- Department of Neurology, West China Hospital of Sichuan University, Chengdu, Sichuan, China
Background: Transcranial direct current stimulation (tDCS) has recently become a novel and non-invasive treatment option for refractory epilepsy. Previous systematic reviews have suggested that tDCS may be effective in treating epilepsy, this study presents the first meta-analysis on its effectiveness.
Methods: We searched PubMed, Embase, Cochrane Library, and Web of Science for relevant randomized controlled trials (RCTs) from database inception to May 2024. The Cochrane risk of bias tool RoB2.0 was used to assess the risk of bias. Primary outcomes included changes in seizure frequency from baseline and the proportion of patients with a ≥50% reduction in seizure frequency.
Results: Of the 608 studies initially identified, 14 were finally included. The pooled results from the random-effects model indicated that tDCS significantly reduced seizure frequency (WMD 0.41, 95% CI 0.24, 0.59). Further subgroup analysis revealed that tDCS significantly reduced seizure frequency in temporal lobe epilepsy, and seizure frequency was more alleviated in studies that had treatment sessions of fewer than 5 times, and followed up within 2 months' post-treatment. Only four studies provided data on patients with a ≥50% reduction in seizure frequency, showing no significant difference (RR 2.96, 95% CI 0.85, 10.32). In the systematic review, three studies analyzed cognitive function changes after tDCS treatment, but none reported significant improvements. The most common side effect during tDCS treatment was transient tingling, and no patients required additional life-support measures due to side effects.
Conclusion: The current meta-analysis on available trials indicates that tDCS can effectively reduce seizure frequency in the short term and is well-tolerated. However, its impact on cognitive improvement in epilepsy patients requires further investigation.
Systematic review registration: https://inplasy.com/inplasy-2024-6-0033/, identifier INPLASY202460033.
1 Introduction
Affecting 70 million people globally, epilepsy stands as the second most prevalent neurological disorder. The World Health Organization has recognized it as one of the five major neurological and psychiatric diseases targeted for prevention and treatment (1). In China, it is estimated that there are around 10 million people with epilepsy, with an annual increase of 400,000 new cases (2). The frequency of seizures and whether antiepileptic drugs are used in combination profoundly impact patients' quality of life and living costs (3).
Among epilepsy patients followed up in clinical settings, about one-third suffer from refractory epilepsy (4). Only a minority of those with focal epileptic lesions are potential candidates for surgical resection (5). The complication rate for epilepsy surgery is ~10%, with serious complications occurring in about 1.5% of cases (6), with postoperative neurological deficits a major factor contributing to patient dissatisfaction (7).
For patients with refractory epilepsy unsuitable for resective surgery, neuromodulation therapies can be implemented. Neurostimulation reduces epileptic activity by delivering electrical or magnetic stimulation to various anatomical targets. Traditional neurostimulation methods include open-loop vagus nerve stimulation (VNS), open-loop deep brain stimulation (DBS), and closed-loop responsive neurostimulation (RNS) (8). However, these invasive treatments come with risks such as intracranial hemorrhage and infection (9), and their execution requires a high level of expertise, limiting them to specialized clinical centers (10). Moreover, these treatments are costly, requiring long-term maintenance of equipment, and their cost-effectiveness is still under evaluation (11, 12).
Two predominant forms of non-invasive brain stimulation techniques are transcranial direct current stimulation (tDCS) and transcranial magnetic stimulation (TMS). Both use electromagnetic principles to non-invasively modulate cortical excitability in a targeted spatial and temporal manner. Using tDCS may modulate cortical excitability by inducing depolarization (anodal stimulation) or hyperpolarization (cathodal stimulation) of resting membrane potentials via low-intensity electrical currents conducted through scalp electrodes, thereby altering spontaneous neuronal excitability and activity (13, 71). Due to advantages such as minimal side effects, low cost, and ease of operation, and with some studies showing potential for sustained home-based therapy (14, 15). This technique has been under investigation as a promising add-on treatment for epilepsy. Fregni et al. conducted the first controlled clinical trial of cathodal tDCS for epilepsy in 2006, demonstrating that tDCS stimulation is safe and significantly reduces epileptiform discharges (16). Beyond epilepsy, tDCS has shown efficacy in chronic pain (17), cognition (18, 19), and psychiatric disorder (20, 21), suggesting potential therapeutic value for epilepsy comorbidities. Mechanistic exploration of tDCS effects continues in animal studies; in pilocarpine-induced epilepsy models, tDCS mitigates hippocampal CA1 neuronal damage, thereby improving cognitive function and potentially reducing seizure frequency and severity (22). Moreover, in pentylenetetrazol (PTZ)-induced epilepsy models, tDCS has been found to suppress post-seizure inflammation and enhance brain function (23). Additionally, it may promote long-term depression (LTD) and potentiation (LTP) for synaptic plasticity and induce dopamine release in the dorsal striatum, further enhancing learning and memory performance (24, 25).
