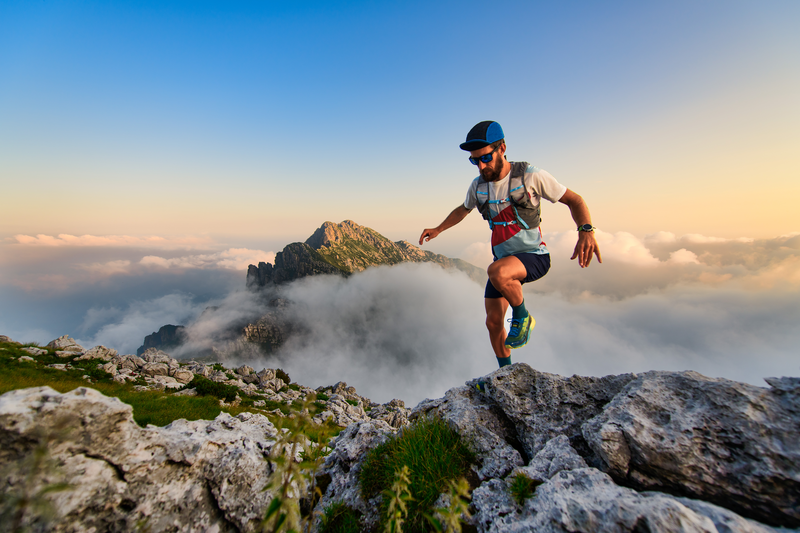
95% of researchers rate our articles as excellent or good
Learn more about the work of our research integrity team to safeguard the quality of each article we publish.
Find out more
ORIGINAL RESEARCH article
Front. Neurol. , 24 December 2024
Sec. Neurorehabilitation
Volume 15 - 2024 | https://doi.org/10.3389/fneur.2024.1482266
This article is part of the Research Topic Rehabilitation Interventions for Mild Traumatic Brain Injury View all 5 articles
Background: Although guidelines support aerobic exercise in sub-acute mild traumatic brain injury (mTBI), evidence for adults with persisting post-concussive symptoms (PPCS) after mTBI is lacking. The objective was to evaluate the impact of a sub-symptom threshold aerobic exercise intervention on overall symptom burden and quality of life in adults with PPCS.
Methods: This prospective cohort study was nested within the ACTBI Trial (Aerobic Exercise for treatment of Chronic symptoms following mild Traumatic Brain Injury). A total of 50 adults with a diagnosis of mTBI, PPCS and exercise intolerance completed a 12-week sub-symptom threshold aerobic exercise intervention either immediately after enrollment (i-AEP group; n = 27) or following 6-weeks of stretching (d-AEP group; n = 23). Data from all participants (n = 50) were included in the combined AEP (c-AEP) group. The primary outcome was symptom burden on the Rivermead Post Concussion Symptoms Questionnaire (RPQ). Secondary outcomes included measures of quality of life and specific post-concussive symptoms (depressive and anxiety symptoms, functional impact of headache, fatigue, sleep, dizziness and exercise tolerance). Heart rate, blood pressure and heart rate variability were also assessed to understand autonomic function response to intervention.
Results: Participants were a mean (SD) of 42.6 (10.9) years old (74% female) and 25.1 (14.1) months post-mTBI. Following 12-weeks of intervention participants had a significant improvement in symptom burden on the RPQ (i-AEP: mean change = −9.415, p < 0.001; d-AEP: mean change = −3.478, p = 0.034; c-AEP: mean change = −6.446, p < 0.001). Participants also had significant improvement in quality of life (i-AEP: mean change = 9.879, p < 0.001; d-AEP: mean change = 7.994, p < 0.001, c-AEP: mean change = 8.937, p < 0.001), dizziness (i-AEP: mean change = −11.159, p = 0.001; d-AEP: mean change = −6.516, p = 0.019; c-AEP: −8.837, p < 0.001) and exercise tolerance (i-AEP: mean change = 5.987, p < 0.001; d-AEP: mean change = 3.421, p < 0.001; c-AEP: mean change = 4.703, p < 0.001). Headache (mean change = −5.522, p < 0.001) and depressive symptoms (mean change = −3.032, p = 0.001) improved in the i-AEP group. There was no change in measures of autonomic function.
Conclusion: A 12-week aerobic exercise intervention improves overall symptom burden, quality of life and specific symptom domains in adults with PPCS. Clinicians should consider prescription of progressive, individualized, sub-symptom threshold aerobic exercise for adults with PPCS even if presenting with exercise intolerance and months-to-years of symptoms.
Mild traumatic brain injury (mTBI) and the resulting persisting symptoms that individuals may experience places significant demands on the healthcare system and rehabilitation services. Adults who do not recover from mTBI in the expected 28 days (1, 2) may go on to experience a diverse array of symptoms grouped under the diagnosis of persisting post-concussive symptoms (PPCS) (3). PPCS commonly presents with headaches, dizziness, fatigue, low mood and sleep disturbance, in addition to exercise intolerance (4–6). Exercise intolerance, defined as worsening of post-concussive symptoms with exertion, can limit engagement in daily activities and re-incorporation of exercise into one’s routine (6, 7). In adults acutely post-injury (within 7 days) exercise intolerance has been associated with symptom burden (number of symptoms and symptom severity) (8). Fear avoidance and kinesiophobia may also play a role in delayed return to activity in those with PPCS (9–11). In adults presenting to outpatient brain injury services, the majority of individuals report not having returned to their pre-injury level of physical activity with only 28% self-reporting meeting physical activity guidelines at time of clinic presentation (12).
At present, the treatment for PPCS is largely symptom-based. While advances have been made in the treatment of PPCS, specifically pharmacologic interventions for post-traumatic headache (13, 14), few non-pharmacologic treatment modalities have shown benefit across multiple symptom domains (15, 16). In non-head injured populations, exercise has shown benefits for sleep (17–19), depression (20, 21), anxiety (22) and headache (23, 24), all of which are common post-concussive symptoms. Therefore, prescription of aerobic exercise is a non-pharmacologic, accessible intervention with the potential to play a role in PPCS rehabilitation. Pediatric studies have shown that adolescents who initiate graded, sub-symptom threshold aerobic exercise within 10 days of injury recover more quickly than those who complete a stretching program (25, 26). Based on degree of exercise tolerance, sub-symptom threshold exercise is prescribed with the goal of being tolerated by patients and not worsening symptoms. Exercise intensity can then be incrementally increased with monitoring.
The majority of past interventions evaluating exercise for rehabilitation post-mTBI have been implemented in the acute phase following injury in adolescents with sport-related concussion (25–27). Few studies have evaluated the role of prescribed exercise in rehabilitation for adults following mTBI; research thus far has either enrolled acutely concussed service members (28) or evaluated a walking intervention (29). Therefore, despite adult clinical guidelines for mTBI recovery/treatment recommending exercise (15, 30, 31), there is little evidence in adult populations with PPCS to guide clinicians in prescribing this exercise. Further, in the chronic phase of injury (>3 months post-injury) there are unique considerations for implementation of exercise interventions, such as tolerability (in those with exercise intolerance), de-conditioning and functional limitations. Here, we examine a structured, personalized 12-week aerobic exercise intervention for improvement in symptom burden for adults with PPCS with and without a precursory 6-week stretching program.
While the underlying pathophysiology of exercise intolerance is poorly understood, altered autonomic function and altered cerebral blood flow response to exertion are two possible theories (32, 33). Heart rate variability (HRV) is the beat-to-beat variation in heart rate (HR) that allows for adequate response to external stimuli (34). HRV is commonly used as a measure of autonomic function as its frequency-domain metrics approximate the contributions of the sympathetic and parasympathetic nervous system (34). Further, it is a non-invasive metric that can be measured longitudinally. Compared to age and sex-matched controls, adults with a history of mTBI have been shown to have lower HRV (35). HRV has a well established relationship with physical activity with increased activity associated with a decreased resting HR and corresponding increase in time-domain HRV (36, 37). However, the ability of aerobic exercise to modulate autonomic function in adults with PPCS has not previously been evaluated. In this study, HR, HRV and blood pressure (BP) were used to examine physiologic response to the intervention and potential for aerobic exercise to specifically improve HRV in this patient population.
