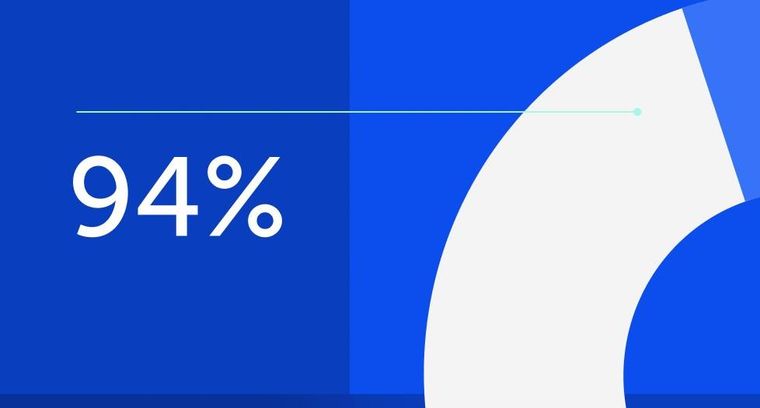
94% of researchers rate our articles as excellent or good
Learn more about the work of our research integrity team to safeguard the quality of each article we publish.
Find out more
ORIGINAL RESEARCH article
Front. Neurol., 10 December 2024
Sec. Neurorehabilitation
Volume 15 - 2024 | https://doi.org/10.3389/fneur.2024.1469877
This article is part of the Research TopicExploring Evidence for Neurorehabilitation AdvancementsView all 25 articles
Background: Theta burst stimulation (TBS) has garnered widespread attention in the scientific community, but a comprehensive bibliometric analysis of TBS research remains absent. This study aims to fill this gap by elucidating the characteristics, hotspots, and trends in TBS publications over the past 20 years using bibliometric methods.
Methods: We retrieved TBS-related publications from January 1, 2004, to December 31, 2023, from the Web of Science Core Collection (WoSCC). The analysis focused on articles and review articles. Data were processed using the bibliometric package in R software, and CiteSpace and VOSviewer were employed for bibliometric and knowledge mapping analyses.
Results: A total of 1,206 publications were identified, with 858 included in the analysis. The annual publication volume showed a fluctuating upward trend. Leading institutions and authors were predominantly from the United States of America (USA) and European countries. Core journals and publications also primarily originated from these regions. Current research hotspots include the clinical applications and mechanisms of TBS in neurorehabilitation and depression. TBS cerebellar stimulation has emerged as a promising therapeutic target. Future research is likely to focus on dysphagia, cognitive impairments, and post-traumatic stress disorder.
Conclusion: This bibliometric analysis provides an overview of the basic knowledge structure, research hotspots, and development trends in TBS research over the past two decades. The findings offer valuable insights into the evolving landscape of TBS research and its potential directions.
Endogenous theta frequency oscillations in hippocampal and cortical circuits are critical for learning, motor function, and memory processing (1). Theta burst stimulation (TBS) mimics this natural electrophysiological activity, providing a unique non-invasive neural stimulation method (2). The most commonly used TBS paradigms include intermittent TBS (iTBS) and continuous TBS (cTBS), each modulating cortical excitability through distinct stimulation patterns. The iTBS protocol consists of 2 s of continuous stimulation followed by an 8-s interval, repeated in cycles, and is believed to induce long-term potentiation (LTP) (3). In contrast, cTBS involves uninterrupted bursts of stimulation at a fixed frequency for 40 to 50 s, which is thought to induce long-term depression (LTD) (4). LTP and LTD are fundamental concepts in synaptic plasticity, considered key mechanisms underlying learning and memory. However, the manifestation and functional role of these mechanisms in the human brain remain contentious, as most human studies on LTP and LTD are extrapolated from animal models (5, 6). Some research suggests that other forms of synaptic plasticity, such as short-term plasticity and synaptic normalization mechanisms, may also play significant roles in learning and memory, potentially interacting with LTP and LTD in a collaborative manner (7). As a form of patterned repetitive transcranial magnetic stimulation (rTMS), TBS offers several advantages over traditional rTMS, including shorter stimulation times, lower intensity, longer-lasting effects, and a stimulation pattern that more closely resembles natural neural activity (8). These characteristics not only enhance the safety and comfort of TBS but also improve its specificity and efficacy in modulating neural network functions (9). Therefore, TBS holds significant potential in both basic neuroscience research and clinical applications, providing new perspectives on the regulation of brain function (10–13).
Despite extensive research into TBS, its diverse and complex research directions present challenges for newcomers and researchers in the field. Bibliometrics, an interdisciplinary field that applies mathematical and statistical methods to analyze written communication, can provide valuable insights into the quantitative aspects of literature, including publication volume, citation impact, and spatial distribution. This method reveals the development status and trends within a field, helping to identify academic frontiers, hotspots, and evolving research themes (14). Bibliometric analysis has widespread applications in academic research, discipline development, scientific evaluation, and information services (15, 16). However, to date, no comprehensive bibliometric analysis has been conducted specifically on TBS research. The systematic knowledge structure, evolutionary paths, and research hotspots in this field remain underexplored. This study aims to fill this gap by using bibliometric methods to analyze TBS-related publications from the Web of Science Core Collection (WoSCC) over the past 20 years. Our goal is to visually present the research framework, identify key trends, and explore the evolving hotspots in TBS research, thereby offering valuable insights for future investigations in this rapidly developing field.
Given that the Web of Science Core Collection (WoSCC) and Scopus are widely recognized as the leading bibliometric databases, other databases that do not provide co-citation data significantly limit the scope and depth of bibliometric analyses (17). Although Scopus is a comprehensive resource, it includes a substantial number of articles without impact factors, which may introduce a degree of uncertainty regarding the reliability of the analytical results (18). To ensure a robust and systematic analysis, this study utilized the WoSCC. The WoSCC encompasses the following sub-databases: the Science Citation Index Expanded (SCI-EXPANDED), the Social Sciences Citation Index (SSCI), the Arts & Humanities Citation Index (A&HCI), the Conference Proceedings Citation Index—Science (CPCI-S), the Emerging Sources Citation Index (ESCI), the Current Chemical Reactions Index (CCR-EXPANDED), and the Index Chemicus (IC). A title-based search was conducted on June 1, 2024, using the query: TI = (“theta burst stimulation”) OR (“iTBS”) OR (“cTBS”) to identify relevant publications from January 1, 2004, to December 31, 2023. Given the relatively limited number of publications in the field of TBS prior to 2004, this timeframe was deemed appropriate to represent the current state of research in this area. The inclusion criteria were restricted to articles and reviews published in English. Following independent searches conducted by two researchers, and subsequent cross-verification, non-relevant publications—such as letters, newspapers, conference papers, and news articles—that did not meet the inclusion criteria were excluded. Duplicate records were also removed. Ultimately, a total of 858 publications were included in the analysis. Relevant documents were exported in TXT format, which included full-text records and references. These data were then imported into bibliometric analysis software for subsequent visualization. The study workflow is illustrated in Figure 1.
For the comprehensive quantitative analysis of publication volume, countries, institutions, authors, journals, references, and keywords, we employed CiteSpace (version 6.1.R3 Advanced), VOSviewer (version 1.6.20), and the R-bibliometrix package (version 4.3.2) (19) (detailed variable analysis is provided in Supplementary material S1). Prior to the analysis, several preprocessing steps were implemented to ensure data quality. These steps included the normalization of synonyms, removal of irrelevant terms, and standardization of variations in author and institutional names (specific preprocessing details are provided in Supplementary material S2). Using CiteSpace, we extracted detailed information from the data, including collaboration networks among countries and institutions, trends in disciplinary development, citation and co-citation analyses, and the identification of emerging research trends (20) (see Supplementary material S3 for detailed visual interpretations). VOSviewer facilitated the extraction and visualization of key insights from the publication data, particularly through the construction of co-occurrence networks of keywords, which revealed the structure and dynamics of scientific research (21). R-bibliometrix, an open-source tool within the R environment, generated various visual outputs, such as cooperation and trend graphs, thereby enabling the intuitive presentation of the analytical results (22). By integrating the functionalities of these tools, we produced co-occurrence, clustering, and highlighting maps that provide a multi-dimensional view of the TBS field, thereby supporting corresponding analyses.