To our knowledge, the most recent comprehensive systematic review on this topic was published in 2021 (26), including studies using tDCS stimulation for treating patients with epilepsy. In follow-up, 84% (21/25) of the included studies reported a reduction in seizure frequency, with no reports of serious adverse events, suggesting it is generally a safe and effective intervention. However, the studies included in this review exhibited low quality and substantial heterogeneity. Recently completed new randomized controlled trials (RCTs) prompt us to conduct a first-time meta-analysis based on RCTs and systematically review the literature on the effects of tDCS on neurocognitive functions, aiming to provide new insights for clinical decision-making. At the time of writing our manuscript, a new systematic review and meta-analysis (27) has been published, which found that active transcranial direct current stimulation treatment significantly reduced seizure frequency by 3.15 seizures per month compared to the sham group. However, we focused on the analysis of seizure reduction rate, instead of seizure reduction numbers, to minimize the differences of baseline seizure frequency. And we further reviewed the improvement in cognitive ability and psychological state in patients. In addition, temporal lobe epilepsy, the most common drug-resistant epilepsy, were chosen for subgroup to review the effects of electrical stimulation.
2 Materials and methods
This study was carried out based on the Cochrane Handbook for the Systematic Review of Interventions (for details, see at: http://training.cochrane.org/handbook) and the Preferred Reporting Items for Systematic Reviews and Meta-Analyses Statement (PRISMA) (28). The research protocol was published on INPLASY (INPLASY202460033). This study does not require ethical approval or patient consent.
2.1 Search strategy
Two researchers independently searched the PubMed, Embase, Web of Science, and Cochrane Library databases using a combined approach of Medical Subject Heading (MeSH) terms and free-text terms. Search terms included epilepsy (MeSH) or Epilep* or seizur*; Transcranial Direct Current Stimulation (MeSH) or tDCS or t-DCS or brain polarization or galvanic stimulation; and randomized controlled trial (Publication Type [pt]) or controlled clinical trial (pt) or random* or placebo (Supplementary material). Searches were conducted from database inception to May 10, 2024.
2.2 Inclusion and exclusion criteria
Inclusion criteria include:
• Study type: Randomized controlled trials or randomized crossover trials.
• Study language: English literature.
• Study subjects: Patients clinically diagnosed with epilepsy according to the International League Against Epilepsy (ILAE).
• Intervention method: The control group received sham stimulation, while the experimental group received cathodal tDCS treatment.
• Study outcomes must include at least seizure frequency.
Exclusion criteria include:
• Duplicate literature.
• Reviews, systematic reviews/meta-analyses, case reports, conference papers, animal experiments, letters, patents, and unrelated literature.
• Literature for which the full text cannot be obtained.
• Studies without seizure frequency as an outcome.
2.3 Literature screening and data extraction
Two researchers rigorously conducted literature searches according to predefined inclusion and exclusion criteria. All identified studies were managed using EndNote X9 software, where duplicates were removed. Titles and abstracts were screened for initial relevance, and full texts were retrieved for further assessment against the criteria for this systematic review. Basic information from selected studies was extracted and cross-checked, including title, first author, publication year, and country of origin. Data extracted also encompassed participant details (sample size, patient age, and gender), parameters (anode and cathode positioning, electric current dose in mA, electrode size in cm2, electrode type), treatment duration and follow-up time (sessions, time of follow-up), and outcome measures. Discrepancies in data extraction were resolved through consensus with a third researcher. When multiple publications from the same trial or cohort were identified, the original reports with all pertinent data elements were included. The intended outcomes include the means, standard deviation, and differences in interictal epileptiform discharges (IEDs) in electroencephalogram (EEG), seizure frequency, neuropsychological test scores, and brain network before and after stimulation. Additionally, it includes the number of participants achieving a seizure reduction of ≥50% and any adverse events. Attempts were made to obtain individual participant data (IPD) from all included studies by contacting corresponding authors. Studies without accessible IPD were analyzed using reported study-level data.
2.4 Quality assessment of studies
Two researchers (CYJ and OZJ) independently conducted methodological assessments of the included literature using the Cochrane Risk of Bias Tool 2.0 (29). Discrepancies were resolved by a third researcher, and consensus was reached through discussion. The assessment covered five domains: bias in the randomization process, bias due to deviations from intended interventions, bias due to missing outcome data, bias in outcome measurement, and bias in selective reporting of results. Each domain was evaluated for “low risk of bias,” “some concerns,” or “high risk of bias.” This standardized approach, facilitated by the official tool, enhances procedural and methodological rigor while minimizing subjective influences.
2.5 Outcome measures
The primary outcome measures included: Seizure Reduction: defined as the change in seizure frequency relative to baseline following tDCS treatment. Favorable Response: defined as the proportion of participants achieving a 50% or greater reduction in seizure frequency. Secondary outcome measures included. EEG results including IED number or frequency. Functional magnetic resonance Imaging (fMRI): Including functional connectivity and task-based imaging. Psychological tests includes assessment for one or more domains of cognitive functions and psychological state. Adverse Effects: Number of participants experiencing adverse events and specific types described in the literature.
2.6 Statistical analysis
Data extraction from the literature was independently conducted by two researchers and entered into StataSE16 (Stata Corp, College Station, TX, USA) for statistical analysis. For binary outcome variables such as response rates, data were entered as event numbers, and relative risk (RR) was used as the effect size. Continuous data, such as improvement rates, were entered in mean and standard deviation format, and the weighted mean difference (WMD) was used as the effect size, with both reported with 95% confidence intervals (95%CI). Heterogeneity among included studies was assessed using the inconsistency index (I2). I2 values < 50% indicate no significant heterogeneity among studies, warranting the use of a fixed-effects model for analysis. I2 values >50% indicate significant heterogeneity among studies, requiring the use of a random-effects model for analysis. To explore potential sources of heterogeneity, subgroup analyses were performed based on follow-up time (within 1 month or within 2 months), treatment duration (sessions ≤ 5 or >5), and epilepsy type (including only temporal lobe epilepsy patients). Sensitivity analyses were subsequently conducted. Publication bias among included studies was assessed using Egger's test. All results were considered statistically significant at P < 0.05. Secondary outcome measures were subjected to descriptive analysis and reported in the systematic review.