The presented prospective cohort study evaluating a 12-week immediate-start aerobic exercise protocol (i-AEP) and delayed-start aerobic exercise protocol (d-AEP) aimed to: (a) evaluate change in symptom burden, quality of life (QoL) and specific post-concussive symptom outcomes; and (b) evaluate change in outcomes between those in the i-AEP and d-AEP groups.
This prospective cohort study was nested within the Aerobic Exercise for treatment of Chronic symptoms following mild Traumatic Brain Injury (ACTBI) Trial. ACTBI study methods have previously been published (38). This study was approved by the University of Calgary Conjoint Research Ethics Board (REB18-1329) and the manuscript adheres to the STROBE checklist for reporting of observational studies. The ACTBI Trial protocol was registered on clinicaltrials.gov (NCT03895450).
Participants were recruited from outpatient brain injury, pain and physiotherapy clinics between May 2019–September 2022; recruitment was paused from March 2020–June 2021 due to COVID-19 restrictions. Inclusion criteria were: (1) adults aged 18–65 years; (2) diagnosis of mTBI (≥ 3 months to < 5 years post-injury) based on ACRM criteria (39); (3) diagnosis of PPCS based on ICD-10 criteria (40); (4) exercise intolerance (acute exacerbation of PPCS on the Buffalo Concussion Treadmill Test [BCTT] (41)) as the BCTT was used to guide exercise prescription; (5) pharmacologically stable (no change to medications, including dose, for >1 month). Exclusion criteria included: (1) neurologic diagnosis, including moderate-to-severe TBI (other than mTBI/PPCS); (2) psychiatric diagnosis (other than depression, anxiety and/or PTSD); (3) cardiopulmonary disorder (including persistent symptoms following COVID-19); (4) chronic musculoskeletal condition limiting engagement in exercise; (5) pregnancy; (6) active cancer; or (7) enrollment in another research study. Participants were cleared for physical activity by the study physician based on the Physical Activity Questionnaire for Everyone (PAR-Q+) (42).
For the parent study, participants were randomized to either the immediate-start AEP (i-AEP) or delayed-start AEP (d-AEP). The d-AEP group completed a 6-week stretching intervention prior to starting the 12-weeks of sub-symptom threshold aerobic exercise. Interested participants completed a phone interview to determine if they met inclusion/exclusion criteria. Following screening, if eligible and still interested in the study, participants were consented, then randomized. A computer-generated randomization sequence with block sizes of 10 was used with randomization following a 1:1 ratio. Group allocation details were contained on cards placed inside sequentially numbered, sealed, opaque envelopes. Group allocation was revealed to participants following consenting. Neither research staff/assessors nor participants were blinded to the intervention.
Participants completed 12-weeks of individual, sub-symptom threshold aerobic exercise at a personalized target HR. Exercise was prescribed for 5x/week for 30 min at a HR of 70–80% of the max HR achieved on the BCTT (a prescriptive treadmill test, described below) (41). A Polar H10 HR monitor (Polar Electro Oy, Kempele, Finland) was worn for the duration of activity sessions to monitor HR. The monitor had Bluetooth connectivity to a mobile application for ease of use and HR monitoring during activity. Mode and location of exercise were at the discretion of participants based on preference and access; the study team discussed potential options with participants. Exercise prescriptions were updated every 3-weeks with a repeat BCTT performed by a trained exercise physiologist and graduate student.
Participants randomized to the d-AEP group completed 6-weeks of stretching prior to initiation of the 12-weeks of aerobic exercise. The stretching protocol has previously been described (43). Briefly, this was a 6-week, low intensity, stretching intervention performed 30 min, 5x/week at a HR not exceeding 50% of age-predicted max HR. Results of the stretching intervention are reported elsewhere (43). Following the 6-weeks of stretching, participants went on to complete 12-weeks of aerobic exercise. They followed the same aerobic exercise intervention as the i-AEP group.
All outcomes were completed at baseline and every 6-weeks thereafter. The BCTT was additionally completed every 3-weeks to update the exercise prescription and monitor exercise tolerance. Participants self-reported demographic information and injury characteristics following enrollment and randomization.
The primary outcome was PPCS symptom burden on the Rivermead Post Concussion Symptoms Questionnaire (RPQ) [0–64 points] (44).
Secondary self-reported outcomes included questionnaires evaluating QoL and specific post-concussive symptoms. These included:
• QoL, measured using the Quality of Life After Brain Injury questionnaire (QOLIBRI) [0–100 points] (45, 46).
• Depressive symptoms, measured using the Patient Health Questionnaire (PHQ-9) [0–27 points] (47).
• Anxiety symptoms, measured using the Generalized Anxiety Disorder Questionnaire (GAD-7) [0–21 points] (48).
• Functional impact of headache, measured using the Headache Impact Test (HIT-6) [36–78 points] (49).
• Fatigue, measured using the Fatigue Severity Scale (FSS) [9–63 points] (50).
• Daytime sleepiness, measured using the Epworth Sleepiness Scale (ESS) [0–24 points] (51).
• Dizziness, measured using the Dizziness Handicap Index (DHI) [0–100 points] (52).
A digital daily exercise diary was completed by participants [previously described (43)]. This allowed for monitoring of adherence and participant symptom response to intervention, ensuring no significant worsening of symptoms during exercise. Any participant concerns could be noted in the diary and were followed-up by the research team.
Participants completed sleep monitoring for 3–6 days and nights prior to starting the exercise protocol (for the d-AEP group this was after the 6-weeks of stretching), following 6-weeks of aerobic exercise, and after 12-weeks of aerobic exercise. Participants wore a MotionWatch8 tri-axial accelerometer (CamNTech, Texas, United States) on their non-dominant wrist during this time. To supplement objective sleep actigraphy monitoring, participants completed a sleep diary every morning upon waking (53). The sleep diary was adapted from the Consensus Sleep Diary (54) and included the following questions: (a) What time did you go to bed last night?; (b) After getting into bed, how long did it take you to fall asleep?; (c) After falling asleep, how many minutes were you awake for during the night?; (d) What time did you wake up in the morning?; (e) What time did you get out of bed?; (f) How many hours did you spend in bed last night (from getting in at night, to getting out in the morning)?; (g) Did you take a nap yesterday; (h) How was the quality of your sleep last night? (1 = very poor; 5 = very good); and (i) Did you take a sleep aid or any medication to help you sleep? Sleep outcomes included sleep duration, sleep onset latency and sleep efficiency. Sleep efficiency is the total time spent asleep as a percentage of time spent in bed. Participants who had travelled >1 time zone in the past 2 months or completed a night shift in the past year did not complete sleep outcomes.
Exercise tolerance (worsening of post-concussive symptoms with exertion) was assessed using the BCTT (41). The BCTT has previously been described and validated (41), but briefly, it is a progressive treadmill test where post-concussive symptom burden is rated on a 0–10 Likert scale at 1-min intervals as treadmill incline increases. HR was monitored using electrocardiogram (ECG) (CardioSoft 6.7 ECG software, GE Medical Systems, Milwaukee WI, United States) or Polar H10 HR monitor during testing. The test is terminated once the participant has a ≥ 3-point increase in symptoms. Maximum HR achieved at point of symptom exacerbation is noted. The BCTT was used to prescribe the exercise intervention as described.
Participants completed measures of autonomic function, including BP, HR and HRV at baseline and following 6-weeks and 12-weeks of intervention. All physiologic monitoring was completed under the supervision of a certified exercise physiologist.
