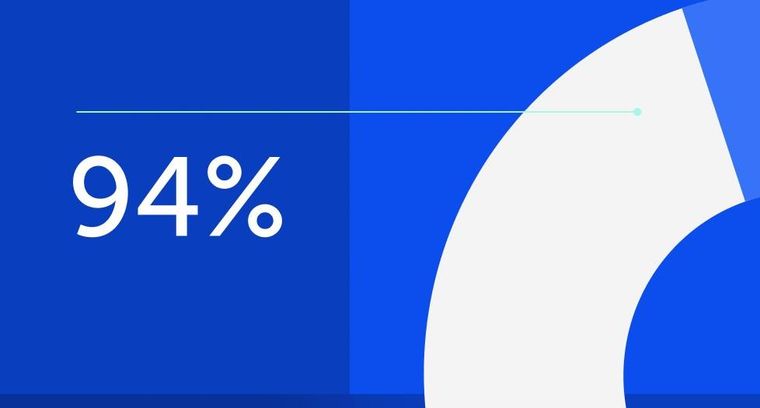
94% of researchers rate our articles as excellent or good
Learn more about the work of our research integrity team to safeguard the quality of each article we publish.
Find out more
SYSTEMATIC REVIEW article
Front. Neurol., 07 March 2025
Sec. Neurorehabilitation
Volume 16 - 2025 | https://doi.org/10.3389/fneur.2025.1500020
Background: Lower extremity dysfunction post-stroke significantly impedes patient independence and quality of life. Non-invasive brain stimulation (NIBS) and robot-assisted gait training (RAGT) have individually shown promising outcomes in gait recovery. However, the synergistic efficacy of non-invasive brain stimulation combined with robot-assisted gait training remains uncertain. This systematic review and meta-analysis aim to evaluate the combined therapy’s effectiveness on gait improvement and related motor functions in stroke patients.
Methods: Following PRISMA guidelines, a comprehensive search was conducted to identify randomized controlled trials (RCTs) published up to September 2024. The primary outcome was assessed using the 6-min walk test (6MWT), with secondary outcomes examining assessed using the Functional Ambulation Category (FAC); the Motion Index (MI) to analyze exercise intensity; the Modified Ashworth Scale (MAS) to assess spasticity; and spatiotemporal gait parameters (SPG).
Results: Six randomized controlled trials involving 191 stroke patients were included. Meta-analysis revealed that combined non-invasive brain stimulation and robot-assisted gait training significantly improved the 6-min walk test scores (mean difference [MD] = 21.81, 95% CI = 0.03–43.59), though effects on strength, activity participation, spasticity, and coordination were non-significant.
Conclusion: Non-invasive brain stimulation combined with robot-assisted gait training shows potential in enhancing gait function but provides limited additional benefits for other motor functions. This combined approach may serve as an effective rehabilitation strategy for post-stroke gait recovery, warranting further large-scale studies to refine intervention protocols.
Systematic review registration: https://www.crd.york.ac.uk/PROSPERO/view/CRD42021283890.
Stroke ranks among the primary causes of long-term neurological impairment and mortality worldwide (1, 2). In the world, the crude incidence rate of stroke was 11.3 cases/1,000 person-years (3). The prevalence of stroke may be underestimated due to the cardiovascular effects of COVID-19 sequelae (1, 4). Disability following a cerebrovascular accident or stroke has become increasingly prominent (5). Independent walking is a critical determinant of overall autonomy and quality of life. Gait is also one of the most reliable indicators of physical function in stroke patients (6). It affects not only general health but also an individual’s independence in daily life (7). Major causes of gait abnormalities include limb weakness or paralysis, impaired balance, and reduced visual acuity (8). Rehabilitation interventions are essential for recovering functional abilities in stroke patients.
Rehabilitation robots stimulate muscle activation, synergy, and neural plasticity through specific repetitive movements and coordinated exercises. Compared to conventional rehabilitation therapy, lower-limb rehabilitation robots provide more robust neural stimulation, promoting the recovery of lower-limb function (9). Currently, lower-limb rehabilitation robots address most of the gait rehabilitation needs of stroke patients by improving standing balance (with and without assistance) (9, 10), enhancing walking ability (11), accelerating walking speed (10, 12), increasing joint mobility (especially in the hip and knee), strengthening leg muscles, improving motor patterns, and correcting abnormal gait (13, 14). These robots can enhance neural plasticity mechanisms related to motor learning and functional recovery after brain injury, including sensorimotor plasticity, effective frontal–parietal connectivity (FPEC), and cross-callosal inhibition (11, 15).
Non-invasive brain stimulation (NIBS), a simple, effective, and measurable rehabilitation technology, has been widely used in scientific research and clinical practice in recent years. It primarily includes transcranial magnetic stimulation (TMS) (16), transcranial alternating current stimulation (tACS), and transcranial direct current stimulation (tDCS) (17). Different paradigms and parameters can be applied according to specific conditions to modulate cortical excitability and regulate neural network functions (18).