3 Results
3.1 Literature retrieval process
A total of 608 articles were retrieved. Specifically, 25 articles were identified through PubMed, 478 through Web of Science, 146 through Embase, and 126 through The Cochrane Library. After screening, 14 articles were included in the study. The flowchart of literature retrieval and results are shown in Figure 1. Among these 14 studies, 13 were randomized, double-blind trials, with four utilizing a crossover design. The remaining study was a randomized single-blind controlled trial. A total of 342 epilepsy patients were included in this meta-analysis, all of whom received catodal tDCS as the treatment modality. The basic characteristics of the included studies are summarized in Table 1. The follow-up time across the selected articles ranged from 1 week to 3 months (median, 1 month), with a notable frequency of 2-month follow-ups observed. We therefore selected 1 and 2 months for subgroup analysis. The treatment sessions were 1–14 (median, 5). Therefore, we used five sessions for subgroup analysis of cut-off value in the following analyses (median, five sessions).
3.2 Quality assessment of included studies
Risk of bias assessment was conducted for the final 14 studies, and a methodological quality assessment summary figure (Figure 2) was generated using Excel software. According to ROB2.0 assessment, among the included studies, 50% exhibited low risk of bias in the intention-to-treat (ITT) analysis, while 10% showed high risk of bias in ITT analysis. For the per-protocol (PP) analysis, 55%, 20%, and 25% of studies displayed low risk, some concerns, and high risk of bias, respectively. Sources of high bias risk included baseline imbalance, improper handling of missing outcome data, and potential reporting biases. Overall, the included literature demonstrated relatively high quality. Detailed ROB2.0 results are presented in Figure 2.
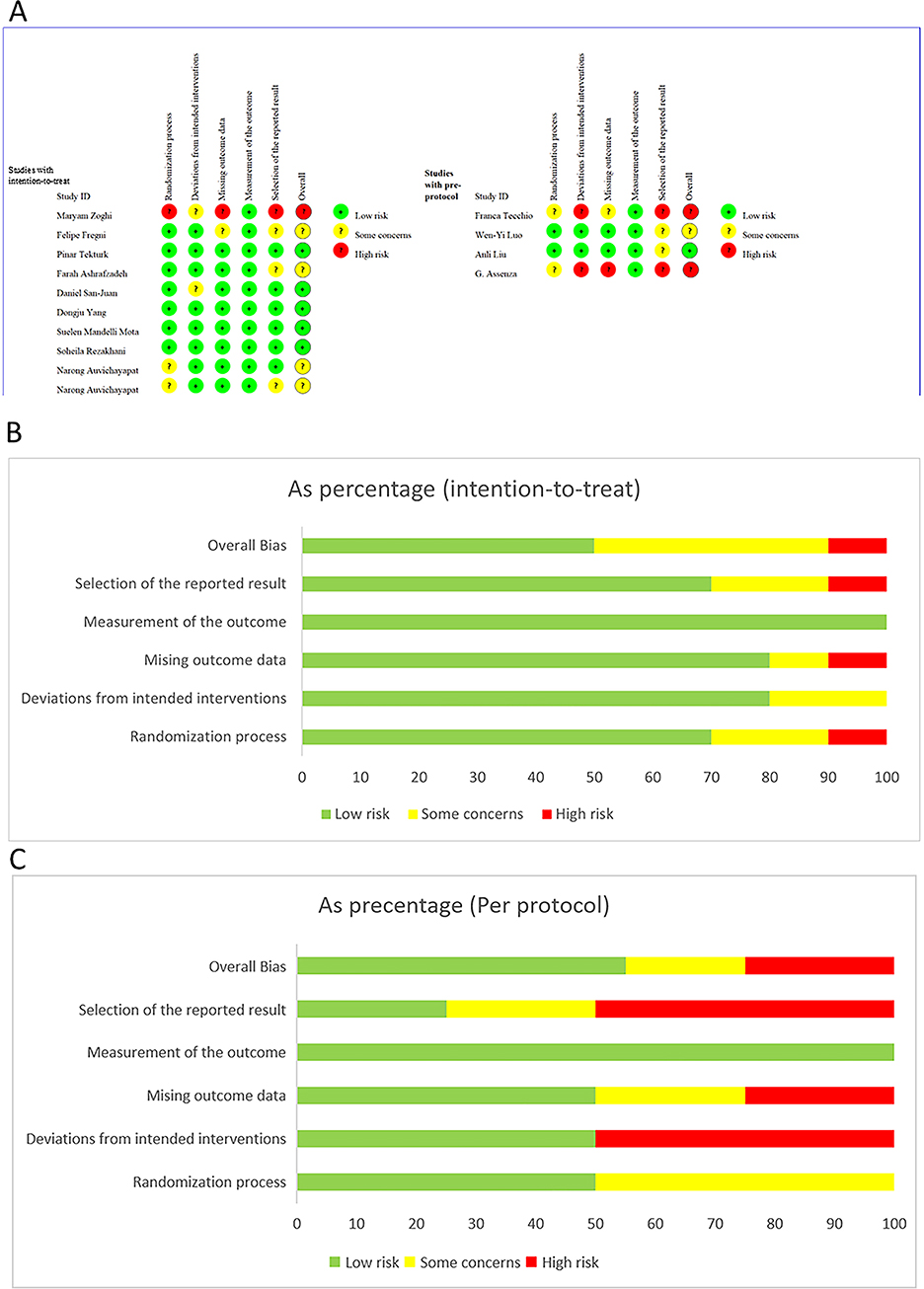
Figure 2. Risk of bias assessment according to the Cochrane collaboration's risk of bias assessment tool. (A) Assessment for 14 included studies, (B) assessment for studies with intention to treat design, (C) assessment for studies with per-protocal design.