A resting period preceded start of physiologic monitoring to establish baseline physiology. Four minutes of resting, quiet, seated HR and BP data, then 4-min of standing data were collected. Data recordings of ≥4 min have been validated for evaluating short-term HRV and shown to sufficiency approximate longer recordings (55). Between seated and standing measures, a ≥ 1 min adaptation period was included to establish resting physiology in the new posture. To ensure quiet, resting data, participants and research staff refrained from talking during testing. To address potential confounders, participants refrained from alcohol (56), caffeine (57), and vigorous exercise (58) for >6 h prior to the assessment; these have all been shown to influence HRV (59).
HR data were collected at 1000 Hz using a 12-lead ECG system, amplifier and acquisition software were used (PowerLab 16/35 amplifier, LabChart8 software, ADInstruments, Colorado Springs, CO, United States) (60). ECG was integrated with Vmax Encore 29 Metabolic Cart (Vyaire Medical, Mettawa, IL, USA). Two manual brachial BPs were taken by an exercise physiologist in both the seated and standing postures. Brachial BPs were averaged in each posture; SBP and DBP are reported.
Using ECG lead 2 from the Vmax system, PowerLab converted signal from analog-to-digital, exporting the raw ECG waveform at 1000 Hz (60). PowerLab was interfaced with LabChart to collect ECG output. ECG data was exported as a text file and imported into Ensemble-R software (V1.0.43, Ensemble, Elucimed, Wellington, NZ). ECG traces were visually inspected to identify any artifact or ectopic beats. Artifacts were replaced with interpolated RR data points using an automatic correction. Recordings with multiple ectopic beats were excluded. No pre-sets or filters were applied. Once data were normalized/cleaned, NN intervals were used to compute HRV parameters using Ensemble-R software. Time domain (SDNN, RMSSD, pNN50) and frequency domain (LF norm, HF norm, LF/HF ratio) HRV metrics are reported (34).
Baseline sample characteristics were reported using descriptive statistics. Chi-square test, independent samples t-test or Mann–Whitney U test were used to compare i-AEP and d-AEP groups where appropriate. Sleep actigraphy data were analyzed using MotionWare1.3.17 software, which was supplemented by the sleep diary data following previously published methods to calculate the reported sleep outcomes (sleep duration, sleep onset latency, sleep efficiency) (53).
I-AEP (n = 27) and d-AEP (n = 23) groups were combined to form the combined-AEP (c-AEP; n = 50) group. The c-AEP group allowed for evaluation of all participants having completed the 12-week intervention.
Intention-to-treat analysis was conducted using a two-level random intercept linear regression clustering around the individual (61) to examine change in outcomes following 12-weeks of intervention for the i-AEP, d-AEP and c-AEP groups. Change in outcomes following 12-weeks of i-AEP relative to d-AEP was also examined. For each model, 95% confidence intervals were constructed using bootstrapping methods (with 1,000 repetitions) at both levels of the random intercept model helping to account for small sample size, but also minimizing the effects on inferences that potential extreme values may have had. Results with a p-value of <0.05 were considered statistically significant with all models adjusted for age, sex, number of previous mTBIs, months since injury, and fit with an interaction term of randomization group by time (discrete) (61). All analyses were conducted using Stata 18 (62). Mean changes with 95% confidence intervals are reported.
A total of 210 individuals were screened for the parent study. Of those, 52 individuals met inclusion criteria and agreed to participate in the parent trial. On initial randomization, 27 participants were randomized to the i-AEP and 25 participants were randomized to begin with stretching prior to d-AEP. Two participants dropped out during the stretching portion of the intervention and thus 23 participants began the d-AEP. A total of 50 participants formed the c-AEP group (n = 27 i-AEP; n = 23 d-AEP) (see Figure 1).
Over the course of the 12-weeks of exercise, a total of 6 participants dropped out. Dropouts were due to medication change (n = 3), loss to follow-up (n = 2) or felt intervention not helpful (n = 1).
Participants were a mean (SD) of 42.6 (10.9) years old. It had been a mean (SD) of 25.1 (14.1) months since their most recent mTBI. Demographic characteristics including age, sex, educational status, work status and involvement in injury-related litigation did not significantly differ between the two groups. The most common mechanism of injury was motor vehicle collision (42%). See Tables 1, 2.
Groups did not significantly differ in symptom burden nor specific symptom outcomes pre-intervention with the exception of functional impact of headache. HIT-6 scores were significantly higher (p = 0.026) in i-AEP compared to d-AEP pre-intervention (see Table 1).
Following 12-weeks of intervention, overall participants had a significant improvement in symptom burden on the RPQ (i-AEP: mean change = −9.415, p < 0.001; d-AEP: mean change = −3.478, p = 0.034; c-AEP: mean change = −6.446, p < 0.001). The i-AEP group had a significantly greater improvement compared to the d-AEP group on the RPQ. When looking at all participants (c-AEP), the improvement on the RPQ exceeded the minimum clinically important difference of 4.6 points (63).
Participants also had significant improvement in QoL on the QOLIBRI (i-AEP: mean change = 9.879, p < 0.001; d-AEP: mean change = 7.994, p < 0.001, c-AEP: mean change = 8.937, p < 0.001). Improvement in QoL did not significantly differ between the two groups (i-AEP, d-AEP).
Following intervention there was improvement in specific post-concussive symptoms, including dizziness (i-AEP: mean change = −11.159, p = 0.001; d-AEP: mean change = −6.516, p = 0.019; c-AEP: −8.837, p < 0.001) and exercise tolerance (i-AEP: mean change = 5.987, p < 0.001; d-AEP: mean change = 3.421, p < 0.001; c-AEP: mean change = 4.703, p < 0.001). Headache (mean change = −5.522, p < 0.001), depression (mean change = −3.032, p = 0.001) and fatigue (mean change = −9.047, p < 0.001) improved in the i-AEP group.
There was no significant change in sleep outcomes (sleep duration, sleep onset latency, sleep efficiency) for either group across 12-weeks of intervention. Results are reported in Table 3 and Figure 2.
Figure 2. Clinical outcomes. Conditions are i-AEP (immediate-start aerobic exercise protocol; n = 27), d-AEP (delayed-start aerobic exercise protocol; n = 23) and c-AEP (combined aerobic exercise protocol; n = 50). Mean change from pre-treatment to 12-weeks of intervention from linear mixed-effect model for (A) symptom burden (Rivermead Post Concussion Symptoms Questionnaire, RPQ [0–64]); (B) quality of life (Quality of Life After Brain Injury Questionnaire, QOLIBRI [0–100]); (C) depressive symptoms (Patient Health Questionnaire-9, PHQ-9 [0–27]); (D) anxiety symptoms (Generalized Anxiety Disorder Scale, GAD-7 [0–21]); (E) functional impact of headache (Headache Impact Test, HIT-6 [36-78]); (F) fatigue (Fatigue Severity Scale, FSS [9-63]); (G) daytime sleepiness (Epworth Sleepiness Scale, ESS [0–24]); (H) dizziness (Dizziness Handicap Index, DHI [0–100]); (I) exercise intolerance (Buffalo Concussion Treadmill Test, BCTT).
There were no significant changes in seated or standing HR, BP or HRV metrics following 12-weeks of intervention in either the i-AEP, d-AEP or c-AEP group. Results did not significantly differ between i-AEP and d-AEP. Results of c-AEP group are reported in Table 4.
There were no reported adverse events related to the intervention itself that occurred during either completion of i-AEP, d-AEP nor during exercise testing.
