- Department of Neurology, Shandong Provincial Hospital Affiliated to Shandong First Medical University, Jinan, Shandong, China
Objective: This study aimed to investigate the roles of neurotrophic factors (NTFs), monoamine neurotransmitters, and inflammatory processes in the pathophysiology of the comorbidity of epilepsy and depression.
Methods: A retrospective analysis was conducted with 57 epilepsy patients (PWE), 50 patients with epilepsy and comorbid depression (PWECD), and 47 healthy controls (HC) admitted between June 2020 and June 2024. Serum levels of brain-derived neurotrophic factor (BDNF), glial-derived neurotrophic factor (GDNF), 5-hydroxytryptamine (5-HT), norepinephrine (NE), dopamine (DA), interleukin-1β (IL-1β), and interleukin-6 (IL-6) were measured using enzyme-linked immunosorbent assay (ELISA). Additionally, BDNF and GDNF levels in cerebrospinal fluid (CSF) samples were analyzed from selected patients in the PWE and PWECD groups.
Results: Serum BDNF levels were significantly lower in both PWE and PWECD groups compared to HC, while no differences between the former two groups. GDNF levels were lower in PWECD compared to HC, but not between PWE and HC. Serum 5-HT was significantly reduced in PWECD compared to both HC and PWE groups. No significant differences were observed in serum DA, NE, and IL-6 levels across the groups. Serum IL-1β levels were elevated in the PWECD group compared to the HC group. The Self-Rating Depression Scale (SDS) score negatively correlated with serum 5-HT and GDNF levels. In terms of predictive ability, serum BDNF demonstrated higher accuracy for the diagnosis of epilepsy [area under the curve, AUC = 0.701, 95% confidence intervals (95% CI) 0.601 ~ 0.801], while serum 5-HT was the best marker for predicting the development of depression in epilepsy patients (AUC = 0.727, 95% CI 0.632 ~ 0.821). No significant correlation was found between serum and CSF BDNF levels within the same subject (r = 0.155; p = 0.221; Spearman correlation), and CSF GDNF levels were too low to be clinically informative.
Conclusion: The findings suggest the involvement of NTFs, monoamine neurotransmitters, and inflammatory processes in the pathogenesis of epilepsy and depression. Decreased serum BDNF levels correlate with epilepsy but not necessarily with comorbid depression, while serum GDNF and 5-HT show potential clinical value in diagnosing this comorbidity. However, the deficient levels of NTFs in CSF suggest a need for more sensitive detection methods.
1 Introduction
Epilepsy, a neurological disorder affecting over 70 million people worldwide, is frequently accompanied by cognitive and psychological challenges (1, 2). Depression is the most common psychiatric comorbidity in patients with epilepsy (PWE), affecting approximately one-third of individuals, especially those with temporal lobe epilepsy (TLE) and poorly controlled seizures (3, 4). Depression has been associated with the course of epilepsy (5), poor quality of life (than seizure frequency) (6), suicide attempt (7). An epidemiological study found that 9.3% of epilepsy patients had undiagnosed major depressive disorder (8). The inability to promptly identify and intervene for patients with epilepsy and comorbid depression (PWECD) greatly diminishes the quality of life for both patients with epilepsy and their caregivers (9, 10). The mechanisms causing PWECD are poorly understood, highlighting the urgent need to study further.
Neurotrophic factors (NTFs) are endogenous peptides or small proteins that regulate the proliferation, migration, differentiation, and survival of neural cells in the nervous system. They are essential for maintaining the function and structure of neurons, acting as critical molecular mediators in central synaptic plasticity (11). Dysregulation of NTFs, particularly brain-derived neurotrophic factor (BDNF), ciliary neurotrophic factor (CNTF), glial cell line-derived neurotrophic factor (GDNF), and nerve growth factor (NGF), has been implicated in various cerebral disorders, including epilepsy and depression. Therefore, NTFs can provide insights into the neuroplastic changes associated with these conditions.
Monoamine neurotransmitters, including dopamine (DA), 5-hydroxytryptamine (5-HT), and norepinephrine (NE), are fundamental to mood regulation. Dysfunction in these neurotransmitters is widely recognized as the biochemical basis for depression (11), and is associated with increased vulnerability to comorbid depression in individuals with epilepsy (12, 13). Additionally, neuroinflammation has emerged as a significant contributor to increased neural excitability and neurotransmitter imbalances (14), indicating a strong link between psychiatric symptoms and inflammation in epilepsy. The interaction between inflammation and neurotransmitters may be a common mechanism underlying both epilepsy and depression, warranting further research.
This study aimed to investigate the mechanisms underlying the co-occurrence of epilepsy and depression by comparing neurotrophic factors, monoamine neurotransmitters, and inflammatory markers among PWE, PWECD, and healthy controls (HC).
2 Materials and methods
2.1 Patients
We conducted a study which included patients with epileptic seizures who admitted to the epilepsy monitoring unit (EMU) of the Shandong Provincial Hospital affiliated with Shandong First Medical University from June 2020 to June 2024 (Figure 1). All the patients with epilepsy underwent electroencephalography (EEG) and magnetic resonance imaging (MRI) of the brain. Both neurologists and psychiatrists evaluated and diagnosed the patients to confirm the presence of epilepsy, with or without comorbid depression, based on the epilepsy criteria outlined by the International League Against Epilepsy (ILAE) (15–17), as well as the DSM-V (The Diagnostic and Statistical Manual of Mental Disorders, 5th edition) and the Mini-International Neuropsychiatric Interview (M.I.N.I.). The severity of depression was evaluated utilizing the Chinese iteration of the Zung scale (SDS, Self-Rating Depression Scale). Cognitive ability was evaluated using the Mini-Mental State Exam (MMSE). Normal MMSE scores were defined as >23 for junior high school and higher education literates, > 20 for primary school literates, and > 17 for illiterate subjects.
Our inclusion criteria were: (1) an established diagnosis of epilepsy; (2) aged 16 years or older; (3) proficiency in the Chinese language; and (4) the ability to provide informed consent and adhere to the study protocol. Participants were excluded if they had: (1) abnormal MMSE scores; (2) alcohol and substance use disorders, psychotic disorders, attention deficit hyperactivity, bipolar disorders (depression with mania or hypomania), manic/hypomanic episodes, or other psychiatric diseases; (3) co-existing medical and/or neurological conditions; and (4) undetermined seizure frequency.
Based on the presence of depression, participants were classified into two groups: PWE (patients with epilepsy) and PWECD (patients with epilepsy and comorbid depression). A total of 107 patients aged 16 years and above, including individuals with epilepsy (PWE, n = 57) and those with epilepsy comorbid with depression (PWECD, n = 50), were recruited. Additionally, 47 generally healthy volunteers of similar age and gender who exhibited no signs of mental disorders at the time of study enrollment were included as HC based on their medical records.
All patients did not receive treatment with antipsychotic drugs, antidepressants, or sedatives. Fifty-eight patients (54.2%) had been administered appropriate antiseizure medications (ASMs) prescribed by experienced epileptologists (standard treatment). The pharmacological treatment for epilepsy patients included first-generation antiepileptic drugs (such as carbamazepine and valproate sodium), second-generation antiepileptic drugs (like lamotrigine, levetiracetam, oxcarbazepine, zonisamide, and topiramate), and novel antiepileptic medications (such as lacosamide and perampanel).