3.3 Meta-analysis results
3.3.1 Seizure frequency
During the follow-up period, 64.3% (9/14) of the included studies reported statistically significant reduction in seizure frequency. Among these, Yang et al.'s study (30) was split into two articles due to different treatment durations, and Tecchio et al. (31) and Assenza et al. (32) were from the same study, with only the latter included in the meta-analysis covering all participants. We employed a random-effects model for data synthesis (I2 = 62.8%, p = 0.003). Overall results showed that tDCS treatment significantly reduced seizure frequency compared to sham stimulation after treatment (WMD 0.41, 95% CI 0.24, 0.59). Subgroup analyses were subsequently conducted to explore sources of heterogeneity. Two studies (30, 33) reported seizure frequency reduction at different time points after tDCS treatment. We found significant reduction in seizure frequency within 1 month post-tDCS treatment (WMD 0.28, 95% CI 0.16, 0.40) and sustained efficacy within 2 months' post-treatment (WMD 0.49, 95% CI 0.20, 0.78; Figure 3A). Treatment session duration (< 5 sessions vs. ≥5 sessions) and exclusively inclusion of temporal lobe epilepsy patients were not identified as sources of overall effect heterogeneity. However, shorter intervention duration (< 5 sessions; WMD 0.50, 95% CI 0.25, 0.74; Figure 3B) and temporal lobe epilepsy patients (WMD 0.50, 95% CI 0.28, 0.72; Figure 3C) showed greater reduction in seizure frequency. Four studies reported the proportion of participants achieving ≥50% reduction in seizures, indicating no significantly higher proportion among tDCS-treated patients compared to those receiving sham stimulation (RR 2.96, 95% CI 0.85, 10.32; Figure 4).
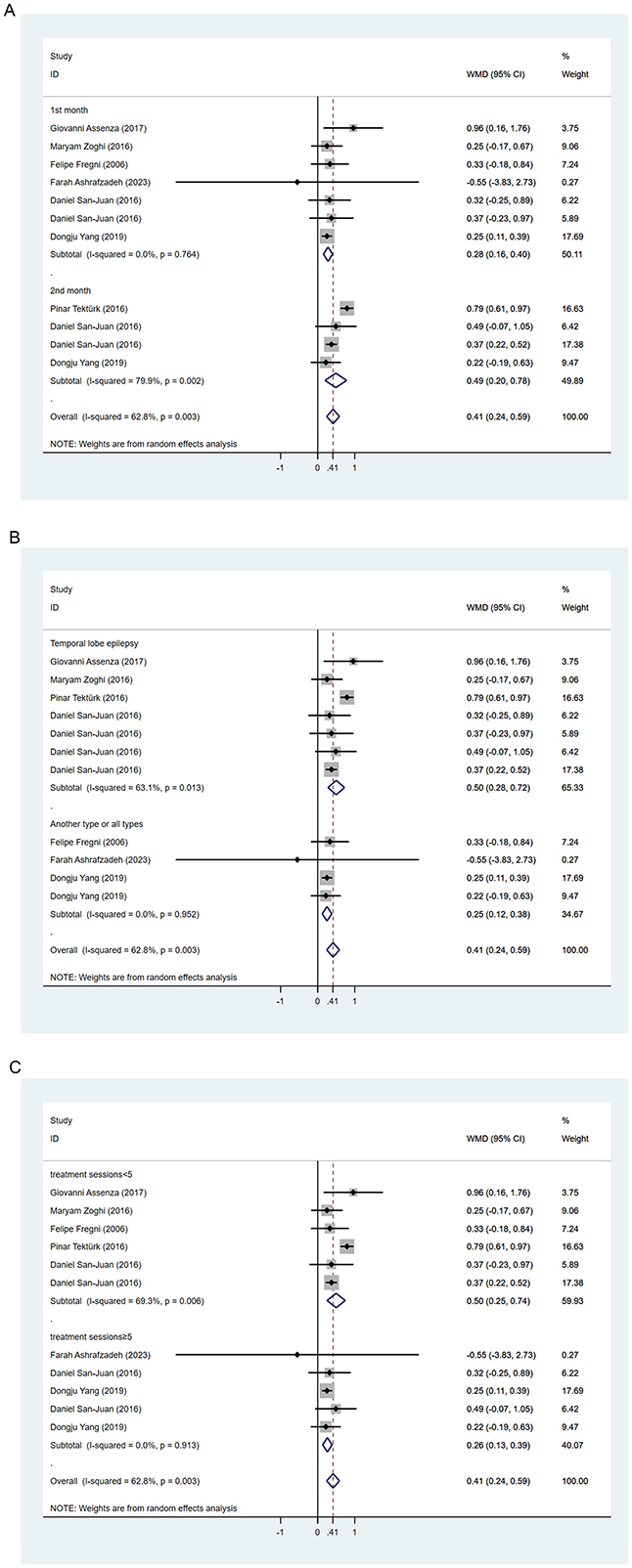
Figure 3. Forest plots of the effects of tDCS on the seizure reduction for (A) follow-up time, (B) seizure type, and (C) treatment session. WMD, Weighted Mean Difference.
