- 1Department of Physical Therapy, School of Health Sciences, Ariel University, Ariel, Israel
- 2Department of Physical Therapy, Sackler Faculty of Medicine, Tel-Aviv University, Tel-Aviv, Israel
- 3The International Institute for the Franklin Method, Zurich, Switzerland
- 4Dana-Dwek Children’s Hospital, Pediatric ADHD Clinic, Sourasky Medical Center, Ichilov, Tel Aviv, Israel
Children with attention deficit hyperactivity disorder (ADHD) exhibit various degrees of motor and cognitive impairments in fine and gross motor skills. These impairments impact social functioning, while also hindering academic achievement, self-esteem, and participation. Specifically, motor impairments are not fully addressed by current therapies. For example, approximately 50% of children with ADHD exhibit significant motor impairments, as per clinical measures, while the other 50% experience more impairments in motor planning, execution and control than do typically developed (TD) children. Such findings indicate that ADHD-specific mechanisms may be underpinning motor impairments. In this paper, we outline ADHD impairments in motor planning, execution, and control, and the potential role of two such mechanisms: internal motor representation and timing perception. Next, we suggest mental imagery as an approach for treating ADHD motor impairments, potentially through addressing internal motor representation and timing perception.
1 ADHD: definitions, etiology and diagnosis
Attention deficit hyperactivity disorder (ADHD) is the most common neurodevelopmental disorder among children and adolescents between the ages of 4–17 in the United States, with a prevalence of approximately 11% (1) and 3.4–10% worldwide (2–6). This clinical condition has a male–female ratio of 2.4–4:1 (1) and is characterized by persistent inattention and/or hyperactivity-impulsivity (1, 7). These symptoms impair academic, educational, social, and leisure activities (1–3, 7) thus impeding daily functioning, self-esteem, and well-being (4, 8).
The clinical presentation of ADHD involves additional motor and cognitive impairments, spanning motor planning, execution, and control (1, 9–11). Further, approximately 70% of children with ADHD present with a coexisting psychiatric (e.g., anxiety) or developmental disorders (e.g., developmental coordination disorder; DCD) (1, 12). Such co-occurrences may aggravate ADHD symptoms’ severity, lead to greater functional impairments, and make diagnosis and treatment more challenging (2, 6, 13–15). In this paper, we discuss ADHD impairments in motor execution, planning, and control, including the existing ambiguity in terms and definitions. We then highlight two mechanisms—internal motor representation of action and timing perception—as potentially underpinning ADHD motor impairments. Lastly, we suggest mental imagery as an appropriate approach for alleviating ADHD motor impairments through addressing those mechanisms in children and adolescents with ADHD.
2 Motor impairments in ADHD
Children with ADHD exhibit impairments in motor planning, execution, and control (11, 16, 17). Such impairments are manifested in both the gross (e.g., jumping, running, object manipulation) (18–23) and fine (e.g., manual dexterity) (22–26) motor skills. Further, impairments in motor planning and control include, among others, decreased accuracy and slower and variable movement time (20, 27, 28) (See Section 2.3). Impairments in motor execution are commonly diagnosed using standardized norm referenced motor tests in which the movements of the study group are compared to those of a normative sample, such as the Movement Assessment Battery for Children (M-ABC-2) (38) and the Bruininks-Oseretsky Test of Motor Proficiency (BOT-2) (30). These tests are, however, commonly used for developmental coordination disorder (DCD) diagnosis (7, 31). This could explain, at least in part, why children with ADHD are occasionally considered as having DCD co-occurrence, even without a comprehensive DCD diagnosis. Specifically, approximately 50% of children with ADHD are diagnosed—based on those standardized norm-referenced motor scales (8–10, 19, 23, 26)—with significant motor impairments, consistent with DCD (8–10, 19, 23, 26). However, current literature using this definition is inconsistent as per subgrouping those with “definite” versus “probable” scores (i.e., a score below 5th percentile or a score between 5th and 15th percentiles, respectively) (31). Further, some studies refer to children with ADHD as a homogenous group (22, 32–34), while others (26, 27, 35, 36) divide them into those with or without DCD/motor impairments based on these standardized motor tests. Interestingly, a few studies have shown that those children with ADHD but without scoring significant levels (i.e., ≥16th percentile) of motor impairments, compared to typically developed (TD) children, however, present decreased motor execution, planning and control (20, 27, 35, 37), thus demonstrating a specified motor profile (signature). These latter findings suggest additional underpinning mechanisms for ADHD motor impairments that are directly linked to ADHD etiology. Yet, the subgroup of children with ADHD as without significant motor impairments (as per standardized tests) is not adequately studied in ADHD research, despite exhibiting motor deficiencies. Therefore, it may be useful to refer to children with ADHD either with clinically detected (i.e., consistent with definite and probable DCD; <15th percentile) or without clinically detected (i.e., consistent without DCD; i.e., ≥16th percentile) motor impairments. Such a subgrouping not only distinguishes ADHD from its DCD co-occurrence, but also allows for better investigating ADHD-specific cognitive-motor mechanisms underpinning impairments in motor planning, execution and control. These current and suggested ADHD subgroupings are illustrated in Figure 1.

Figure 1. A metaphorical representation of ADHD population’s subgroups, based on clinical and motor control tests. Throw’s outcome represents clinical tests’ (e.g., M-ABC-2) scores: hit = ≥16th percentile, partial miss = 5th–15th percentile, complete miss = <5th percentile; Ball trajectory represents motor control (i.e., accuracy, response time) impairments: smooth = no impairments, jerky = impairments; (A) ADHD with significant motor impairments and motor control impairments (complete miss and jerky trajectory), (B) ADHD with probable/mild to moderate motor impairments with motor control impairments (partial miss and jerky trajectory), (C) ADHD without motor impairments and with motor control impairments (hit with jerky trajectory), (D) Typically developed (hit with smooth trajectory). Subgroups (A,B) are also labeled “ADHD with clinically detected motor impairmentsˮ and subgroup (C) is also labeled “ADHD with no clinically detected motor impairmentsˮ (Drawn by Eric Franklin).
2.1 Motor execution impairments in ADHD
Motor execution impairments in children with ADHD are exhibited in a variety of tasks, such as walking, hopping, and ball and balance skills. Several studies found motor execution impairments in children with ADHD compared to TD, with only some of these studies subgrouping the ADHD population into with or without DCD (Table 1). These studies used standardized clinical tests (e.g., M-ABC-2, BOT2) (30, 38) and found that 30–60% of children with ADHD exhibited significant motor impairments in jumping, balance, manual dexterity, and ball skills (18, 22, 25, 32, 37) (Table 1). Further, studies that sub-grouped the ADHD population into with and without DCD showed that both subgroups exhibited motor impairments.
2.2 Motor planning and control impairments in ADHD
Motor planning and control impairments in ADHD include increased movement variability (20, 27, 28, 39, 40), timing deficiencies, timing variability and misperception (20, 27, 28, 34, 39), decreased movement accuracy (27, 28), increased execution time (27, 39, 40), and slow and variable reaction time (20, 28) (Table 2). Specifically, increased reaction time has been suggested to relate to impaired motor planning (11, 17) and to underlie altered inhibition intertwined with executive functions (11). Such impairments have been identified in a variety of tasks, including finger tapping (20, 27, 28, 40), jumping (39), and walking (34) (Table 2). Numerous studies have suggested that impairments in motor planning and control in children with ADHD could be attributed, at least in part, to impairments in timing perception (17, 20, 41), movement timing related circuitry in the cerebellum and basal ganglia (20), disturbed visuospatial working memory, inaccurate processing of the motor commands, dependence on visual feed-back during movement execution, and problems with internal representations of objects and visual space (20, 27, 35). Interestingly, a few studies that assessed motor planning and control in ADHD, and sub-grouped participants into ADHD with and without clinically detected motor impairments, found decreased motor control in both groups, compared to TD children (27, 35, 36). These findings support the role of ADHD itself—regardless of DCD—in motor planning and control impairments in children with ADHD.