2.2 Sample collection and measurement
a. Cerebrospinal fluid (CSF) samples: Lumbar puncture was performed for clinical diagnostic purposes, and CSF samples were collected between 10–12 am to reduce potential circadian fluctuations in CSF composition. Routine laboratory analysis included evaluations for cell counts, total protein levels, and glucose concentrations. Extra 8–10 mL of CSF was promptly frozen at –80°C post-collection.
b. Blood samples were obtained via venipuncture after an 8-h fast, left at around 21°C to 23°C for 1 h, then centrifuged (2000 g × 10 min) and divided into smaller portions. Serum samples were transferred to Eppendorf tubes and stored at –80°C.
Neurotrophic factor, neurotransmitter, and inflammatory factor concentrations were determined utilizing human enzyme-linked immunosorbent assays (ELISA) sourced from commercially available kits (ELISA BDNF, GDNF, IL-1β, IL-6 kits; Multi Sciences, Hangzhou, China; ELISA 5-HT, DA, NE kits; Elisa Lab, Wuhan, China). For BDNF and GDNF levels in CSF, 100 μL of the undiluted test sample was added to the sample well, deviating from the manufacturer’s instructions due to the low concentrations of neurotrophic factors in CSF.
2.3 Statistical analysis
Statistical analysis was conducted using SPSS Statistics 26.0 software and GraphPad Prism version 9.4.1 software (GraphPad Software, Inc., San Diego, CA, USA). Continuous variables were summarized using mean and standard deviation, while categorical variables were reported as median with interquartile range or percentage. Normality of distribution was assessed using the Shapiro–Wilk and Kolmogorov–Smirnov tests. Quantitative data were compared across multiple independent groups using either the ANOVA test or the Kruskal–Wallis test based on their distribution. Post hoc analysis involved the Tukey test or the Bonferroni test. Categorical variable comparisons were conducted using the Chi-square test or Fisher’s exact test. Spearman rank correlation test was performed to evaluate the correlation between the CSF and serum levels of BDNF. Multiple linear regression analysis was performed to identify the independent factors affecting SDS scores. The SDS score served as the dependent variable, while the independent variables included age, gender, and serum levels of BDNF, GDNF, 5-HT, and IL-1β. A significance level of p < 0.05 was considered statistically significant. Receiver operating characteristic (ROC) curve analysis was used to assess the diagnostic performance of the potential biomarkers for differentiating PWE from HC group, as well as PWECD from PWE group. For each biomarker, the area under the curve (AUC) was calculated to evaluate diagnostic accuracy. The optimal cutoff values for distinguishing between groups were determined based on the highest Youden index.
3 Results
3.1 Characteristics of the patients and the healthy control groups
The demographic and clinical information for PWE, PWECD, and HC groups are presented in Table 1. No significant differences were found in gender, age, BMI, educational background, employment status, smoking habits, and common laboratory parameters. None of the participants in the study were taking antidepressant medications. Twenty-nine patients (51%) in the PWE group and 20 patients (40%) in the PWECD group were not on ASMs.
The PWECD group had higher levels of depressed mood and suicidal ideation compared to PWE, based on the SDS score. Cognitive levels (MMSE scale) showed no significant differences between PWE and PWECD. Seizure type did not impact cognitive and depression levels (Kruskal-Wallis test; for SDS, p = 0.403; for MMSE, p = 0.175), and seizure frequency did not impact cognitive and depression levels (Kruskal-Wallis test; for SDS, p = 0.630; for MMSE, p = 0.512).
3.2 Neurotrophic factors in serum and CSF
Serum BDNF levels were significantly lower in the PWE and PWECD groups compared to HC, with no difference between the PWE and PWECD groups (Figure 2A; Table 1). Serum GDNF levels were significantly lower in PWECD than HC, with no difference between the PWE and HC groups and between the PWE and PWECD (Figure 2A; Table 1).
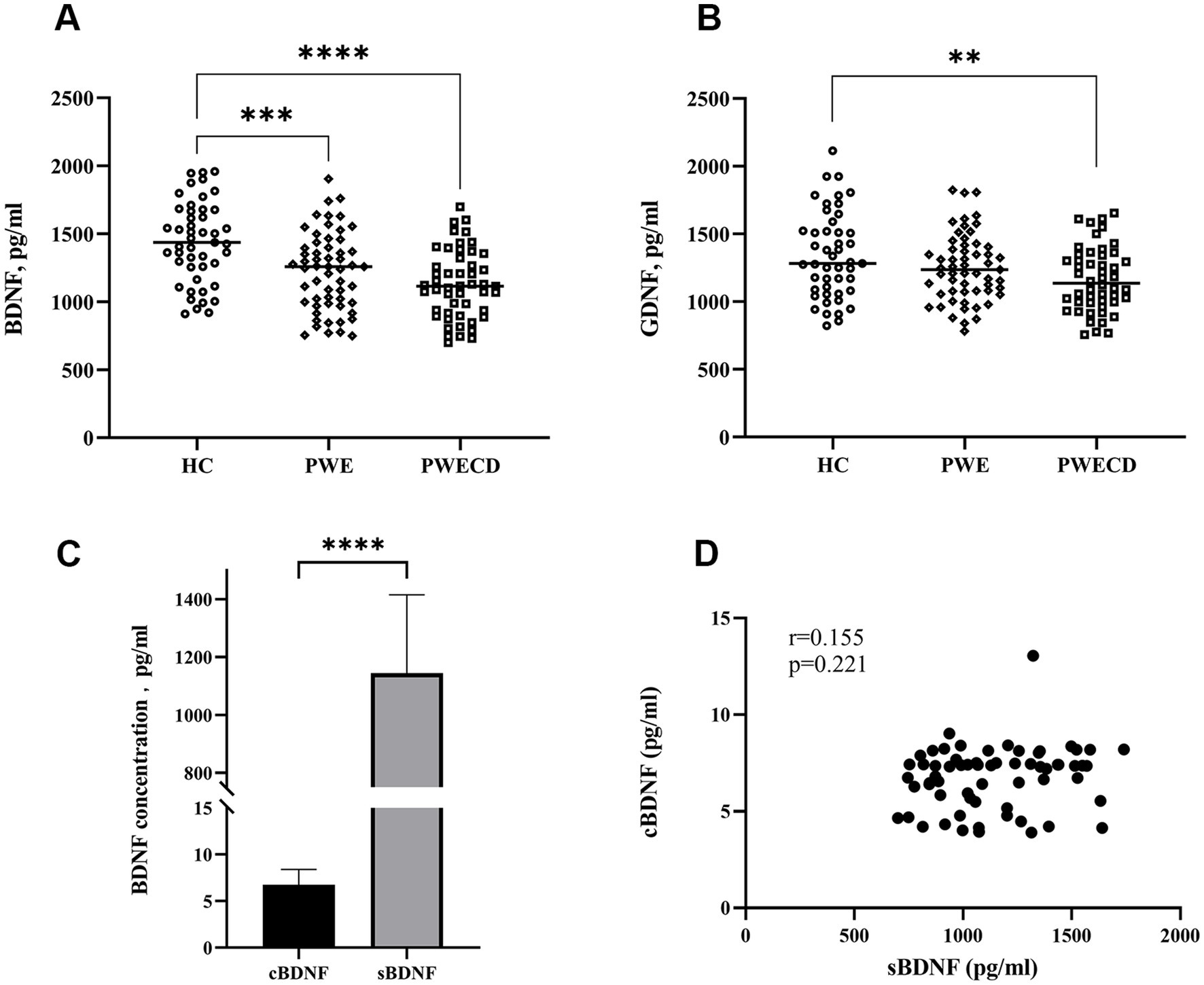
Figure 2. Neurotrophic factors in blood serum of PWE, PWCED, and the HC group: (A) BDNF; (B) GDNF. BDNF in biological fluids (cerebrospinal fluid and serum) of epilepsy patients with or without comorbid depression (C,D). One-way ANOVA (with post hoc Tukey test) for Neurotrophic factors was used to compare multiple unrelated groups. Differences in BDNF between serum and CSF were assessed by the Wilcoxon test. **p < 0.01, ***p < 0.001, ****p < 0.0001.