3.3.2 Neuropsychological evaluation
Four studies utilized neuropsychological tests to assess changes in cognition and mood in epilepsy patients before and after tDCS treatment (34–37). Three studies employed the Montreal Cognitive Assessment (MoCA) or Mini-Mental State Examination (MMSE) to evaluate cognitive function comprehensively. Only Rezakhani observed an improvement in MoCA scores 2 weeks post-stimulation (34). However, this difference was not significant upon re-evaluation 1 month later, potentially due to a learning effect in MoCA testing. Across these studies, no statistically significant improvements or deteriorations in overall cognition were found with tDCS treatment. Liu et al. (35) further evaluated improvements in cognitive domains such as working memory and executive function post-treatment, with no significant improvements observed during the 1-month follow-up.
3.3.3 fMRI and EEG results
Ten studies reported changes in electroencephalogram (EEG) before and after tDCS treatment. Four studies did not find significant changes in pre-ictal epileptic activity following tDCS treatment (35, 38–40). Six studies (33, 34, 37, 41–43) found a reduction in interictal epileptic-like activity post-treatment. Among these, Fregni observed patients with cortical dysplasia after treatment showed a trend toward reduced epileptic activity, albeit possibly only maintained for a few sessions (41). Rezakhani observed a reduction in interictal epileptic-like discharges 3 months post-treatment (36), while San-Juan's study showed a transient effect of reduced interictal epileptic-like discharges post-tDCS treatment (34). Luo et al. (37) used graph theory-based EEG analysis to study brain network characteristics in epilepsy, finding a decrease in small-worldness index post-tDCS treatment, suggesting refined information transfer efficiency, potentially inhibiting the propagation of epileptic-like discharges, although this study did not find a decrease in seizure frequency. Tecchio et al. (32) proposed functional magnetic resonance imaging (fMRI) findings in epilepsy patients post-tDCS treatment, indicating changes in functional connectivity in epileptic regions. Changes in functional connectivity values across all frequency bands were significantly correlated with seizure frequency, suggesting tDCS has the potential to disrupt pathological network activity in epilepsy patients.
3.3.4 Adverse effects
Most adverse effects associated with tDCS are mild, with the most common being tingling sensations. Other frequently reported side effects include headache and burning sensations. These side effects are mostly self-limiting, transient, and tolerable. One participant withdrew from the study due to scalp burning sensation and pain. Two studies reported a total of five seizures (30, 37), which were brief focal seizures without additional intervention such as medication, and they were not directly attributable to stimulation, as these patients had a history of frequent seizures prior to treatment. Overall, tDCS appears to be safe for epilepsy treatment, though large-scale trials are needed for further validation.
3.4 Publication bias and sensitivity analysis
We conducted a publication bias analysis for the included indicators. A funnel plot was used to visually present publication bias, and Egger's test was employed to analyze the funnel plot, with p > 0.05 indicating no publication bias (Figure 5). The results indicate no publication bias present across the included indicators. Sensitivity analysis was performed by individually excluding each study from the meta-analysis to rule out the excessive influence of any single study on the meta-analysis results. The sensitivity analysis results indicated that the meta-analysis results were stable and reliable (Figure 6).
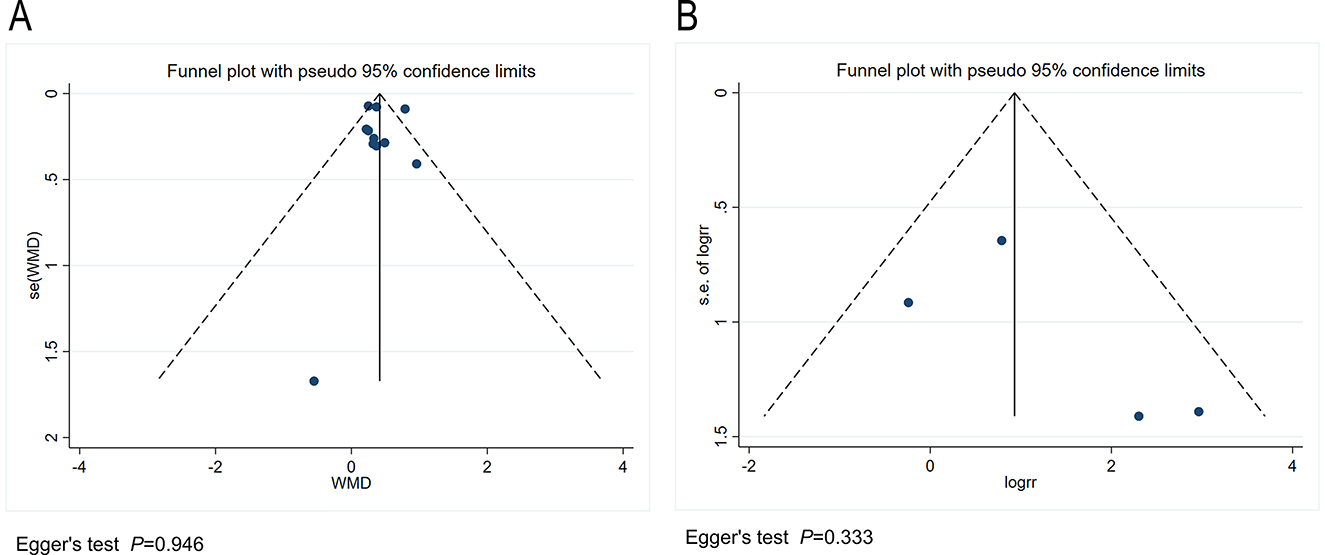
Figure 5. (A) Funnel plot for the publication bias regarding the seizure reduction compared with baseline. (B) Funnel plot for the publication bias regarding the responder rate.
