- 1Department of Physical Therapy and Human Movement Sciences, Northwestern University, Chicago, IL, United States
- 2USF Health Morsani College of Medicine, University of South Florida, Tampa, FL, United States
- 3Department of Medical Social Sciences, Northwestern University Feinberg School of Medicine, Chicago, IL, United States
- 4Creighton University School of Medicine, Creighton University, Omaha, NE, United States
- 5Department of Neurology, Rutgers-Robert Wood Johnson Medical School, New Brunswick, NJ, United States
- 6Department of Neurology, Northwestern University Feinberg School of Medicine, Chicago, IL, United States
- 7Department of Neurosurgery, Rush University Medical Center, Chicago, IL, United States
- 8Department of Neurological Surgery, Northwestern University Feinberg School of Medicine, Chicago, IL, United States
- 9Department of Kinesiology and Nutrition, University of Illinois at Chicago, Chicago, IL, United States
Introduction: The long-term effects of surgery for subthalamic nucleus deep brain stimulation (STN-DBS) on cognitive aspects of motor control for people with Parkinson’s disease (PD) are largely unknown. We compared saccade latency and reach reaction time (RT) pre- and post-surgery while participants with PD were off-treatment.
Methods: In this preliminary study, we assessed people with PD approximately 1 month pre-surgery while OFF medication (OFF-MEDS) and about 8 months post-surgery while OFF medication and STN-DBS treatment (OFF-MEDS/OFF-DBS). We examined saccade latency and reach reaction time (RT) performance during a visually-guided reaching task requiring participants to look at and reach toward a visual target.
Results: We found that both saccade latency and reach RT significantly increased post-surgery compared to pre-surgery. In addition, there was no significant change in Movement Disorder Society-Unified Parkinson’s Disease Rating Scale (MDS-UPDRS) Part III score.
Discussion: We found detrimental post-surgical changes to saccade latency and reach RT. We discuss the potential contributions of long-term tissue changes and withdrawal from STN-DBS on this detrimental cognitive effect.
1 Introduction
Following surgery for subthalamic nucleus deep brain stimulation (STN-DBS), people with Parkinson’s disease (PD) frequently experience a beneficial acute microlesion effect (1–11). A microlesion occurs after electrode insertion, resulting from damage to the cells within the penetrated nucleus. The acute microlesion effect is an improvement in PD motor signs, typically measured as a decrease in OFF treatment Unified Parkinson’s Disease Rating Scale (UPDRS) Part III score. However, the beneficial acute microlesion effect on PD motor signs declines in the months following surgery (1, 7–9).
Much less is known about the effects of electrode insertion on the cognitive aspects of movement, such as latency and reaction time (RT), which both reflect attention and processing speed (12–14). Interestingly, Antoniades et al. (15) found significantly increased or worsened visually-guided saccade latency 24 h post-surgery compared to pre-surgery, even while UPDRS Part III scores were improved in the same participants. While Antoniades et al. (15) showed that the detrimental effect on saccade latency declined after 3–4 weeks while ON medication before stimulation initiation, it is unknown how saccade latency is affected while OFF treatment after months post-surgery. Evaluation of the long-term effects post-surgery is important to determine if the acute detrimental effects persist. In this preliminary study, we aimed to determine if there were detrimental long-term pre- to post-surgical changes of both saccade latency and reach RT while participants were OFF treatment. While no studies have reported post-surgical changes in RT of a reach, it is important to determine if the effect of surgery on cognitive aspects of movement can be identified across effectors. A small cohort of people with PD performed a visually-guided reaching task about 1 month pre-surgery while OFF medication (OFF-MEDS) and about 8 months post-surgery while OFF medication and STN-DBS treatment (OFF-MEDS/OFF-DBS). We examined the change in saccade latency and reach RT post-surgery compared to pre-surgery.
2 Methods
Northwestern University and Rush University Medical Center Institutional Review Boards approved this study, and all experiments were completed in accord with the Helsinki Declaration of 1975. We obtained informed consent from all participants. Nine participants with PD completed OFF-MEDS testing about 1 month before undergoing STN-DBS surgery and OFF-MEDS/OFF-DBS testing on average 8 months after surgery (range = 6–10 months, average = 7.89 months) (Table 1). All participants with PD had a good response to bilateral STN-DBS, as evidenced by an average of 66% decrease in OFF-MEDS/ON-DBS Movement Disorder Society-UPDRS (MDS-UPDRS) Part III score compared to OFF MEDS/OFF-DBS.