The CSF BDNF levels did not show significant differences between PWE and PWECD (Supplementary Table S1). There was an approximately 150-fold increase in the serum BDNF levels compared to the CSF levels (Figure 2C). No significant correlation was found between the serum and CSF BDNF levels within the same subject (r = 0.155; p = 0.221; Spearman correlation) (Figure 2D).
Although highly sensitive assays were employed (refer to the Sample collection and measurement section), CSF levels of GDNF were below the detection limit, providing no data to demonstrate its role in the central nervous system.
3.3 Monoamine neurotransmitters
Serum levels of 5-HT were significantly lower in the PWECD group compared to both the HC and PWE groups, with no difference between HC and PWE groups (Figure 3A; Table 1). Serum DA and NE levels showed no significant differences among the three groups (Figures 3B,C; Table 1).
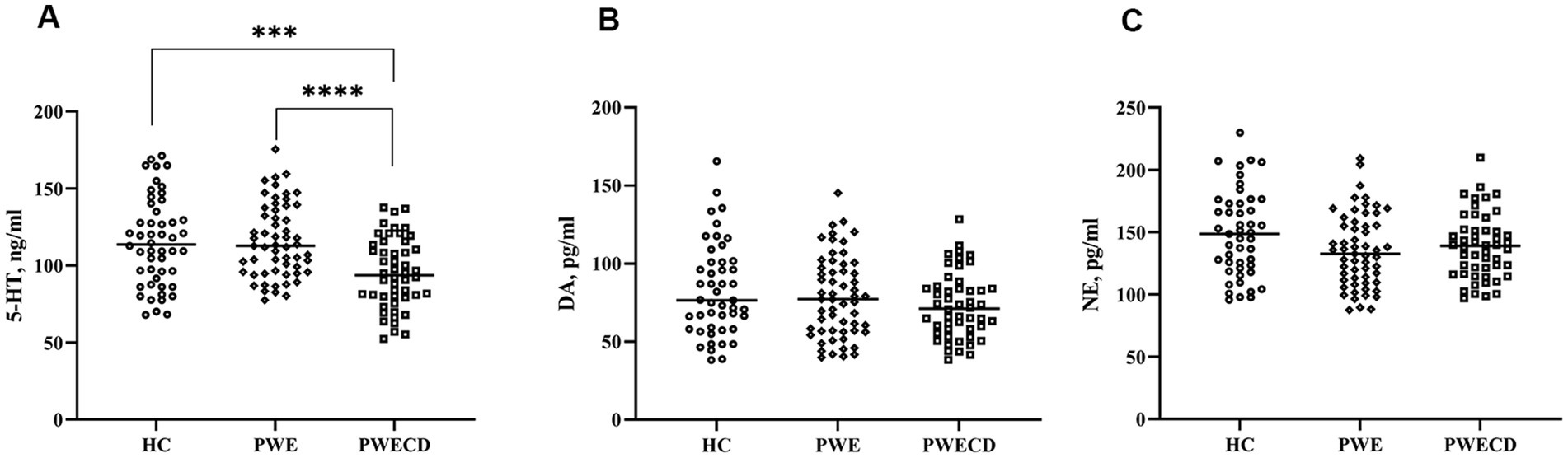
Figure 3. Monoamine neurotransmitters in blood serum of PWE, PWCED, and the HC group: (A) 5-HT; (B) DA; (C) NE. One-way ANOVA (with post hoc Tukey test) for Monoamine neurotransmitters was used to compare multiple unrelated groups. ***p < 0.001, ****p < 0.0001.
3.4 Inflammatory factors
Serum levels of IL-1β were significantly elevated in the PWECD group compared to HC (Figure 4A; Table 1), while there was no significant difference between the PWE and HC groups or between the PWE and PWECD groups. No significant differences were observed in IL-6 levels among the three groups (Figure 4B; Table 1).
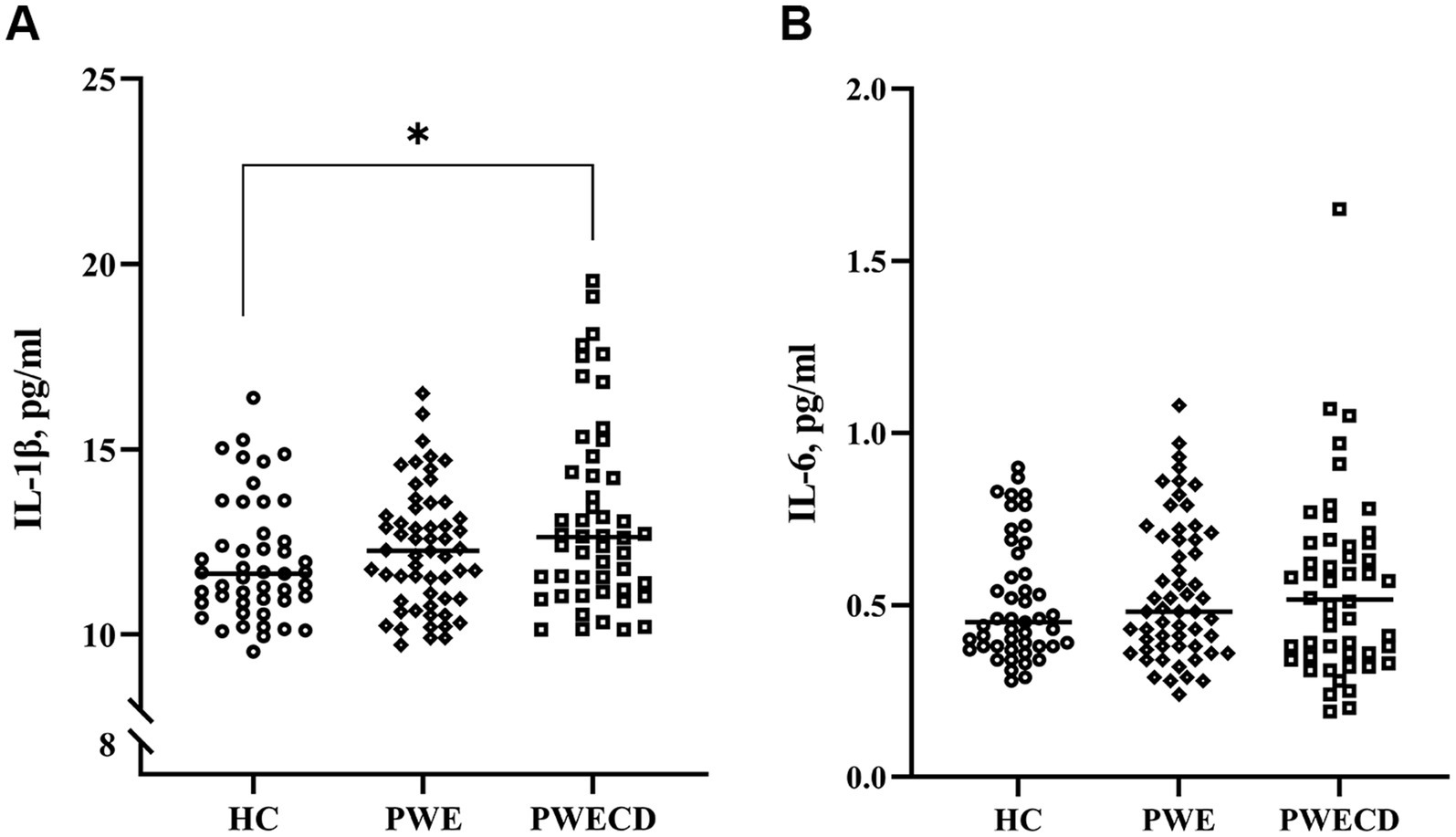
Figure 4. Inflammatory factors in blood serum of PWE, PWCED, and the HC group: (A) IL-1β; (B) IL-6. the Kruskal-Wallis test (with post hoc Bonferroni test) for IL-1β was used to compare multiple unrelated groups. *p < 0.05.