3 Suggested mechanisms underpinning motor impairments in ADHD
The reasons for impairments in motor execution, planning, and control in children with ADHD are not fully understood, with numerous mechanisms being proposed (Figure 2). Among those mechanisms are DCD co-occurrence (9, 10, 21), ADHD core symptoms (9, 10, 22, 40), and executive functions (working memory, inhibition, and set shifting) (17, 42–44). Timing perception (20, 27, 39, 45) and internal motor representation of action (herein referred to as motor representation) mechanisms (20, 35, 41) have been less researched, although both potentially rely on—and thus share—the neuro-cognitive networks associated with motor planning (46–48). All of these mechanisms are likely to be intertwined and involve numerous brain regions (e.g., cerebellum, basal ganglia, thalamus, and frontal and prefrontal cortices) (49). For example, dopaminergic pathways involving dopamine and noradrenaline, particularly in the mesocortical, mesolimbic, and nigrostriatal areas, have been suggested to be associated with decreased attention, restlessness, impaired learning (50), executive functions (1, 43), motivational behavior (1), and timing (48, 51). A meta-analysis of functional magnetic resonance imaging (fMRI) studies of executive functions suggested that children with ADHD demonstrate cognitive-domain dissociated multisystem impairments in several right and left hemispheric dorsal, ventral, and medial fronto-cingulo-striato-thalamic and fronto-parieto-cerebellar networks, all of which mediate cognitive control, attention, timing, and working memory (48). Also, the inferior frontal cortex-parieto-cerebellar is one pathway that has been particularly associated with timing deficits (48).
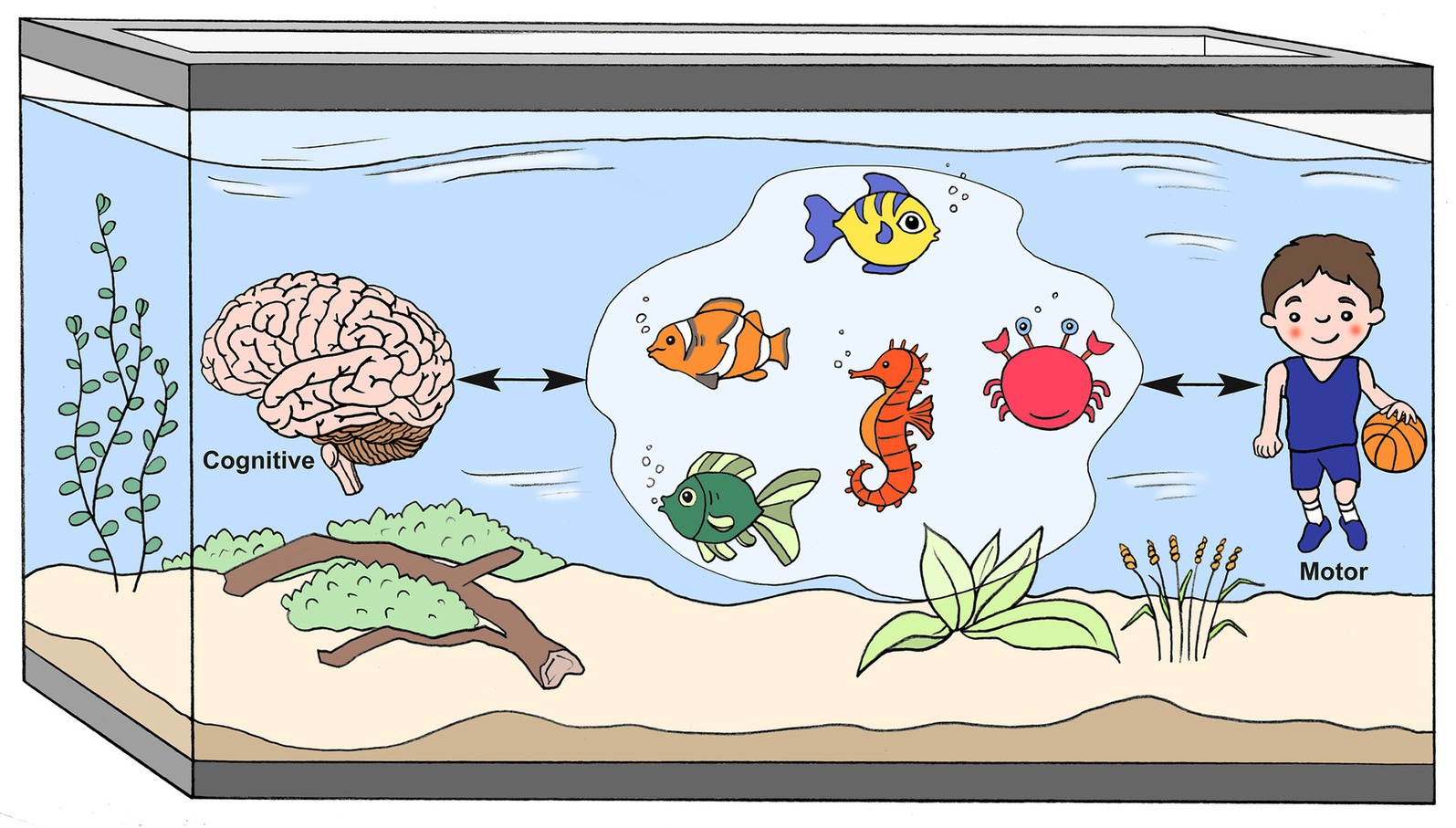
Figure 2. A metaphorical model of suggested cognitive-motor mechanisms underlying ADHD motor impairments. , DCD co-occurrence;
, Executive Functions;
, ADHD Core Symptoms;
, Timing;
, Mental Representations (Drawn by Eric Franklin).
Specifically, the high co-occurrence of DCD with ADHD in children suggests the role of the former in ADHD motor impairments, including learning and executing coordinated movements (31, 52, 53), thus resulting in low and slow motor performance (52–54). The role of ADHD core symptoms (inattention, hyperactivity, and impulsivity) in ADHD motor impairments is suggested by children with ADHD who are not clinically detected on the DCD standardized measures, and still exhibit motor impairments than TD children (26, 27, 35, 36). This clinical presentation is supported by research showing a positive correlation between ADHD core symptoms and impairments in motor execution and control (22, 40). A study comparing 42 children with ADHD and 42 age-matched TD (M age: 8.25 years) (22) found that level of attention and impulse control (based on Gordon Diagnostic System) predicted lower performance in balance and visual-motor control (Bruininks-Oseretsky Test of Motor Proficiency-BOTOMP) (55). Additional evidence for the possible interaction of ADHD core symptoms with ADHD motor impairments can be found in the positive effect of stimulant therapies on ADHD motor impairments (19, 34). Lastly, the role of executive functions in ADHD motor impairments is supported by literature reporting that children with ADHD exhibit deficits in brain regions (e.g., prefrontal cortex) that are involved in executive functions (4, 48, 56). Other studies have found positive correlations between deficits in executive functions and levels of ADHD motor impairments (17, 44).