A previous systematic review and meta-analysis evaluated tDCS as an intervention for stroke patients (19). Robot-assisted gait training, combined with physiotherapy and body weight support, appears to be an effective intervention for gait recovery following stroke (20). However, whether non-invasive brain stimulation, when used in conjunction with robot-assisted therapy, improves motor activity or function in stroke patients remains debated (21, 22). The primary objective of this systematic review and meta-analysis is to determine whether combining robot therapy with non-invasive brain stimulation can improve lower-limb walking function in stroke patients beyond what robot-assisted training alone can achieve. The secondary objective is to explore the potential synergistic effects of NIBS on robotic training. Additionally, we assess the effectiveness of NIBS in combination with RAGT in enhancing strength, reducing spasticity, and improving functional independence.
This systematic review and meta-analysis adhered to the Preferred Reporting Items for Systematic Reviews and Meta-Analyses (PRISMA) guidelines (23) and was registered in PROSPERO (registration ID: CRD42021283890).
Two authors (JYW and JNM) conducted a comprehensive literature search up to September 2024 across the professional databases MEDLINE (via PubMed), EMBASE, and the Cochrane Central Register of Controlled Trials (CENTRAL). To identify studies that may have been missed in database searches, the reference lists of all relevant papers were also manually reviewed. The databases were searched using the following keywords: gait, walking, robot, exoskeleton, non-invasive brain stimulation, transcranial direct current stimulation, and transcranial magnetic stimulation. The full search strategy is detailed in the Supplementary Table 1.
The flowchart in Figure 1 illustrates the study selection process (24). Studies were selected according to the PICOS framework outlined in the PRISMA guidelines. We included studies based on the following criteria: (1) participants diagnosed with a cerebrovascular accident or stroke; (2) randomized controlled trials (RCTs); (3) combined intervention of non-invasive brain stimulation and robot-assisted walking training; (4) at least one assessment of lower limb function was conducted before and after the intervention.
Studies were excluded if they met the following criteria: (1) only protocols, abstracts, or conference papers; (2) participants younger than 18 years of age were included; (3) individuals with lower limb weakness from other conditions. Two independent investigators (JYW and JNM) selected the studies based on the inclusion and exclusion criteria. Any disagreements were resolved through discussion and reviewed by a third investigator (HHZ).
Two researchers independently extracted data on the following parameters for the combination therapy and control groups: author, country, sample size, patient age and sex, type of intervention, randomization, time since stroke, inclusion and exclusion criteria, number of dropouts, side of cerebrovascular accident, type of stroke (ischemic/hemorrhagic), length and severity of rehabilitation, assessment period, and outcomes.
For outcome evaluation, the 6-min walking test (6MWT) was the primary measure of physical function, as it reflects cardiopulmonary function and exercise tolerance and is one of the best predictors of community walking (2, 25). Secondary measures included activity participation [assessed using the Functional Ambulation Category (FAC)]; the Motion Index (MI) to analyze exercise intensity; the Modified Ashworth Scale (MAS) to assess spasticity; and spatiotemporal gait parameters (SPG) described by step frequency and the ratio of single-phase to dual-phase support periods. We selected the ratio of single-phase to dual-phase support periods as the outcome to describe physical coordination and symmetry, as it provides a more comprehensive assessment than step frequency alone. Follow-up was assessed by recording the number of adverse events and dropouts in each group.
Three comparisons were made in 3-arm studies: according to the recommendations of the Cochrane Group, the shared group in the study was divided into smaller subgroups to ensure the independence of comparisons. In Picelli et al. (26), Group 2, which received sham tDCS combined with cathodal tsDCS (n = 10), was designated as the shared group. This group was compared with Group 1, which received anodal tDCS combined with sham tsDCS (n = 10), and Group 3, which received tDCS combined with cathodal tsDCS (n = 10). Consequently, the number of participants in Group 2 was divided by 2. In Geroin et al. (27), Group 1, which underwent RAGT combined with tDCS (n = 10), was defined as the shared group. This group was compared with Group 2, which underwent RAGT combined with sham tDCS (n = 10), and Group 3, which performed overground walking (n = 10). As a result, the number of participants in Group 1 was divided by 2. After confirming normal distribution, data collected as medians and quartiles were transformed into means and standard deviations (28, 29).
For studies requiring clarification or access to missing data, corresponding authors were contacted for further details.
The quality of the six included studies was assessed using the Cochrane Collaboration’s Risk of Bias (RoB) 1.0 tool. Two reviewers (JNM and XL) assessed potential bias using the following criteria: (1) random sequence generation, (2) allocation concealment, (3) blinding of participants and personnel, (4) selective outcome assessment, (5) incomplete outcome data, (6) selective reporting, and (7) other bias. Disagreements were resolved through consensus. The analysis was performed using RevMan 5.4 (The Cochrane Collaboration, London, United Kingdom).
To assess publication bias, funnel plots for the primary variable (function) were examined. Duval and Tweedie’s trim-and-fill method was used to adjust effect estimates by accounting for unpublished studies with smaller effects.