A detailed description of inclusion criteria, data collection, data processing, and statistics can be found in our previous publication (16). In brief, all antiparkinson medications were withdrawn overnight for at least 12-h (17), and bilateral STN-DBS was washed-out for at least 3-h before testing (18). We collected antiparkinson medication information to calculate the levodopa equivalent daily dose (LEDD), administered the Montreal Cognitive Assessment (MoCA) while participants were ON-MEDS or ON-MEDS/ON-DBS, and administered the MDS-UPDRS Part III while participants were OFF-MEDS or OFF-MEDS/OFF-DBS. The MoCA was completed ON treatment because it was administered on a separate intake day. We compared pre- to post-surgical averages in these measures using two-tailed paired t-tests. Additionally, we recorded eye movements (Eyelink II, SR Research Ltd) and upper limb movements (Optotrak 3020, Northern Digital) during a visually-guided reaching task. The task involved participants fixating on a central cue (2000–3000 ms) and then looking at and reaching to a peripheral rightward target after a 200 ms gap (Figure 1). We calculated our primary outcome measures, saccade latency and reach RT, as the time difference between target onset and movement onset of the eyes and upper limb, respectively, as identified by a custom MATLAB code (The MathWorks Inc).
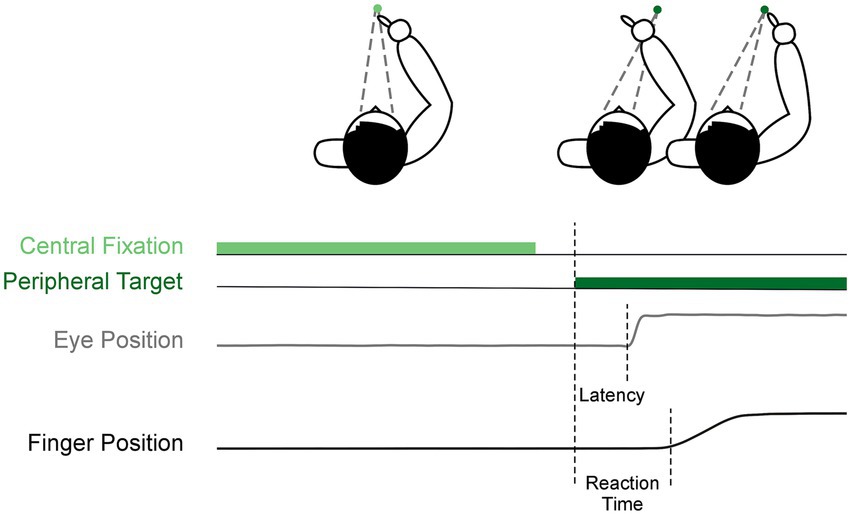
Figure 1. Visually-guided reach task. Each trial began with central fixation (light green), then after a 200 ms gap, a peripheral target appeared to the right (dark green). This was the cue for participants to saccade and reach to the target as shown by the example eye position (gray) and finger position (black).
Pre- to post-surgical differences were assessed using linear mixed-effect regression models with the fixed effect being time (pre vs. post) and the random effect being participant to account for the dependence between trials within participant. Latency and RT were log transformed because the data was right skewed, and we present the estimated difference transformed back to the original scale (Est diffBT). Back-transformation was performed on the pre- and post-surgery estimated means and then the difference in means was calculated. To determine the influence of change in disease severity on latency and RT, change in MDS-UPDRS Part III was included as a covariate. However, this change score had no significant effect and did not improve the model. Therefore, it was not included in the final models presented.
3 Results
MDS-UPDRS Part III scores were not significantly different between post-surgery (OFF-MEDS/OFF-DBS) and pre-surgery (OFF-MEDS) (Figure 2A; Table 2). Similarly, MoCA scores were not significantly different between post-surgery (ON-MEDS/ON-DBS) and pre-surgery (ON-MEDS) (Table 2). Finally, levodopa equivalent daily dose was significantly decreased by 69% post-surgery compared to pre-surgery (Table 2).