This prospective cohort study evaluating a 12-week sub-symptom threshold aerobic exercise intervention for adults with PPCS is an important first step in providing data to support the prescription of aerobic exercise for this specific patient population. While aerobic exercise is increasingly being recognized for its important role in rehabilitation following mTBI in adolescent and athletic populations, studies evaluating the impact of exercise for adults with persisting symptoms following injury are lacking (27, 64). Here, we demonstrated that among participants who completed a 12-week exercise intervention (c-AEP; n = 50), there was a significant improvement in overall symptom burden, QoL and specific symptom domains (depressive symptoms, headache, dizziness, exercise tolerance). Individuals who completed a precursory 6-week stretching program (d-AEP group) did not have a greater response to exercise compared to those who engaged in the exercise immediately following enrollment (i-AEP group). This trial suggests that adults presenting with months-to-years of symptoms following mTBI can both tolerate and benefit from a graded, personalized aerobic exercise intervention. While altered autonomic function has been recognized in cohorts with mTBI and PPCS (32, 65), the described intervention did not change resting HR, BP nor measures of HRV. While further study of the mechanisms by which exercise may improve post-concussive symptoms and exercise tolerance is needed, this work provides preliminary data to support prescription of personalized HR-targeted aerobic exercise for adults with PPCS.
Landmark trials evaluating exercise prescription following adolescent sport-related concussion found that initiation of a sub-symptom threshold aerobic exercise program within 10 days of injury could speed recovery and lead to a reduced incidence of PPCS compared to stretching (25, 26). Similarly, an exploratory RCT in an adolescent cohort with 4–16 weeks of PPCS also showed greater improvement in symptom burden in those who completed a 7-week aerobic exercise program compared to stretching (66). Several other studies, in both adults and adolescents, have investigated rehabilitation programs with an exercise component and shown benefit, but where evaluation of a structured exercise intervention was not the primary aim (64). In this study we demonstrated that adults with months-to-years of PPCS (c-AEP; n = 50) who completed 12-weeks of tailored, sub-symptom threshold aerobic exercise had a significant improvement in post-concussive symptoms as measured on the RPQ. This improvement (c-AEP group; mean change = −6.446) exceeded the suggested minimal clinically important difference. The RPQ measure of symptom burden is composed of 16-items representing the most common post-concussive symptoms and therefore is a clinically relevant measure of symptom improvement over time. Further, specific symptom questionnaires were useful in identifying symptoms that were most responsive to the intervention. When looking at all participants (c-AEP), there was significant improvement in depressive symptoms, functional impact of headache, fatigue and dizziness over time. Aerobic exercise has been well documented to improve many of these same clinical symptoms in non-head injured populations, specifically depression (21) and headache (23, 24); this trial suggests similar benefits for individuals with PPCS.
On the most comprehensive self-reported outcome, QoL measured using the QOLIBRI questionnaire, both groups (d-AEP; i-AEP) had a significant improvement in their scores across the intervention. Further, the d-AEP and i-AEP had a similar improvement in QoL over time, suggesting that initiation of a stretching program prior to exercise does not confer additional exercise benefit. While no minimal clinically important difference is available for the QOLIBRI, the 8.9 point improvement (out of 100 points) for the c-AEP group, combined with improvements to exercise tolerance and multiple other symptoms suggests QoL improvement was not only statistically, but also functionally significant. A significant improvement on the QOLIBRI was also found following an 8-week walking intervention in adults with PPCS (29). Improvements in QoL have also been reported in a cohort with all severity TBI, where those who reported exercising 90 min/week had higher QoL compared to those who did not in a secondary analysis (67). The mechanism by which exercise improves QoL in this patient population was beyond the scope of this study; however, increased self-efficacy, a return to an important pre-morbid activity (30% of participants were engaged in sports at the time of injury) and increased socialization (many participants reported completing the activity with a partner or friend) may all have contributed.
I-AEP and d-AEP groups both had improvements in symptom burden and measures of specific post-concussive symptoms. On some outcomes, including symptom burden, functional impact of headache, fatigue, exercise tolerance and sleep efficiency, the i-AEP group had a significantly greater improvement compared d-AEP. Given the groups were relatively well matched it is unclear the exact reason for these differences in response; however, we propose several factors contributed. In terms of symptom burden, the significantly greater improvement in the i-AEP group may have been in part driven by the d-AEP group’s completion of the precursory stretching program. While the stretching lead to a non-significant improvement in symptom burden (43), it is possible that this initial improvement in symptoms resulted from the additional weeks of interaction that the d-AEP group had with the study team ahead of starting the 12-week aerobic protocol and having already been primed to the study protocol. By randomizing to an arm with 6 weeks of stretching first (d-AEP) we were able to evaluate the influence of the placebo effect on our clinical outcomes, an important consideration in any randomized clinical trial. Difference in functional outcome of headache response (significantly greater improvement in the i-AEP vs. d-AEP) may have been in part due to between group differences at baseline, where the i-AEP group had higher headache scores compared to d-AEP. Differences in sleep efficiency response between groups was driven by the d-AEP group, which decreased by 1.6%, whereas the i-AEP group had a marginal improvement in sleep efficiency of 0.8%. There is no minimal clinical importance difference threshold for sleep efficiency based on actigraphy in PPCS to our knowledge. However, studies showing significant improvement in sleep efficiency following cognitive behavioural therapy for insomnia (gold standard intervention) in adults with cardiovascular disease showed a mean improvement in sleep efficiency (measured using actigraphy) of 0.5–16%, suggesting that the marginal changes to sleep efficiency in this study are likely not of clinical significance (68–71).
Aerobic exercise has been suggested as a treatment modality to improve autonomic function following mTBI (72). Following 12-weeks of intervention we found no significant change in HR, HRV or BP in either the seated or standing posture. Several factors likely contributed to this null result, including intervention intensity, duration and participant age. At the start of the exercise intervention, participants presented with a high degree of exercise intolerance, meaning they were able to complete relatively few stages on the BCTT prior to symptom worsening. Given this, exercise prescriptions were of relatively low intensity at initiation of intervention. As participants progressed through the study, exercise prescriptions were updated in accordance with results on the BCTT. However, many participants still did not reach their age-predicted max HR on treadmill testing without becoming symptomatic at the final assessment. This suggests the intervention may not have been of sufficient duration to fully return individuals to age-predicted level of exercise. Several previous studies have shown that physical activity volume and intensity are associated with HRV metrics (37, 73, 74). In a community-based study of 985 older adults Soares-Miranda et al. demonstrated that higher total leisure-time activity, walking distance and walking pace were all positively associated with 24-h SDNN (73). These results were built upon by de Sousa et al. who evaluated association between resting HRV measures and amount of very vigorous physical activity measured via hip accelerometry (75). All HRV variables (including SDNN and RMSSD) demonstrated a linear trend through the quintiles of very vigorous physical activity; those who completed a greater duration of very vigorous physical activity had higher time-domain HRV metrics (75). Age also likely plays a role in HRV response to physical activity. HRV data estimated by photoplethysmography has suggested a greater volume of activity is required to see HRV changes with increasing age, which could be another contributing factor in this study (37). Using photoplethysmography data Natarajan et al. suggested that individuals aged 20–24 years could increase their HF power by 1ms2 with an additional ~30 steps/day, but that 200–300 steps/day would be needed to see the same improvement in those aged 50–54 years (37). Taken together, these results suggest that a longer intervention with greater time to build exercise tolerance, and thus allow for engagement in more vigorous exercise, is likely required to observe improvement in HRV parameters in adults with PPCS. Future work may consider inclusion of additional parameters of autonomic function to further understand physiologic response to exercise interventions in adults with PPCS.
There were limitations to this study, notably the lack of a control group for the duration of the 12-weeks of exercise. While significant improvement was observed over the course of the intervention, a longer intervention would likely have provided additional insights into symptom change over time with exercise. A longer follow-up period would have also allowed more time for participants to build up their exercise tolerance and thus engage in more moderate-to-vigorous prescribed exercise. This prospective cohort study was conducted at a single center and recruited from specialized brain injury and pain clinics; therefore, the results are generalizable to individuals with PPCS presenting to specialized care. Multi-centered trials across multiple sites with broader recruitment are needed to fully understand generalizability of such an intervention for adults with PPCS. A greater proportion of females (74%) compared to males (26%) were enrolled in the study. This may have been in part due to the fact that more females than males go on to experience persisting symptoms following mTBI, or that women are more likely to seek care (76, 77). Regardless, this too has implications for generalizability. In terms of autonomic testing, resting HR, BP and HRV measures were completed. In future, additional measures of autonomic function (including assessments such as facial cooling, valsalva maneuver or baroreceptor sensitivy) might reveal more subtle alterations in autonomic function that were not appreciated in this study.