Tracking the path of causality between either of these mechanisms—as well as the interaction among them—and ADHD motor impairments is, however, challenging for numerous reasons. For example, neuro-imaging findings do not perfectly correlate with motor impairments (49), ADHD core symptoms of inattention and hyperactivity do not always correlate with motor impairments (35), and medications for ADHD do not fully alleviate ADHD motor impairments (19, 34). The role of the other two mechanisms—motor representation and timing perception—is detailed below.
3.1 Internal motor representation of action
Internal motor representation of action (aka motor representation) refers to one’s mental depiction of an action, including its spatio-temporal characteristics and effect (57, 58). This cognitive image relies on, among others, one’s previous experience with, and understanding of, the task (57, 58). Motor representation is part of the internal forward model, a neural framework that simulates the body’s dynamic behavior and predicts its effect in relation to the environment (35, 57, 59, 60). Of note, using motor representation implies mentally imaging (simulating) the task in one’s mind, a process known as motor imagery. This simulation process underlies successful motor planning, execution, and control (57, 61–63). To date, the role of motor representation in motor impairments in children has been mostly studied with regards to DCD (36, 57, 64–66). However, deficits in motor representation could be also reflected in ADHD impairments in motor execution and control, such as timing misperception and reduced accuracy (20, 27, 41), as well as in cognitive tasks (35, 36). For example, children with ADHD (N = 32; M age: 9.9 years) exhibited significantly more errors than TD children (N = 31; M age: 10.2 years) on both visually interrupted and non-interrupted drawing tasks, with a greater difference in the former (41). However, no between-group differences were detected on two basic motor dexterity tasks. The authors concluded that ADHD motor impairments could be due to problems with motor representation more so than any problems with basic motor dexterity issues (41).
3.2 Timing perception
One likely mechanism associated with motor representation is timing perception (herein referred to as timing) of motor tasks (20, 28, 39). Children with ADHD exhibit altered brain activity in areas associated with timing (45, 48), which may underpin impaired rhythmicity (34, 37, 39), greater variability in movement time (20, 27, 28, 39), reduced accuracy (27, 28), and slower reaction time (17, 40). Further, children with ADHD exhibit greater difficulties in following cued (compared to non-cued) and changing (compared to constant) rhythmic movements (27, 37, 39). A study with 545 participants (age range: 5–19 years) evaluated timing properties of manual tasks (externally cued and non-cued maximal speed press-a-button, and tapping) in children with ADHD, their affected and non-affected siblings, and controls (28). Results showed that on the externally cued press-a-button task, children with ADHD and their affected siblings exhibited decreased accuracy and increased variability, while non-affected siblings showed increased variability only, compared to controls. On the non-cued maximal speed press-a-button task, both children with ADHD and their affected siblings exhibited slower and more variable performance, compared to controls. Non-affected siblings exhibited no differences from controls. On the self-generated tapping task, no between-groups differences were detected. The authors suggested that timing variability, accuracy, and speed could be all genetically linked to ADHD etiology, with the former being most clearly associated. Other studies investigated timing properties of jumping tasks in children with ADHD (37, 39). One study compared rope jumping under cued constant and variable tempo among ten children with ADHD (M age: 9.6 ± 1.27 years) and 10 TD (M age: 9.9 ± 1.54 years) (39). The ADHD group exhibited greater hand-foot deviation time (i.e., temporal synchronization) in both constant and variable tasks, as well as increased timing variability of the foot and rope whirling, separately. Another study investigated jumping jacks spatio-temporal kinematics in 17 children with ADHD with DCD (M age: 8.5 ± 1.25 years) and 20 TD children (M age: 9 ± 0.95 years) (37). Results showed that the ADHD with DCD group exhibited greater impairments in upper-lower limbs coordination (measured by number of correct jumps), higher jerk (i.e., reduced movement smoothness), and greater performance time variability, than did the TD group.
4 Current therapies for ADHD
Currently available pharmaceutical and non-pharmaceutical therapies for ADHD (67) focus on a multimodal approach (68, 69) that combines stimulants (e.g., Methylphenidate, Amphetamine) and behavioral therapy (e.g., parental and children training to support children’s positive behaviors (3), neurofeedback (70)). Such a combined approach is considered most effective (1, 4, 67, 71). As per pharmaceutical therapies, approximately 70–80% of individuals with ADHD have been found to respond positively (i.e., decreased symptoms and improved cognitive and behavioral functioning) to stimulants (1, 42, 67). Further, medications have also improved motor execution in children with ADHD, further supporting the role of ADHD core symptoms in motor impairments (19, 34). Studies into non-pharmaceutical—namely physical exercise—therapies for ADHD suggest some positive effects of multi-faceted exercise training programs on short- and long-term physical, cognitive, mental, and social well-being of children with ADHD (72, 73). Some studies, however, lack details regarding interventions’ characteristics (e.g., duration, exercise descriptors), and vary in methodologies (72–74). One study (75) assessed the effects of a moderate- to high-intensity physical activity program on fitness, motor skills, cognitive functions, and ADHD-related behavior in middle school age children with ADHD who did versus did not receive a 10-week physical activity program (including warm-up, progressive aerobic, muscular, and motor skills exercises and cool down). Results showed that the program improved locomotion and raw motor score (measured by the Test of Gross Motor Development, 2nd Edition; TGMD2) (76) and the number of push-ups). However, while current ADHD clinical guidelines refer to assessing co-morbidities, they do not address motor assessment or treatment (2, 4, 6, 15). Therefore, only half of children with ADHD with clinically detected motor impairments are eligible for physical therapy treatments designed to improve motor impairments (8).
Limited research only suggests cognitive training to enhance motor performance in children with ADHD (77, 78). One study (78) assessed the effect of a 12-week intervention of a problem-solving approach [Cognitive Orientation to daily Occupational Performance (Co-Op)] (79) on motor performance (measured by Bruininks–Oseretsky Test of Motor Proficiency; BOTOMP) (55) in children with ADHD (N = 6; age range: 7–12 years) (78). Results showed improvements in motor performance in 5 out of the 6 participants. One research-based approach that merges motor and cognitive components is mental imagery (80). Such an approach could serve as a promising avenue for treating cognitive-motor impairments in children with ADHD, as described below.
5 Mental imagery for addressing motor impairments in ADHD
One promising—yet understudied—approach for addressing motor (35, 81) and non-motor (82) impairments in children with ADHD is mental imagery. Mental imagery is the cognitive process of creating any (e.g., visual, kinesthetic, auditory) experience in the mind (83). Specifically, mentally imaging movement—known as motor imagery (MI)—is typically performed without overt physical execution (84–86). Using and relying on the motor task’s efference copy (i.e., internal model) (59), MI involves predicting and simulating somatosensory and functional consequences of the imaged movement or motor task (57, 58, 87). As such, MI can be used for practicing and enhancing motor planning, execution, and control (57, 88, 89), including when actual movement is impaired or not possible (due to injury, for example). The beneficial effect of MI on motor and non-motor spheres of performance have been widely documented (60, 85, 86, 90). Further, MI serves for studying motor representation as well as a method for updating and enhancing it (89, 91). Such updates following MI could possibly be due, in part, to the absence of motor and non-motor constraints (e.g., physical limitations, fatigue, pain, fear avoidance) that are often associated with physical execution (80). Recent literature has shown the benefits of mental imagery combined with actual movement (92–94). Such cognitive-motor dyads—namely dynamic motor imagery (83, 94, 95) or dynamic neuro-cognitive imagery (DNI) (93, 96)—have been shown to benefit motor performance in various populations. Their potential for ameliorating motor planning, execution, and control in children with ADHD through various mechanisms (e.g., attentional focus) is yet to be revealed.