Based on the research strategy outlined, a total of 858 publications related to TBS were retrieved from the WoSCC database for the period 2004–2023. The annual number of publications (Np), the average citations per article (ACI), and the Hirsch index (H-index) are presented in Figure 2. No related publications were recorded in 2004. From 2005 to 2009, the number of publications grew slowly and steadily, with a slight stagnation observed in 2010, followed by a rapid increase after 2018. The H-index of publications from 2004 to 2010 gradually increased, remained stable from 2010 to 2018, and declined post-2019 due to time constraints. The ACI was relatively high between 2005 and 2008, with the highest value observed in 2005.
A total of 57 countries/regions have contributed to TBS-related research. Statistics for the top 10 countries/regions, based on the number of TBS publications, are presented in Table 1. The USA (Np: 180) and China (Np: 161) are the leading contributors, followed by Germany, Canada, and other regions with fewer than 100 publications each. Notably, while the USA and China together account for nearly 40% of the publications in the TBS field, the number of citations (Nc) for the USA was 4,066, which is 2.7 times greater than that of China (Nc: 1,499). The USA also exhibited the highest betweenness centrality (Bc: 0.6), indicating its dominant influence in terms of both the quantity and quality of publications in this field. In the country co-occurrence map (Figure 3A), purple circular nodes represent countries with high Bc (≥0.1). The top five countries by Bc are the USA, the United Kingdom (UK), Germany, Australia, and Canada. Figure 3B illustrates the strong international collaboration, with the most frequent partnerships occurring between the USA and the UK, followed by collaborations between the USA and Germany.
Figure 3. The national/regional analysis of TBS-related research. (A) The co-occurrence country map of TBS research. Node size indicates co-occurrence frequency, with purple circles representing high Bc (≥0.1). (B) The network graph illustrating publication output and collaboration between countries/regions.
Figure 4A presents the co-occurrence network of major research institutions, with detailed information on the top 10 institutions by publication volume shown in Table 2. Figure 4B displays a Nightingale rose chart representing the overall publication volume. In terms of the number of publications, the University of London (UK) contributed the most (Np: 54), followed by the University of Toronto (Canada) with 46 publications and the Centre for Addiction and Mental Health (Canada) with 33 publications. In terms of Bc, the University of London, the University of California, and Harvard University (USA) ranked in the top three. Institutions with high Bc demonstrate close collaboration, indicating a strong scientific capability in TBS research.
Figure 4. The institutional analysis of TBS-related research. (A) Co-occurrence institutional map of TBS research. (B) South-Nightingale rose diagram showing institutional publication output.
Price’s Law was applied to calculate the minimum publication volume of core authors using the mathematical model , where M represents the minimum number of publications for core authors, and is the highest publication count by a single author. Analysis using CiteSpace software identified , leading to . Therefore, authors with four or more publications are classified as core authors, totaling 190 authors (4.96% of all authors). Based on CiteSpace data, a ranking table of the top 10 authors was constructed (Table 3). John C. Rothwell from the Institute of Neurology at University College London leads with 25 publications, followed by Zafiris J. Daskalakis from the University of Toronto and Ying-Zu Huang from Chang Gung University College of Medicine, each with 21 publications. In the co-occurrence network of core authors, most scholars are associated with their own research teams, demonstrating close internal cooperation but a lack of prominent high-Bc authors (Figure 5A). The trend graph of annual publication volumes of high-output authors (Figure 5B) highlights recent active authors in this field, including Zafiris J. Daskalakis, Daniel M. Blumberger, and Chris Baeken. These authors exhibit significant academic vitality, and their research outputs warrant further attention.
Figure 5. The author analysis of TBS-related research. (A) Co-occurrence authorship map of TBS research. (B) Annual publication trend of prolific authors.
During the study period, TBS-related publications appeared in 236 journals. Table 4 shows that the journal with the most publications is Brain Stimulation (Np: 54), followed by Clinical Neurophysiology (Np: 44), Frontiers in Neuroscience (Np: 34), and Frontiers in Human Neuroscience (Np: 30). Figure 6A presents a dual-map overlay of journals, visually representing journal distribution, citation patterns, and shifts in research focus. In the TBS field, journals in the categories of molecular biology/immunology and neurology/sports science/ophthalmology frequently cite articles published in journals within the fields of molecular biology/genetics. Figure 6B shows the results of grouping journals according to Bradford’s Law, with the core zone (Zone 1) comprising 10 journals, the secondary core zone (Zone 2) consisting of 37 journals, and the non-core zone (Zone 3) containing 186 journals.
Figure 6. The journal analysis of TBS-related research. (A) Double-map overlay of TBS research journals. The left side depicts citing journal clusters, while the right side depicts cited journals, with colored trajectories indicating citation relationships. (B) Grouping of academic journals based on Bradford’s Law.
Based on Table 5, the top 10 most cited publications on TBS are presented, with the top three each cited over 300 times. The most cited publication is “Theta Burst Stimulation of the Human Motor Cortex” by Huang Y. Z. et al., published in Neuron in 2005, which has been cited 2,758 times. This pivotal study introduced the definition of TBS, providing a foundation for subsequent research in the field. The second most cited work is “Effectiveness of Theta Burst versus High-Frequency Repetitive Transcranial Magnetic Stimulation in Patients with Depression (THREE-D): A Randomised Non-Inferiority Trial” by Blumberger D. M. et al., published in The Lancet in 2018, with 609 citations. The top 10 references cover a broad spectrum of topics, including neurophysiological mechanisms, clinical applications, and the optimization of TBS protocols.
In Figure 7A, studies with higher Bc predominantly focus on the application and potential of TBS in areas such as neurophysiological and pathological mechanisms, as well as neurosurgical rehabilitation. The cluster analysis of references (Figure 7B) provides an objective view of the knowledge structure in TBS research. The references are categorized into 15 clusters based on the degree of correlation between publications. The largest cluster, #0 focuses on depression, while earlier research clusters include #3 calcium-binding proteins, #8 predictive force control, #11 premotor cortex, and #14 vermis. Subsequent studies have evolved into clusters focused on #0 depression and #2 rehabilitation. In recent years, however, the connectivity between research fields has decreased, with clusters such as #6 post-traumatic stress disorder, #9 transcranial magnetic stimulation combined with electroencephalography (TMS-EEG), #10 auditory feedback, #12 mild cognitive impairment, and #13 dysphagia becoming more independent. These clusters reflect a growing focus on the extension and refinement of TBS applications in clinical disease-related syndromes.
Figure 7. The reference analysis of TBS-related research. (A) Co-citation relationship map among references. (B) Clustering of references based on similarity, including 15 main clusters such as #0 depression, #1 human, #2 rehabilitation, etc. (C) Dependency analysis between literature clusters, reflecting the evolution process among them. (D) Top 25 references with the highest citation bursts, indicated by red lines in corresponding years.
Dependency analysis of the reference clusters, conducted using CiteSpace (Figure 7C), provides a clearer understanding of the current research hotspots and the evolutionary relationships among these clusters. The clusters can be categorized into three main groups: foundational research, bridging clusters, and frontier clusters. Foundational research clusters refer to those that have evolved into other clusters, such as Cluster #11 premotor cortex, Cluster #8 predictive force control, Cluster #1 human, and Cluster #5 spatial attention. Bridging clusters, on the other hand, are those that have both evolved from other clusters and subsequently given rise to additional clusters, serving a connective role in the research process. These include Cluster #3 calcium-binding proteins, Cluster #14 vermis, Cluster #7 cortical excitability, Cluster #2 rehabilitation, and Cluster #0 depression. Among these, Cluster #0 depression stands out with the highest link strength, indicating its significant research prominence. It has evolved from Clusters #14 vermis, #11 premotor cortex, #8 predictive force control, #5 spatial attention, #3 calcium-binding proteins, and #1 human, and further evolved into Clusters #12 mild cognitive impairment, #13 dysphagia, and #10 auditory feedback. A closely related cluster, Cluster #2 rehabilitation, evolved from Clusters #1 human, #3 calcium-binding proteins, #5 spatial attention, and #7 cortical excitability, and subsequently evolved into Clusters #6 post-traumatic stress disorder, #9 TMS-EEG, and #13 dysphagia. Finally, Clusters #6 post-traumatic stress disorder, #9 TMS-EEG, #10 auditory feedback, #12 mild cognitive impairment, and #13 dysphagia have evolved from other clusters but have not yet evolved into additional clusters, suggesting that these topics likely represent the frontier areas of TBS research in recent years.