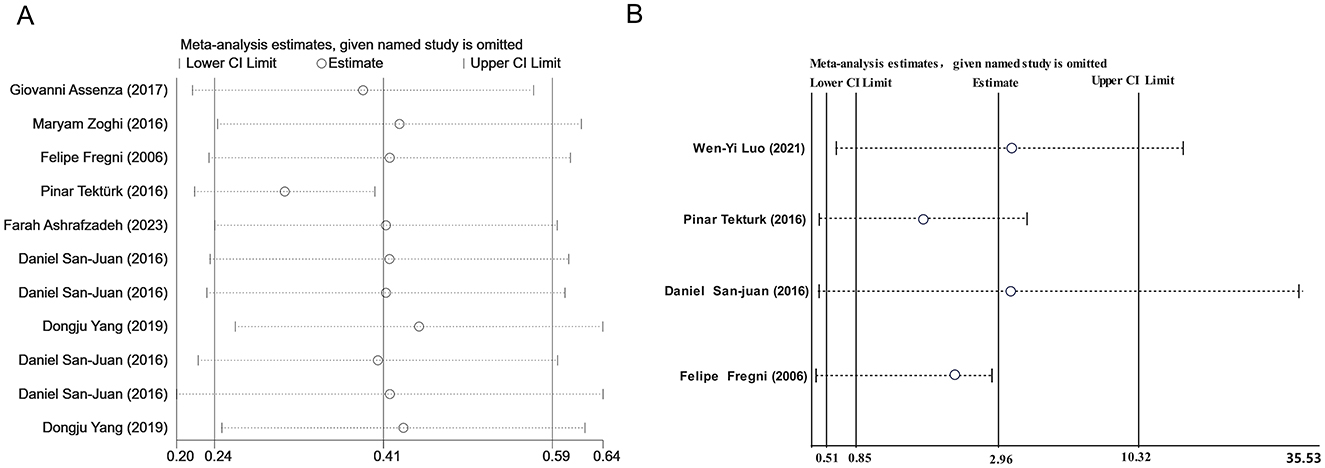
Figure 6. (A) Sensitivity analysis of seizure reduction compared with baseline. (B) Sensitivity analysis of responder rate.
4 Discussion
Despite several systematic reviews indicating (26, 44–46) that tDCS may reduce seizure frequency in the treatment of epilepsy, the findings regarding its effectiveness are inconclusive, and its efficacy has not been quantified. Here, we provided an updated meta-analysis of RCTs available to address this knowledge gap concerning the efficacy of tDCS in epilepsy treatment. We used seizure frequency reduction as the primary outcome to mitigate the influence of baseline differences on outcomes and employed WMD as the effect size measure for easier interpretation. Our results indicated that tDCS reduced seizure frequency by ~28 and 49% within 1- and 2-month follow-ups post-treatment, respectively. Further subgroup analysis found that it was more effective for participants with temporal lobe epilepsy. However, we did not find that it effectively increased the proportion of patients whose seizure frequency was reduced by 50% or more. On the other hand, consistent with systematic review findings, tDCS showed no clear improvement in cognitive and emotional functions.
To mitigate the heterogeneity caused by varying follow-up durations, this meta-analysis quantitatively analyzed changes in seizure frequency within 1 and 2 months intervals post-tDCS treatment. It observed a short-term reduction in seizure frequency following tDCS treatment, consistent with our clinical observations. Additionally, Kaufmann et al. prospectively included 15 patients with refractory focal epilepsy to explore the acute effects of tDCS, finding significant reduction in seizure frequency and epileptic-like discharges 48 h post-treatment, with the most pronounced effects observed 3–21 h post-stimulation (47). Initial placebo effects was large treated with brain stimulation (48, 49), potentially overestimating its immediate efficacy. In fact, traditional invasive neuromodulation techniques only achieve seizure frequency reduction of 14.7% (VNS), 32.1% (RNS), and 40.4% (DBS) within the first 3 months post-treatment (50). Nevertheless, leveraging placebo effects effectively could aid epilepsy patients and potentially synergize with other therapeutic mechanisms (49). The implementation of blinding closely correlates with placebo effects, with all included studies in our analysis employing blinding. However, few studies assessed the quality of blinding. Encouragingly, our results suggested that the efficacy within 2 months of treatment was more pronounced than within 1 month, contrasting with findings for TMS, which showed short-term effects in refractory epilepsy interventions but an increasing trend in seizure propensity over time with stimulation (51). Given the limited number of studies included for the 2-month follow-up, caution is warranted in interpreting the long-term effects of tDCS. Future studies should be designed to explore the time-course effects of tDCS.