Previous reviews of treatments for PPCS have provided the guidance to “consider offering graded physical exercise in addition to other treatment” despite a lack of evidence for how this exercise should be prescribed, its benefits and tolerability (15). Here, we present an exercise intervention with symptom and QoL benefits that could be incorporated into the multi-disciplinary care of adults with PPCS. Given the exercise intervention was well tolerated, without adverse events, it presents a low-cost, non-pharmacologic, personalized intervention that could be prescribed without fear of exacerbating symptoms so long as adequate monitoring is in place. Participants largely found the HR monitoring using an HR monitor to be helpful for compliance during independent exercise sessions. Currently, there are few clinical settings where this type of exercise testing (BCTT) and subsequent exercise prescription is available for patients with PPCS. Other groups have published methods for prescribing HR-targeted exercise based on age-predicted HR maximum, which may be considered when formal exercise testing is not available (78, 79). Additionally, more work is needed in this area to better understand which patients may preferentially respond to this type of intervention, the optimal duration of intervention, and adherence from participants upon study termination (long follow-up timelines). Given the intervention was well tolerated, in select patient we suggest prescription of activity at 80–90% of max HR achieved on the BCTT could be trialed (instead of the 70–80% used in this study).
To date, few studies have evaluated the prescription of sub-symptom threshold aerobic exercise in adult PPCS cohorts and thus limited data are available to treating clinicians in terms of prescribing such an intervention. We found that following 12-weeks of sub-symptom threshold aerobic exercise, participants (c-AEP; n = 50) had significant improvement in symptom burden, QoL and specific symptom domains. Further, there was improvement in exercise tolerance enabling engagement in more vigorous exercise over time. This provides preliminary data to support prescription of individualized, sub-symptom threshold aerobic exercise for adults with PPCS following a graduated approach of increasing intensity based on exercise tolerance.
The datasets presented in this article are not readily available because a formal data sharing agreement would have to be initiated by any researchers requesting this data set. Requests to access the datasets should be directed to Leah Mercier, bGVhaC5tZXJjaWVyQHVjYWxnYXJ5LmNh.
This study was approved by the University of Calgary Conjoint Research Ethics Board (REB18-1329) and was conducted in accordance with the local legislation and institutional requirements. The participants provided their written informed consent to participate in this study.
LM: Conceptualization, Data curation, Formal analysis, Investigation, Methodology, Project administration, Writing – original draft, Writing – review & editing. SM: Data curation, Investigation, Project administration, Writing – review & editing. CB: Data curation, Investigation, Writing – review & editing. JJ: Data curation, Investigation, Project administration, Writing – review & editing. JB: Data curation, Investigation, Project administration, Writing – review & editing. J-MG: Formal analysis, Writing – review & editing. JSB: Methodology, Writing – review & editing. JS: Methodology, Software, Writing – review & editing. ME: Methodology, Writing – review & editing. KS: Methodology, Writing – review & editing. SD: Methodology, Supervision, Writing – review & editing. AH: Conceptualization, Funding acquisition, Methodology, Writing – review & editing. CD: Conceptualization, Funding acquisition, Methodology, Supervision, Writing – review & editing.
The author(s) declare that financial support was received for the research, authorship, and/or publication of this article. This trial was supported by a New Frontiers in Research Fund Exploration Grant, Foundations of Physical Medicine and Rehabilitation Mid-Career Grant and a Hotchkiss Brain Institute PFUND Award. Additional support was provided by the Hotchkiss Brain Institute. L. J. Mercier was supported by a Graduate Studentship in Patient-Oriented Research from the Alberta SPOR Support Unit (jointly funded by Alberta Innovates-Health Solutions and the Canadian Institute of Health Research), a Dr. Matthew Galati Brain Changer Award from Brain Canada, a Graduate Studentship from the Integrated Concussion Research Program and University of Calgary Scholarships. The Dr. Matthew Galati Brain Changer Award has been made possible by the Canada Brain Research Fund (CBRF), an innovative arrangement between the Government of Canada (through Health Canada) and Brain Canada Foundation, and by the Brain Changes Initiative. S. J. McIntosh was supported by an O’Brien Centre Summer Studentship Award and University of Calgary Scholarships. C. Boucher was supported by an Alberta Innovates Summer Research Studentship. A. D. Harris holds a Canada Research Chair in Magnetic Resonance Spectroscopy in Brain Injury.
We would like to acknowledge the ACTBI team, including Mehak Stokoe, Amanda Ip, Kayla Millar, Dr. Tiffany Bell and Emmy Munro. We thank the CTEP (Clinical & Translational Exercise Physiology Laboratory) team, including Dr. Karen Kendall, Courtney Ellis, Connor Snow, Jordan Bentley-Swanson and Michaela Chadder, for their help with exercise testing data collection.
The authors declare that the research was conducted in the absence of any commercial or financial relationships that could be construed as a potential conflict of interest.
All claims expressed in this article are solely those of the authors and do not necessarily represent those of their affiliated organizations, or those of the publisher, the editors and the reviewers. Any product that may be evaluated in this article, or claim that may be made by its manufacturer, is not guaranteed or endorsed by the publisher.
ACRM, American Congress of Rehabilitation Medicine; ACTBI Trial, Aerobic Exercise for treatment of Chronic symptoms following mild Traumatic Brain Injury Trial; BCTT, Buffalo Concussion Treadmill Test; BP, blood pressure; c-AEP, combined aerobic exercise protocol; d-AEP, delayed-start aerobic exercise protocol; DHI, Dizziness Handicap Index; ECG, electrocardiogram; ESS, Epworth Sleepiness Scale; FSS, Fatigue Severity Scale; GAD-7, Generalized Anxiety Disorder Questionnaire; HF norm, high frequency power in normalized units; HIT-6, Headache Impact Test; HR, heart rate; HRV, heart rate variability; i-AEP, immediate-start aerobic exercise protocol; ICD-10, International Classification of Diseases, Tenth Revision; LF/HF ratio, low frequency to high frequency ratio; LF norm, low frequency power in normalized units; mTBI, mild traumatic brain injury; PAR-Q+, Physical Activity Questionnaire for Everyone; PHQ-9, Patient Health Questionnaire; pNN50, percent of RR intervals differing by more than 50 ms; PPCS, persisting post-concussive symptoms; RMSSD, root mean square of successive RR interval differences; RPQ, Rivermead Post Concussion Symptoms Questionnaire; SDNN, standard deviation of RR intervals; QoL, quality of life; QOLIBRI, Quality of Life After Brain Injury Questionnaire.