Figure 7D displays the top 25 references with citation bursts, where the burst duration exceeds 2 years, and the average burst strength is over 9 years, indicating their significant academic contribution. The strongest burst (strength = 36.31) occurred for the groundbreaking 2005 study by Huang Y. Z. et al. In the past 5 years, the study by Blumberger D. M. et al., published in The Lancet in 2018, has become highly prominent.
The keyword density map in Figure 8A highlights the primary research focal points within the TBS field. Prominent terms such as plasticity, depression, excitability, motor cortex, prefrontal cortex, efficacy, and stroke frequently appear, each occurring over 80 times with a link strength exceeding 500. These keywords are primarily associated with investigations into the neurophysiological mechanisms of TBS and its clinical applications in specific diseases and symptoms.
Figure 8. The keyword analysis of TBS-related research. (A) Co-occurrence network of keywords in TBS research. (B) Timeline view of keyword clusters.
Figure 8B presents both a cluster map and a timeline view of TBS-related keywords, illustrating the temporal distribution of prominent research topics. Cluster #0 cortical excitability stands out as the largest and most frequently cited research hotspot, having evolved into a major area of focus. Alongside Cluster #0, Clusters #1 plasticity, #2 meta-analysis, #3 dorsolateral prefrontal cortex, #4 speech motor control, #7 magnetic resonance imaging, and #10 ischemic stroke exhibit extended time spans, representing sustained areas of interest in TBS research. In contrast, clusters #5 inhibitory control, #8 intracranial electroencephalography, #9 Parkinson’s disease, and #11 activities of daily living have emerged as relatively new areas of investigation. Furthermore, certain keywords, such as cortical excitability and plasticity, have remained consistent throughout the course of research, while others, such as meta-analysis and near-infrared spectroscopy, have only gained significant attention in recent years. Overall, the evolution of these key research terms reflects an initial focus on TBS paradigms and neurophysiological mechanisms, whereas contemporary research has increasingly centered on TBS applications across various diseases and symptoms, as well as an exploration of the central mechanisms involved, particularly within the framework of multimodal diagnostic tools.
This study employs bibliometric analysis to provide a comprehensive examination of the development and key trends in TBS research, outlining two distinct phases of its progression: the exploratory phase from 2005 to 2018, and the period of rapid growth from 2018 to the present. The analysis investigates several critical metrics, including publication volume, collaboration networks, and citation frequencies, offering a clear view of the global distribution and influence of TBS research. Notably, leading countries, prominent institutions, and key authors have played a pivotal role in shaping the field. However, challenges remain in promoting international collaboration and enhancing academic diversity. Furthermore, the focus of TBS research has gradually shifted from investigating neurophysiological mechanisms to exploring its clinical applications, particularly in neurological rehabilitation and mental health disorders. This bibliometric analysis provides valuable insights into the evolution of TBS research and highlights current research hotspots and emerging trends expected to significantly impact future investigations in the field.
The analysis of annual publication and citation data in TBS research reveals two key developmental phases: the first phase (2005–2018) and the second phase (2018–present). During the first phase, the field experienced initial growth, characterized by fluctuating publication volumes. Although the average annual publication count did not exceed 20 articles between 2005 and 2008, the ACI remained notably high, peaking at 31.65 in 2005. This suggests that early publications had a significant foundational impact, laying the groundwork for subsequent research. Between 2009 and 2018, the publication volume gradually increased, and the H-index remained consistently high, signaling the field’s maturation and transition towards more systematic research. The second phase (2018–present) is marked by rapid development, with a sharp increase in annual publication volumes. Notably, 2020 saw a 25-article rise over 2019. This surge is largely attributed to the growing research output from leading countries, such as the USA and China, underscoring the increasing academic interest in TBS. This trend suggests that TBS research is poised to continue its robust growth in the foreseeable future.
This study spans 57 countries/regions. The USA maintains a significant lead in the field, with 180 publications, a total link strength of 147, and a Bc of 0.6, highlighting its core influence in TBS research. Despite ranking fifth in publication volume, the UK leads in citation count, indicating that its publications have garnered high academic quality and recognition despite lower output. China ranks second in publication volume but shows weaker performance in citation count, total link strength, and Bc, suggesting that TBS research in China is still in the early stages of development. Analysis of the co-occurrence network of countries/regions reveals the top five countries by Bc are the USA, the UK, Germany, Australia, and Canada, underscoring their pivotal roles in the TBS research collaboration network.
Among institutions, all of the top 10 have published over 20 articles. University College London leads in publication output and ranks first in Bc. Although not leading in publication volume, the University of California and Harvard University rank second and third, respectively, in Bc. This highlights their central roles in academic networking and fostering collaborations with other institutions. The co-occurrence network of institutions reveals diverse collaborative patterns among research entities across countries. While a substantial international network of cooperation exists, the majority of collaborations remain concentrated within national boundaries, suggesting the need for enhanced international academic exchanges moving forward.
Core authors have had a profound impact on TBS research, with their work frequently cited and playing a pivotal role in advancing the field. For instance, John C. Rothwell has emerged as a leading figure due to his extensive publications and critical contributions to research on motor cortex responses, which have influenced subsequent studies (23, 24). The co-occurrence map of core authors highlights distinct research teams within the TBS field, each led by prominent figures and comprising active scholars. However, collaboration between these teams remains limited, suggesting that further inter-team cooperation is essential for fostering broader development and innovation within the field. In this context, widespread collaboration between research institutions becomes particularly crucial. Such institutional partnerships can help alleviate the increasing costs associated with research infrastructure while promoting cooperation across specialized fields such as basic and clinical medicine. Additionally, these collaborations can serve as bridges, facilitating interactions among researchers and laying the foundation for new joint projects in diverse areas of research. By encouraging collaboration, institutional partnerships and joint projects can enable scientists and scholars to explore various research systems, institutions, and funding opportunities, thereby enhancing overall research capacity.
Analysis of journal data reveals that Brain Stimulation publishes the most TBS papers, followed by Clinical Neurophysiology, Frontiers in Neuroscience, and Frontiers in Human Neuroscience, which are also highly co-cited. These journals predominantly focus on neurophysiological mechanisms and diseases, which aligns with the findings from dual-map overlay analyses. This overlay method intuitively reveals journal geographic distribution, citation trajectory evolution, and shifts in research focus (25). The interdisciplinary citation patterns in TBS research demonstrate that journals in this field extend beyond their specific scopes, fostering academic exchange and knowledge integration across disciplines. Such integration plays a vital role in constructing knowledge systems and advancing scientific development. Utilizing Bradford’s Law to categorize journals by publication count identifies core journals in TBS-related fields, enhancing research efficiency and supporting the construction of a cohesive knowledge system (15).
Bibliometrics plays a pivotal role in processing and analyzing extensive datasets, providing researchers with valuable insights into emerging research trends (26). By examining shifts in frequently cited references and keywords, bibliometrics highlights key themes and facilitates a deeper understanding of the evolution within specific academic fields (27). Before delving into a detailed analysis, it is beneficial to first review the progression of TBS research from 2004 to 2023. Initially, research primarily focused on the paradigms of TBS, including preliminary investigations into its neurophysiological and pathological mechanisms. Over time, the focus expanded to include the molecular mechanisms and clinical applications of TBS in the treatment of various diseases, leading to a significant increase in related keyword and reference clusters. TBS research in disease treatment applications has largely concentrated on two key areas: (1) neurological rehabilitation: TBS has shown promise in enhancing motor and cognitive functions in post-stroke patients (28, 29), as well as in improving rehabilitation outcomes for individuals with aphasia (30), Parkinson’s disease (PD) (31), spinal cord injury (32), and swallowing disorders (33). (2) Mental disorders: TBS has demonstrated potential efficacy in treating mental disorders, including depression (34, 35), schizophrenia (36), post-traumatic stress disorder (PTSD) (37), and obsessive-compulsive disorder (OCD) (38). The sustained scholarly focus on the physiological and pathological mechanisms of TBS in the context of various diseases has significantly advanced our understanding of its underlying molecular and cellular processes, while simultaneously underscoring its considerable clinical potential.