The feasibility of a meta-analysis was assessed following data extraction. A meta-analysis was conducted if the intervention procedures and outcomes of at least three studies were comparable. The meta-analysis was performed using RevMan 5.4 software. When certain outcomes were presented on different scales, they were represented using the standardized mean difference (SMD). If all studies used the same measurement tool, mean difference (MD) was calculated. To account for adverse events and loss to follow-up, risk difference (RD) was computed. For each outcome, a 95% confidence interval (95% CI) was calculated.
Publication bias was assessed using a funnel plot. Heterogeneity was assessed using the I2 statistic. Statistical heterogeneity was considered significant when I2 ≥ 50% (30). Sensitivity analysis was performed to assess the potential contribution of high-risk studies to heterogeneity and their impact on result stability. Results were considered statistically significant when the p-value was less than 0.05 in the corresponding z-test.
In the final search conducted in January 2025, 396 records were identified from the databases. Ninety-nine duplicates, review, and registration only were identified, and after screening the titles and abstracts, 287 records were excluded. The full texts of 10 articles were examined for eligibility. Furthermore, 4 articles were excluded based on the following five exclusion criteria: (1) non-randomized studies (n = 2), (2) treatment protocols (n = 1), (3) no original data (n = 1). Finally, the systematic review and meta-analysis included six RCTs (26, 27, 31–34) that met the inclusion criteria (Figure 1).
The six studies included non-invasive brain stimulation and robot-assisted walking training, with a total of 191 participants (126 females, 66.0%; 98 participants in the intervention group, 51.3%). The maximum mean age was 75.25 years, and the minimum mean age was 52.7 years. In four studies (26, 32–34), the stroke onset time was more than 6 months; in one study (31) the onset time was between 2 weeks and 6 months (subacute stroke); and in one study (27), the onset time exceeded 12 months. Treatment frequency was five times per week. Treatment lasted for 2 weeks (26, 27, 32–34) or 4 weeks (31). The treatment duration ranged from 20 to 65 min. Except for the control group, there was no difference in treatment duration between the experimental and control groups. Only one of the six studies did not include follow-up (31). Table 1 displays the sociodemographic and clinical characteristics of the study participants. Table 2 lists the study’s intervention measures and evaluation methods.
Figure 2 illustrates the risk of bias in the included controlled studies. Risk of bias was assessed in the selected randomized controlled trials. One study (27) did not implement blinding due to the establishment of a blank control group, while three studies (31, 33, 34) used single-blind methods, and two studies (26, 32) used double-blind methods. For random sequence generation, one study (31) did not specify the method of sequence generation, while the other studies (26, 27, 32–34) demonstrated adequate randomization. Allocation concealment was implemented in four studies (26, 32–34), but was not reported in two studies (27, 31). Only one study (32) was considered to have blinded outcome assessment. All studies (26, 27, 31–34) were considered to have complete data. Only one study (27) was considered to have other biases due to its early publication date.
Figure 2. Risk of bias summary. Review authors’ judgments about each risk of bias item for each included study (upper figure). Risk of bias item presented as percentages across all included studies (lower figure).
The trim-and-fill method was used to adjust for asymmetry in the funnel plot’s main variable distribution. The corrected results are presented in Figure 3. The distribution of variables in the funnel plot using the trim-and-fill method was symmetrical, indicating a low risk of publication bias.
Figure 3. Funnel plot modified by trim and fill method comparison between RAGT associated with NIBS (active) versus RAGT associated with sham/none NIBS (control) for the main outcome. Asymmetries were not observed.
A quantitative analysis of the primary outcome, lower limb function, was conducted following the objectives of this meta-analysis and the PROSPERO protocol. Lower limb function was assessed using the six-minute walk test (6MWT), which reflects cardiopulmonary function and exercise tolerance, and is a strong predictor of community walking (2, 25). The effect on strength was investigated in five studies (26, 27, 32–34). The effects on activities and participation were investigated in six studies (26, 27, 31–34) spasticity in three studies (26, 33, 34), and physical coordination was measured using the ratio of single- to double-support duration in four studies (26, 27, 33, 34).
Among the RCTs, five studies performed the 6MWT and were divided into seven groups. Pooled data demonstrated that, compared to the control group, NIBS combined with RAGT significantly improved lower limb function (mean difference [MD] = 21.81, 95% confidence interval [CI] = 0.03–43.59, n = 140), with low heterogeneity (I2 = 24%, p = 0.24) (Figure 4).
Figure 4. Forest plot of all trials comparing RAGT associated with NIBS (active) versus RAGT associated with sham/none NIBS (control) for body structure/function outcomes.
The meta-analysis indicated that NIBS did not enhance the effects of RAGT in improving strength in stroke patients (standardized mean difference [SMD] = 0.51, 95% CI: −0.37 to 1.38, n = 119) and displayed considerable heterogeneity (I2 = 75%, p < 0.01) (Figure 5A). Geroin et al. (27) significantly influenced the results. When excluded (I2 = 0%; p = 0.97), a random-effects model was applied (SMD = 0.09; 95% CI: −0.30 to 0.49; p = 0.65), and heterogeneity decreased compared to the initial analysis (I2 = 75%, p < 0.01). The sensitivity analysis results remained stable, suggesting that the excluded study was a source of heterogeneity (Supplementary Figure 1).