3.5 Multiple linear regression analysis for the SDS
Table 2 listed the details of Multiple Linear Regression Analysis. After adjusting for age and gender, multiple linear regression analysis demonstrated that both 5-HT and GDNF were independent factors affecting SDS score among all 107 epilepsy patients (Beta = −0.345, p < 0.001; Beta = −0.228, p = 0.042, respectively). Age, gender, BDNF and IL-1β had no significant impacts on the SDS score. Based on the standardized coefficient, there was a significant negative correlation between serum 5-HT and SDS scores, as well as between serum GDNF and SDS scores.
3.6 Optimal cutoffs of BDNF, GDNF, 5-HT, NE, DA, IL-1β, IL-6 predictors for the probability assessment of PWE, PWECD
The diagnostic performance of various biomarkers was evaluated using ROC curve analysis. Among these, BDNF demonstrated the highest efficacy in distinguishing PWE from HC, with an area under the curve (AUC) of 0.701 (p < 0.001) and an optimal cutoff value of <1360.16, as shown in Figure 5A and Table 3. Additionally, serum 5-HT levels were found to be the most effective in distinguishing PWECD from PWE, with an AUC of 0.727 (p = 0.001) and an optimal cutoff value of <93.09, as shown in Figure 5B and Table 4.
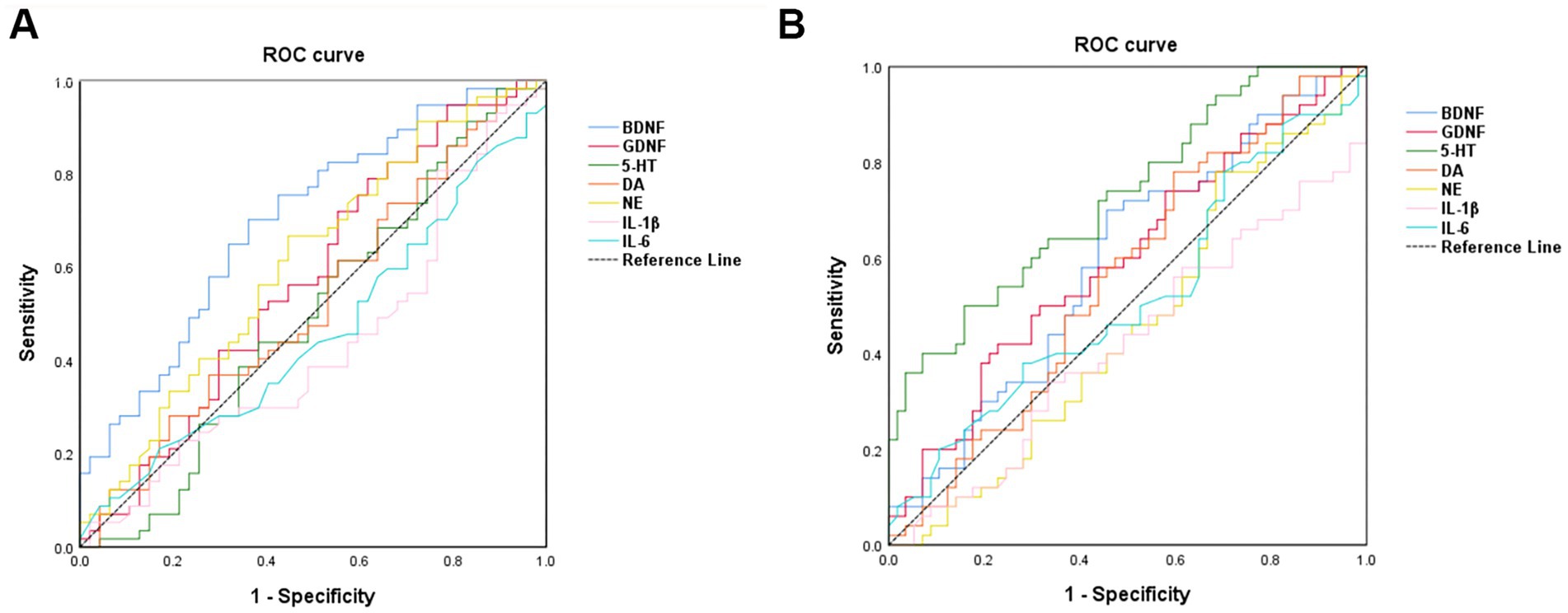
Figure 5. Receiver operating characteristic curve analysis of the 7 serum markers. (A) BDNF was the best marker to discriminate PWE from HC (area under the curve = 0.701). (B) 5-HT was the best marker to discriminate PWCED from PWE (area under the curve = 0.727).
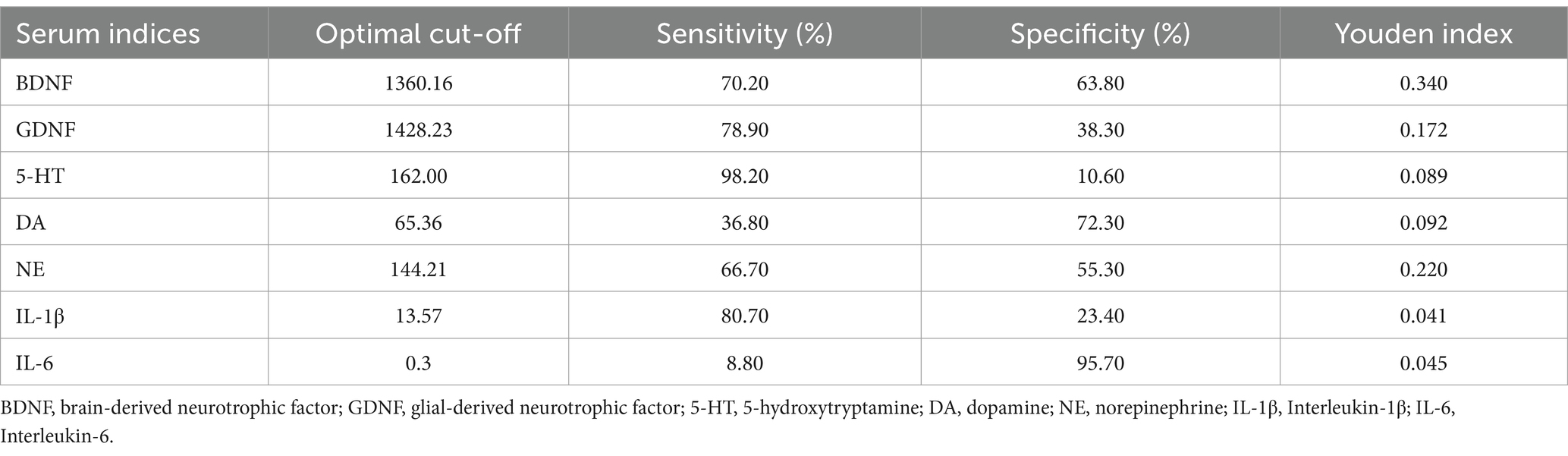
Table 3. Clinical utility indexes of neurotrophic factors, monoamine neurotransmitters, and inflammatory factors for predicting patients with epilepsy.