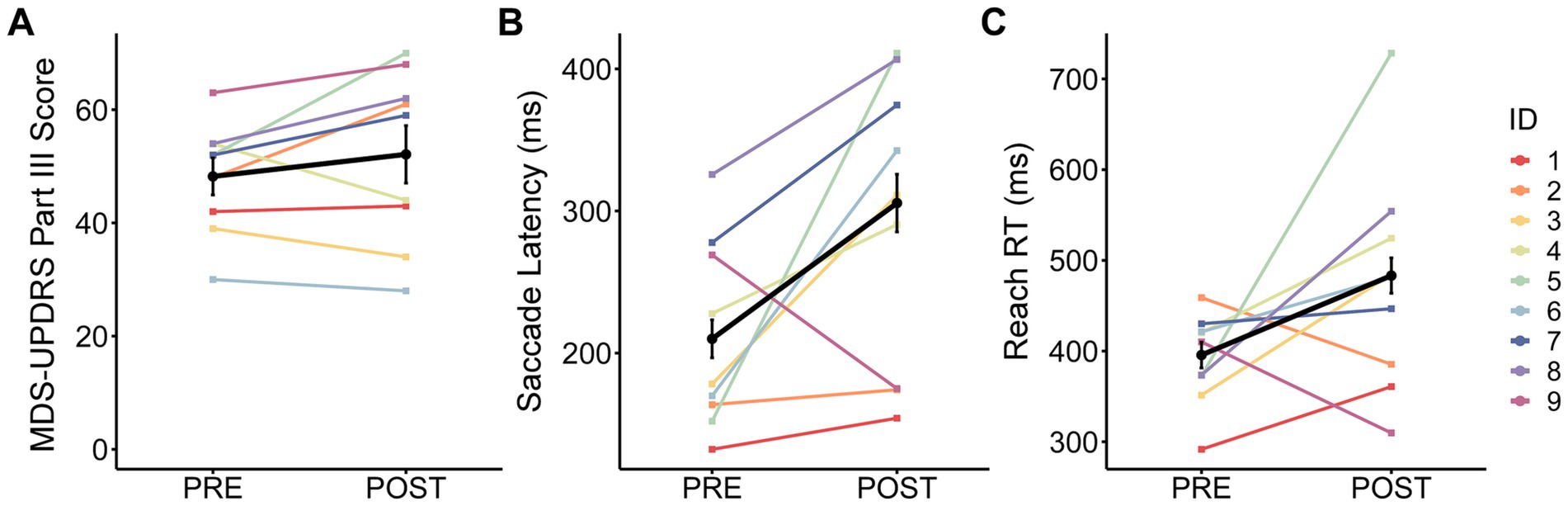
Figure 2. Change from pre- to post-surgery. The observed change in group mean ± standard error (black line and error bars) and individual means (color lines for each ID) from pre- to post-surgery for (A) Movement Disorder Society-Unified Parkinson’s Disease Rating Scale (MDS-UPDRS) Part III score, (B) saccade latency, and (C) reach reaction time (RT).
Saccade latency was significantly increased by 38% post-surgery (OFF-MEDS/OFF-DBS) compared to pre-surgery (OFF-MEDS) (Est diffBT = 72.15 ms; Figure 2B; Table 2). Additionally, reach RT was significantly increased by 20% post-surgery (OFF-MEDS/OFF-DBS) compared to pre-surgery (OFF-MEDS) (Est diffBT = 76.72 ms; Figure 2C; Table 2).
4 Discussion
We found significant detrimental effects on the cognitive aspects of movement, i.e., saccade latency and reach RT, when participants were tested 8 months post-surgery compared to pre-surgery. Not only were these effects statistically significant, but also the magnitude of the change is much larger than our previously reported effects of medication and STN-DBS on these same measures (16). Meanwhile, there was no difference between pre- and post-surgical OFF treatment MDS-UPDRS Part III scores or ON treatment MoCA scores and an expected decrease in post-surgical LEDD. We will discuss (1) four potential factors that could impact the post-surgical change in saccade latency and reach RT, (2) the lack of change in our clinical scores, and (3) how these results compare to the effect of stimulation.
4.1 Long-term tissue changes due to implantation
One potential factor is the long-term tissue changes due to lead implantation. While the acute microlesion in the STN benefits motor performance, the lead could also impact neural function in the tissue it passes through to impair cognitive performance. On a cellular level, animal and human studies have shown long-term tissue changes due to implantation, including sustained gliosis around the implant (19). This long-term gliosis can alter the neuronal function in the vicinity of the implant, the connectivity within associated circuits, and the volume and metabolic activity of connected regions (19). These tissue changes can impact many different brain areas. At our surgical centers, the lead typically passes through the middle frontal gyrus, frontal white matter, reticular thalamus, internal capsule, thalamic fasciculus and lenticular fasciculus, zona incerta, and STN. Several studies have hypothesized that the electrode trajectory through frontal regions could negatively affect cognitive functions (20, 21). Similarly, studies have shown that the location of the electrode cortical entry point is related to post-surgical verbal fluency decline (22, 23). Changes to frontal activity could impact saccade latency and reach RT directly or through its connections with the parietal cortex, which is associated with attention and sensory-motor transformations (24, 25). Additionally, the lead implantation could intersect and damage white matter tracts that carry motor and oculomotor information (26), although the exact tracts that are damaged are still being explored (27). For example, the internal capsule contains projections leading to the superior colliculus that can affect saccade latency (28) and the longitudinal fasciculus contains projections associated with spatial coordination and attention, which can impact both saccade and reach performance (29). Finally, damage to the zona incerta could also impact saccade latency because it sends projections to the superior colliculus and receives input from frontal and parietal eye fields (30). We have previously shown that turning on STN-DBS decreases saccade latency compared to OFF-DBS in this same task (16). However, STN-DBS does not significantly improve reach RT (16), suggesting that long-term tissue changes could still affect performance even with stimulation.