The interaction between motor representation and timing is particularly relevant within the context of MI (57, 97). Given that both actual and imaged movements involve retrieval of task-specific spatio-temporal information from long term memory (61, 98), both types of movements rely on the same central mechanisms (88), including motor representation (99) and timing. As such, it is not surprising that actual and imaged movements exhibit spatial (i.e., brain activity) (100) and temporal (i.e., time duration; aka chronometry) (101, 102) similarities. Specifically, chronometry provides information about the individual’s temporal organization of the motor task and the ability to preserve it (101), which is one feature of motor representation (103). These spatial and temporal similarities are used for measuring and training cognitive-motor competencies in research and clinical settings.
Children with motor impairments, including ADHD, may experience difficulties in accurately mentally imaging motor tasks (65, 104). Such difficulties could be explained by, at least in part, deficient motor experience that results in compromised motor representation (58, 105). The majority of research into MI in children has focused, however, on DCD (65, 106) and found diminished MI ability in hand mental rotation and visually guided point tasks (57, 97, 104, 107). Such impairments in MI ability are suggested to support the internal deficit model in DCD (59, 97), in which there is a difficulty in “picturing” the desired movement and comparing it to the actual executed movement (106). Limited research into MI abilities in ADHD found reduced MI ability in children with ADHD with and without DCD, with the latter subgroup exhibiting better ability (35, 36). One study compared MI ability (measured by difficulty index, defined as the speed-target width-difficulty relation during real and imaged visually guided point task) among 4 groups of children (age range: 8–12 years): ADHD with DCD (N = 14), ADHD without DCD (N = 14), DCD only (N = 15), and TD (N = 15). Results showed that both ADHD groups and the TD group exhibited better MI ability compared to the DCD only group (36). Further interestingly, both ADHD groups exhibited slower MI compared to the TD group, however faster than the DCD only group. A follow-up study added measures of attention (The Test of Everyday Attention for Children; TEA-Ch (108)), Conners parents’ ratings (109), working memory (Cambridge Neuropsychological Test Automated Battery; CANTAB (110)) as well as a mental rotation task for the same four groups of children (age range: 7–12 years) (35). Results showed reduced accuracy in the mental rotation task in the DCD groups, compared to the TD group. On the visually guided pointed task, both ADHD groups, unlike the DCD only group, imaged movements conformed to the speed-target width difficulty relation. Interestingly, however, no correlations were detected between these results and either attention, sustained attention or working memory. The authors suggested that deficits in MI ability may underlie, or at least contribute to, some of the motor impairments in ADHD. While the association between MI abilities and ADHD motor impairments has not been empirically established, the results of these studies promote MI as a relevant therapeutic avenue for children with ADHD. Specifically, the similarity in MI abilities between children with ADHD with various degrees of motor impairments (i.e., with and without DCD) further supports MI’s suitability for the whole spectrum of ADHD motor impairments. This view aligns with previous recommendations for integrating MI within pediatric rehabilitation (111). Further, the impairments in MI ability detected in children with ADHD compared to TD children should not discourage clinicians from using MI in this population, given previous literature demonstrating gains in participants’ views towards MI and MI abilities, following MI training (112, 113).
As per MI training, a significant body of evidence supports the beneficial effects of MI training on motor execution and control (e.g., movement speed, accuracy) in children (60, 90), including those with DCD (114). One study (81) with 60 adolescents with ADHD (age range: 12–17 years) compared the effect of a single session of four types of interventions (MI, physical practice, combined MI and physical practice, and control) on a dart throwing task. No details regarding the MI contents were provided. Results showed no difference among groups in throwing scores at baseline. The retention (one-day) test showed that the combined intervention resulted in significantly better throwing scores compared to all other groups. The physical practice group had significantly better throwing scores compared to the MI and control groups, and the MI group had significantly better throwing scores than the control group. This study highlights that adolescents with ADHD may benefit from MI for improving motor execution. Another study (82) assessed the effect of a 6-month imagery-movement (“attention education”) training on attention control, using the Conners’ continuous performance test (CPT) for vigilance and attention (115) in 30 children (age range: 6–9 years) with ADHD (intervention: N = 17; control: N = 13). The intervention involved teachers’ participation in guiding the children in attentional control strategies, including 25 exercises of visual, kinesthetic, and auditory MI, and transferring them into academic activities. Some of the exercises included actual movement. Results showed that the intervention group improved in CPT reaction time compared to the control group. Of note, however, no studies to date have explored the simultaneous combination of imagery and movement for addressing motor and cognitive impairments in children with ADHD.
6 Conclusion
Children with ADHD exhibit various degrees of impairment in motor planning, execution, and control, compared to TD children. Assessment of these impairments relies mostly on standardized functional motor tests. Such a reality may overlook children with ADHD who present—based on these clinical tests—no or non-significant impairments, and yet exhibit motor-functional impairments that impede social functioning. Timing perception and internal representation are two suggested—yet under researched—mechanisms to underpin ADHD motor impairments in ADHD. Deficiencies in these mechanisms may impact children’s ability to mentally image movement. If so, children with and without clinically detected motor impairments stand to benefit from using various subtypes of mental imagery. Integrating mental imagery within ADHD management may enhance motor skill planning, execution, and control. Such gains could be due to mental imagery addressing, among others, timing perception and motor representation. The potential of mental imagery in identifying and treating cognitive and motor impairments in ADHD advocates continued research into novel applications for ADHD management and treatment protocols.
Author contributions
AR: Conceptualization, Data curation, Investigation, Methodology, Project administration, Resources, Validation, Visualization, Writing – original draft, Writing – review & editing. EF: Conceptualization, Visualization, Writing – review & editing. YL: Methodology, Writing – review & editing. AA: Conceptualization, Methodology, Supervision, Visualization, Writing – original draft, Writing – review & editing.
Funding
The author(s) declare that no financial support was received for the research, authorship, and/or publication of this article.
Conflict of interest
The authors declare that the research was conducted in the absence of any commercial or financial relationships that could be construed as a potential conflict of interest.
Generative AI statement
The author(s) declare that no Generative AI was used in the creation of this manuscript.
Publisher’s note
All claims expressed in this article are solely those of the authors and do not necessarily represent those of their affiliated organizations, or those of the publisher, the editors and the reviewers. Any product that may be evaluated in this article, or claim that may be made by its manufacturer, is not guaranteed or endorsed by the publisher.