Figure 5. Forest plot of all trails comparing RAGT associated with NIBS (active) versus RAGT associated with Sham/none NIBS (control) for secondary outcomes. (A) Effect on strength. (B) Effect on activities and participation. (C) Effect on spasticity. (D) Effect on coordination.
The Functional Ambulation Category (FAC) scores were used to represent activities and participation. The analysis of NIBS combined with RAGT did not reveal any significant effects on activities and participation (MD = 0.23, 95% CI = −0.26 to 0.72, n = 192) and showed considerable heterogeneity (I2 = 91%, p < 0.01) (Figure 5B). Geroin et al. (27) also significantly influenced the results. When excluded (I2 = 0%; p = 0.66), a random-effects model was applied (MD = 0.21; 95% CI = −0.03 to 0.45; p = 0.08), and heterogeneity decreased (I2 = 91%, p < 0.01). The sensitivity analysis results remained stable, suggesting that the excluded study was a source of heterogeneity (Supplementary Figure 2).
Spasticity was assessed using the Modified Ashworth Scale (MAS). Figure 5C shows the pooled results. The results showed that NIBS-RAGT had no significant effect on spasticity compared with control interventions (MD = −0.04; 95% CI = −0.74 to 0.67, n = 89), and the data revealed low heterogeneity (I2 = 0%, p = 0.88).
The trials assessing the effect on physical coordination are summarized in Figure 5D. The total effect on physical coordination did not reveal a significant difference between the experimental and control groups (MD = 0.05; 95% CI = −0.16 to 0.26, n = 119), with high heterogeneity (I2 = 78%, p < 0.01). Similar findings were observed in a sensitivity analysis excluding studies with a high risk of bias (MD = −0.06; 95% CI = −0.18 to 0.07; p = 0.37), which reduced heterogeneity (I2 = 6%; p = 0.37) (Supplementary Figure 3).
All studies were included in the analysis. Studies documenting adverse events and the number of patients lost to follow-up are listed in Figure 6. The analysis showed no significant difference between the two groups in the risk of adverse events (RD = −0.02; 95% CI = −0.04 to 0.07) or in the number of patients lost to follow-up (RD = 0.00; 95% CI = −0.06 to 0.06). All six included studies reported on adverse reactions; however, only Leon et al. (31) in the experimental group reported two cases of temporary mild pruritus and one case of exclusion due to mild headache during and after stimulation.
Figure 6. Forest plot of comparison between RAGT associated with NIBS (active) versus RT associated with sham/none NIBS (control) for adverse event (upper figure) and lost to follow-up (lower figure).
This systematic review and meta-analysis summarize the clinical effects of NIBS-Robot training on lower extremity function, safety profiles, strength, spasticity, activities, participation, and physical coordination in stroke patients. The NIBS protocols in the study included transcranial direct current stimulation (tDCS), cerebellar transcranial direct current stimulation (tcDCS), and transcutaneous spinal direct current stimulation (tsDCS) spatiotemporal gait parameters. Currently, evidence on the effectiveness of NIBS-RAGT in previous clinical trials remains inconclusive. The meta-analysis indicated a substantial improvement in the primary outcome (lower limb function) and non-significant improvements in the secondary outcomes (strength, spasticity, activities, participation, and physical coordination).
Ambulation involves motor patterns generated by central pattern generators (CPGs) and cortical and subcortical structures (35). Due to the complexity of motion control, NIBS combined with robotic therapy may better support the functional reorganization of motor networks (36). The NIBS protocols in this review were divided into two strategies: direct stimulation of the affected M1 and indirect stimulation through the dentate-thalamo-cortical pathway, both based on the interhemispheric competition model. This hypothesis may limit the benefits for some stroke patients whose corticospinal tracts are severely damaged (18). Individualized NIBS therapy, tailored to patient conditions, may play a stronger role in enhancing robotic therapy (37). In this meta-analysis, heterogeneity was mainly attributed to Geroin et al. (27), likely due to the different types of robots and NIBS paradigms. Interestingly, the placebo effect of sham transcranial direct current stimulation was investigated, revealing insights into the mechanism of intervention (38). Additionally, factors such as NIBS parameters, electrode size, stimulation site, duration, and frequency may influence the intervention’s efficacy (39). Individual variability in NIBS includes differences in brain anatomy, cerebral cortex activity, muscle precontractions, focus of attention, and even variability caused by menstrual cycles and circadian rhythms (40). Non-invasive brain stimulation, particularly transcranial direct current stimulation, has shown potential for improving motor function in patients with neurological impairments. However, the results are often inconsistent, which can be attributed in part to the standardized, non-tailored approach used in many studies. A key limitation of current tDCS protocols is their reliance on fixed stimulation parameters, such as electrode placement, stimulation intensity, and polarity (anodal vs. cathodal). These protocols do not account for the individual variability in neurophysiological responses across patients. Future research could benefit from a more tailored approach, where tDCS is adjusted to preserve critical neural pathways, such as the corticospinal tract, and guided by motor evoked potentials (MEPs). This individualized approach, in which both stimulation parameters and electrode sites are customized to each patient’s unique brain activity, may enhance the efficacy of tDCS and lead to more consistent improvements in motor function (17). In the future, neuronavigation and real-time EEG monitoring of NIBS effects may help reduce variability in outcomes (41). Clarifying the response mechanisms and biomarkers may be key to improving success rates.