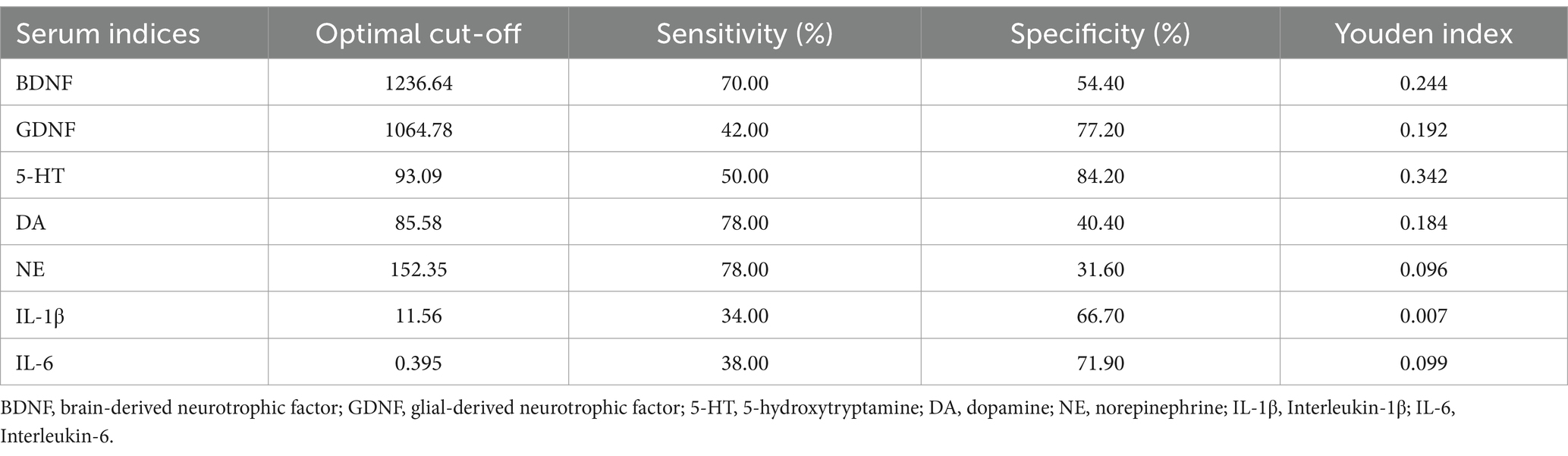
Table 4. Clinical utility indexes of neurotrophic factors, monoamine neurotransmitters, and inflammatory factors for predicting epilepsy comorbid with depression.
4 Discussion
In this study, we revealed the significant differences in serum BDNF, GDNF, 5-HT, and IL-1β among the HC, PWE, and PWECD groups, along with the negative correlation with depression severity in serum GDNF and 5-HT levels, supporting their role in epilepsy and comorbid depression.
It is not surprising to find decreased serum levels of GDNF and 5-HT in the PWECD group compared to the control. GDNF functions by binding to its receptor GFR-α1 and activating the c-Ret tyrosine kinase signaling pathway, which supports the development, survival, and differentiation of dopaminergic, serotonergic, and GABAergic neurons (18–20). Given our results showing lower serum GDNF levels in the PWECD group, we hypothesize that GDNF deficiency-induced neurotransmitter disruption may be a key mechanism in the pathogenesis of depression comorbid with epilepsy. Recent studies have found that reduced levels of GDNF in plasma (21), serum (22), and lacrimal fluid (23) are significant for diagnosing and predicting depressive disorders, providing further support for our hypothesis. Similarly, 5-HT is widely recognized as a crucial neurotransmitter for mood regulation and is a classical target of antidepressant therapies, helping explain our findings of significantly decreased 5-HT levels in the PWECD group. Furthermore, the ROC curve analysis demonstrated the ability to distinguish between PWE and PWECD groups, highlighting the potential of 5-HT as a biomarker for identifying depression comorbid with epilepsy.
Interestingly, while BDNF levels also decreased in both PWE and PWECD groups compared with the control, there was no difference between the PWE and PWECD groups. This observation is consistent with previous studies (24–26), which have also reported decreased BDNF levels in the serum of epilepsy patients, aligning with our results and reinforcing the association between BDNF reduction and seizure activity. Although reduced serum BDNF levels have been linked to be associated with an increased risk of developing major depression and suicidal ideation (27, 28), findings are inconsistent across all studies (29, 30), and the underlying mechanisms remain unclear. It is possible that central BDNF levels, rather than serum BDNF, may be more closely correlated with mood disorders and suicidality, as studies have demonstrated lower BDNF levels in the prefrontal cortex and hippocampus of individuals with major depression and those who have died by suicide (31–33). Despite our attempt to assess BDNF changes in CSF, we found no significant differences between the PWE and PWECD groups and could not establish a correlation between serum and CSF BDNF levels. Based on our current findings and literature review (34), it remains unclear whether the reduction in serum BDNF levels associated with recurrent seizures could be a mechanism contributing to the development of PWECD. This question warrants further investigation.
Preclinical studies have shown that seizures can be suppressed by the overexpression of BDNF or GDNF in the hippocampus or cerebral cortex in animal models of epilepsy, suggesting that the lack of these NTFs may play a role in epileptogenesis (35–37). However, due to the presence of the blood–brain barrier (BBB), serum NTF levels do not necessarily reflect NTFs levels in brain tissue. NTFs are released from both peripheral tissues and the central nervous system. For instance, in the CNS, neurons, astrocytes, and microglia are the main producers of BDNF, whereas BDNF is also extensively synthesized by peripheral cells, such as megakaryocytes in endothelial cells (38), bone marrow (39), and various immune cells (40), including B and T lymphocytes and monocytes. The relationship between NTFs levels in the CNS and peripheral systems, as well as their clinical relevance to CNS disorders, remains unclear. Early studies in rodents suggested the use of peripheral measurements as proxies for CNS levels (41, 42). However, recent research has challenged this assumption (43–45). Our study contributes to this discussion by demonstrating the lack of a clear correlation between CSF and serum NTFs. Using ELISA to measure BDNF and GDNF in undiluted CSF, we found that GDNF levels in CSF were too low to detect, and BDNF levels, though measurable, were more than 100 times lower than in serum. These findings highlight the challenges of relying on CSF-based BDNF measurements in clinical practice (46). This significant discrepancy may be attributed to the dual synthesis of NTFs in both the central and peripheral systems, as well as the effects of the BBB (47). Therefore, further investigation is need to clarify the implications of decreased peripheral NTF levels for diagnosing and managing nervous system disorders.