References
1. Antshel, KM, and Barkley, R. Attention deficit hyperactivity disorder. Handb Clin Neurol. (2020) 174:37–45. doi: 10.1016/B978-0-444-64148-9.00003-X
2. Bélanger, SA, Andrews, D, Gray, C, and Korczak, D. ADHD in children and youth: part 1-etiology, diagnosis, and comorbidity. Paediatr Child Health (Canada). (2018) 23:447–53. doi: 10.1093/pch/pxy109
3. Childress, AC, and Stark, JG. Diagnosis and treatment of attention-deficit/hyperactivity disorder in preschool-aged children. J Child Adolesc Psychopharmacol. (2018) 28:606–14. doi: 10.1089/cap.2018.0057
4. Faraone, SV, Banaschewski, T, Coghill, D, Zheng, Y, Biederman, J, Bellgrove, MA, et al. The world federation of ADHD international consensus statement: 208 evidence-based conclusions about the disorder. Neurosci Biobehav Rev. (2021) 128:789–818. doi: 10.1016/j.neubiorev.2021.01.022
5. Gallo, EF, and Posner, J. Moving towards causality in attention-deficit hyperactivity disorder: overview of neural and genetic mechanisms. Lancet Psychiatry. (2016) 3:555–67. doi: 10.1016/S2215-0366(16)00096-1
6. Wolraich, ML, Hagan, JF, Allan, C, and Chan, E. Clinical practice guideline for the diagnosis, evaluation, and treatment of ADHD in children and adolescents. Dev Pediatr. (2015) 144:54–4. doi: 10.1542/peds.2019-2528
7. American Psychiatric Association. Diagnostic and statistical manual of mental disorders. 5th edition. Washington DC: American Psychiatric Association; (2013). 59–66.
8. Fliers, E, Franke, B, Lambregts-Rommelse, N, Altink, M, Buschgens, C, Nijhuis-van der Sanden, M, et al. Undertreatment of motor problems in children with ADHD. Child Adolesc Ment Health. (2010) 15:85–90. doi: 10.1111/j.1475-3588.2009.00538.x
9. Goulardins, JB, Marques, JCB, and De Oliveira, JA. Attention deficit hyperactivity disorder and motor impairment: A critical review. Percept Mot Skills. (2017) 124:425–40. doi: 10.1177/0031512517690607
10. Kaiser, ML, Schoemaker, MM, Albaret, JM, and Geuze, RH. What is the evidence of impaired motor skills and motor control among children with attention deficit hyperactivity disorder (ADHD)? Systematic review of the literature. Res Dev Disabil. (2015) 36:338–57. doi: 10.1016/j.ridd.2014.09.023
11. Valori, I, Della Longa, L, Angeli, A, Marfia, G, and Farroni, T. Reduced motor planning underlying inhibition of prepotent responses in children with ADHD. Sci Rep. (2022) 12:18202–11. doi: 10.1038/s41598-022-22318-6
12. Larsson, H, Dilshad, R, Lichtenstein, P, and Barker, ED. Developmental trajectories of DSM-IV symptoms of attention-deficit/ hyperactivity disorder: genetic effects, family risk and associated psychopathology. J Child Psychol Psychiatry. (2011) 52:954–63. doi: 10.1111/j.1469-7610.2011.02379.x
13. Coghill, D, Banaschewski, T, Cortese, S, Asherson, P, Brandeis, D, Buitelaar, J, et al. The management of ADHD in children and adolescents: bringing evidence to the clinic: perspective from the European ADHD guidelines group (EAGG). Eur Child Adolesc Psychiatry. (2021) 32:1337–61. doi: 10.1007/s00787-021-01871-x
14. Kleeren, L, Hallemans, A, Hoskens, J, Klingels, K, Smits-Engelsman, B, and Verbecque, E. A critical view on motor-based interventions to improve motor skill performance in children with ADHD: A systematic review and meta-analysis. J Atten Disord. (2023) 27:354–67. doi: 10.1177/10870547221146244
15. Kooij, JJS, Bijlenga, D, Salerno, L, Jaeschke, R, Bitter, I, Balázs, J, et al. Updated European consensus statement on diagnosis and treatment of adult ADHD. Eur Psychiatry. (2019) 56:14–34. doi: 10.1016/j.eurpsy.2018.11.001
16. Dahan, A, Ryder, CH, and Reiner, M. Components of motor deficiencies in ADHD and possible interventions. Neuroscience. (2018) 378:34–53. doi: 10.1016/j.neuroscience.2016.05.040
17. Klimkeit, EI, Mattingley, JB, Sheppard, DM, Lee, P, and Bradshaw, JL. Motor preparation, motor execution, attention, and executive functions in attention deficit/hyperactivity disorder (ADHD). Child Neuropsychol. (2005) 11:153–73. doi: 10.1080/092970490911298
18. Adhvaryu, KP, Karthikbabu, S, and Rao, PT. Motor performance of children with attention deficit hyperactivity disorder: focus on the Bruininks-Oseretsky test of motor proficiency. Clin Exp Pediatr. (2022) 65:512–20. doi: 10.3345/cep.2021.00962
19. Brossard-Racine, M, Shevell, M, Snider, L, Bélanger, SA, and Majnemer, A. Motor skills of children newly diagnosed with attention deficit hyperactivity disorder prior to and following treatment with stimulant medication. Res Dev Disabil. (2012) 33:2080–7. doi: 10.1016/j.ridd.2012.06.003
20. Hotham, E, Haberfield, M, Hillier, S, White, JM, and Todd, G. Upper limb function in children with attention-deficit/hyperactivity disorder (ADHD). J Neural Transm. (2018) 125:713–26. doi: 10.1007/s00702-017-1822-8
21. Piek, JP, Pitcher, TM, and David, AH. Motor coordination and kinaesthesis in boys with attention deficit-hyperactivity disorder. Dev Med Child Neurol. (1999) 41:159–65. doi: 10.1111/j.1469-8749.1999.tb00575.x
22. Tseng, H, and Chow, Y. Relationship between motor proficiency, attention, impulse, and activity in children with ADHD. Dev Med Child Neurol. (2004) 46:381–8. doi: 10.1017/S0012162204000623
23. Watemberg, N, Waiserberg, N, Zuk, L, and Lerman-Sagie, T. Developmental coordination disorder in children with attention-deficit-hyperactivity disorder and physical therapy intervention. Dev Med Child Neurol. (2007) 49:920–5. doi: 10.1111/j.1469-8749.2007.00920.x
24. Raab, M, Johnson, JG, and Heekeren, HR.(Eds.). Mind and motion: the bidirectional link between thought and action. Prog Brain Res. Elsevier. (2009). 174.
25. Pitcher, TM, Piek, JP, and Hay, DA. Fine and gross motor ability in males with ADHD. Dev Med Child Neurol. (2003) 45:525–35. doi: 10.1111/j.1469-8749.2003.tb00952.x
26. Villa, M, Barriopedro, MI, and Ruiz, LM. Motor competence difficulties and attention deficit and hyperactivity disorder (ADHD) among secondary students TT – Problemas de competencia motriz y el trastorno por déficit de atención e hiperactividad (TDAH) entre los estudiantes de Secundaria TT – P. Cuad Psicol Deporte. (2020) 20:47–62. doi: 10.6018/cpd.360491
27. Eliasson, AC, Rösblad, B, and Forssberg, H. Disturbances in programming goal-directed arm movements in children with ADHD. Dev Med Child Neurol. (2004) 46:19–27. doi: 10.1111/j.1469-8749.2004.tb00429.x
28. Rommelse, NNJ, Altink, ME, Oosterlaan, J, Beem, L, Buschgens, CJM, Buitelaar, J, et al. Speed, variability, and timing of motor output in ADHD: which measures are useful for endophenotypic research? Behav Genet. (2008) 38:121–32. doi: 10.1007/s10519-007-9186-8
29. Henderson, S, and Sugden, D. The movement assessment battery for children. London: The Psychological Corporation (1992).
30. Bruininks, RH, and Bruininks, BD. Bruininks-Oseretsky test of motor proficiency, second edition (BOT-2). Pearson Assessments, USA: APA PsycTests (2005).