While the primary outcomes demonstrated significant improvement, the secondary outcomes did not show marked changes. A closer look at the potential reasons for this discrepancy reveals the influence of different robotic-assisted gait training (RAGT) systems and experimental designs. One such system is the Lokomat (42), a robotic gait orthosis designed to assist patients with impaired mobility by providing repetitive, adjustable movement patterns during walking. The Lokomat system is equipped with adjustable robotic legs that support the patient’s lower limbs while encouraging a natural gait pattern, offering a combination of body weight support and assistance in leg movement. The system can be calibrated for different levels of assistance, ranging from low to high intensity, based on individual patient needs. However, the impact of the Lokomat on secondary outcomes such as gait performance may be limited if the system is not tailored to the specific therapeutic goals of the patient (43). Furthermore, the protocols used in these interventions—including session duration, frequency, and intensity—might also explain the limited changes observed in secondary outcomes. These factors highlight the complexity of evaluating gait improvement and motor function in clinical settings, where secondary outcomes might not always align with the primary measures of success.
Regarding adverse events, NIBS-Robot training can be considered a safe treatment. All studies reported that NIBS-Robot training was safe, with no severe adverse events. Only one included study observed mild adverse reactions, consistent with earlier research (44).
Robots in this study were categorized as exoskeletons or end-effector devices. All exoskeleton robots in the included studies were static, utilizing a fixed treadmill. Compared to overground robots, the combination with NIBS may involve different mechanisms (45). Moreover, in all randomized controlled trials, the robot settings were not adequately tailored to patient characteristics. Larger and more comparative studies are needed to determine the optimal timing and program design for individualized treatment.
Limitations of this meta-analysis include: (1) we included only six studies for meta-analysis, with three from the same group (Picelli et al.). This limits the generalizability of our findings due to potential biases in study design and sample selection. Future research should incorporate more diverse data to improve result reliability. (2) Most subjects had chronic stroke, so findings cannot be extended to patients with subacute stroke. (3) The sample sizes in the included studies were generally small, with only one study reaching 50 participants (31). Larger sample sizes may influence the outcomes. (4) Differences between intervention programs and equipment. (5) A lack of neurophysiological assessments of cortical excitability and brain connectivity, with only one study evaluating motor evoked potentials (MEP) via transcranial magnetic stimulation. (6) The majority of trials lacked long-term follow-up. (7) All NIBS treatments followed the interhemispheric competition model, which may not be suitable for all stroke patients.
The meta-analysis showed that NIBS combined with RAGT may be beneficial for lower limb function, but these results should be interpreted cautiously due to the limitations of this study. Furthermore, no significant improvements were found for NIBS combined with RAGT in terms of strength, spasticity, activities, participation, or physical coordination. Regarding adverse events, NIBS combined with RAGT can be considered a safe treatment with few adverse reactions. Moreover, the heterogeneity of subjects, variability of NIBS settings and RAGT devices, and unpredictability of results make comparisons between trials difficult. Future RCTs need to stratify patients based on the type and stage of cerebrovascular accident, include larger sample sizes, longer follow-up periods, and consider adverse reactions to determine the optimal parameters of NIBS combined with RAGT.
The original contributions presented in the study are included in the article/Supplementary material, further inquiries can be directed to the corresponding author.
JW: Funding acquisition, Methodology, Writing – original draft, Data curation, Formal analysis. HZ: Conceptualization, Data curation, Writing – original draft. JM: Data curation, Formal analysis, Writing – review & editing. LG: Formal analysis, Methodology, Writing – review & editing. XL: Conceptualization, Data curation, Funding acquisition, Writing – review & editing.
The author(s) declare that financial support was received for the research, authorship, and/or publication of this article. This study was supported by General Scientific Research Project of Zhejiang Department of Education, PRC (Y202145964).
The authors declare that the research was conducted in the absence of any commercial or financial relationships that could be construed as a potential conflict of interest.
All claims expressed in this article are solely those of the authors and do not necessarily represent those of their affiliated organizations, or those of the publisher, the editors and the reviewers. Any product that may be evaluated in this article, or claim that may be made by its manufacturer, is not guaranteed or endorsed by the publisher.