4.2 Electrophysiological changes after withdrawal of chronic STN-DBS
Another potential factor is the electrophysiological neural change after withdrawal of chronic STN-DBS. For instance, increased alpha band power has been shown after 1-h withdrawal of chronic STN-DBS at 3 years compared to initial programming (31). Alpha power has been related to attention (31) and increased alpha power could contribute to or reflect greater attentional deficits, leading to increased saccade latency and reach RT. There is also some evidence that chronic stimulation could induce changes in brain volume (32, 33) and functional network organization (34), but no conclusions can yet be drawn (35). Network changes due to chronic STN-DBS could explain the difference between our findings and those of Antoniades et al. (15). While Antoniades et al. (15) reported a return to pre-surgery saccade latency values within 3–4 weeks before stimulation was initiated, we show a substantial prolongation of latency at 6–10 months after withdrawal from chronic STN-DBS.
4.3 Reduction in medication
Another potential factor is the reduction in medication post-surgery due to STN-DBS. One hallmark benefit of STN-DBS is that daily medication dosage can be reduced (36). We demonstrated that LEDD was significantly reduced post-surgery, such that medication dosage was reduced, on average, by 69% in our sample. This reduction indicates that our participants had a beneficial and typical response to STN-DBS itself.
If the reduction of medication was an important underlying factor, we would expect that reducing medication would result in a decrease in latency. This is because we have previously reported that medication prolongs saccade latency compared to being OFF medication (16, 37). However, we observe the opposite relationship: a post-surgical reduction in medication accompanied by a prolongation of saccade latency. Thus, it is unlikely that the post-surgical reduction in medication dosage accounts for our findings.
4.4 Disease progression
Finally, another potential factor is disease progression. In PD, disease progression is typically characterized by a change in UPDRS Part III score. While disease progression is not linear and changes with disease stage, a 2.4 point increase is expected per year on average early in the disease (38). In our sample, change in MDS-UPDRS Part III score was not a significant covariate and, when included in our linear mixed model, had no effect on the significance of the pre- to post-surgical differences in saccade latency or reach RT. This suggests that disease progression did not significantly affect our results.
The effect of disease progression on visually-guided saccades or reaching has not been studied. However, a preliminary study reported that disease progression over 12 months did not change antisaccade latency in people with PD without STN-DBS (39). Therefore, it is unlikely that the observed increase in latency and RT is solely due to disease progression considering the magnitude of change.
4.5 Lack of change in MDS-UPDRS III and MoCA scores
We found that there was no change in OFF treatment MDS-UPDRS Part III or ON treatment MoCA scores post- vs. pre-surgery, demonstrating that our saccade and reach findings cannot be explained by a global deterioration of motor or cognitive performance. Although it should be noted that since MoCA scores were collected ON treatment, it is possible that deterioration of general cognitive performance could be detected OFF treatment and this should be considered in future studies. Previous reports on UPDRS Part III similarly demonstrated that there was no change between pre- and 1–48 months post-surgery (7, 9, 40). In addition, recent studies have shown that there was no change in MoCA score between pre- and 6–12 months post-surgery (41, 42). Thus, our data agrees with previous literature that there are limited changes in average motor severity or general cognitive function in the months post-surgery. This signifies the importance of using sensitive and quantitative behavioral measures, such as saccade latency and reach RT, to elucidate the behavioral changes after surgery.
4.6 Comparison with the beneficial effects of STN-DBS
Previous studies reported that bilateral STN-DBS decreased saccade latency in a saccade only task (43–45) and decreased reach RT in a simple RT task (45–47) compared to OFF stimulation. Similarly, our previous study using the same visually-guided reach task found that bilateral STN-DBS significantly decreased saccade latency, but only non-significantly decreased reach RT compared to OFF stimulation (16). This is important to note because STN-DBS reduced latency and RT toward healthy control levels. Therefore, while our current findings show an OFF treatment post-surgical detriment to saccade latency and reach RT, turning on STN-DBS will likely counteract this detriment. Although it should be considered that long-term tissue changes could still affect performance even with stimulation, as bilateral STN-DBS did not significantly improve reach RT in our previous study (16).
As individuals with STN-DBS are typically being stimulated, the detriment we present here should not impact daily function. However, our results indicate that there are significant behavioral changes due to implantation itself, withdrawal of chronic stimulation, or both, suggesting that long-term neurological changes are occurring. These long-term changes are important to understand in the instance that a patient discontinues STN-DBS temporarily or permanently, or that STN-DBS efficacy declines. Understanding the OFF treatment behavioral changes also highlights which circuits are being affected by implantation and stimulation.