Although our results underscore the significant roles of NTFs in epilepsy or depression, their involvement in other neuropsychiatric disorders, particularly neurodegenerative diseases such as Parkinson’s disease (PD), Alzheimer’s disease (AD), Huntington’s disease (HD), and amyotrophic lateral sclerosis (ALS), is also receiving growing attention (48). GDNF has been extensively studied in PD, where reduced serum levels are associated with the degeneration of dopaminergic neurons. GDNF supports the survival and differentiation of these neurons, and clinical trials involving direct brain delivery of GDNF have shown promise in halting or even reversing motor symptoms in PD patients (49, 50). Similarly, BDNF is vital for synaptic plasticity and memory formation, playing a key role in diseases like HD and AD. BDNF supports various neuron types, including cholinergic and dopaminergic neurons, and its depletion has been linked to cognitive decline and motor dysfunction (51, 52). Both GDNF and BDNF are increasingly implicated in a broader range of neurodegenerative and neuropsychiatric disorders, and understanding their interactions with disease pathology could position them as vital therapeutic targets.
Neuroinflammatory changes have been identified in cerebrospinal fluid (CSF) and brain samples from patients with epilepsy, especially those with refractory epilepsy (53, 54). The pathogenesis may involve prolonged activation that leads to local microgliosis and astrogliosis, as well as disruption of the blood–brain barrier (BBB), contributing to chronic neuroinflammation and increased synaptic excitability (55–57). Additionally, sterile neuroinflammation is suggested as a fundamental mechanism underlying various psychiatric disorders and PWECD as well (56). We found that IL-1β levels were significantly elevated in the PWECD group, further supporting the hypothesis of neuroinflammation’s role. The reason for highlighting neuroinflammation is that if we aim to investigate the distribution of NTFs in serum and CSF further and confirm their roles in PWECD and PWE, it is essential to take into consideration the impact of chronic inflammation and the potential effects of BBB disruption. Understanding these factors will be crucial for elucidating the mechanisms of NTFs in these conditions. However, the current results do not yet demonstrate a direct relationship between these factors.
This study has the following limitations. First, it predominantly provides insights into a clinical phenomenon through observational outcomes and further studies are needed to investigate the underlying mechanisms in detail. (1) Employing more sensitive detection methods to accurately measure low levels of NTFs in cerebrospinal fluid (CSF); (2) Paying attention to potential neuroinflammatory mechanisms and assessing whether changes in blood–brain barrier permeability may affect the distribution of NTFs in the CSF and serum; and (3) Conducting longitudinal studies and intervention trials to clarify the temporal and causal relationships between these biomarkers and comorbid depression in epilepsy patients, and to seek more reliable evidence validating the clinical significance of these biomarkers in disease pathogenesis and prognosis.
5 Conclusion
In conclusion, this study identified significant differences in serum BDNF, GDNF, 5-HT, and IL-1β among the HC, PWE, and PWECD groups, suggesting their involvement in epilepsy and comorbid depression. The negative correlation between GDNF, 5-HT and the SDS score highlights their potential as biomarkers for PWECD. These findings enhance our understanding of PWECD and may pave the way for new diagnostic and therapeutic approaches in the future.
Data availability statement
The raw data supporting the conclusions of this article will be made available by the authors, without undue reservation.
Ethics statement
The studies involving humans were approved by the Ethics Committee of the Shandong Provincial Hospital Affiliated to Shandong First Medical University (SWYX: NO. 2024-381). The studies were conducted in accordance with the local legislation and institutional requirements. The participants provided their written informed consent to participate in this study.
Author contributions
SS: Writing – review & editing, Writing – original draft, Supervision, Methodology, Formal analysis, Data curation, Conceptualization. YH: Formal analysis, Data curation, Writing – review & editing, Writing – original draft, Methodology, Conceptualization. XL: Writing – review & editing, Writing – original draft, Methodology, Formal analysis, Data curation. LY: Writing – review & editing, Data curation. TH: Writing – review & editing, Writing – original draft. YL: Writing – review & editing, Data curation. YF: Writing – review & editing, Writing – original draft, Supervision, Project administration, Methodology, Conceptualization.
Funding
The author(s) declare that financial support was received for the research, authorship, and/or publication of this article. This work was supported by the Natural Science Foundation of Shandong Province (Grant No. ZR2020MH152).
Acknowledgments
The authors express their gratitude to the dedicated staff of the Neurology Subject Group at Shandong Provincial Hospital Affiliated to Shandong First Medical University.
Conflict of interest
The authors declare that the research was conducted in the absence of any commercial or financial relationships that could be construed as a potential conflict of interest.
Publisher’s note
All claims expressed in this article are solely those of the authors and do not necessarily represent those of their affiliated organizations, or those of the publisher, the editors and the reviewers. Any product that may be evaluated in this article, or claim that may be made by its manufacturer, is not guaranteed or endorsed by the publisher.
Supplementary material
The Supplementary material for this article can be found online at: https://www.frontiersin.org/articles/10.3389/fneur.2024.1480854/full#supplementary-material
References
1. Kanner, AM. Psychiatric comorbidities in new onset epilepsy: should they be always investigated? Seizure. (2017) 49:79–82. doi: 10.1016/j.seizure.2017.04.007
2. Keezer, MR, Sisodiya, SM, and Sander, JW. Comorbidities of epilepsy: current concepts and future perspectives. Lancet Neurol. (2016) 15:106–15. doi: 10.1016/S1474-4422(15)00225-2
3. Ajinkya, S, Fox, J, and Lekoubou, A. Trends in prevalence and treatment of depressive symptoms in adult patients with epilepsy in the United States. Epilepsy Behav. (2020) 105:106973. doi: 10.1016/j.yebeh.2020.106973
4. Kanner, AM, and Balabanov, A. Depression and epilepsy: how closely related are they? Neurology. (2002) 58:S27–39. doi: 10.1212/wnl.58.8_suppl_5.s27