1. Patricios, JS, Schneider, KJ, Dvorak, J, Ahmed, OH, Blauwet, C, Cantu, RC, et al. Consensus statement on concussion in sport: the 6th international conference on concussion in sport-Amsterdam, October 2022. Br J Sports Med. (2023) 57:695–711. doi: 10.1136/bjsports-2023-106898
2. Yeates, KO, Räisänen, AM, Premji, Z, Debert, CT, Frémont, P, Hinds, S, et al. What tests and measures accurately diagnose persisting post-concussive symptoms in children, adolescents and adults following sport-related concussion? A systematic review. Br J Sports Med. (2023) 57:780–8. doi: 10.1136/bjsports-2022-106657
3. Cancelliere, C, Verville, L, Stubbs, JL, Yu, H, Hincapié, CA, Cassidy, JD, et al. Post-concussion symptoms and disability in adults with mild traumatic brain injury: a systematic review and meta-analysis. J Neurotrauma. (2023) 40:1045–59. doi: 10.1089/neu.2022.0185
4. Dwyer, B, and Katz, DI. Postconcussion syndrome. Handb Clin Neurol. (2018) 158:163–78. doi: 10.1016/B978-0-444-63954-7.00017-3
5. Ellis, MJ, Leddy, JJ, and Willer, B. Physiological, vestibulo-ocular and cervicogenic post-concussion disorders: an evidence-based classification system with directions for treatment. Brain Inj. (2015) 29:238–48. doi: 10.3109/02699052.2014.965207
6. Antonellis, P, Campbell, KR, Wilhelm, JL, Shaw, JD, Chesnutt, JC, and King, LA. Exercise intolerance after mild traumatic brain injury occurs in all subtypes in the adult population. J Neurotrauma. (2024) 41:635–45. doi: 10.1089/neu.2023.0168
7. DeGroot, A, Huber, DL, Leddy, JJ, Raff, H, McCrea, MA, Johnson, BD, et al. Use of the Buffalo concussion treadmill test in community adult patients with mild traumatic brain injury. PM R. (2024) 16:826–35. doi: 10.1002/pmrj.13132
8. Thorne, J, Hellewell, SC, Cowen, G, Ring, A, Jefferson, A, Chih, H, et al. Symptoms associated with exercise intolerance and resting heart rate following mild traumatic brain injury. J Head Trauma Rehabil. (2024) 39:E381–92. doi: 10.1097/HTR.0000000000000928
9. Mikolic, A, Klotz, T, Brasher, P, Yeates, K, Vranceanu, AM, Kendall, KD, et al. Canadian traumatic brain injury research consortium (CTRC). Graded exposure therapy for fear avoidance behaviour after concussion (GET FAB): protocol for a multisite Canadian randomised controlled trial. BMJ Open. (2024) 14:e086602. doi: 10.1136/bmjopen-2024-086602
10. Suzuki, S, Mattson, CL, Obermeier, MC, Casanova, AD, Doda, AK, Sayles, LA, et al. Athletic fear avoidance in athletes receiving rehabilitation for sport-related concussion: a preliminary study. Sports Health. (2024) 16:457–64. doi: 10.1177/19417381231172513
11. Smulligan, KL, Wingerson, MJ, Seehusen, CN, Little, CC, Wilson, JC, and Howell, DR. More physical activity is correlated with reduction in kinesiophobia for adolescents with persistent symptoms after concussion. J Sport Rehabil. (2022) 32:196–202. doi: 10.1123/jsr.2022-0193
12. Mercier, LJ, Kowalski, K, Fung, TS, Joyce, JM, Yeates, KO, and Debert, CT. Characterizing physical activity and sedentary behavior in adults with persistent postconcussive symptoms after mild traumatic brain injury. Arch Phys Med Rehabil. (2021) 102:1918–25.e1. doi: 10.1016/j.apmr.2021.05.002
13. Ashina, H, Eigenbrodt, AK, Seifert, T, Sinclair, AJ, Scher, AI, Schytz, HW, et al. Post-traumatic headache attributed to traumatic brain injury: classification, clinical characteristics, and treatment. Lancet Neurol. (2021) 20:460–9. doi: 10.1016/S1474-4422(21)00094-6
14. Minen, MT, Mahmood, N, Khan, F, Waire, EK, George, A, and Datta, S. Treatment options for posttraumatic headache: a current review of the literature. Curr Pain Headache Rep. (2024) 28:205–10. doi: 10.1007/s11916-023-01199-y
15. Rytter, HM, Graff, HJ, Henriksen, HK, Aaen, N, Hartvigsen, J, Hoegh, M, et al. Nonpharmacological treatment of persistent postconcussion symptoms in adults: a systematic review and meta-analysis and guideline recommendation. JAMA Netw Open. (2021) 4:e2132221. doi: 10.1001/jamanetworkopen.2021.32221
16. Schneider, KJ, Critchley, ML, Anderson, V, Davis, GA, Debert, CT, Feddermann-Demont, N, et al. Targeted interventions and their effect on recovery in children, adolescents and adults who have sustained a sport-related concussion: a systematic review. Br J Sports Med. (2023) 57:771–9. doi: 10.1136/bjsports-2022-106685
17. Amiri, S, Hasani, J, and Satkin, M. Effect of exercise training on improving sleep disturbances: a systematic review and meta-analysis of randomized control trials. Sleep Med. (2021) 84:205–18. doi: 10.1016/j.sleep.2021.05.013
18. Kovacevic, A, Mavros, Y, Heisz, JJ, and Fiatarone Singh, MA. The effect of resistance exercise on sleep: a systematic review of randomized controlled trials. Sleep Med Rev. (2018) 39:52–68. doi: 10.1016/j.smrv.2017.07.002
19. De Nys, L, Anderson, K, Ofosu, EF, Ryde, GC, Connelly, J, and Whittaker, AC. The effects of physical activity on cortisol and sleep: a systematic review and meta-analysis. Psychoneuroendocrinology. (2022) 143:105843. doi: 10.1016/j.psyneuen.2022.105843
20. Pearce, M, Garcia, L, Abbas, A, Strain, T, Schuch, FB, Golubic, R, et al. Association between physical activity and risk of depression: a systematic review and meta-analysis. JAMA Psychiatry. (2022) 79:550–9. doi: 10.1001/jamapsychiatry.2022.0609
21. Noetel, M, Sanders, T, Gallardo-Gómez, D, Taylor, P, Del Pozo, CB, van den Hoek, D, et al. Effect of exercise for depression: systematic review and network meta-analysis of randomised controlled trials. BMJ. (2024) 384:e075847. doi: 10.1136/bmj-2023-075847
22. Aylett, E, Small, N, and Bower, P. Exercise in the treatment of clinical anxiety in general practice - a systematic review and meta-analysis. BMC Health Serv Res. (2018) 18:559. doi: 10.1186/s12913-018-3313-5
23. Machado-Oliveira, L, da Silva Gauto, YO, de Santana Neto, FJ, da Silva, MG, Germano-Soares, AH, and Diniz, PRB. Effects of different exercise intensities on headache: a systematic review. Am J Phys Med Rehabil. (2020) 99:390–6. doi: 10.1097/PHM.0000000000001349
24. Lemmens, J, De Pauw, J, Van Soom, T, Michiels, S, Versijpt, J, van Breda, E, et al. The effect of aerobic exercise on the number of migraine days, duration and pain intensity in migraine: a systematic literature review and meta-analysis. J Headache Pain. (2019) 20:16. doi: 10.1186/s10194-019-0961-8
25. Leddy, JJ, Haider, MN, Ellis, MJ, Mannix, R, Darling, SR, Freitas, MS, et al. Early subthreshold aerobic exercise for sport-related concussion: a randomized clinical trial. JAMA Pediatr. (2019) 173:319–25. doi: 10.1001/jamapediatrics.2018.4397
26. Leddy, JJ, Master, CL, Mannix, R, Wiebe, DJ, Grady, MF, Meehan, WP, et al. Early targeted heart rate aerobic exercise versus placebo stretching for sport-related concussion in adolescents: a randomised controlled trial. Lancet Child Adolesc Health. (2021) 5:792–9. doi: 10.1016/S2352-4642(21)00267-4
27. Leddy, JJ, Burma, JS, Toomey, CM, Hayden, A, Davis, GA, Babl, FE, et al. Rest and exercise early after sport-related concussion: a systematic review and meta-analysis. Br J Sports Med. (2023) 57:762–70. doi: 10.1136/bjsports-2022-106676
28. Haider, MN, Cole, WR, Willer, BS, McCulloch, K, Horn, EC, Bertz, PE, et al. Early targeted heart rate exercise is safe and may hasten return-to-duty in service members with acute concussion, a preliminary study. Brain Inj. (2024) 38:119–25. doi: 10.1080/02699052.2024.2306334