4.7 Limitations
There are several limitations of this preliminary study. First, we had a small sample size with data from only 9 individuals. However, previous studies have used a similar sample size to demonstrate the difference between pre- and post-surgery (10, 15, 48). Second, there is a high variability between participants in task performance. However, the majority of individuals have increased saccade latency (8 out of 9) and reach RT (7 out of 9) post-surgery, so the direction of change was relatively consistent in our sample. Third, there is high variability in our participant demographics and clinical scores, but this is representative of the natural variability of those individuals who opt for STN-DBS. While there was a high variability in individual change in MDS-UPDRS Part III, this variability has been reflected in previous studies who have compared UPDRS Part III pre- and months post-surgery (7, 9, 40). We also confirmed that every participant had a beneficial response to bilateral STN-DBS, as shown by a decrease in MDS-UPDRS Part III score when ON stimulation (Table 1). Each of these limitations emphasizes the need for these findings to be replicated in a larger sample.
5 Conclusion
Our preliminary findings suggest that there are large increases in saccade latency and reach RT after STN-DBS surgery while OFF-MEDS/OFF-DBS compared to pre-surgery when people with PD are OFF-MEDS. We suggest that this detrimental effect on a cognitive aspect of movement could be due to long-term tissue changes after lead implantation and/or neural changes after the withdrawal of chronic STN-DBS.
Data availability statement
The raw data supporting the conclusions of this article will be made available by the authors, without undue reservation.
Ethics statement
The studies involving humans were approved by the Institutional Review Boards of Northwestern University and Rush University Medical Center. The studies were conducted in accordance with the local legislation and institutional requirements. The participants provided their written informed consent to participate in this study.
Author contributions
MM: Conceptualization, Data curation, Formal analysis, Investigation, Methodology, Project administration, Software, Validation, Visualization, Writing – original draft, Writing – review & editing. RA: Investigation, Writing – review & editing. YR: Investigation, Project administration, Writing – review & editing. QD: Investigation, Writing – review & editing. GP: Resources, Writing – review & editing. LV: Resources, Writing – review & editing. SS: Resources, Writing – review & editing. JR: Resources, Writing – review & editing. LG: Conceptualization, Investigation, Methodology, Supervision, Writing – review & editing. DC: Conceptualization, Funding acquisition, Methodology, Supervision, Writing – review & editing. FD: Conceptualization, Data curation, Investigation, Methodology, Project administration, Software, Supervision, Validation, Writing – review & editing.
Funding
The author(s) declare that financial support was received for the research, authorship, and/or publication of this article. This study was supported by the National Institutes of Health [R01 NS09295001 (DMC), F31 NS12069501 (MJM), T32 HD07418 (MJM)].
Acknowledgments
We would like to acknowledge the people with Parkinson’s disease who volunteered to participate in this study.
Conflict of interest
The authors declare that the research was conducted in the absence of any commercial or financial relationships that could be construed as a potential conflict of interest.
The author(s) declared that they were an editorial board member of Frontiers, at the time of submission. This had no impact on the peer review process and the final decision.
Publisher’s note
All claims expressed in this article are solely those of the authors and do not necessarily represent those of their affiliated organizations, or those of the publisher, the editors and the reviewers. Any product that may be evaluated in this article, or claim that may be made by its manufacturer, is not guaranteed or endorsed by the publisher.
References
1. Jech, R, Mueller, K, Urgošík, D, Sieger, T, Holiga, Š, Růžička, F, et al. The subthalamic microlesion story in Parkinson’s disease: electrode insertion-related motor improvement with relative cortico-subcortical hypoactivation in fMRI. PLoS One. (2012) 7:e49056. doi: 10.1371/journal.pone.0049056