31. Blank, R, Barnett, AL, Cairney, J, Green, D, Kirby, A, Polatajko, H, et al. International clinical practice recommendations on the definition, diagnosis, assessment, intervention, and psychosocial aspects of developmental coordination disorder. Dev Med Child Neurol. (2019) 61:242–85. doi: 10.1111/dmcn.14132
32. Buker, N, Salik Sengul, Y, and Ozbek, A. Physical fitness and dynamic balance in medication Naïve Turkish children with ADHD. Percept Mot Skills. (2020) 127:858–73. doi: 10.1177/0031512520938517
33. Harvey, WJ, Reid, G, Grizenko, N, Mbekou, V, Ter-Stepanian, M, and Joober, R. Fundamental movement skills and children with attention-deficit hyperactivity disorder: peer comparisons and stimulant effects. J Abnorm Child Psychol. (2007) 35:871–82. doi: 10.1007/s10802-007-9140-5
34. Leitner, Y, Barak, R, Giladi, N, Peretz, C, Eshel, R, Gruendlinger, L, et al. Gait in attention deficit hyperactivity disorder: effects of methylphenidate and dual tasking. J Neurol. (2007) 254:1330–8. doi: 10.1007/s00415-006-0522-3
35. Williams, J, Omizzolo, C, Galea, MP, and Vance, A. Motor imagery skills of children with attention deficit hyperactivity disorder and developmental coordination disorder. Hum Mov Sci. (2013) 32:121–35. doi: 10.1016/j.humov.2012.08.003
36. Lewis, M, Vance, A, Maruff, P, Wilson, P, and Cairney, S. Differences in motor imagery between children with developmental coordination disorder with and without the combined type of ADHD. Dev Med Child Neurol. (2008) 50:608–12. doi: 10.1111/j.1469-8749.2008.03030.x
37. Ricci, M, Terribili, M, Giannini, F, Errico, V, Pallotti, A, Galasso, C, et al. Wearable-based electronics to objectively support diagnosis of motor impairments in school-aged children. J Biomech. (2019) 83:243–52. doi: 10.1016/j.jbiomech.2018.12.005
38. Henderson, SE, Sugden, D, and Barnett, AL. Movement assessment battery for Children-2. London: Pearson (2007).
39. Chen, YY, Liaw, LJ, Liang, JM, Hung, WT, Guo, LY, and Wu, WL. Timing perception and motor coordination on rope jumping in children with attention deficit hyperactivity disorder. Phys Ther Sport. (2013) 14:105–9. doi: 10.1016/j.ptsp.2012.03.012
40. Shiels Rosch, K, Dirlikov, B, and Mostofsky, SH. Increased intrasubject variability in boys with ADHD across tests of motor and cognitive control. J Abnorm Child Psychol. (2013) 41:485–95. doi: 10.1007/s10802-012-9690-z
41. Macoun, SJ, and Kerns, KA. Evidence of motor-control difficulties in children with attention deficit hyperactivity disorder, explored through a hierarchical motor-systems perspective. J Clin Exp Neuropsychol. (2016) 38:183–96. doi: 10.1080/13803395.2015.1094028
42. Barkley, RA. Deficits in executive functioning scale--children and adolescents (BDEFS-CA). New York: Guilford Press (2012).
43. Barkley, RA. Behavioral inhibition, sustained attention, and executive functions: constructing a unifying theory of ADHD. Psychol Bull. (1997) 121:65–94. doi: 10.1037/0033-2909.121.1.65
44. Ziereis, S, and Jansen, P. Correlation of motor abilities and executive functions in children with ADHD. Appl Neuropsychol Child. (2016) 5:138–48. doi: 10.1080/21622965.2015.1038746
45. Hart, H, Radua, J, Mataix-Cols, D, and Rubia, K. Meta-analysis of fMRI studies of timing in attention-deficit hyperactivity disorder (ADHD). Neurosci Biobehav Rev. (2012) 36:2248–56. doi: 10.1016/j.neubiorev.2012.08.003
46. Anat, D, and Miriam, R. Evidence for deficient motor planning in ADHD. Sci Rep. (2017) 7:9631–10. doi: 10.1038/s41598-017-09984-7
47. Halliday, DWR, Kim, Y, MacDonald, SWS, Garcia-Barrera, MA, Hundza, SR, and Macoun, SJ. Intraindividual variability in executive and motor control tasks in children with attention deficit hyperactivity disorder. J Clin Exp Neuropsychol. (2021) 43:568–78. doi: 10.1080/13803395.2021.1965097
48. Rubia, K. Cognitive neuroscience of attention deficit hyperactivity disorder (ADHD) and its clinical translation. Front Hum Neurosci. (2018) 12:1–23. doi: 10.3389/fnhum.2018.00100
49. Brossard-Racine, M, Majnemer, A, and Shevell, MI. Exploring the neural mechanisms that underlie motor difficulties in children with attention deficit hyperactivity disorder. Dev Neurorehabil. (2011) 14:101–11. doi: 10.3109/17518423.2010.547545
50. Mehta, TR, Monegro, A, Nene, Y, Fayyaz, M, and Bollu, PC. Neurobiology of ADHD: A review. Curr Dev Disord Rep. (2019) 6:235–40. doi: 10.1007/s40474-019-00182-w
51. Sonuga-Barke, E, Bitsakou, P, and Thompson, M. Beyond the dual pathway model: evidence for the dissociation of timing, inhibitory, and delay-related impairments in attention-deficit/hyperactivity disorder. J Am Acad Child Adolesc Psychiatry. (2010) 49:345–55. doi: 10.1016/j.jaac.2009.12.018
52. Farmer, M, Echenne, B, Drouin, R, and Bentourkia, M. Insights in developmental coordination disorder. Curr Pediatr Rev. (2017) 13:111–9. doi: 10.2174/1573396313666170726113550
53. Ferguson, GD, Jelsma, J, Versfeld, P, and Smits-Engelsman, BCM. Using the ICF framework to explore the multiple interacting factors associated with developmental coordination disorder. Curr Dev Disord Rep. (2014) 1:86–101. doi: 10.1007/s40474-014-0013-7
54. van Cappellen-van Maldegem, SJM, van Abswoude, F, Krajenbrink, H, and Steenbergen, B. Motor learning in children with developmental coordination disorder: the role of focus of attention and working memory. Hum Mov Sci. (2018) 62:211–20. doi: 10.1016/j.humov.2018.11.001
55. Bruininks, RH, and Bruininks, BD. Bruininks-Oseretsky test of motor proficiency APA PsycTests (1978). Minnesota, USA: Circle Pines.