29. Alarie, C, Gagnon, I, de Guise, E, McKerral, M, Kersalé, M, van Het, B, et al. A remotely delivered progressive walking intervention for adults with persistent symptoms of a mild traumatic brain injury: feasibility and exploration of its impact. Front Rehabil Sci. (2022) 3:898804. doi: 10.3389/fresc.2022.898804
30. Ontario Neurotrauma Foundation. Guideline for concussion/Mild Traumatic Brain Injury & Persistent Symptoms. (2018)
31. Silverberg, ND, Iaccarino, MA, Panenka, WJ, Iverson, GL, McCulloch, KL, Dams-O'Connor, K, et al. American congress of rehabilitation medicine brain injury interdisciplinary special interest group mild TBI Task Force. Management of concussion and mild traumatic brain injury: a synthesis of practice guidelines. Arch Phys Med Rehabil. (2020) 101:382–93. doi: 10.1016/j.apmr.2019.10.179
32. Pelo, R, Suttman, E, Fino, PC, McFarland, MM, Dibble, LE, and Cortez, MM. Autonomic dysfunction and exercise intolerance in concussion: a scoping review. Clin Auton Res. (2023) 33:149–63. doi: 10.1007/s10286-023-00937-x
33. Clausen, M, Pendergast, DR, Willer, B, and Leddy, J. Cerebral blood flow during treadmill exercise is a marker of physiological postconcussion syndrome in female athletes. J Head Trauma Rehabil. (2016) 31:215–24. doi: 10.1097/HTR.0000000000000145
34. Shaffer, F, and Ginsberg, JP. An overview of heart rate variability metrics and norms. Front Public Health. (2017) 5:258. doi: 10.3389/fpubh.2017.00258
35. Hilz, MJ, DeFina, PA, Anders, S, Koehn, J, Lang, CJ, Pauli, E, et al. Frequency analysis unveils cardiac autonomic dysfunction after mild traumatic brain injury. J Neurotrauma. (2011) 28:1727–38. doi: 10.1089/neu.2010.1497
36. Rennie, KL, Hemingway, H, Kumari, M, Brunner, E, Malik, M, and Marmot, M. Effects of moderate and vigorous physical activity on heart rate variability in a British study of civil servants. Am J Epidemiol. (2003) 158:135–43. doi: 10.1093/aje/kwg120
37. Natarajan, A, Pantelopoulos, P, Emir-Farinas, H, and Natarajan, P. Heart rate variability with photoplethysmography in 8 million individuals: a cross-sectional study. Lancet Digit Health. (2020) 2:e650–7. doi: 10.1016/S2589-7500(20)30246-6
38. Mercier, LJ, Fung, TS, Harris, AD, Dukelow, SP, and Debert, CT. Improving symptom burden in adults with persistent post-concussive symptoms: a randomized aerobic exercise trial protocol. BMC Neurol. (2020) 20:46. doi: 10.1186/s12883-020-1622-x
39. Definition of mild traumatic. Definition of mild traumatic brain injury. J Head Trauma Rehabil. (1993) 8:86–7. doi: 10.1097/00001199-199309000-00010
40. World Health Organization. The ICD-10 classification of mental and Behavioural disorders: diagnostic criteria for research. Geneva: World Health Organization (1993).
41. Leddy, JJ, and WIller, B. Use of graded exercise testing in concussion and return-to-activity management. Curr Sports Med Rep. (2013) 12:370–6. doi: 10.1249/JSR.0000000000000008
42. Warburton, DER, Jamnik, VK, Bredin, SSD, and Glehill, N. The physical activity readiness questionnaire for everyone (PAR-Q+) and electronic physical activity readiness medical examination (ePARmed-X+). Health Fitness J Canada. (2011) 4:3–17. doi: 10.14288/hfjc.v4i2.103
43. Mercier, LJ, McIntosh, SJ, Boucher, C, Joyce, JM, Batycky, J, Galarneau, JM, et al. Effect of aerobic exercise on symptom burden and quality of life in adults with persistent post-concussive symptoms: the ACTBI randomized clinical trial. Arch Phys Med Rehabil. (2024). doi: 10.1016/j.apmr.2024.10.002
44. King, NS, Crawford, S, Wenden, FJ, Moss, NE, and Wade, DT. The Rivermead post concussion symptoms questionnaire: a measure of symptoms commonly experienced after head injury and its reliability. J Neurol. (1995) 242:587–92. doi: 10.1007/BF00868811
45. von Steinbüchel, N, Wilson, L, Gibbons, H, Hawthorne, G, Höfer, S, Schmidt, S, et al. QOLIBRI Task Force. Quality of life after brain injury (QOLIBRI): scale development and metric properties. J Neurotrauma. (2010) 27:1167–85. doi: 10.1089/neu.2009.1076
46. von Steinbüchel, N, Wilson, L, Gibbons, H, Hawthorne, G, Höfer, S, Schmidt, S, et al. Quality of life after brain injury (QOLIBRI): scale validity and correlates of quality of life. J Neurotrauma. (2010) 27:1157–65. doi: 10.1089/neu.2009.1077
47. Kroenke, K, Spitzer, RL, and Williams, JB. The PHQ-9: validity of a brief depression severity measure. J Gen Intern Med. (2001) 16:606–13. doi: 10.1046/j.1525-1497.2001.016009606.x
48. Spitzer, RL, Kroenke, K, Williams, JBW, and Löwe, B. A brief measure for assessing generalized anxiety disorder: the GAD-7. Arch Intern Med. (2006) 166:1092–7. doi: 10.1001/archinte.166.10.1092
49. Kosinski, M, Bayliss, MS, Bjorner, JB, Ware, JE, Garber, WH, Batenhorst, A, et al. A six-item short-form survey for measuring headache impact: the HIT-6. Qual Life Res. (2003) 12:963–74. doi: 10.1023/a:1026119331193
50. Krupp, LB, LaRocca, NG, Muir-Nash, J, and Steinberg, AD. The fatigue severity scale. Application to patients with multiple sclerosis and systemic lupus erythematosus. Arch Neurol. (1989) 46:1121–3. doi: 10.1001/archneur.1989.00520460115022
51. Johns, MW. A new method for measuring daytime sleepiness: the Epworth sleepiness scale. Sleep. (1991) 14:540–5. doi: 10.1093/sleep/14.6.540
52. Jacobson, GP, and Newman, CW. The development of the dizziness handicap inventory. Arch Otolaryngol Head Neck Surg. (1990) 116:424–7. doi: 10.1001/archotol.1990.01870040046011
53. McIntosh, SJ, Mercier, LJ, Boucher, C, Yip, R, Batycky, JM, Joyce, J, et al. Assessment of sleep parameters in adults with persistent post-concussive symptoms. Sleep Med. (2024) 119:406–16. doi: 10.1016/j.sleep.2024.05.030
54. Carney, CE, Buysse, DJ, Ancoli-Israel, S, Edinger, JD, Krystal, AD, Lichstein, KL, et al. The consensus sleep diary: standardizing prospective sleep-monitoring. Sleep. (2012) 35:287–302. doi: 10.5665/sleep.1642
55. Burma, JS, Graver, S, Miutz, LN, Macaulay, A, Copeland, PV, and Smirl, JD. The validity and reliability of ultra-short-term heart rate variability parameters and the influence of physiological covariates. J Appl Physiol. (2021) 130:1848–67. doi: 10.1152/japplphysiol.00955.2020
56. Brunner, S, Winter, R, Werzer, C, von Stülpnagel, L, Clasen, I, Hameder, A, et al. Impact of acute ethanol intake on cardiac autonomic regulation. Sci Rep. (2021) 11:13255. doi: 10.1038/s41598-021-92767-y
57. Grant, SS, Kim, K, and Friedman, BH. How long is long enough? Controlling for acute caffeine intake in cardiovascular research. Brain Sci. (2023) 13:224. doi: 10.3390/brainsci13020224
58. Burma, JS, Copeland, PV, Macaulay, A, Khatra, O, and Smirl, JD. Effects of high-intensity intervals and moderate-intensity exercise on baroreceptor sensitivity and heart rate variability during recovery. Appl Physiol Nutr Metab. (2020) 45:1156–64. doi: 10.1139/apnm-2019-0810
59. Ellingson, CJ, Singh, J, Ellingson, CA, Dech, R, Piskorski, J, and Neary, JP. The influence of external stressors on physiological testing: implication for return-to-play protocols. Curr Res Physiol. (2022) 5:240–5. doi: 10.1016/j.crphys.2022.06.003
60. Burma, JS, Lapointe, AP, Soroush, A, Oni, IK, Smirl, JD, and Dunn, JF. Insufficient sampling frequencies skew heart rate variability estimates: implications for extracting heart rate metrics from neuroimaging and physiological data. J Biomed Inform. (2021) 123:103934. doi: 10.1016/j.jbi.2021.103934