The ongoing scholarly focus on the physiological and pathological mechanisms of TBS in various diseases has considerably advanced our understanding of its underlying molecular and cellular processes, while also underscoring its significant potential for clinical application. Early research, as reflected in reference clustering #3 calcium-binding proteins and keywords clustering #6 synaptic plasticity, primarily focused on the physiological mechanisms of TBS, providing valuable insights into its biological basis. As research evolved, these clusters gradually expanded to include disease-specific areas, such as #0 depression, #2 rehabilitation, #4 cerebellum, and #13 dysphagia. In terms of cognitive function, Wu et al. (39) found that cTBS enhances glymphatic fluid transport, particularly the exchange between cerebrospinal fluid and interstitial fluid. This process reduces amyloid-β deposition and enhances spatial memory cognition. Additionally, Sridharan et al. (40) demonstrated that TBS-induced [Ca2+]i oscillations may activate gene expression related to memory. Another study suggests that iTBS can mitigate cognitive decline in an Alzheimer’s disease mouse model by upregulating iron-sulfur cluster assembly, thus promoting mitochondrial respiration and function (41). In the context of Parkinson’s disease (PD, keywords clustering #9), iTBS has been shown to reduce dopaminergic neuron degeneration, increase dopamine levels in the substantia nigra, and produce lasting effects on motor function (42). Research on stroke rehabilitation (reference clustering #2, keywords clustering #10) reveals that iTBS confers neuroprotection in ischemic stroke by reducing infarct volume and potentially suppressing neuronal apoptosis through miR-34c-5p regulation of the p53/Bax signaling pathway (43). Wu et al. (44) further demonstrated that cTBS treatment reduces the number of Iba-1-positive microglia and GFAP-positive astrocytes, modulating microglial polarization to reduce infarct volume. Additionally, iTBS may protect against motor deficits and neuronal damage caused by stroke by inhibiting the TLR4/NF-κB/NLRP3 signaling pathway, thereby regulating the M1/M2 phenotype balance in microglia (45). Studies focusing on magnetic resonance imaging in spinal cord injury (reference clustering #7) suggest that iTBS significantly increases serotoninergic nerve fibers at the injury site and promotes the growth of descending propriospinal fibers below the injury site. This suggests early neuroprotective potential and regenerative effects related to descending motor pathways (46). Furthermore, research on TBS gene polymorphisms has indicated that individual factors, such as gender, significantly influence the efferent properties of iTBS on neurogenesis. For example, iTBS increases the size of mossy fiber terminals forming synapses on CA3 pyramidal neurons in male mice (47). Additionally, individuals with the Val66Met genotype show more pronounced post-effects changes following cTBS compared to those with the Val66Val genotype (48). In conclusion, the physiological and pathological studies on TBS have revealed its multifaceted roles in regulating neural plasticity, improving cognitive function, promoting neural regeneration, and treating neurodegenerative diseases. These studies, through various molecular and cellular mechanisms, offer new strategies and insights for understanding and treating neuro-related diseases.
Research in reference cluster #2 rehabilitation and keyword cluster #10 ischemic stroke has demonstrated the potential of TBS in enhancing motor function, cognitive function, and unilateral spatial neglect in post-stroke patients. Meng et al. (49) found that a combined treatment regimen of 1 Hz rTMS and iTBS enhances motor function in subacute stroke patients more effectively than 1 Hz rTMS alone. Additionally, ipsilesional cTBS has been shown to improve rehabilitation outcomes in patients with chronic post-stroke sequelae (50). In a comparison of iTBS and rTMS for motor function rehabilitation post-stroke, Huang (51) reported that while both methods were effective, iTBS significantly boosted rehabilitation efficiency. Systematic reviews and meta-analyses further support iTBS’s potential to enhance motor and daily functions in stroke patients (4, 52–54). In terms of addressing cognitive impairment, Tsai et al. (55) demonstrated that iTBS improves global cognition, attention, and memory functions in patients with post-stroke cognitive impairment. For speech motor control in post-stroke patients, Szaflarski et al. (56) highlighted the therapeutic potential of iTBS in aphasia by stimulating the ipsilesional hemisphere. This view is supported by Zheng et al. (30), who found that cTBS modulates brain activity and connectivity, leading to enhanced language abilities in post-stroke patients. Meta-analytic findings also suggest positive effects of cTBS and iTBS on unilateral spatial neglect in post-stroke patients (57). The evolution of research in reference cluster #2 indicates a gradual expansion toward #13 dysphagia and #9 TMS-EEG, suggesting a future research focus on post-stroke dysphagia rehabilitation and the use of multimodal approaches, such as EEG and functional near-infrared spectroscopy, to validate TBS mechanisms.
Beyond stroke, TBS applications have also gained attention in spinal cord injury, aphasia, and PD rehabilitation. Fassett et al. (58) demonstrated that iTBS induces short-term neuroplastic changes in corticospinal output in spinal cord injury patients, while Feng et al. (32) showed that combining iTBS with physical therapy enhances lower limb motor recovery. Gharooni et al. (59) confirmed the safety and feasibility of iTBS for upper limb sensorimotor dysfunction in post-spinal cord injury patients. In aphasia treatment, Szaflarski et al. (60) observed combined therapy efficacy, and Zheng et al. (30) highlighted the potential of cTBS to enhance language abilities. In PD, Rashid-Lopez et al. (61) validated the benefits of iTBS on motor symptoms, while Degardin et al. (62) reported iTBS’s effectiveness in reducing motor slowness. Additionally, continuous cTBS has been shown to alleviate levodopa-induced dyskinesia (63). Overall, TBS shows considerable therapeutic promise across these conditions, warranting ongoing exploration, though current research interest has not yet reached the level of stroke treatment.
TBS reference clustering #0 identifies depression as the largest cluster. The cluster evolution diagram reveals a progression from various research groups, eventually transitioning into cutting-edge studies, underscoring its pivotal role in current research trends. Depression, a prevalent and severe mental health disorder, has long been a central focus of mental health treatment. Research suggests that TBS offers promising potential for alleviating depressive symptoms. Both cTBS and iTBS have demonstrated beneficial effects on mood, cognitive functions, and specific symptoms in patients with depression (64–67). Within the depression-related keyword cluster, research on the design of TBS targets and their combination with other treatments remains a primary area of exploration. For example, some studies propose that iTBS, when combined with D-cycloserine, may enhance clinical response and remission rates in patients with major depressive disorder (68). Another study suggests that, despite the limited sample size and number of studies, both cTBS and iTBS show preliminary efficacy in treating treatment-resistant depression and depressive episodes in bipolar disorder (69). Furthermore, bilateral burst TMS has been shown to significantly reduce depressive symptoms and may also improve brain responses associated with emotion processing (70). These findings provide compelling scientific evidence supporting the effectiveness and safety of TBS as a treatment for depression and offer guidance for future clinical applications and large-scale studies on TBS in depression treatment.
Examining the references and keyword clusters concerning the application of TBS in psychiatric disorders beyond depression, research has primarily focused on schizophrenia, PTSD, and OCD. In schizophrenia, Tyagi et al. (71) demonstrated that cTBS might alleviate auditory hallucinations by modulating cortical excitability, while iTBS shows promise in reducing negative symptoms, particularly when applied to the cerebellar vermis. For PTSD, iTBS has been shown to be as effective as traditional 10 Hz rTMS, and as a short-term treatment, it significantly improves core PTSD symptoms (72). Additionally, a case study suggests that TBS targeting the bilateral dorsolateral prefrontal cortex may offer significant improvements in severe PTSD symptoms, particularly when these symptoms co-occur with depression (73). These findings introduce new strategies for the clinical treatment of PTSD and emphasize the necessity for further research to explore the long-term effects and optimal application protocols of TBS in PTSD treatment. In OCD, cTBS targeting the bilateral supplementary motor area has been shown to markedly improve clinical symptoms (74). Moreover, cTBS stimulation of the orbitofrontal cortex demonstrates good safety and tolerability, with significant improvements in anxiety symptoms and overall severity (75). As the clinical application of TBS expands, research in these areas is likely to become a prominent future trend.