5. Ribot, R, and Kanner, AM. Neurobiologic properties of mood disorders may have an impact on epilepsy: should this motivate neurologists to screen for this psychiatric comorbidity in these patients? Epilepsy Behav. (2019) 98:298–301. doi: 10.1016/j.yebeh.2019.01.026
6. Siarava, E, Hyphantis, T, Katsanos, AH, Pelidou, SH, Kyritsis, AP, and Markoula, S. Depression and quality of life in patients with epilepsy in Northwest Greece. Seizure. (2019) 66:93–8. doi: 10.1016/j.seizure.2019.02.012
7. Lin, CY, Harnod, T, Lin, CL, Shen, WC, and Kao, CH. Differences in incidence and risks of suicide attempt and suicidal drug overdose between patients with epilepsy with and without comorbid depression. Int J Environ Res Public Health. (2019) 16:4533. doi: 10.3390/ijerph16224533
8. Tan, JK, Khoo, CS, Beh, HC, Hod, R, Baharudin, A, Yahya, W, et al. Prevalence and associated risk factors of undiagnosed depression among people with epilepsy in a multiethnic society. Epilepsy Res. (2021) 178:106772. doi: 10.1016/j.eplepsyres.2021.106772
9. Mula, M, and Kaufman, KR. Double stigma in mental health: epilepsy and mental illness. BJPsych Open. (2020) 6:e72. doi: 10.1192/bjo.2020.58
10. Vinti, V, Dell'Isola, GB, Tascini, G, Mencaroni, E, Cara, GD, Striano, P, et al. Temporal lobe epilepsy and psychiatric comorbidity. Front Neurol. (2021) 12:775781. doi: 10.3389/fneur.2021.775781
11. Malhi, GS, and Mann, JJ. Depression. Lancet. (2018) 392:2299–312. doi: 10.1016/S0140-6736(18)31948-2
12. Medel-Matus, JS, Shin, D, Sankar, R, and Mazarati, A. Inherent vulnerabilities in monoaminergic pathways predict the emergence of depressive impairments in an animal model of chronic epilepsy. Epilepsia. (2017) 58:e116–21. doi: 10.1111/epi.13822
13. Mazarati, A, Siddarth, P, Baldwin, RA, Shin, D, Caplan, R, and Sankar, R. Depression after status epilepticus: behavioural and biochemical deficits and effects of fluoxetine. Brain. (2008) 131:2071–83. doi: 10.1093/brain/awn117
14. Shin, HR, Chu, K, Lee, WJ, Lee, HS, Kim, EY, Son, H, et al. Neuropsychiatric symptoms and seizure related with serum cytokine in epilepsy patients. Sci Rep. (2022) 12:7138. doi: 10.1038/s41598-022-10865-x
15. Fisher, RS, Cross, JH, D'Souza, C, French, JA, Haut, SR, Higurashi, N, et al. Instruction manual for the ILAE 2017 operational classification of seizure types. Epilepsia. (2017) 58:531–42. doi: 10.1111/epi.13671
16. Fisher, RS, Cross, JH, French, JA, Higurashi, N, Hirsch, E, Jansen, FE, et al. Operational classification of seizure types by the international league against epilepsy: position paper of the ILAE Commission for Classification and Terminology. Epilepsia. (2017) 58:522–30. doi: 10.1111/epi.13670
17. Scheffer, IE, Berkovic, S, Capovilla, G, Connolly, MB, French, J, Guilhoto, L, et al. ILAE classification of the epilepsies: position paper of the ILAE Commission for Classification and Terminology. Epilepsia. (2017) 58:512–21. doi: 10.1111/epi.13709
18. Sharma, AN, Silva BF, DCE, Soares, JC, Carvalho, AF, and Quevedo, J. Role of trophic factors GDNF, IGF-1 and VEGF in major depressive disorder: a comprehensive review of human studies. J Affect Disord. (2016) 197:9–20. doi: 10.1016/j.jad.2016.02.067
19. Bonafina, A, Trinchero, MF, Ríos, AS, Bekinschtein, P, Schinder, AF, Paratcha, G, et al. GDNF and GFRα1 are required for proper integration of adult-born hippocampal neurons. Cell Rep. (2019) 29:4308–19.e4. doi: 10.1016/j.celrep.2019.11.100
20. Ducray, A, Krebs, SH, Schaller, B, Seiler, RW, Meyer, M, and Widmer, HR. GDNF family ligands display distinct action profiles on cultured GABAergic and serotonergic neurons of rat ventral mesencephalon. Brain Res. (2006) 1069:104–12. doi: 10.1016/j.brainres.2005.11.056
21. Liu, X, Li, P, Ma, X, Zhang, J, Sun, X, Luo, X, et al. Association between plasma levels of BDNF and GDNF and the diagnosis, treatment response in first-episode MDD. J Affect Disord. (2022) 315:190–7. doi: 10.1016/j.jad.2022.07.041
22. Zhang, Y, Jiang, H, Yue, Y, Yin, Y, Zhang, Y, Liang, J, et al. The protein and mRNA expression levels of glial cell line-derived neurotrophic factor in post stroke depression and major depressive disorder. Sci Rep. (2017) 7:8674. doi: 10.1038/s41598-017-09000-y
23. Shpak, AA, Rider, FK, Druzhkova, TA, Zhanina, MY, Popova, SB, Guekht, AB, et al. Reduced levels of lacrimal glial cell line-derived neurotrophic factor (GDNF) in patients with focal epilepsy and focal epilepsy with comorbid depression: a biomarker candidate. Int J Mol Sci. (2023) 24:818. doi: 10.3390/ijms242316818
24. Chen, NC, Chuang, YC, Huang, CW, Lui, CC, Lee, CC, Hsu, SW, et al. Interictal serum brain-derived neurotrophic factor level reflects white matter integrity, epilepsy severity, and cognitive dysfunction in chronic temporal lobe epilepsy. Epilepsy Behav. (2016) 59:147–54. doi: 10.1016/j.yebeh.2016.02.029
25. LaFrance, WCJr, Leaver, K, Stopa, EG, Papandonatos, GD, and Blum, AS. Decreased serum BDNF levels in patients with epileptic and psychogenic nonepileptic seizures. Neurology. (2010) 75:1285–91. doi: 10.1212/WNL.0b013e3181f612bb
26. Hong, Z, Li, W, Qu, B, Zou, X, Chen, J, Sander, JW, et al. Serum brain-derived neurotrophic factor levels in epilepsy. Eur J Neurol. (2014) 21:57–64. doi: 10.1111/ene.12232
27. Ai, M, Wang, J, Chen, J, Wang, W, Xu, X, Gan, Y, et al. Plasma brain-derived neurotrophic factor (BDNF) concentration and the BDNF Val66Met polymorphism in suicide: a prospective study in patients with depressive disorder. Pharmgenomics Pers Med. (2019) 12:97–106. doi: 10.2147/pgpm.S201187
28. Emon, MPZ, Das, R, Nishuty, NL, Shalahuddin Qusar, MMA, Bhuiyan, MA, and Islam, MR. Reduced serum BDNF levels are associated with the increased risk for developing MDD: a case-control study with or without antidepressant therapy. BMC Res Notes. (2020) 13:83. doi: 10.1186/s13104-020-04952-3
29. Filimonova, EA, Pashkov, AA, Moysak, GI, Tropynina, AY, Zhanaeva, SY, Shvaikovskaya, AA, et al. Brain but not serum BDNF levels are associated with structural alterations in the hippocampal regions in patients with drug-resistant mesial temporal lobe epilepsy. Front Neurosci. (2023) 17:1217702. doi: 10.3389/fnins.2023.1217702
30. Alvim, MKM, Morita-Sherman, ME, Yasuda, CL, Rocha, NP, Vieira, ÉL, Pimentel-Silva, LR, et al. Inflammatory and neurotrophic factor plasma levels are related to epilepsy independently of etiology. Epilepsia. (2021) 62:2385–94. doi: 10.1111/epi.17023
31. Ray, MT, Shannon Weickert, C, and Webster, MJ. Decreased BDNF and TrkB mRNA expression in multiple cortical areas of patients with schizophrenia and mood disorders. Transl Psychiatry. (2014) 4:e389. doi: 10.1038/tp.2014.26
32. Thompson Ray, M, Weickert, CS, Wyatt, E, and Webster, MJ. Decreased BDNF, trkB-TK+ and GAD67 mRNA expression in the hippocampus of individuals with schizophrenia and mood disorders. J Psychiatry Neurosci. (2011) 36:195–203. doi: 10.1503/jpn.100048