2. Aygun, D, Dere, UA, Yildiz, O, Temel, Y, and Kocabicak, E. Characterizing the intraoperative microelectrode recording-induced microlesion effect on motor symptoms in patients with Parkinson’s disease undergoing deep brain stimulation of the subthalamic nucleus. Turk Neurosurg. (2019) 29:430–3. doi: 10.5137/1019-5149.JTN.24348-18.3
3. Koop, MM, Andrzejewski, A, Hill, BC, Heit, G, and Bronte-Stewart, HM. Improvement in a quantitative measure of bradykinesia after microelectrode recording in patients with Parkinson’s disease during deep brain stimulation surgery. Mov Disord. (2006) 21:673–8. doi: 10.1002/mds.20796
4. Lange, SF, Kremer, NI, van Laar, T, Lange, F, Steendam-Oldekamp, TE, Oterdoom, DLM, et al. The intraoperative microlesion effect positively correlates with the short-term clinical effect of deep brain stimulation in Parkinson’s disease. Neuromodulation. (2021) 26:459–65. doi: 10.1111/ner.13523
5. Mann, JM, Foote, KD, Garvan, CW, Fernandez, HH, Jacobson, CE, Rodriguez, RL, et al. Brain penetration effects of microelectrodes and DBS leads in STN or GPi. J Neurol Neurosurg Psychiatry. (2009) 80:794–8. doi: 10.1136/jnnp.2008.159558
6. Derrey, S, Lefaucheur, R, Chastan, N, Gérardin, E, Hannequin, D, Desbordes, M, et al. Alleviation of off-period dystonia in Parkinson disease by a microlesion following subthalamic implantation. J Neurosurg. (2010) 112:1263–6. doi: 10.3171/2009.10.JNS091032
7. Holiga, Š, Mueller, K, Möller, HE, Urgošík, D, Růžička, E, Schroeter, ML, et al. Resting-state functional magnetic resonance imaging of the subthalamic microlesion and stimulation effects in Parkinson’s disease: indications of a principal role of the brainstem. Neuroimage Clin. (2015) 9:264–74. doi: 10.1016/j.nicl.2015.08.008
8. Luo, B, Lu, Y, Qiu, C, Dong, W, Xue, C, Liu, D, et al. Altered regional homogeneity and functional connectivity during microlesion period after deep brain stimulation in Parkinson’s disease. Parkinsons Dis. (2021) 2021:1–10. doi: 10.1155/2021/2711365
9. Luo, B, Lu, Y, Qiu, C, Dong, W, Xue, C, Zhang, L, et al. Altered spontaneous neural activity and functional connectivity in Parkinson’s disease with subthalamic microlesion. Front Neurosci. (2021) 15:699010. doi: 10.3389/fnins.2021.699010
10. Singh, A, Kammermeier, S, Mehrkens, JH, and Bötzel, K. Movement kinematic after deep brain stimulation associated microlesions. J Neurol Neurosurg Psychiatry. (2012) 83:1022–6. doi: 10.1136/jnnp-2012-302309
11. Tykocki, T, Nauman, P, Koziara, H, and Mandat, T. Microlesion effect as a predictor of the effectiveness of subthalamic deep brain stimulation for Parkinson’s disease. Stereotact Funct Neurosurg. (2013) 91:12–7. doi: 10.1159/000342161
12. Jordan, N, Sagar, HJ, and Cooper, JA. Cognitive components of reaction time in Parkinson’s disease. J Neurol Neurosurg Psychiatry. (1992) 55:658–64. doi: 10.1136/jnnp.55.8.658
13. Cooper, JA, Sagar, HJ, Tidswell, P, and Jordan, N. Slowed central processing in simple and go/no-go reaction time tasks in Parkinson’s disease. Brain. (1994) 117:517–29. doi: 10.1093/brain/117.3.517
14. Hutton, SB. Cognitive control of saccadic eye movements. Brain Cogn. (2008) 68:327–40. doi: 10.1016/j.bandc.2008.08.021
15. Antoniades, CA, Buttery, P, FitzGerald, JJ, Barker, RA, Carpenter, RHS, and Watts, C. Deep brain stimulation: eye movements reveal anomalous effects of electrode placement and stimulation. PLoS One. (2012) 7:e32830. doi: 10.1371/journal.pone.0032830
16. Munoz, MJ, Arora, R, Rivera, YM, Drane, QH, Pal, GD, Verhagen Metman, L, et al. Medication only improves limb movements while deep brain stimulation improves eye and limb movements during visually-guided reaching in Parkinson’s disease. Front Human Neurosci. (2023) 17:1224611. doi: 10.3389/fnhum.2023.1224611
17. Langston, JW, Widner, H, Goetz, CG, Brooks, D, Fahn, S, Freeman, T, et al. Core assessment program for intracerebral transplantations (CAPIT). Mov Disord. (1992) 7:2–13. doi: 10.1002/mds.870070103
18. Temperli, P, Ghika, J, Villemure, JG, Burkhard, PR, Bogousslavsky, J, and Vingerhoets, FJG. How do parkinsonian signs return after discontinuation of subthalamic DBS? Neurology. (2003) 60:78–81. doi: 10.1212/WNL.60.1.78