The Supplementary material for this article can be found online at: https://www.frontiersin.org/articles/10.3389/fneur.2025.1500020/full#supplementary-material
1. Lindsay, LR, Thompson, DA, and O'Dell, MW. Updated approach to stroke rehabilitation. Med Clin North Am. (2020) 104:199–211. doi: 10.1016/j.mcna.2019.11.002
2. Selves, C, Stoquart, G, and Lejeune, T. Gait rehabilitation after stroke: review of the evidence of predictors, clinical outcomes and timing for interventions. Acta Neurol Belg. (2020) 120:783–90. doi: 10.1007/s13760-020-01320-7
3. Wang, Q, Mejía-Guevara, I, Rist, PM, Walter, S, Capistrant, BD, and Glymour, MM. Changes in memory before and after stroke differ by age and sex, but not by race. Cerebrovasc Dis. (2014) 37:235–43. doi: 10.1159/000357557
4. Al-Aly, Z, Xie, Y, and Bowe, B. High-dimensional characterization of post-acute sequelae of COVID-19. Nature. (2021) 594:259–64. doi: 10.1038/s41586-021-03553-9
5. GBD 2015 Neurological Disorders Collaborator Group. Global, regional, and national burden of neurological disorders during 1990-2015: a systematic analysis for the Global Burden of Disease Study 2015. Lancet Neurol. (2017) 16:877–97. doi: 10.1016/S1474-4422(17)30299-5
6. Doi, T, Nakakubo, S, Tsutsumimoto, K, Kurita, S, Ishii, H, and Shimada, H. Spatiotemporal gait characteristics and risk of mortality in community-dwelling older adults. Maturitas. (2021) 151:31–5. doi: 10.1016/j.maturitas.2021.06.007
7. Alawieh, A, Zhao, J, and Feng, W. Factors affecting post-stroke motor recovery: implications on neurotherapy after brain injury. Behav Brain Res. (2018) 340:94–101. doi: 10.1016/j.bbr.2016.08.029
8. Chieffo, R, Comi, G, and Leocani, L. Noninvasive neuromodulation in Poststroke gait disorders: rationale, feasibility, and state of the art. Neurorehabil Neural Repair. (2016) 30:71–82. doi: 10.1177/1545968315586464
9. Poli, P, Morone, G, Rosati, G, and Masiero, S. Robotic technologies and rehabilitation: new tools for stroke patients’ therapy. Biomed Res Int. (2013) 2013:153872. doi: 10.1155/2013/153872
10. Benito-Penalva, J, Edwards, DJ, Opisso, E, Cortes, M, Lopez-Blazquez, R, Murillo, N, et al. Gait training in human spinal cord injury using electromechanical systems: effect of device type and patient characteristics. Arch Phys Med Rehabil. (2012) 93:404–12. doi: 10.1016/j.apmr.2011.08.028
11. Calabrò, RS, Cacciola, A, Bertè, F, Manuli, A, Leo, A, Bramanti, A, et al. Robotic gait rehabilitation and substitution devices in neurological disorders: where are we now? Neurol Sci. (2016) 37:503–14. doi: 10.1007/s10072-016-2474-4
12. Uçar, DE, Paker, N, and Buğdaycı, D. Lokomat: a therapeutic chance for patients with chronic hemiplegia. NeuroRehabilitation. (2014) 34:447–53. doi: 10.3233/NRE-141054
13. Husemann, B, Müller, F, Krewer, C, Heller, S, and Koenig, E. Effects of locomotion training with assistance of a robot-driven gait orthosis in hemiparetic patients after stroke: a randomized controlled pilot study. Stroke. (2007) 38:349–54. doi: 10.1161/01.STR.0000254607.48765.cb
14. Esquenazi, A, Lee, S, Packel, AT, and Braitman, L. A randomized comparative study of manually assisted versus robotic-assisted body weight supported treadmill training in persons with a traumatic brain injury. PM R. (2013) 5:280–90. doi: 10.1016/j.pmrj.2012.10.009
15. Hara, Y. Brain plasticity and rehabilitation in stroke patients. J Nippon Med Sch. (2015) 82:4–13. doi: 10.1272/jnms.82.4
16. Kubis, N. Non-invasive brain stimulation to enhance post-stroke recovery. Front Neural Circuits. (2016) 10:56. doi: 10.3389/fncir.2016.00056
17. Woods, AJ, Antal, A, Bikson, M, Boggio, PS, Brunoni, AR, Celnik, P, et al. A technical guide to tDCS, and related non-invasive brain stimulation tools. Clin Neurophysiol. (2016) 127:1031–48. doi: 10.1016/j.clinph.2015.11.012
18. Di Pino, G, Pellegrino, G, Assenza, G, Capone, F, Ferreri, F, Formica, D, et al. Modulation of brain plasticity in stroke: a novel model for neurorehabilitation. Nat Rev Neurol. (2014) 10:597–608. doi: 10.1038/nrneurol.2014.162
19. Li, Y, Fan, J, Yang, J, He, C, and Li, S. Effects of transcranial direct current stimulation on walking ability after stroke: a systematic review and meta-analysis. Restor Neurol Neurosci. (2018) 36:59–71. doi: 10.3233/RNN-170770