56. Hinshaw, SP. Attention deficit hyperactivity disorder (ADHD): controversy, developmental mechanisms, and multiple levels of analysis. Annu Rev Clin Psychol. (2018) 14:291–316. doi: 10.1146/annurev-clinpsy-050817-084917
57. Gabbard, C. Studying action representation in children via motor imagery. Brain Cogn. (2009) 71:234–9. doi: 10.1016/j.bandc.2009.08.011
58. Schack, T, and Frank, C. Mental representation and the cognitive architecture of skilled action. Rev Philos Psychol. (2021) 12:527–46. doi: 10.1007/s13164-020-00485-7
59. Wolpert, DM. In bilingual computational approaches control. Nature. (1997) 1:209–16. doi: 10.1016/S1364-6613(97)01070-X
60. Behrendt, F, Zumbrunnen, V, Brem, L, Suica, Z, Gäumann, S, Ziller, C, et al. Effect of motor imagery training on motor learning in children and adolescents: A systematic review and meta-analysis. Int J Environ Res Public Health. (2021) 18:9467. doi: 10.3390/ijerph18189467
61. Schack, T. The cognitive architecture of complex movement. Int J Sport Exerc Psychol. (2004) 2:403–38. doi: 10.1080/1612197X.2004.9671753
62. Schack, T. Measuring mental representations In: G Tenenbaum, RC Eklund, and A Kamata, editors. Measurement in sport and exercise psychology. Champaign, IL: Human Kinetics (2012)
63. Schack, T, and Ritter, H. The cognitive nature of action — functional links between cognitive psychology, movement science, and robotics. Prog Brain Res. (2009) 174:231–50. doi: 10.1016/S0079-6123(09)01319-3
64. Adams, ILJ, Steenbergen, B, Lust, JM, and Smits-Engelsman, BCM. Motor imagery training for children with developmental coordination disorder – study protocol for a randomized controlled trial. BMC Neurol. (2016) 16:1–9. doi: 10.1186/s12883-016-0530-6
65. Barhoun, P, Fuelscher, I, Kothe, EJ, He, JL, Youssef, GJ, Enticott, PG, et al. Motor imagery in children with DCD: A systematic and meta-analytic review of hand-rotation task performance. Neurosci Biobehav Rev. (2019) 99:282–97. doi: 10.1016/j.neubiorev.2019.02.002
66. Wilson, PH, Adams, ILJ, Caeyenberghs, K, Thomas, P, Smits-Engelsman, B, and Steenbergen, B. Motor imagery training enhances motor skill in children with DCD: A replication study. Res Dev Disabil. (2016) 57:54–62. doi: 10.1016/j.ridd.2016.06.014
67. Catalá-López, F, Hutton, B, Núñez-Beltrán, A, Page, MJ, Ridao, M, Saint-Gerons, DM, et al. The pharmacological and non-pharmacological treatment of attention deficit hyperactivity disorder in children and adolescents: A systematic review with network meta-analyses of randomised trials. PLoS One. (2017) 12:1–31. doi: 10.1371/journal.pone.0180355
68. Hinshaw, SP, and Arnold, LE. Attention-deficit hyperactivity disorder, multimodal treatment, and longitudinal outcome: evidence, paradox, and challenge. Wiley Interdiscip Rev Cogn Sci. (2015) 6:39–52. doi: 10.1002/wcs.1324
69. Ritchers, J, Arnold, L, Jensen, P, Abikoff, H, Conners, C, Green hill, L, et al. NIMH collaborative multisite multimodal treatment study of children with ADHD: I. Background and rationale. J Am Acad Child Adolesc Psychiatry. (1995) 34:987–1000. doi: 10.1097/00004583-199508000-00008
70. Van Doren, J, Arns, M, Heinrich, H, Vollebregt, MA, Strehl, UK, and Loo, S. Sustained effects of neurofeedback in ADHD: a systematic review and meta-analysis. Eur Child Adolesc Psychiatry. (2019) 28:293–305. doi: 10.1007/s00787-018-1121-4
71. Ng, QX, Ho, CYX, Chan, HW, Yong, BZJ, and Yeo, WS. Managing childhood and adolescent attention-deficit/hyperactivity disorder (ADHD) with exercise: A systematic review. Complement Ther Med. (2017) 34:123–8. doi: 10.1016/j.ctim.2017.08.018
72. Neudecker, C, Mewes, N, Reimers, AK, and Woll, A. Exercise interventions in children and adolescents with ADHD: A systematic review. J Atten Disord. (2019) 23:307–24. doi: 10.1177/1087054715584053
73. Den Heijer, AE, Groen, Y, Tucha, L, Fuermaier, ABM, Koerts, J, Lange, KW, et al. Sweat it out? The effects of physical exercise on cognition and behavior in children and adults with ADHD: a systematic literature review. J Neural Transm. (2017) 124:3–26. doi: 10.1007/s00702-016-1593-7
74. Suarez-Manzano, S, Ruiz-Ariza, A, De La Torre-Cruz, M, and Martínez-López, EJ. Acute and chronic effect of physical activity on cognition and behaviour in young people with ADHD: A systematic review of intervention studies. Res Dev Disabil. (2018) 77:12–23. doi: 10.1016/j.ridd.2018.03.015
75. Chevalier, N, Parent, V, Rouillard, M, Simard, F, Guay, MC, and Verret, C. The impact of a motor-cognitive remediation program on attentional functions of preschoolers with ADHD symptoms. J Atten Disord. (2017) 21:1121–9. doi: 10.1177/1087054712468485
77. Frisch, C, Tirosh, E, and Rosenblum, S. Parental occupation executive training (POET): an efficient innovative intervention for Young children with attention deficit hyperactive disorder. Phys Occup Ther Pediatr. (2020) 40:47–61. doi: 10.1080/01942638.2019.1640336
78. Gharebaghy, S, Rassafiani, M, and Cameron, D. Effect of cognitive intervention on children with ADHD. Phys Occup Ther Pediatr. (2015) 35:13–23. doi: 10.3109/01942638.2014.957428
79. Missiuna, C, Mandich, AD, Polatajko, HJ, and Malloy, T. Cognitive orientation to daily occupational performance (CO-OP): Part I- theoretical foundations. Phys Occup Ther Pediatr. (2001) 20:69–81. doi: 10.1080/J006v20n02_05
80. Jeannerod, M. Mental imagery in the motor context. Neuropsychologia. (1995) 33:1419–32. doi: 10.1016/0028-3932(95)00073-C
81. Salehian, HH, Hosseinzadeh Peygan, R, Shafaeianfard, F, and Khajeaflaton, MS. The effects of mental imagery and physical practice on learning dart-throwing in children with ADHD. J Mod Psychol. (2022) 2:1–7. Available from: http://creativecommons.org/licenses/by/4.0
82. Chevalier, N, Poissant, H, Bergeron, H, and Girard-Lajoie, A. The effect of visual-motor imagery training on CPT performance in children with attention deficit hyperactivity disorder. J Cogn Educ Psychol. (2003) 3:120–36. doi: 10.1891/194589503787383091
83. Kosslyn, SM, Ganis, G, and Thompson, WL. Neural foundations of imagery. Nat Rev Neurosci. (2001) 2:635–42. doi: 10.1038/35090055
84. Jeannerod, M, and Decety, J. Mental motor imagery: a window into the representational stages of action. Curr Opin Neurobiol. (1995) 5:727–32. doi: 10.1016/0959-4388(95)80099-9
85. Ladda, AM, Lebon, F, and Lotze, M. Using motor imagery practice for improving motor performance – A review. Brain Cogn. (2021) 150:105705. doi: 10.1016/j.bandc.2021.105705
86. Lotze, M, and Halsband, U. Motor imagery. J Physiol Paris. (2006) 99:386–95. doi: 10.1016/j.jphysparis.2006.03.012
87. Kilteni, K, Andersson, BJ, Houborg, C, and Ehrsson, HH. Motor imagery involves predicting the sensory consequences of the imagined movement. Nat Commun. (2018) 9:1617–9. doi: 10.1038/s41467-018-03989-0
