/j.media.2004.06.009
Pubmed Abstract | Pubmed Full Text | CrossRef Full Text | Google Scholar
Salmelin, R., Schnitzler, A., Schmitz, F., and Freund, H. J. (2000). Single word reading in developmental stutterers and fluent speakers. Brain 123(Pt. 6), 1184–1202. doi: 10.1093/brain/123.6.1184
Pubmed Abstract | Pubmed Full Text | CrossRef Full Text | Google Scholar
Sled, J. G., and Pike, G. B. (1998). Standing-wave and RF penetration artifacts caused by elliptic geometry: an electrodynamic analysis of MRI. IEEE Trans. Med. Imaging 17, 653–662. doi: 10.1109/42.730409
Pubmed Abstract | Pubmed Full Text | CrossRef Full Text | Google Scholar
Smith, S. M. (2002). Fast robust automated brain extraction. Hum. Brain Mapp. 17, 143–155. doi: 10.1002/hbm.10062
Pubmed Abstract | Pubmed Full Text | CrossRef Full Text | Google Scholar
Smith, A., Goffman, L., Sasisekaran, J., and Weber-Fox, C. (2012). Language and motor abilities of preschool children who stutter: evidence from behavioral and kinematic indices of nonword repetition performance. J. Fluency Disord. 37, 344–358. doi: 10.1016/j.jfludis.2012.06.001
Pubmed Abstract | Pubmed Full Text | CrossRef Full Text | Google Scholar
Smith, A., and Kleinow, J. (2000). Kinematic correlates of speaking rate changes in stuttering and normally fluent adults. J. Speech Lang. Hear. Res. 43, 521–536. doi: 10.1044/jslhr.4302.521
Pubmed Abstract | Pubmed Full Text | CrossRef Full Text | Google Scholar
Smith, A., and Zelaznik, H. N. (2004). The development of functional synergies for speech motor coordination in childhood and adolescence. Dev. Psychobiol. 45, 22–33. doi: 10.1002/dev.20009
Pubmed Abstract | Pubmed Full Text | CrossRef Full Text | Google Scholar
Smits-Bandstra, S., De Nil, L. F., and Saint-Cyr, J. A. (2006). Speech and nonspeech sequence skill learning in adults who stutter. J. Fluency Disord. 31, 116–136. doi: 10.1016/j.jfludis.2006.04.003
Pubmed Abstract | Pubmed Full Text | CrossRef Full Text | Google Scholar
Sommer, M., Koch, M. A., Paulus, W., Weiller, C., and Büchel, C. (2002). Disconnection of speech-relevant brain areas in persistent developmental stuttering. Lancet 360, 380–383. doi: 10.1016/s0140-6736(02)09610-1
Pubmed Abstract | Pubmed Full Text | CrossRef Full Text | Google Scholar
Sowell, E. R., Delis, D., Stiles, J., and Jernigan, T. L. (2001). Improved memory functioning and frontal lobe maturation between childhood and adolescence: a structural MRI study. J. Int. Neuropsychol. Soc. 7, 312–322. doi: 10.1017/s135561770173305x
Pubmed Abstract | Pubmed Full Text | CrossRef Full Text | Google Scholar
Sowell, E. R., Peterson, B. S., Thompson, P. M., Welcome, S. E., Henkenius, A. L., and Toga, A. W. (2003). Mapping cortical change across the human life span. Nat. Neurosci. 6, 309–315. doi: 10.1038/nn1008
Pubmed Abstract | Pubmed Full Text | CrossRef Full Text | Google Scholar
Sowell, E. R., Thompson, P. M., Leonard, C. M., Welcome, S. E., Kan, E., and Toga, A. W. (2004). Longitudinal mapping of cortical thickness and brain growth in normal children. J. Neurosci. 24, 8223–8231. doi: 10.1523/jneurosci.1798-04.2004
Pubmed Abstract | Pubmed Full Text | CrossRef Full Text | Google Scholar
Tohka, J., Zijdenbos, A., and Evans, A. (2004). Fast and robust parameter estimation for statistical partial volume models in brain MRI. Neuroimage 23, 84–97. doi: 10.1016/j.neuroimage.2004.05.007