61. Rabe-Hesketh, S, and Skrondal, A. Multilevel and longitudinal modeling using Stata. 4th ed (2022).
63. Silverberg, ND, and Otamendi, T. Advice to rest for more than 2 days after mild traumatic brain injury is associated with delayed return to productivity: a case-control study. Front Neurol. (2019) 10:362. doi: 10.3389/fneur.2019.00362
64. McIntyre, M, Kempenaar, A, Amiri, M, Alavinia, SM, and Kumbhare, D. The role of subsymptom threshold aerobic exercise for persistent concussion symptoms in patients with postconcussion syndrome: a systematic review. Am J Phys Med Rehabil. (2020) 99:257–64. doi: 10.1097/PHM.0000000000001340
65. Mercier, LJ, Batycky, J, Campbell, C, Schneider, K, Smirl, J, and Debert, CT. Autonomic dysfunction in adults following mild traumatic brain injury: a systematic review. NeuroRehabilitation. (2022) 50:3–32. doi: 10.3233/NRE-210243
66. Kurowski, BG, Hugentobler, J, Quatman-Yates, C, Taylor, J, Gubanich, PJ, Altaye, M, et al. Aerobic exercise for adolescents with prolonged symptoms after mild traumatic brain injury: an exploratory randomized clinical trial. J Head Trauma Rehabil. (2017) 32:79–89. doi: 10.1097/HTR.0000000000000238
67. Hoffman, JM, Bell, KR, Powell, JM, Behr, J, Dunn, EC, Dikmen, S, et al. A randomized controlled trial of exercise to improve mood after traumatic brain injury. PM R. (2010) 2:911–9. doi: 10.1016/j.pmrj.2010.06.008
68. Abdelaziz, A, Hafez, AH, Roshdy, MR, Abdelaziz, M, Eltobgy, MA, Elsayed, H, et al. Cognitive behavioral therapy for the treatment of insomnia in patients with cardiovascular diseases: a meta-analysis with GRADE analysis. J Behav Med. (2024) 47:819–27. doi: 10.1007/s10865-024-00490-6
69. Harris, KM, Schiele, SE, and Emery, CF. Pilot randomized trial of brief behavioral treatment for insomnia in patients with heart failure. Heart Lung. (2019) 48:373–80. doi: 10.1016/j.hrtlng.2019.06.003
70. Redeker, NS, Jeon, S, Andrews, L, Cline, J, Jacoby, D, and Mohsenin, V. Feasibility and efficacy of a self-management intervention for insomnia in stable heart failure. J Clin Sleep Med. (2015) 11:1109–19. doi: 10.5664/jcsm.5082
71. Redeker, NS, Yaggi, HK, Jacoby, D, Hollenbeak, CS, Breazeale, S, Conley, S, et al. Cognitive behavioral therapy for insomnia has sustained effects on insomnia, fatigue, and function among people with chronic heart failure and insomnia: the HeartSleep study. Sleep. (2022) 45:252. doi: 10.1093/sleep/zsab252
72. Callaway, CCM, and Kosofsky, BE. Autonomic dysfunction following mild traumatic brain injury. Curr Opin Neurol. (2019) 32:802–7. doi: 10.1097/WCO.0000000000000751
73. Soares-Miranda, L, Sattelmair, J, Chaves, P, Duncan, GE, Siscovick, DS, Stein, PK, et al. Physical activity and heart rate variability in older adults: the cardiovascular health study. Circulation. (2014) 129:2100–10. doi: 10.1161/CIRCULATIONAHA.113.005361
74. May, R, McBerty, V, Zaky, A, and Gianotti, M. Vigorous physical activity predicts higher heart rate variability among younger adults. J Physiol Anthropol. (2017) 36:24. doi: 10.1186/s40101-017-0140-z
75. de Sousa, TLW, Ostoli, TLVP, Sperandio, EF, Arantes, RL, Gagliardi, ART, Romiti, M, et al. Dose-response relationship between very vigorous physical activity and cardiovascular health assessed by heart rate variability in adults: cross-sectional results from the EPIMOV study. PLoS One. (2019) 14:e0210216. doi: 10.1371/journal.pone.0210216
76. Koerte, IK, Schultz, V, Sydnor, VJ, Howell, DR, Guenette, JP, Dennis, E, et al. Sex-related differences in the effects of sports-related concussion: a review. J Neuroimaging. (2020) 30:387–409. doi: 10.1111/jon.12726
77. Gupte, R, Brooks, W, Vukas, R, Pierce, J, and Harris, J. Sex differences in traumatic brain injury: what we know and what we should know. J Neurotrauma. (2019) 36:3063–91. doi: 10.1089/neu.2018.6171
78. Bezherano, I, Haider, MN, Willer, BS, and Leddy, JJ. Practical management: prescribing subsymptom threshold aerobic exercise for sport-related concussion in the outpatient setting. Clin J Sport Med. (2021) 31:465–8. doi: 10.1097/JSM.0000000000000809
79. Hutchison, MG, Di Battista, AP, Lawrence, DW, Pyndiura, K, Corallo, D, and Richards, D. Randomized controlled trial of early aerobic exercise following sport-related concussion: progressive percentage of age-predicted maximal heart rate versus usual care. PLoS One. (2022) 17:e0276336. doi: 10.1371/journal.pone.0276336
Keywords: concussion, mild traumatic brain injury, aerobic exercise, quality of life, persisting post-concussive symptoms
Citation: Mercier LJ, McIntosh SJ, Boucher C, Joyce JM, Batycky J, Galarneau J-M, Burma JS, Smirl JD, Esser MJ, Schneider KJ, Dukelow SP, Harris AD and Debert CT (2024) Evaluating a 12-week aerobic exercise intervention in adults with persisting post-concussive symptoms. Front. Neurol. 15:1482266. doi: 10.3389/fneur.2024.1482266
Received: 18 August 2024; Accepted: 26 November 2024;
Published: 24 December 2024.
Edited by:
Ryan Tierney, Temple University, United StatesReviewed by:
Eirik Vikane, Haukeland University Hospital, NorwayCopyright © 2024 Mercier, McIntosh, Boucher, Joyce, Batycky, Galarneau, Burma, Smirl, Esser, Schneider, Dukelow, Harris and Debert. This is an open-access article distributed under the terms of the Creative Commons Attribution License (CC BY). The use, distribution or reproduction in other forums is permitted, provided the original author(s) and the copyright owner(s) are credited and that the original publication in this journal is cited, in accordance with accepted academic practice. No use, distribution or reproduction is permitted which does not comply with these terms.
*Correspondence: Leah J. Mercier, bGVhaC5tZXJjaWVyQHVjYWxnYXJ5LmNh
Disclaimer: All claims expressed in this article are solely those of the authors and do not necessarily represent those of their affiliated organizations, or those of the publisher, the editors and the reviewers. Any product that may be evaluated in this article or claim that may be made by its manufacturer is not guaranteed or endorsed by the publisher.
Research integrity at Frontiers
Learn more about the work of our research integrity team to safeguard the quality of each article we publish.