19. Hamani, C, Davidson, B, Lipsman, N, Abrahao, A, Nestor, SM, Rabin, JS, et al. Insertional effect following electrode implantation: an underreported but important phenomenon. Brain Commun. (2024) 6:fcae093. doi: 10.1093/braincomms/fcae093
20. Borden, A, Wallon, D, Lefaucheur, R, Derrey, S, Fetter, D, Verin, M, et al. Does early verbal fluency decline after STN implantation predict long-term cognitive outcome after STN-DBS in Parkinson’s disease? J Neurol Sci. (2014) 346:299–302. doi: 10.1016/j.jns.2014.07.063
21. Aiello, M, Eleopra, R, Lettieri, C, Mondani, M, D’Auria, S, Belgrado, E, et al. Emotion recognition in Parkinson’s disease after subthalamic deep brain stimulation: differential effects of microlesion and STN stimulation. Cortex. (2014) 51:35–45. doi: 10.1016/j.cortex.2013.11.003
22. Le Goff, F, Derrey, S, Lefaucheur, R, Borden, A, Fetter, D, Jan, M, et al. Decline in verbal fluency after subthalamic nucleus deep brain stimulation in Parkinson’s disease: a microlesion effect of the electrode trajectory? J Parkinsons Dis. (2015) 5:95–104. doi: 10.3233/JPD-140443
23. Askari, A, Greif, TR, Lam, J, Maher, AC, Persad, CC, and Patil, PG. Decline of verbal fluency with lateral superior frontal gyrus penetration in subthalamic nucleus deep brain stimulation for Parkinson disease. J Neurosurg. (2022) 137:729–34. doi: 10.3171/2021.11.JNS211528
24. McDowell, JE, Dyckman, KA, Austin, BP, and Clementz, BA. Neurophysiology and neuroanatomy of reflexive and volitional saccades: evidence from studies of humans. Brain Cogn. (2008) 68:255–70. doi: 10.1016/j.bandc.2008.08.016
25. Rizzo, JR, Hosseini, M, Wong, EA, Mackey, WE, Fung, JK, Ahdoot, E, et al. The intersection between ocular and manual motor control: eye-hand coordination in acquired brain injury. Front Neurol. (2017) 8:227. doi: 10.3389/fneur.2017.00227
26. Granziera, C, Pollo, C, Russmann, H, Staedler, C, Ghika, J, Villemure, JG, et al. Sub-acute delayed failure of subthalamic DBS in Parkinson’s disease: the role of micro-lesion effect. Parkinsonism Relat Disord. (2008) 14:109–13. doi: 10.1016/j.parkreldis.2007.06.013
27. Costentin, G, Derrey, S, Gérardin, E, Cruypeninck, Y, Pressat-Laffouilhere, T, Anouar, Y, et al. White matter tracts lesions and decline of verbal fluency after deep brain stimulation in Parkinson’s disease. Hum Brain Mapp. (2019) 40:2561–70. doi: 10.1002/hbm.24544
28. Terao, Y, Fukuda, H, and Hikosaka, O. What do eye movements tell us about patients with neurological disorders? - an introduction to saccade recording in the clinical setting. Proc Jpn Acad Ser B Phys Biol Sci. (2017) 93:772–801. doi: 10.2183/pjab.93.049
29. Janelle, F, Iorio-Morin, C, D’amour, S, and Fortin, D. Superior longitudinal fasciculus: a review of the anatomical descriptions with functional correlates. Front Neurol. (2022) 13:794618. doi: 10.3389/fneur.2022.794618
30. Arena, G, Londei, F, Ceccarelli, F, Ferrucci, L, Borra, E, and Genovesio, A. Disentangling the identity of the zona incerta: a review of the known connections and latest implications. Ageing Res Rev. (2024) 93:102140. doi: 10.1016/j.arr.2023.102140
31. Anderson, RW, Wilkins, KB, Parker, JE, Petrucci, MN, Kehnemouyi, Y, Neuville, RS, et al. Lack of progression of beta dynamics after long-term subthalamic neurostimulation. Ann Clin Transl Neurol. (2021) 8:2110–20. doi: 10.1002/acn3.51463
32. Sankar, T, Li, SX, Obuchi, T, Fasano, A, Cohn, M, Hodaie, M, et al. Structural brain changes following subthalamic nucleus deep brain stimulation in Parkinson’s disease. Mov Disord. (2016) 31:1423–5. doi: 10.1002/mds.26707
33. Kern, DS, Uy, D, Rhoades, R, Ojemann, S, Abosch, A, and Thompson, JA. Discrete changes in brain volume after deep brain stimulation in patients with Parkinson’s disease. J Neurol Neurosurg Psychiatry. (2020) 91:928–37. doi: 10.1136/jnnp-2019-322688
34. Ge, J, Wang, M, Lin, W, Wu, P, Guan, Y, Zhang, H, et al. Metabolic network as an objective biomarker in monitoring deep brain stimulation for Parkinson’s disease: a longitudinal study. EJNMMI Res. (2020) 10:131. doi: 10.1186/s13550-020-00722-1
35. Kricheldorff, J, Göke, K, Kiebs, M, Kasten, FH, Herrmann, CS, Witt, K, et al. Evidence of Neuroplastic changes after transcranial magnetic, electric, and deep brain stimulation. Brain Sci. (2022) 12:929. doi: 10.3390/brainsci12070929