Further subgroup analyses did not reveal any influence of treatment duration on efficacy. This may be due to several reasons: Firstly, the lack of dose-response relationship trials resulted in a less compressive categorization of treatment duration. Secondly, this observation might suggest that tDCS was effective in specific populations, such as mesial temporal lobe epilepsy and Lennox-Gastaut syndrome (52). For instance, both shorter (< 5 sessions) and longer (>5 sessions) treatments have been found effective for medial temporal lobe epilepsy, consistent with quantitative analysis results targeting temporal lobe epilepsy, possibly due to the specific treatment targets of this epilepsy syndrome. Abuhaib et al. stimulated the FT7 area in patients with temporal lobe epilepsy and used magnetic resonance spectroscopy to find a decrease in γ-aminobutyric acid (GABA) in the temporal region, which was related to interictal epileptic discharges (53). Compared to repetitive transcranial magnetic stimulation (rTMS), tDCS may have better efficacy for temporal lobe epilepsy, while the latter may be more effective for neocortical epilepsy (54). Although the comparison of these two was not the focus of this study, it suggested the need for future studies to include a more diverse range of epilepsy syndromes and explore other relevant biomarkers besides electroencephalography.
Through systematic review, we have preliminarily explored the mechanisms of tDCS, which may act by inhibiting cortical excitability, modulating cortical excitation/inhibition balance, and reshaping epilepsy networks. Four studies have reported the frequency of IED showed no significant change following tDCS stimulation when compared to sham groups. Among these, three studies reported no reduction in seizure frequency as well. Equally, six studies reported IED frequency decrease after treatment, with four of them reported seizure reduction. The observed decrease in IEDs post-treatment can possibly be a biomarker linked to favorable treatment response. In addition, the results in IED change after tDCS stimulation differed both in shorter and longer treatment. Recent study explored the immediate effects of tDCS, using stereo-electroencephalography (SEEG) recordings to accurately localize epileptogenic zone (55). One finding is that IED changes were also inconsistent after shorter treatment (one session, 20 min). Importantly, one patient after sham stimulation reported significant IED decrease and they pointed out most patients had no insufficient IED for statistical analysis as well as inter-subject variability in baseline IEDs, and Fregni reported the number of IEDs were largely variable among patients accordingly. More uniform and less variable markers linked to brain response are required in the future.
Despite previous studies reporting that slow oscillatory transcranial direct current stimulation (so-tDCS) improves memory consolidation during naps in temporal lobe epilepsy patients (56), and high-definition transcranial direct current stimulation (HD-tDCS) enhances attention and working memory in frontal lobe epilepsy patients (57, 58). Further systematic review results suggest no significant improvement in cognition with tDCS. This may be attributed to several reasons: Firstly, in terms of treatment sites, the dorsolateral prefrontal cortex is a common target for treating cognitive impairments (59–62). In three studies assessing cognition as an outcome, two used cathodal electrode stimulation at epileptic foci, and the studies utilized modified versions of tDCS, such as so-tDCS aiming to simulate slow waves during sleep, enhancing endogenous slow oscillatory activity (63), while HD-tDCS offers advantages in terms of current concentration (64). Secondly, there is a lack of analysis on cognition domains with included studies using MOCA and MMSE, which may have lower sensitivity to evaluate tDCS treatment effects. Thirdly, small samples in these three articles may result in insufficient statistical power, leading to type II statistical errors and false-negative results.
Limitations of this work include the recent emergence of tDCS as a treatment modality for epilepsy, which has not yet advanced to the stage of large-scale randomized controlled trials, resulting in small sample sizes. Additionally, inavailability to clinical data related to seizure frequency in some studies has limited the reliability of our quantitative analysis conclusions. In terms of heterogeneity, existing studies have employed various stimulation parameters (e.g., stimulation duration, current intensity, stimulation intervals, and electrode size), all of which may influence the post-treatment effects of tDCS, potentially even reversing its excitatory and inhibitory effects (65, 66). Moreover, factors such as participants' medication use, seizure frequency, and different etiologies of epilepsy could be underlying sources of heterogeneity in the studies. For instance, cathodal tDCS enhances GABA-mediated intracortical inhibition (67), while NMDA receptor antagonists may inhibit the effects of cathodal tDCS post-treatment (68).
Future research should focus on several key areas: Firstly, conducting larger-scale multicenter randomized controlled trials to enhance the reliability and generalizability of results. Secondly, systematically studying the impact of different stimulation parameters on efficacy to identify optimal stimulation protocols. Additionally, long-term follow-up studies are needed to understand the sustained effectiveness and potential side effects of tDCS. Lastly, as neuromodulation becomes increasingly important in the coming years, research should particularly focus on identifying specific populations that can benefit from various neuromodulation techniques beyond tDCS, and there is a need for more cost-effectiveness studies to guide treatment decisions and resource allocation. These studies will contribute to optimizing the application strategies of neuromodulation and improving its practical effectiveness in epilepsy treatment.