33. Guilloux, JP, Douillard-Guilloux, G, Kota, R, Wang, X, Gardier, AM, and Martinowich, K. Molecular evidence for BDNF-and GABA-related dysfunctions in the amygdala of female subjects with major depression. Mol Psychiatry. (2012) 17:1130–42. doi: 10.1038/mp.2011.113
34. Nowroozi, A, Salehi, MA, and Mohammadi, S. Brain-derived neurotrophic factor in patients with epilepsy: a systematic review and meta-analysis. Epilepsy Res. (2021) 178:106794. doi: 10.1016/j.eplepsyres.2021.106794
35. Kanter-Schlifke, I, Georgievska, B, Kirik, D, and Kokaia, M. Seizure suppression by GDNF gene therapy in animal models of epilepsy. Mol Ther. (2007) 15:1106–13. doi: 10.1038/sj.mt.6300148
36. Kustova, AO, Gavrish, MS, Sergeeva, MA, Avlasenko, DA, Kiseleva, AO, Epifanova, EA, et al. The influence of neurotrophic factors BDNF and GDNF overexpression on the functional state of mice and their adaptation to Audiogenic seizures. Brain Sci. (2022) 12:1039. doi: 10.3390/brainsci12081039
37. Paolone, G, Falcicchia, C, Lovisari, F, Kokaia, M, Bell, WJ, Fradet, T, et al. Long-term, targeted delivery of GDNF from encapsulated cells is neuroprotective and reduces seizures in the pilocarpine model of epilepsy. J Neurosci. (2019) 39:2144–56. doi: 10.1523/jneurosci.0435-18.2018
38. Grasman, JM, and Kaplan, DL. Human endothelial cells secrete neurotropic factors to direct axonal growth of peripheral nerves. Sci Rep. (2017) 7:4092. doi: 10.1038/s41598-017-04460-8
39. Chacón-Fernández, P, Säuberli, K, Colzani, M, Moreau, T, Ghevaert, C, Barde, YA, et al. Brain-derived neurotrophic factor in megakaryocytes. J Biol Chem. (2016) 291:9872–81. doi: 10.1074/jbc.M116.720029
40. Kerschensteiner, M, Gallmeier, E, Behrens, L, Leal, VV, Misgeld, T, Klinkert, WE, et al. Activated human T cells, B cells, and monocytes produce brain-derived neurotrophic factor in vitro and in inflammatory brain lesions: a neuroprotective role of inflammation? J Exp Med. (1999) 189:865–70. doi: 10.1084/jem.189.5.865
41. Klein, AB, Williamson, R, Santini, MA, Clemmensen, C, Ettrup, A, Rios, M, et al. Blood BDNF concentrations reflect brain-tissue BDNF levels across species. Int J Neuropsychopharmacol. (2011) 14:347–53. doi: 10.1017/s1461145710000738
42. Pan, W, Banks, WA, Fasold, MB, Bluth, J, and Kastin, AJ. Transport of brain-derived neurotrophic factor across the blood-brain barrier. Neuropharmacology. (1998) 37:1553–61. doi: 10.1016/s0028-3908(98)00141-5
43. Tikhonova, MA, Shvaikovskaya, AA, Zhanaeva, SY, Moysak, GI, Akopyan, AA, Rzaev, JA, et al. Concordance between the in vivo content of Neurospecific proteins (BDNF, NSE, VILIP-1, S100B) in the Hippocampus and blood in patients with epilepsy. Int J Mol Sci. (2023) 25:502. doi: 10.3390/ijms25010502
44. Gadad, BS, Vargas-Medrano, J, Ramos, EI, Najera, K, Fagan, M, Forero, A, et al. Altered levels of interleukins and neurotrophic growth factors in mood disorders and suicidality: an analysis from periphery to central nervous system. Transl Psychiatry. (2021) 11:341. doi: 10.1038/s41398-021-01452-1
45. Bharani, KL, Ledreux, A, Gilmore, A, Carroll, SL, and Granholm, AC. Serum pro-BDNF levels correlate with phospho-tau staining in Alzheimer's disease. Neurobiol Aging. (2020) 87:49–59. doi: 10.1016/j.neurobiolaging.2019.11.010
46. Mizui, T, Hattori, K, Ishiwata, S, Hidese, S, Yoshida, S, Kunugi, H, et al. Cerebrospinal fluid BDNF pro-peptide levels in major depressive disorder and schizophrenia. J Psychiatr Res. (2019) 113:190–8. doi: 10.1016/j.jpsychires.2019.03.024
47. Thorne, RG, and Frey, WH2nd. Delivery of neurotrophic factors to the central nervous system: pharmacokinetic considerations. Clin Pharmacokinet. (2001) 40:907–46. doi: 10.2165/00003088-200140120-00003
48. Allen, SJ, Watson, JJ, Shoemark, DK, Barua, NU, and Patel, NK. GDNF, NGF and BDNF as therapeutic options for neurodegeneration. Pharmacol Ther. (2013) 138:155–75. doi: 10.1016/j.pharmthera.2013.01.004
49. Bondarenko, O, and Saarma, M. Neurotrophic factors in Parkinson's disease: clinical trials, open challenges and nanoparticle-mediated delivery to the brain. Front Cell Neurosci. (2021) 15:682597. doi: 10.3389/fncel.2021.682597
50. Barker, RA, Björklund, A, Gash, DM, Whone, A, Van Laar, A, Kordower, JH, et al. GDNF and Parkinson's disease: where next? A summary from a recent workshop. J Parkinsons Dis. (2020) 10:875–91. doi: 10.3233/jpd-202004
51. Budni, J, Bellettini-Santos, T, Mina, F, Garcez, ML, and Zugno, AI. The involvement of BDNF, NGF and GDNF in aging and Alzheimer's disease. Aging Dis. (2015) 6:331–41. doi: 10.14336/ad.2015.0825
52. Gao, L, Zhang, Y, Sterling, K, and Song, W. Brain-derived neurotrophic factor in Alzheimer's disease and its pharmaceutical potential. Transl Neurodegener. (2022) 11:4. doi: 10.1186/s40035-022-00279-0
53. de Vries, EE, van den Munckhof, B, Braun, KP, van Royen-Kerkhof, A, de Jager, W, and Jansen, FE. Inflammatory mediators in human epilepsy: a systematic review and meta-analysis. Neurosci Biobehav Rev. (2016) 63:177–90. doi: 10.1016/j.neubiorev.2016.02.007
54. Campos-Bedolla, P, Feria-Romero, I, and Orozco-Suárez, S. Factors not considered in the study of drug-resistant epilepsy: drug-resistant epilepsy: assessment of neuroinflammation. Epilepsia Open. (2022) 7 Suppl 1:S68–s80. doi: 10.1002/epi4.12590
55. Vezzani, A, French, J, Bartfai, T, and Baram, TZ. The role of inflammation in epilepsy. Nat Rev Neurol. (2011) 7:31–40. doi: 10.1038/nrneurol.2010.178
56. Bauer, ME, and Teixeira, AL. Neuroinflammation in mood disorders: role of regulatory immune cells. Neuroimmunomodulation. (2021) 28:99–107. doi: 10.1159/000515594
Keywords: epilepsy, depression, brain-derived neurotrophic factor, glial cell line-derived neurotrophic factor, blood serum, cerebrospinal fluid, monoamine neurotransmitters
Citation: Sun S, Han Y, Liu X, Yang L, Han T, Lin Y and Feng Y (2024) Reduced serum neurotrophic factors and monoamine neurotransmitters in epilepsy patients with comorbid depression. Front. Neurol. 15:1480854. doi: 10.3389/fneur.2024.1480854
Edited by:
Rani Sarkis, Brigham and Women’s Hospital and Harvard Medical School, United StatesReviewed by:
Ching Soong Khoo, National University of Malaysia, MalaysiaRodrigo Ramos-Zúñiga, University of Guadalajara, Mexico
Copyright © 2024 Sun, Han, Liu, Yang, Han, Lin and Feng. This is an open-access article distributed under the terms of the Creative Commons Attribution License (CC BY). The use, distribution or reproduction in other forums is permitted, provided the original author(s) and the copyright owner(s) are credited and that the original publication in this journal is cited, in accordance with accepted academic practice. No use, distribution or reproduction is permitted which does not comply with these terms.
*Correspondence: Youting Lin, linyouting@hotmail.com; Yabo Feng, fyb-sd@hotmail.com