20. Moucheboeuf, G, Griffier, R, Gasq, D, Glize, B, Bouyer, L, Dehail, P, et al. Effects of robotic gait training after stroke: a meta-analysis. Ann Phys Rehabil Med. (2020) 63:518–34. doi: 10.1016/j.rehab.2020.02.008
21. Reis, SB, Bernardo, WM, Oshiro, CA, Krebs, HI, and Conforto, AB. Effects of robotic therapy associated with noninvasive brain stimulation on upper-limb rehabilitation after stroke: systematic review and Meta-analysis of randomized clinical trials. Neurorehabil Neural Repair. (2021) 35:256–66. doi: 10.1177/1545968321989353
22. Comino-Suárez, N, Moreno, JC, Gómez-Soriano, J, Megía-García, Á, Serrano-Muñoz, D, Taylor, J, et al. Transcranial direct current stimulation combined with robotic therapy for upper and lower limb function after stroke: a systematic review and meta-analysis of randomized control trials. J Neuroeng Rehabil. (2021) 18:148. doi: 10.1186/s12984-021-00941-0
23. Shamseer, L, Moher, D, Clarke, M, Ghersi, D, Liberati, A, Petticrew, M, et al. Preferred reporting items for systematic review and meta-analysis protocols (PRISMA-P) 2015: elaboration and explanation. BMJ. 354:i4086. doi: 10.1136/bmj.i4086
24. Page, MJ, McKenzie, JE, Bossuyt, PM, Boutron, I, Hoffmann, TC, Mulrow, CD, et al. The PRISMA 2020 statement: an updated guideline for reporting systematic reviews. BMJ. (2021) 372:n71. doi: 10.1136/bmj.n71
25. ATS Committee on Proficiency Standards for Clinical Pulmonary Function Laboratories. ATS statement: guidelines for the six-minute walk test. Am J Respir Crit Care Med. (2002) 166:111–7. doi: 10.1164/ajrccm.166.1.at1102
26. Picelli, A, Chemello, E, Castellazzi, P, Roncari, L, Waldner, A, Saltuari, L, et al. Combined effects of transcranial direct current stimulation (tDCS) and transcutaneous spinal direct current stimulation (tsDCS) on robot-assisted gait training in patients with chronic stroke: a pilot, double blind, randomized controlled trial. Restor Neurol Neurosci. (2015) 33:357–68. doi: 10.3233/RNN-140474
27. Geroin, C, Picelli, A, Munari, D, Waldner, A, Tomelleri, C, and Smania, N. Combined transcranial direct current stimulation and robot-assisted gait training in patients with chronic stroke: a preliminary comparison. Clin Rehabil. (2011) 25:537–48. doi: 10.1177/0269215510389497
28. Wan, X, Wang, W, Liu, J, and Tong, T. Estimating the sample mean and standard deviation from the sample size, median, range and/or interquartile range. BMC Med Res Methodol. (2014) 14:135. doi: 10.1186/1471-2288-14-135
29. Luo, D, Wan, X, Liu, J, and Tong, T. Optimally estimating the sample mean from the sample size, median, mid-range, and/or mid-quartile range. Stat Methods Med Res. (2018) 27:1785–805. doi: 10.1177/0962280216669183
30. Cumpston, M, Li, T, Page, MJ, Chandler, J, Welch, VA, Higgins, JP, et al. Updated guidance for trusted systematic reviews: a new edition of the Cochrane handbook for systematic reviews of interventions. Cochrane Database Syst Rev. (2019) 10:Ed000142. doi: 10.1002/14651858.ED000142
31. Leon, D, Cortes, M, Elder, J, Kumru, H, Laxe, S, Edwards, DJ, et al. tDCS does not enhance the effects of robot-assisted gait training in patients with subacute stroke. Restor Neurol Neurosci. (2017) 35:377–84. doi: 10.3233/RNN-170734
32. Seo, HG, Lee, WH, Lee, SH, Yi, Y, Kim, KD, and Oh, BM. Robotic-assisted gait training combined with transcranial direct current stimulation in chronic stroke patients: a pilot double-blind, randomized controlled trial. Restor Neurol Neurosci. (2017) 35:527–36. doi: 10.3233/RNN-170745
33. Picelli, A, Chemello, E, Castellazzi, P, Filippetti, M, Brugnera, A, Gandolfi, M, et al. Combined effects of cerebellar transcranial direct current stimulation and transcutaneous spinal direct current stimulation on robot-assisted gait training in patients with chronic brain stroke: a pilot, single blind, randomized controlled trial. Restor Neurol Neurosci. (2018) 36:161–71. doi: 10.3233/RNN-170784
34. Picelli, A, Brugnera, A, Filippetti, M, Mattiuz, N, Chemello, E, Modenese, A, et al. Effects of two different protocols of cerebellar transcranial direct current stimulation combined with transcutaneous spinal direct current stimulation on robot-assisted gait training in patients with chronic supratentorial stroke: a single blind, randomized controlled trial. Restor Neurol Neurosci. (2019) 37:97–107. doi: 10.3233/RNN-180895