88. Frank, C, Land, WM, Popp, C, and Schack, T. Mental representation and mental practice: experimental investigation on the functional links between motor memory and motor imagery. PLoS One. (2014) 9:e95175. Available from. doi: 10.1371/journal.pone.0095175
89. Schack, T, Essig, K, Frank, C, and Koester, D. Mental representation and motor imagery training. Front Hum Neurosci. (2014) 8:1–10. doi: 10.3389/fnhum.2014.00328
90. Gentile, AE, Rinella, S, Desogus, E, Verrelli, CM, Iosa, M, Perciavalle, V, et al. Motor imagery for paediatric neurorehabilitation: How much do we know? Perspectives from a systematic review. Front Hum Neurosci. (2024) 18, 18:1245707. doi: 10.3389/fnhum.2024.1245707
91. Munzert, J, Lorey, B, and Zentgraf, K. Cognitive motor processes: the role of motor imagery in the study of motor representations. Brain Res Rev. (2009) 60:306–26. doi: 10.1016/j.brainresrev.2008.12.024
92. Abraham, A, Hart, A, Andrade, I, and Hackney, ME. Dynamic neuro-cognitive imagery improves mental imagery ability, disease severity, and motor and cognitive functions in people with Parkinson’s disease. Neural Plast. (2018) 2018:1–15. doi: 10.1155/2018/6168507
93. Abraham, A, Hart, A, Dickstein, R, and Hackney, ME. Will you draw me a pelvis?ˮ Dynamic neuro-cognitive imagery improves pelvic schema and graphic-metric representation in people with Parkinsonʼs disease: A randomized controlled trial. Complement Ther Med. (2019) 43:28–35. doi: 10.1016/j.ctim.2018.11.020
94. Guillot, A, Moschberger, K, and Collet, C. Coupling movement with imagery as a new perspective for motor imagery practice. Behav Brain Funct. (2013) 9:8. doi: 10.1186/1744-9081-9-8
95. Fusco, A, Iasevoli, L, Iosa, M, Gallotta, MC, Padua, L, Tucci, L, et al. Dynamic motor imagery mentally simulates uncommon real locomotion better than static motor imagery both in young adults and elderly. PLoS One. (2018) 14:e0218378. doi: 10.1371/journal.pone.0218378
96. Heiland, T, and Rovetti, R. Examining effects of Franklin method metaphorical and anatomical mental images on college dancers’ jumping height. Res Dance Educ. (2013) 14:141–61. doi: 10.1080/14647893.2012.712105
97. Adams, ILJ, Lust, JM, Wilson, PH, and Steenbergen, B. Compromised motor control in children with DCD: A deficit in the internal model?—A systematic review. Neurosci Biobehav Rev. (2014) 47:225–44. doi: 10.1016/j.neubiorev.2014.08.011
98. Kim, T, Park, H, and Schack, T. A functional link between mental representation in long-term memory and cognitive performance in working memory. Adv Cogn Psychol. (2019) 15:11–20. doi: 10.5709/acp-0252-y
99. Calmels, C, Holmes, P, Lopez, E, and Naman, V. Chronometric comparison of actual and imaged complex movement patterns. J Mot Behav. (2006) 38:339–48. doi: 10.3200/JMBR.38.5.339-348
100. Hardwick, RM, Caspers, S, Eickhoff, SB, and Swinnen, SP. Neural correlates of action: comparing meta-analyses of imagery, observation, and execution. Neurosci Biobehav Rev. (2018) 94:31–44. doi: 10.1016/j.neubiorev.2018.08.003
101. Guillot, A, Hoyek, N, Louis, M, and Collet, C. Understanding the timing of motor imagery: recent findings and future directions. Int Rev Sport Exerc Psychol. (2012) 5:3–22. doi: 10.1080/1750984X.2011.623787
102. Holmes, PS, and Collins, DJ. The PETTLEP approach to motor imagery: A functional equivalence model for sport psychologists. J Appl Sport Psychol. (2001) 13:60–83. doi: 10.1080/10413200109339004
103. Summers, JJ. The role of timing in motor program representation. J Mot Behav. (1975) 7:229–41. doi: 10.1080/00222895.1975.10735040
104. Williams, J, Thomas, PR, Maruff, P, and Wilson, PH. The link between motor impairment level and motor imagery ability in children with developmental coordination disorder. Hum Mov Sci. (2008) 27:270–85. doi: 10.1016/j.humov.2008.02.008
105. Gromeier, M, Schack, T, and Koester, D. Effects of age and expertise on mental representation of the throwing movement among 6- to 16-year-olds. Front Psychol. (2022) 13:13. doi: 10.3389/fpsyg.2022.799316
106. Scott, MW, Wood, G, Holmes, PS, Williams, J, Marshall, B, and Wright, DJ. Combined action observation and motor imagery: an intervention to combat the neural and behavioural deficits associated with developmental coordination disorder. Neurosci Biobehav Rev. (2021) 127:638–46. doi: 10.1016/j.neubiorev.2021.05.015
107. Maruff, P, Wilson, P, Trebilcock, M, and Currie, J. Abnormalities of imagined motor sequences in children with developmental coordination disorder. Neuropsychologia. (1999) 37:1317–24. doi: 10.1016/S0028-3932(99)00016-0
108. Manly, T. The test of everyday attention for children (TEA-CH). Bury St Edmonds UK: Thames Valley Test Company (1999).
110. Robins, TW. Cambridge neuropsychological test automated battery (CANTAB). London: The Institution of Electrical Engineers (1993).
111. Spruijt, S, van der Kamp, J, and Steenbergen, B. Current insights in the development of children’s motor imagery ability. Front Psychol. (2015) 6:6. doi: 10.3389/fpsyg.2015.00787
112. Abraham, A, Dunsky, A, and Dickstein, R. The effect of motor imagery practice on Elevé performance in adolescent female dance students: A randomized controlled trial. J Imag Res Sport Phys Act. (2017) 12:20160006. doi: 10.1515/jirspa-2016-0006
113. Abraham, A, Gose, R, Schindler, R, Nelson, BH, and Hackney, ME. Dynamic neuro-cognitive imagery (DNI™) improves Developpé performance, kinematics, and mental imagery ability in university-level dance students. Front Psychol. (2019) 10:10. doi: 10.3389/fpsyg.2019.00382
114. Wilson, P, Maruff, P, Butson, M, Williams, J, Lum, J, and Thomas, P. Internal representation of movement in children with developmental coordination disorder: a mental rotation task. Dev Med Child Neurol. (2007) 46:754–9. doi: 10.1111/j.1469-8749.2004.tb00995.x
Keywords: ADHD, cognition, motor impairments, internal motor representation, timing, mental imagery, motor imagery
Citation: Ron Baum A, Franklin E, Leitner Y and Abraham A (2024) Mental imagery for addressing mechanisms underlying motor impairments in children with attention deficit hyperactivity disorder (ADHD). Front. Neurol. 15:1501871. doi: 10.3389/fneur.2024.1501871
Edited by:
Daniel L. Eaves, Newcastle University, United KingdomReviewed by:
Luigi Bianchi, University of Rome Tor Vergata, ItalyKishor Lakshminarayanan, Vellore Institute of Technology (VIT), India
Copyright © 2024 Ron Baum, Franklin, Leitner and Abraham. This is an open-access article distributed under the terms of the Creative Commons Attribution License (CC BY). The use, distribution or reproduction in other forums is permitted, provided the original author(s) and the copyright owner(s) are credited and that the original publication in this journal is cited, in accordance with accepted academic practice. No use, distribution or reproduction is permitted which does not comply with these terms.
*Correspondence: Amit Abraham, YW1pdGFiQGFyaWVsLmFjLmls