Pubmed Abstract | Pubmed Full Text | CrossRef Full Text | Google Scholar
Tourville, J. A., Reilly, K. J., and Guenther, F. H. (2008). Neural mechanisms underlying auditory feedback control of speech. Neuroimage 39, 1429–1443. doi: 10.1016/j.neuroimage.2007.09.054
Pubmed Abstract | Pubmed Full Text | CrossRef Full Text | Google Scholar
Walsh, B., and Smith, A. (2002). Articulatory movements in adolescents: evidence for protracted development of speech motor control processes. J. Speech Lang. Hear. Res. 45, 1119–1133. doi: 10.1044/1092-4388(2002/090)
Pubmed Abstract | Pubmed Full Text | CrossRef Full Text | Google Scholar
Watkins, K. E., Smith, S. M., Davis, S., and Howell, P. (2008). Structural and functional abnormalities of the motor system in developmental stuttering. Brain 131, 50–59. doi: 10.1093/brain/awm241
Pubmed Abstract | Pubmed Full Text | CrossRef Full Text | Google Scholar
Weber-Fox, C., Spruill, J. E., Spencer, R., and Smith, A. (2008). Atypical neural functions underlying phonological processing and silent rehearsal in children who stutter. Dev. Sci. 11, 321–337. doi: 10.1111/j.1467-7687.2008.00678.x
Pubmed Abstract | Pubmed Full Text | CrossRef Full Text | Google Scholar
Yairi, E., and Ambrose, N. G. (1999). Early childhood stuttering I: persistency and recovery rates. J. Speech Lang. Hear. Res. 42, 1097–1112. doi: 10.1044/jslhr.4205.1097
Pubmed Abstract | Pubmed Full Text | CrossRef Full Text | Google Scholar
Zijdenbos, A. P., Forghani, R., and Evans, A. C. (2002). Automatic “pipeline” analysis of 3-D MRI data for clinical trials: application to multiple sclerosis. IEEE Trans. Med. Imaging 21, 1280–1291. doi: 10.1109/tmi.2002.806283
Pubmed Abstract | Pubmed Full Text | CrossRef Full Text | Google Scholar
Keywords: developmental stuttering, Broca’s area, speech production, motor control, cortical thickness, neurodevelopment, inferior frontal gyrus, developmental disorders
Citation: Beal DS, Lerch JP, Cameron B, Henderson R, Gracco VL and De Nil LF (2015) The trajectory of gray matter development in Broca’s area is abnormal in people who stutter. Front. Hum. Neurosci. 9:89. doi: 10.3389/fnhum.2015.00089
Received: 15 August 2014; Accepted: 04 February 2015;
Published online: 03 March 2015.
Edited by:
Angela T. Morgan, Royal Childrens Hospital and University of Melbourne, AustraliaReviewed by:
Ruthger Righart, Technische Universität München, GermanyAnne Smith, Purdue University, USA
Copyright © 2015 Beal, Lerch, Cameron, Henderson, Gracco and De Nil. This is an open-access article distributed under the terms of the Creative Commons Attribution License (CC BY). The use, distribution and reproduction in other forums is permitted, provided the original author(s) or licensor are credited and that the original publication in this journal is cited, in accordance with accepted academic practice. No use, distribution or reproduction is permitted which does not comply with these terms.
*Correspondence: Deryk S. Beal, Department of Communication Sciences and Disorders and the Institute for Stuttering Treatment and Research, Faculty of Rehabilitation Medicine, University of Alberta, Suite 1500, College Plaza, 8215 – 112 Street NW, Edmonton, AB T6G 2C8, Canada e-mail:ZGJlYWxAdWFsYmVydGEuY2E=