35. Ellingson, PJ, Barnett, WH, Kueh, D, Vargas, A, Calabrese, RL, and Cymbalyuk, GS. Comodulation of H- and Na(+)/K(+) pump currents expands the range of functional bursting in a central pattern generator by navigating between dysfunctional regimes. J Neurosci. (2021) 41:6468–83. doi: 10.1523/JNEUROSCI.0158-21.2021
36. Paul, T, Hensel, L, Rehme, AK, Tscherpel, C, Eickhoff, SB, Fink, GR, et al. Early motor network connectivity after stroke: an interplay of general reorganization and state-specific compensation. Hum Brain Mapp. (2021) 42:5230–43. doi: 10.1002/hbm.25612
37. Lin, YL, Potter-Baker, KA, Cunningham, DA, Li, M, Sankarasubramanian, V, Lee, J, et al. Stratifying chronic stroke patients based on the influence of contralesional motor cortices: an inter-hemispheric inhibition study. Clin Neurophysiol. (2020) 131:2516–25. doi: 10.1016/j.clinph.2020.06.016
38. Burke, MJ, Romanella, SM, Mencarelli, L, Greben, R, Fox, MD, Kaptchuk, TJ, et al. Placebo effects and neuromodulation for depression: a meta-analysis and evaluation of shared mechanisms. Mol Psychiatry. (2022) 27:1658–66. doi: 10.1038/s41380-021-01397-3
39. Chhatbar, PY, Ramakrishnan, V, Kautz, S, George, MS, Adams, RJ, and Feng, W. Transcranial direct current stimulation post-stroke upper extremity motor recovery studies exhibit a dose-response relationship. Brain Stimul. (2016) 9:16–26. doi: 10.1016/j.brs.2015.09.002
40. Terranova, C, Rizzo, V, Cacciola, A, Chillemi, G, Calamuneri, A, Milardi, D, et al. Is there a future for non-invasive brain stimulation as a therapeutic tool? Front Neurol. (2018) 9:1146. doi: 10.3389/fneur.2018.01146
41. Oh, H, Kim, JH, and Yau, JM. EPI distortion correction for concurrent human brain stimulation and imaging at 3T. J Neurosci Methods. (2019) 327:108400. doi: 10.1016/j.jneumeth.2019.108400
42. Wu, L, Xu, G, and Wu, Q. The effect of the Lokomat® robotic-orthosis system on lower extremity rehabilitation in patients with stroke: a systematic review and meta-analysis. Front Neurol. (2023) 14:1260652. doi: 10.3389/fneur.2023.1260652
43. van Kammen, K, Boonstra, AM, van der Woude, LHV, Visscher, C, Reinders-Messelink, HA, and den Otter, R. Lokomat guided gait in hemiparetic stroke patients: the effects of training parameters on muscle activity and temporal symmetry. Disabil Rehabil. (2020) 42:2977–85. doi: 10.1080/09638288.2019.1579259
44. Rossi, S, Antal, A, Bestmann, S, Bikson, M, Brewer, C, Brockmöller, J, et al. Safety and recommendations for TMS use in healthy subjects and patient populations, with updates on training, ethical and regulatory issues: expert guidelines. Clin Neurophysiol. (2021) 132:269–306. doi: 10.1016/j.clinph.2020.10.003
Keywords: non-invasive brain stimulation, robot, rehabilitation, stroke, gait
Citation: Wang J, Zhang H, Ma J, Gu L and Li X (2025) Efficacy of combined non-invasive brain stimulation and robot-assisted gait training on lower extremity recovery post-stroke: a systematic review and meta-analysis of randomized controlled trials. Front. Neurol. 16:1500020. doi: 10.3389/fneur.2025.1500020
Received: 22 September 2024; Accepted: 25 February 2025;
Published: 07 March 2025.
Edited by:
Jane Margaret Mary Maguire, University of Technology Sydney, AustraliaReviewed by:
Mirko Filippetti, University of Verona, ItalyCopyright © 2025 Wang, Zhang, Ma, Gu and Li. This is an open-access article distributed under the terms of the Creative Commons Attribution License (CC BY). The use, distribution or reproduction in other forums is permitted, provided the original author(s) and the copyright owner(s) are credited and that the original publication in this journal is cited, in accordance with accepted academic practice. No use, distribution or reproduction is permitted which does not comply with these terms.
*Correspondence: Xiang Li, bGl4aWFuZzYzOUBmb3htYWlsLmNvbQ==
Disclaimer: All claims expressed in this article are solely those of the authors and do not necessarily represent those of their affiliated organizations, or those of the publisher, the editors and the reviewers. Any product that may be evaluated in this article or claim that may be made by its manufacturer is not guaranteed or endorsed by the publisher.
Research integrity at Frontiers
Learn more about the work of our research integrity team to safeguard the quality of each article we publish.