36. Limousin, P, Krack, P, Pollak, P, Benazzouz, A, Ardouin, C, Hoffmann, D, et al. Electrical stimulation of the subthalamic nucleus in advanced Parkinson’s disease. N Engl J Med. (1998) 339:1105–11. doi: 10.1056/NEJM199810153391603
37. Munoz, MJ, Reilly, JL, Pal, GD, Verhagen Metman, L, Rivera, YM, Drane, QH, et al. Medication adversely impacts visually-guided eye movements in Parkinson’s disease. Clin Neurophysiol. (2022) 143:145–53. doi: 10.1016/j.clinph.2022.07.505
38. Holden, SK, Finseth, T, Sillau, SH, and Berman, BD. Progression of MDS-UPDRS scores over five years in De novo Parkinson disease from the Parkinson’s progression markers initiative cohort. Mov Disord Clin Pract. (2018) 5:47–53. doi: 10.1002/mdc3.12553
39. Waldthaler, J, Stock, L, Sommerkorn, J, Krüger-Zechlin, C, and Timmermann, L. Antisaccade latency is sensitive to longitudinal change of motor and cognitive symptoms in Parkinson’s disease. Mov Disord. (2021) 36:266–8. doi: 10.1002/mds.28374
40. Rodriguez-Oroz, MC, Obeso, JA, Lang, AE, Houeto, JL, Pollak, P, Rehncrona, S, et al. Bilateral deep brain stimulation in Parkinson’s disease: a multicentre study with 4 years follow-up. Brain. (2005) 128:2240–9. doi: 10.1093/brain/awh571
41. Zirone, E, Ruggiero, F, Molisso, MT, Ferrucci, R, De Sandi, A, Marfoli, A, et al. The protective role of cognitive reserve: a preliminary study on parkinsonian patients undergoing deep brain stimulation. J Clin Med. (2024) 13:4578. doi: 10.3390/jcm13154578
42. Polat, S, Erdem, M, and Çekinmez, M. Comparison of apathy and cognitive symptoms in pre- and postoperative period in deep brain stimulation surgery. Psychiatry Clin Psychopharmacol. (2023) 33:238–45. doi: 10.5152/pcp.2023.23621
43. Goelz, LC, David, FJ, Sweeney, JA, Vaillancourt, DE, Poizner, H, Metman, LV, et al. The effects of unilateral versus bilateral subthalamic nucleus deep brain stimulation on prosaccades and antisaccades in Parkinson’s disease. Exp Brain Res. (2017) 235:615–26. doi: 10.1007/s00221-016-4830-2
44. Fawcett, AP, González, EG, Moro, E, Steinbach, MJ, Lozano, AM, and Hutchison, WD. Subthalamic nucleus deep brain stimulation improves saccades in Parkinson’s disease. Neuromodulation. (2010) 13:17–25. doi: 10.1111/j.1525-1403.2009.00246.x
45. Antoniades, CA, Carpenter, RHS, and Temel, Y. Deep brain stimulation of the subthalamic nucleus in Parkinson’s disease: similar improvements in saccadic and manual responses. Neuroreport. (2012) 23:179–83. doi: 10.1097/WNR.0b013e32834f6daa
46. Brown, RG, Dowsey, PL, Brown, P, Jahanshahi, M, Pollak, P, Benabid, AL, et al. Impact of deep brain stimulation on upper limb akinesia in Parkinson’s disease. Ann Neurol. (1999) 45:473–88. doi: 10.1002/1531-8249(199904)45:4<473::AID-ANA9>3.0.CO;2-V
47. Temel, Y, Blokland, A, Ackermans, L, Boon, P, van Kranen-Mastenbroek, VHJM, Beuls, E, et al. Differential effects of subthalamic nucleus stimulation in advanced Parkinson disease on reaction time performance. Exp Brain Res. (2006) 169:389–99. doi: 10.1007/s00221-005-0151-6
Keywords: Parkinson’s disease, deep brain stimulation surgery, saccade, latency, reach, reaction time, cognitive aspects
Citation: Munoz MJ, Arora R, Rivera YM, Drane QH, Pal GD, Verhagen Metman L, Sani SB, Rosenow JM, Goelz LC, Corcos DM and David FJ (2024) Cognitive aspects of motor control deteriorate while off treatment following subthalamic nucleus deep brain stimulation surgery in Parkinson’s disease. Front. Neurol. 15:1463970. doi: 10.3389/fneur.2024.1463970
Edited by:
Thyagarajan Subramanian, The University of Toledo College of Medicine and Life Sciences, United StatesReviewed by:
Ioannis Ugo Isaias, Julius Maximilian University of Würzburg, GermanyMarina Raguz, Dubrava Clinical Hospital, Croatia
Copyright © 2024 Munoz, Arora, Rivera, Drane, Pal, Verhagen Metman, Sani, Rosenow, Goelz, Corcos and David. This is an open-access article distributed under the terms of the Creative Commons Attribution License (CC BY). The use, distribution or reproduction in other forums is permitted, provided the original author(s) and the copyright owner(s) are credited and that the original publication in this journal is cited, in accordance with accepted academic practice. No use, distribution or reproduction is permitted which does not comply with these terms.
*Correspondence: Miranda J. Munoz, bWlyYW5kYW11bm96MjAyMkB1Lm5vcnRod2VzdGVybi5lZHU=