Reference clusters #4 cerebellum and #14 vermis provide an overview of studies investigating the cerebellum as a potential target for TBS. The cerebellum, a crucial constituent of the central nervous system, plays an indispensable role not only in the regulation of motor control but also in the mediation of cognitive and emotional processes (33). Halko et al. (76) demonstrated that TBS applied to specific cerebellar areas, including the lateral crus I/II and vermal lobule VII, modulates brain networks, such as the default mode network and the dorsal attention system, thereby underscoring the cerebellum’s pivotal role in regulating large-scale neural circuits. Furthermore, cerebellar TBS has shown promising results in improving gait and balance in patients with multiple sclerosis (77), enhancing visuomotor learning in stroke survivors (29), alleviating negative symptoms in schizophrenia (36), boosting upper-limb sensory-motor function following spinal cord injury (59), and reducing dyskinesia in Parkinson’s disease (63). Within the broader context of TBS research, these findings align with prior studies focusing on other brain regions, such as the motor cortex (M1) and dorsolateral prefrontal cortex (DLPFC), while also expanding the scope of research in these domains. Early meta-analyses and systematic reviews have underscored the therapeutic efficacy of TBS in these regions for a variety of conditions, including motor rehabilitation, cognitive enhancement, and emotional regulation (4, 78, 79). For instance, TBS targeting M1 has been extensively investigated for its potential to improve motor function following stroke (80). Similarly, TBS applied to the DLPFC has shown promise in the treatment of depression, with evidence suggesting that it modulates DLPFC activity to improve both emotional and cognitive functions (81). These studies indicate that, despite targeting different brain regions, TBS exerts its therapeutic effects by modulating specific neural networks and pathways. However, the cerebellum, often overshadowed by the motor cortex and prefrontal cortex in TBS research, offers distinct advantages due to its dual role in both motor and non-motor processes. Thus, the exploration of cerebellar TBS not only complements existing studies on M1 and DLPFC, but also opens novel avenues for therapeutic innovation, underscoring the urgent need for further investigation into the cerebellum’s role in brain network modulation and its potential for treatment.
This study has several limitations. First, all data were sourced exclusively from the WoSCC. While the WoSCC covers a broad spectrum of scholarly publications, it is possible that some relevant studies were omitted from the analysis. Second, the variability in the quality of the articles included in the dataset may affect the reliability of the results. Furthermore, the study predominantly focused on English-language papers and reviews, which introduces the potential for language bias and quality discrepancies, potentially undermining the robustness of the analysis. Lastly, the bibliometric analysis software used in this study has inherent limitations. Specifically, the extraction and clustering of terms from titles, abstracts, and keywords may introduce variability, and there is no guarantee that terms with similar meanings will be grouped consistently.
In conclusion, global research on TBS continues to progress rapidly, with the USA emerging as a significant contributor to the field. Among influential journals, Brain Stimulation has established itself as a key publication in this domain. Prominent researchers such as Huang Y. Z. and John C. Rothwell have made substantial contributions to TBS studies. Current research is particularly focused on the clinical applications of TBS in neurorehabilitation and depression, as well as investigations into the underlying mechanisms. These areas are expected to remain central to future research efforts. Future trends may increasingly explore TBS applications for conditions such as dysphagia, cognitive impairments, and PTSD. Notably, TBS cerebellar stimulation has emerged as a promising therapeutic approach for addressing psychiatric and cognitive issues. This bibliometric analysis provides an objective overview of the TBS field, offering valuable insights to scholars tracking the evolution of the knowledge base and research directions in this area.
The original contributions presented in the study are included in the article/Supplementary material, further inquiries can be directed to the corresponding authors.
MiL: Conceptualization, Data curation, Formal analysis, Investigation, Resources, Validation, Visualization, Writing – original draft, Writing – review & editing. SJ: Conceptualization, Formal analysis, Methodology, Writing – original draft, Writing – review & editing. MeL: Formal analysis, Methodology, Resources, Writing – original draft, Writing – review & editing. BY: Formal analysis, Investigation, Writing – review & editing. QW: Formal analysis, Writing – review & editing. CF: Investigation, Writing – review & editing. ZL: Supervision, Validation, Writing – review & editing. LW: Methodology, Supervision, Writing – review & editing.
The author(s) declare that financial support was received for the research, authorship, and/or publication of this article. We thank the Henan Provincial Science and Technology Major Project (No. 221100310200) and Henan Province Medical Science and Technology Research Project (No. SBGJ202002092) for funding this research.
The authors thank all editors and reviewers for their participation and contributions.
The authors declare that the research was conducted in the absence of any commercial or financial relationships that could be construed as a potential conflict of interest.
All claims expressed in this article are solely those of the authors and do not necessarily represent those of their affiliated organizations, or those of the publisher, the editors and the reviewers. Any product that may be evaluated in this article, or claim that may be made by its manufacturer, is not guaranteed or endorsed by the publisher.
The Supplementary material for this article can be found online at: https://www.frontiersin.org/articles/10.3389/fneur.2024.1469877/full#supplementary-material
1. Hermiller, MS, Chen, YF, Parrish, TB, and Voss, JL. Evidence for immediate enhancement of hippocampal memory encoding by network-targeted theta-burst stimulation during concurrent fMRI. J Neurosci. (2020) 40:7155–68. doi: 10.1523/JNEUROSCI.0486-20.2020
2. Huang, YZ, Edwards, MJ, Rounis, E, Bhatia, KP, and Rothwell, JC. Theta burst stimulation of the human motor cortex. Neuron. (2005) 45:201–6. doi: 10.1016/j.neuron.2004.12.033
3. Grossheinrich, N, Rau, A, Pogarell, O, Hennig-Fast, K, Reinl, M, Karch, S, et al. Theta burst stimulation of the prefrontal cortex: safety and impact on cognition, mood, and resting electroencephalogram. Biol Psychiatry. (2009) 65:778–84. doi: 10.1016/j.biopsych.2008.10.029
4. Chen, S, Zhang, S, Yang, W, Chen, Y, Wang, B, Chen, J, et al. The effectiveness of intermittent theta burst stimulation for upper limb motor recovery after stroke: a systematic review and meta-analysis of randomized controlled trials. Front Neurosci. (2023) 17:1272003. doi: 10.3389/fnins.2023.1272003
5. He, Y, Liu, M-G, Gong, K-R, and Chen, J. Differential effects of long and short train theta burst stimulation on LTP induction in rat anterior cingulate cortex slices: multi-electrode array recordings. Neurosci Bull. (2009) 25:309–18. doi: 10.1007/s12264-009-0831-5
6. Cao, G, and Harris, KM. Augmenting saturated LTP by broadly spaced episodes of theta-burst stimulation in hippocampal area CA1 of adult rats and mice. J Neurophysiol. (2014) 112:1916–24. doi: 10.1152/jn.00297.2014
7. Quarta, E, Fulgenzi, G, Bravi, R, Cohen, EJ, Yanpallewar, S, Tessarollo, L, et al. Deletion of the endogenous TrkB.T1 receptor isoform restores the number of hippocampal CA1 parvalbumin-positive neurons and rescues long-term potentiation in pre-symptomatic mSOD1(G93A) ALS mice. Mol Cell Neurosci. (2018) 89:33–41. doi: 10.1016/j.mcn.2018.03.010
8. Bulteau, S, Sebille, V, Fayet, G, Thomas-Ollivier, V, Deschamps, T, Bonnin-Rivalland, A, et al. Efficacy of intermittent theta burst stimulation (iTBS) and 10-Hz high-frequency repetitive transcranial magnetic stimulation (rTMS) in treatment-resistant unipolar depression: study protocol for a randomised controlled trial. Trials. (2017) 18:17. doi: 10.1186/s13063-016-1764-8
9. Lindsey, A, Ellison, RL, Herrold, AA, Aaronson, AL, Kletzel, SL, Stika, MM, et al. rTMS/iTBS and cognitive rehabilitation for deficits associated with TBI and PTSD: a theoretical framework and review. J Neuropsychiatr Clin Neurosci. (2023) 35:28–38. doi: 10.1176/appi.neuropsych.21090227
10. Rachid, F. Safety and efficacy of theta-burst stimulation in the treatment of psychiatric disorders: a review of the literature. J Nerv Ment Dis. (2017) 205:823–39. doi: 10.1097/NMD.0000000000000742
11. Lowe, CJ, Manocchio, F, Safati, AB, and Hall, PA. The effects of theta burst stimulation (TBS) targeting the prefrontal cortex on executive functioning: a systematic review and meta-analysis. Neuropsychologia. (2018) 111:344–59. doi: 10.1016/j.neuropsychologia.2018.02.004
12. Pabst, A, Proksch, S, Mede, B, Comstock, DC, Ross, JM, and Balasubramaniam, R. A systematic review and meta-analysis of the efficacy of intermittent theta burst stimulation (iTBS) on cognitive enhancement. Neurosci Biobehav Rev. (2022) 135:104587. doi: 10.1016/j.neubiorev.2022.104587
13. Lan, X-J, Yang, X-H, Qin, Z-J, Cai, D-B, Liu, Q-M, Mai, J-X, et al. Efficacy and safety of intermittent theta burst stimulation versus high-frequency repetitive transcranial magnetic stimulation for patients with treatment-resistant depression: a systematic review. Front Psychiatry. (2023) 14:1244289. doi: 10.3389/fpsyt.2023.1244289
14. Donthu, N, Kumar, S, Mukherjee, D, Pandey, N, and Lim, WM. How to conduct a bibliometric analysis: an overview and guidelines. J Bus Res. (2021) 133:285–96. doi: 10.1016/j.jbusres.2021.04.070
15. Cobo, MJ, Martinez, MA, Gutierrez-Salcedo, M, Fujita, H, and Herrera-Viedma, E. 25 years at knowledge-based systems: a bibliometric analysis. Knowl-Based Syst. (2015) 80:3–13. doi: 10.1016/j.knosys.2014.12.035
16. Ellegaard, O, and Wallin, JA. The bibliometric analysis of scholarly production: how great is the impact? Scientometrics. (2015) 105:1809–31. doi: 10.1007/s11192-015-1645-z
17. Echchakoui, S. Why and how to merge Scopus and Web of Science during bibliometric analysis: the case of sales force literature from 1912 to 2019. J Market Anal. (2020) 8:165–84. doi: 10.1057/s41270-020-00081-9
18. AlRyalat, SAS, Malkawi, LW, and Momani, SM. Comparing bibliometric analysis using PubMed, Scopus, and Web of Science Databases. J Vis Exp. (2019):e58494. doi: 10.3791/58494
19. Cortese, S, Sabe, M, Chen, C, Perroud, N, and Solmi, M. Half a century of research on attention-deficit/hyperactivity disorder: a scientometric study. Neurosci Biobehav Rev. (2022) 140:104769. doi: 10.1016/j.neubiorev.2022.104769
20. Chen, C, Dubin, R, and Kim, MC. Emerging trends and new developments in regenerative medicine: a scientometric update (2000–2014). Expert Opin Biol Ther. (2014) 14:1295–317. doi: 10.1517/14712598.2014.920813
21. Solmi, M, Chen, C, Daure, C, Buot, A, Ljuslin, M, Verroust, V, et al. A century of research on psychedelics: a scientometric analysis on trends and knowledge maps of hallucinogens, entactogens, entheogens and dissociative drugs. Eur Neuropsychopharmacol. (2022) 64:44–60. doi: 10.1016/j.euroneuro.2022.09.004
22. Moral-Munoz, JA, Herrera-Viedma, E, Santisteban-Espejo, A, and Cobo, MJ. Software tools for conducting bibliometric analysis in science: an up-to-date review. Prof Inferm. (2020) 29:e290103. doi: 10.3145/epi.2020.ene.03
23. Monte-Silva, K, Ruge, D, Teo, JT, Paulus, W, Rothwell, JC, and Nitsche, MA. D2 receptor block abolishes theta burst stimulation-induced neuroplasticity in the human motor cortex. Neuropsychopharmacology. (2011) 36:2097–102. doi: 10.1038/npp.2011.100
24. Ma, K, Rothwell, JC, and Goetz, SM. A revised calcium-dependent model of transcranial magnetic theta-burst stimulation. Clin Neurophysiol. (2022) 144:41–9. doi: 10.1016/j.clinph.2022.09.009
25. Kim, MC, and Chen, C. A scientometric review of emerging trends and new developments in recommendation systems. Scientometrics. (2015) 104:239–63. doi: 10.1007/s11192-015-1595-5
26. Yan, L, and Wang, Z. Mapping the literature on academic publishing: a bibliometric analysis on WOS. SAGE Open. (2023) 13:21582440231158562. doi: 10.1177/21582440231158562
27. Wu, H, Cheng, K, Guo, Q, Yang, W, Tong, L, Wang, Y, et al. Mapping knowledge structure and themes trends of osteoporosis in rheumatoid arthritis: a bibliometric analysis. Front Med. (2021) 8:787228. doi: 10.3389/fmed.2021.787228
28. Baker, TE, Lin, M-H, Gueth, M, Biernacki, K, and Parikh, S. Beyond the motor cortex: theta burst stimulation of the anterior midcingulate cortex. Biol Psychiatry Cogn Neurosci Neuroimaging. (2020) 5:1052–60. doi: 10.1016/j.bpsc.2020.06.009
29. Bonni, S, Motta, C, Pellicciari, MC, Casula, EP, Cinnera, AM, Maiella, M, et al. Intermittent cerebellar theta burst stimulation improves visuo-motor learning in stroke patients: a pilot study. Cerebellum. (2020) 19:739–43. doi: 10.1007/s12311-020-01146-2
30. Zheng, K, Xu, X, Ji, Y, Fang, H, Gao, F, Huang, G, et al. Continuous theta burst stimulation-induced suppression of the right fronto-thalamic-cerebellar circuit accompanies improvement in language performance in poststroke aphasia: a resting-state fMRI study. Front Aging Neurosci. (2023) 14:1079023. doi: 10.3389/fnagi.2022.1079023
31. Pastore-Wapp, M, Kaufmann, BC, Nyffeler, T, Wapp, S, Bohlhalter, S, and Vanbellingen, T. Feasibility of a combined intermittent theta-burst stimulation and video game-based dexterity training in Parkinson’s disease. J Neuroeng Rehabil. (2023) 20:2. doi: 10.1186/s12984-023-01123-w
32. Feng, X, Wang, T, Jiang, Y, Liu, Y, Yang, H, Duan, Z, et al. Cerebral theta-burst stimulation combined with physiotherapy in patients with incomplete spinal cord injury: a pilot randomized controlled trial. J Rehabil Med. (2023) 55:jrm00375. doi: 10.2340/jrm.v55.4375
33. Wang, B, Sun, H, Pan, X, Ma, W, Dong, L, Wang, Q, et al. The effects of intermittent theta burst stimulation of the unilateral cerebellar hemisphere on swallowing-related brain regions in healthy subjects. Front Hum Neurosci. (2023) 17:1100320. doi: 10.3389/fnhum.2023.1100320
34. Wathra, RA, Mulsant, BH, Daskalakis, ZJ, Downar, J, McClintock, SM, Nestor, SM, et al. Effect of prior pharmacotherapy on remission with sequential bilateral theta-burst versus standard bilateral repetitive transcranial magnetic stimulation in treatment-resistant late-life depression. Br J Psychiatry. (2023) 223:504–6. doi: 10.1192/bjp.2023.81
35. Blumberger, DM, Mulsant, BH, Thorpe, KE, McClintock, SM, Konstantinou, GN, Lee, HH, et al. Effectiveness of standard sequential bilateral repetitive transcranial magnetic stimulation vs bilateral theta burst stimulation in older adults with depression the FOUR-D randomized noninferiority clinical trial. JAMA Psychiatry. (2022) 79:1065–73. doi: 10.1001/jamapsychiatry.2022.2862
36. Basavaraju, R, Ithal, D, Thanki, MV, Ramalingaiah, AH, Thirthalli, J, Reddy, RP, et al. Intermittent theta burst stimulation of cerebellar vermis enhances fronto-cerebellar resting state functional connectivity in schizophrenia with predominant negative symptoms: a randomized controlled trial. Schizophr Res. (2021) 238:108–20. doi: 10.1016/j.schres.2021.10.005
37. Nursey, J, Sbisa, A, Knight, H, Ralph, N, Cowlishaw, S, Forbes, D, et al. Exploring theta burst stimulation for post-traumatic stress disorder in Australian veterans-a pilot study. Milit Med. (2020) 185:E1770–8. doi: 10.1093/milmed/usaa149
38. Harika-Germaneau, G, Rachid, F, Chatard, A, Lafay-Chebassier, C, Solinas, M, Thirioux, B, et al. Continuous theta burst stimulation over the supplementary motor area in refractory obsessive-compulsive disorder treatment: a randomized sham-controlled trial. Brain Stimul. (2019) 12:1565–71. doi: 10.1016/j.brs.2019.07.019
39. Wu, C, Lin, T, Ding, Q, Zhang, N, Ou, Z, Cai, G, et al. Continuous theta-burst stimulation promotes paravascular CSF-interstitial fluid exchange through regulation of aquaporin-4 polarization in APP/PS1 mice. Mediat Inflamm. (2022) 2022:2140524–12. doi: 10.1155/2022/2140524
40. Sheridan, GK, Moeendarbary, E, Pickering, M, O’Connor, JJ, and Murphy, KJ. Theta-burst stimulation of hippocampal slices induces network-level calcium oscillations and activates analogous gene transcription to spatial learning. PLoS One. (2014) 9:e100546. doi: 10.1371/journal.pone.0100546
41. Zhu, Y, Huang, H, Chen, Z, Tao, Y, Liao, L-Y, Gao, S-H, et al. Intermittent theta burst stimulation attenuates cognitive deficits and Alzheimer’s disease-type pathologies via ISCA1-mediated mitochondrial modulation in APP/PS1 mice. Neurosci Bull. (2024) 40:182–200. doi: 10.1007/s12264-023-01098-7
42. Jovanovic, MZ, Stanojevic, J, Stevanovic, I, Stekic, A, Bolland, SJ, Jasnic, N, et al. Intermittent theta burst stimulation improves motor and behavioral dysfunction through modulation of NMDA receptor subunit composition in experimental model of Parkinson’s disease. Cells. (2023) 12:1525. doi: 10.3390/cells12111525
43. Hu, S, Wang, X, Yang, X, Ouyang, S, Pan, X, Fu, Y, et al. Long-term iTBS improves neural functional recovery by reducing the inflammatory response and inhibiting neuronal apoptosis via miR-34c-5p/p53/Bax signaling pathway in cerebral ischemic rats. Neuroscience. (2023) 527:37–51. doi: 10.1016/j.neuroscience.2023.07.014
44. Wu, C, Li, M, Feng, Y, He, X, Li, W, Liang, F, et al. Continuous theta burst stimulation provides neuroprotection by accelerating local cerebral blood flow and inhibiting inflammation in a mouse model of acute ischemic stroke. Brain Res. (2020) 1726:146488. doi: 10.1016/j.brainres.2019.146488
45. Luo, L, Liu, M, Fan, Y, Zhang, J, Liu, L, Li, Y, et al. Intermittent theta-burst stimulation improves motor function by inhibiting neuronal pyroptosis and regulating microglial polarization via TLR4/NFκB/NLRP3 signaling pathway in cerebral ischemic mice. J Neuroinflamm. (2022) 19:141. doi: 10.1186/s12974-022-02501-2
46. Liu, J-L, Wang, S, Chen, Z-H, Wu, R-J, Yu, H-Y, Yang, S-B, et al. The therapeutic mechanism of transcranial iTBS on nerve regeneration and functional recovery in rats with complete spinal cord transection. Front Immunol. (2023) 14:1153516. doi: 10.3389/fimmu.2023.1153516
47. Zhang, TR, Askari, B, Kesici, A, Guilherme, E, Vila-Rodriguez, F, and Snyder, JS. Intermittent theta burst transcranial magnetic stimulation induces hippocampal mossy fibre plasticity in male but not female mice. Eur J Neurosci. (2023) 57:310–23. doi: 10.1111/ejn.15891
48. Harvey, DY, DeLoretta, L, Shah-Basak, PP, Wurzman, R, Sacchetti, D, Ahmed, A, et al. Variability in cTBS aftereffects attributed to the interaction of stimulus intensity with BDNF Val66Met polymorphism. Front Hum Neurosci. (2021) 15:585533. doi: 10.3389/fnhum.2021.585533
49. Meng, Y, Zhang, D, Hai, H, Zhao, Y-Y, and Ma, Y-W. Efficacy of coupling intermittent theta-burst stimulation and 1 Hz repetitive transcranial magnetic stimulation to enhance upper limb motor recovery in subacute stroke patients: a randomized controlled trial. Restor Neurol Neurosci. (2020) 38:109–18. doi: 10.3233/RNN-190953
50. Di Lazzaro, V, Rothwell, JC, Talelli, P, Capone, F, Ranieri, F, Wallace, AC, et al. Inhibitory theta burst stimulation of affected hemisphere in chronic stroke: a proof of principle, sham-controlled study. Neurosci Lett. (2013) 553:148–52. doi: 10.1016/j.neulet.2013.08.013
51. Huang, Y-Z. The modulation of cortical motor circuits and spinal reflexes using theta burst stimulation in healthy and dystonic subjects. Restor Neurol Neurosci. (2010) 28:449–57. doi: 10.3233/RNN-2010-0554
52. Talelli, P, Wallace, A, Dileone, M, Hoad, D, Cheeran, B, Oliver, R, et al. Theta burst stimulation in the rehabilitation of the upper limb: a semirandomized, placebo-controlled trial in chronic stroke patients. Neurorehabil Neural Repair. (2012) 26:976–87. doi: 10.1177/1545968312437940
53. Khan, F, and Chevidikunnan, F. Theta burst stimulation a new paradigm of non-invasive brain stimulation for post-stroke upper limb motor rehabilitation. Turk J Phys Med Rehabil. (2017) 63:193–6. doi: 10.5606/tftrd.2017.417
54. Huang, W, Chen, J, Zheng, Y, Zhang, J, Li, X, Su, L, et al. The effectiveness of intermittent theta burst stimulation for stroke patients with upper limb impairments: a systematic review and meta-analysis. Front Neurol. (2022) 13:896651. doi: 10.3389/fneur.2022.896651
55. Tsai, P-Y, Lin, W-S, Tsai, K-T, Kuo, C-Y, and Lin, P-H. High-frequency versus theta burst transcranial magnetic stimulation for the treatment of poststroke cognitive impairment in humans. J Psychiatry Neurosci. (2020) 45:262–70. doi: 10.1503/jpn.190060
56. Szaflarski, JP, Nenert, R, Allendorfer, JB, Martin, AN, Amara, AW, Griffis, JC, et al. Intermittent theta burst stimulation TBS for treatment of chronic post-stroke aphasia: results of a pilot randomized, double-blind, sham-controlled trial. Med Sci Monitor. (2021) 27:e931468. doi: 10.12659/MSM.931468
57. Cotoi, A, Mirkowski, M, Iruthayarajah, J, Anderson, R, and Teasell, R. The effect of theta-burst stimulation on unilateral spatial neglect following stroke: a systematic review. Clin Rehabil. (2019) 33:183–94. doi: 10.1177/0269215518804018
58. Fassett, HJ, Turco, CV, El-Sayes, J, Lulic, T, Baker, S, Richardson, B, et al. Transcranial magnetic stimulation with intermittent theta burst stimulation alters corticospinal output in patients with chronic incomplete spinal cord injury. Front Neurol. (2017) 8:380. doi: 10.3389/fneur.2017.00380
59. Gharooni, A-A, Nair, KPS, Hawkins, D, Scivill, I, Hind, D, and Hariharan, R. Intermittent theta-burst stimulation for upper-limb dysfunction and spasticity in spinal cord injury: a single-blind randomized feasibility study. Spinal Cord. (2018) 56:762–8. doi: 10.1038/s41393-018-0152-5
60. Szaflarski, JP, Griffis, J, Vannest, J, Allendorfer, JB, Nenert, R, Amara, AW, et al. A feasibility study of combined intermittent theta burst stimulation and modified constraint-induced aphasia therapy in chronic post-stroke aphasia. Restor Neurol Neurosci. (2018) 36:503–18. doi: 10.3233/RNN-180812
61. Rashid-Lopez, R, Macias-Garcia, P, Sanchez-Fernandez, FL, Cano-Cano, F, Sarrias-Arrabal, E, Sanmartino, F, et al. Neuroimaging and serum biomarkers of neurodegeneration and neuroplasticity in Parkinson’s disease patients treated by intermittent theta-burst stimulation over the bilateral primary motor area: a randomized, double-blind, sham-controlled, crossover trial study. Front Aging Neurosci. (2023) 15:1258315. doi: 10.3389/fnagi.2023.1258315
62. Degardin, A, Devos, D, Defebvre, L, Destee, A, Plomhause, L, Derambure, P, et al. Effect of intermittent theta-burst stimulation on akinesia and sensorimotor integration in patients with Parkinson’s disease. Eur J Neurosci. (2012) 36:2669–78. doi: 10.1111/j.1460-9568.2012.08158.x
63. Brusa, L, Ceravolo, R, Kiferle, L, Monteleone, F, Iani, C, Schillaci, O, et al. Metabolic changes induced by theta burst stimulation of the cerebellum in dyskinetic Parkinson’s disease patients. Parkinsonism Relat Disord. (2012) 18:59–62. doi: 10.1016/j.parkreldis.2011.08.019
64. Blumberger, DM, Vila-Rodriguez, F, Thorpe, KE, Feffer, K, Noda, Y, Giacobbe, P, et al. Effectiveness of theta burst versus high-frequency repetitive transcranial magnetic stimulation in patients with depression (THREE-D): a randomised non-inferiority trial. Lancet. (2018) 391:1683–92. doi: 10.1016/S0140-6736(18)30295-2
65. Li, C-T, Chen, M-H, Juan, C-H, Liu, R-S, Lin, W-C, Bai, Y-M, et al. Effects of prefrontal theta-burst stimulation on brain function in treatment-resistant depression: a randomized sham-controlled neuroimaging study. Brain Stimul. (2018) 11:1054–62. doi: 10.1016/j.brs.2018.04.014
66. Lee, JC, Corlier, J, Wilson, AC, Tadayonnejad, R, Marder, KG, Ngo, D, et al. Subthreshold stimulation intensity is associated with greater clinical efficacy of intermittent theta-burst stimulation priming for major depressive disorder. Brain Stimul. (2021) 14:1015–21. doi: 10.1016/j.brs.2021.06.008
67. Huang, C-C, Liang, C-S, Chu, H-T, Chang, H-A, and Yeh, T-C. Intermittent theta burst stimulation for the treatment of autonomic dysfunction and depressive symptoms in dementia with Lewy bodies. Asian J Psychiatr. (2022) 75:103212. doi: 10.1016/j.ajp.2022.103212
68. Wrightson, JG, Cole, J, Sohn, MN, and McGirr, A. The effects of D-cycloserine on corticospinal excitability after repeated spaced intermittent theta-burst transcranial magnetic stimulation: a randomized controlled trial in healthy individuals. Neuropsychopharmacology. (2023) 48:1217–24. doi: 10.1038/s41386-023-01575-7
69. Cai, D-B, Qin, Z-J, Lan, X-J, Liu, Q-M, Qin, X-D, Wang, J-J, et al. Accelerated intermittent theta burst stimulation for major depressive disorder or bipolar depression: a systematic review and meta-analysis. Asian J Psychiatr. (2023) 85:103618. doi: 10.1016/j.ajp.2023.103618
70. Chou, P-H, Tu, C-H, Chen, C-M, Lu, M-K, Tsai, C-H, Hsieh, W-T, et al. Bilateral theta-burst stimulation on emotional processing in major depressive disorder: a functional neuroimaging study from a randomized, double-blind, sham-controlled trial. Psychiatry Clin Neurosci. (2023) 77:233–40. doi: 10.1111/pcn.13524
71. Tyagi, P, Dhyani, M, Khattri, S, Tejan, V, Tikka, SK, and Garg, S. Efficacy of intensive bilateral temporo-parietal continuous theta-burst stimulation for auditory VErbal hallucinations (TPC-SAVE) in schizophrenia: a randomized sham-controlled trial? Asian J Psychiatr. (2022) 74:103176. doi: 10.1016/j.ajp.2022.103176
72. Yuan, H, Liu, B, Li, F, Jin, Y, Zheng, S, Ma, Z, et al. Effects of intermittent theta-burst transcranial magnetic stimulation on post-traumatic stress disorder symptoms: a randomized controlled trial. Psychiatry Res. (2023) 329:115533. doi: 10.1016/j.psychres.2023.115533
73. Chang, C-H, Liu, C-Y, Chen, S-J, and Tsai, H-C. Accelerated theta-burst transcranial magnetic stimulation of the bilateral dorsolateral prefrontal cortex in a patient with post-traumatic stress disorder and major depressive disorder: case report. Front Psych. (2023) 14:1061088. doi: 10.3389/fpsyt.2023.1061088
74. Guo, Q, Wang, K, Han, H, Li, P, Cheng, J, Zhu, J, et al. Continuous theta burst stimulation over the bilateral supplementary motor area in obsessive-compulsive disorder treatment: a clinical randomized single-blind sham-controlled trial. Eur Psychiatry. (2022) 65:e64. doi: 10.1192/j.eurpsy.2022.2323
75. Dutta, P, Dhyani, M, Garg, S, Tikka, SK, Khattri, S, Mehta, S, et al. Efficacy of intensive orbitofrontal continuous theta burst stimulation (iOFcTBS) in obsessive compulsive disorder: a randomized placebo controlled study. Psychiatry Res. (2021) 298:113784. doi: 10.1016/j.psychres.2021.113784
76. Halko, MA, Farzan, F, Eldaief, MC, Schmahmann, JD, and Pascual-Leone, A. Intermittent theta-burst stimulation of the lateral cerebellum increases functional connectivity of the default network. J Neurosci. (2014) 34:12049–56. doi: 10.1523/JNEUROSCI.1776-14.2014
77. Tramontano, M, Grasso, MG, Soldi, S, Casula, EP, Bonni, S, Mastrogiacomo, S, et al. Cerebellar intermittent theta-burst stimulation combined with vestibular rehabilitation improves gait and balance in patients with multiple sclerosis: a preliminary double-blind randomized controlled trial. Cerebellum. (2020) 19:897–901. doi: 10.1007/s12311-020-01166-y
78. Kishi, T, Ikuta, T, Sakuma, K, Hatano, M, Matsuda, Y, Wilkening, J, et al. Theta burst stimulation for depression: a systematic review and network and pairwise meta-analysis. Mol Psychiatry. (2024) 29:3893–9. doi: 10.1038/s41380-024-02630-5
79. Ticini, LF, Dolk, T, Waszak, F, and Schütz-Bosbach, S. IPL-M1 interaction shapes pre-reflective social differentiation in the human action system: new insights from TBS and TMS combined. Sci Rep. (2018) 8:12001. doi: 10.1038/s41598-018-30480-z
80. Platz, T, Adler-Wiebe, M, Roschka, S, and Lotze, M. Enhancement of motor learning by focal intermittent theta burst stimulation (iTBS) of either the primary motor (M1) or somatosensory area (S1) in healthy human subjects. Restor Neurol Neurosci. (2018) 36:117–30. doi: 10.3233/RNN-170774
Keywords: theta burst stimulation, bibliometric analysis, hotspots and trends, VOSviewer, CiteSpace
Citation: Liu M, Jin S, Liu M, Yang B, Wang Q, Fan C, Li Z and Wu L (2024) Global research hotspots and trends of theta burst stimulation from 2004 to 2023: a bibliometric analysis. Front. Neurol. 15:1469877. doi: 10.3389/fneur.2024.1469877
Received: 24 July 2024; Accepted: 29 November 2024;
Published: 10 December 2024.
Edited by:
Kosuke Oku, Kawasaki University of Medical Welfare, JapanReviewed by:
Ying Shen, The First Affiliated Hospital of Nanjing Medical University, ChinaCopyright © 2024 Liu, Jin, Liu, Yang, Wang, Fan, Li and Wu. This is an open-access article distributed under the terms of the Creative Commons Attribution License (CC BY). The use, distribution or reproduction in other forums is permitted, provided the original author(s) and the copyright owner(s) are credited and that the original publication in this journal is cited, in accordance with accepted academic practice. No use, distribution or reproduction is permitted which does not comply with these terms.
*Correspondence: Zhe Li, emhlbGkxOTc0QDE2My5jb20=; Liang Wu, MTk3Mnd1bGlhbmdAc2luYS5jb20=
†These authors have contributed equally to this work and share first authorship
Disclaimer: All claims expressed in this article are solely those of the authors and do not necessarily represent those of their affiliated organizations, or those of the publisher, the editors and the reviewers. Any product that may be evaluated in this article or claim that may be made by its manufacturer is not guaranteed or endorsed by the publisher.
Research integrity at Frontiers
Learn more about the work of our research integrity team to safeguard the quality of each article we publish.