5 Conclusion
This study demonstrates that tDCS significantly reduces seizure frequency post-treatment. Future research should include longer-term and larger RCTs, as well as other cohort studies, to validate the findings of this study.
Data availability statement
The original contributions presented in the study are included in the article/Supplementary material, further inquiries can be directed to the corresponding authors.
Author contributions
YC: Data curation, Formal analysis, Resources, Writing – original draft, Writing – review & editing. ZO: Data curation, Formal analysis, Resources, Writing – original draft, Writing – review & editing. NH: Data curation, Funding acquisition, Resources, Writing – original draft. HZ: Data curation, Resources, Writing – original draft. EZ: Data curation, Resources, Writing – original draft. DZ: Funding acquisition, Writing – review & editing. XW: Funding acquisition, Writing – review & editing, Conceptualization, Supervision.
Funding
The author(s) declare financial support was received for the research, authorship, and/or publication of this article. This work was funded by Xintong Wu from Science and Technology Department (No. 2021YFC2401204 and 2022YFC2503805), Science and Technology Department of Sichuan Province (No. 2023YFQ0109), Dong Zhou from the 1.3.5 Project for Disciplines of Excellence, West China Hospital, Sichuan University (Nos. ZY2017305 and ZYGD20011), Nanya Hao from the National Natural Science Foundation of China (No. 81901327), and the Post-Doctor Research Project of West China Hospital (No. 2019HXBH080).
Conflict of interest
The authors declare that the research was conducted in the absence of any commercial or financial relationships that could be construed as a potential conflict of interest.
Publisher's note
All claims expressed in this article are solely those of the authors and do not necessarily represent those of their affiliated organizations, or those of the publisher, the editors and the reviewers. Any product that may be evaluated in this article, or claim that may be made by its manufacturer, is not guaranteed or endorsed by the publisher.
Supplementary material
The Supplementary Material for this article can be found online at: https://www.frontiersin.org/articles/10.3389/fneur.2024.1462364/full#supplementary-material
References
1. Pong AW, Xu KJ, Klein P. Recent advances in pharmacotherapy for epilepsy. Curr Opin Neurol. (2023) 36:77–85. doi: 10.1097/WCO.0000000000001144
2. Ding D, Zhou D, Sander JW, Wang W, Li S, Hong Z. Epilepsy in China: major progress in the past two decades. Lancet Neurol. (2021) 20:316–26. doi: 10.1016/S1474-4422(21)00023-5
3. Taylor RS, Sander JW, Taylor RJ, Baker GA. Predictors of health-related quality of life and costs in adults with epilepsy: a systematic review. Epilepsia. (2011) 52:2168–80. doi: 10.1111/j.1528-1167.2011.03213.x
4. Sultana B, Panzini MA, Veilleux Carpentier A, Comtois J, Rioux B, Gore G, et al. Incidence and prevalence of drug-resistant epilepsy: a systematic review and meta-analysis. Neurology. (2021) 96:805–17. doi: 10.1212/WNL.0000000000011839
5. Jetté N, Sander JW, Keezer MR. Surgical treatment for epilepsy: the potential gap between evidence and practice. Lancet Neurol. (2016) 15:982–94. doi: 10.1016/S1474-4422(16)30127-2
6. Hader WJ, Tellez-Zenteno J, Metcalfe A, Hernandez-Ronquillo L, Wiebe S, Kwon CS, et al. Complications of epilepsy surgery: a systematic review of focal surgical resections and invasive EEG monitoring. Epilepsia. (2013) 54:840–7. doi: 10.1111/epi.12161
7. Macrodimitris S, Sherman EM, Williams TS, Bigras C, Wiebe S. Measuring patient satisfaction following epilepsy surgery. Epilepsia. (2011) 52:1409–17. doi: 10.1111/j.1528-1167.2011.03160.x
8. Haneef Z, Skrehot HC. Neurostimulation in generalized epilepsy: a systematic review and meta-analysis. Epilepsia. (2023) 64:811–20. doi: 10.1111/epi.17524
9. Alomar AS, Saeedi JR. Different modalities of invasive neurostimulation for epilepsy. Neurol Sci. (2020) 41:3527–36. doi: 10.1007/s10072-020-04614-z
10. Gouveia FV, Warsi NM, Suresh H, Matin R, Ibrahim GM. Neurostimulation treatments for epilepsy: deep brain stimulation, responsive neurostimulation and vagus nerve stimulation. Neurotherapeutics. (2024) 21:e00308. doi: 10.1016/j.neurot.2023.e00308
11. Ngan Kee N, Foster E, Marquina C, Tan A, Pang SST, O'Brien TJ, et al. Systematic review of cost-effectiveness analysis for surgical and neurostimulation treatments for drug-resistant epilepsy in adults. Neurology. (2023) 100:e1866–77. doi: 10.1212/WNL.0000000000207137
12. Lundstrom BN, Lin C, Starnes DK, Middlebrooks EH, Tatum W, Grewal SS, et al. Safety and management of implanted epilepsy devices for imaging and surgery. Mayo Clin Proc. (2022) 97:2123–38. doi: 10.1016/j.mayocp.2022.06.012
13. George MS, Lisanby SH, Sackeim HA. Transcranial magnetic stimulation: applications in neuropsychiatry. Arch Gen Psychiatry. (1999) 56:300–11. doi: 10.1001/archpsyc.56.4.300
14. Yap JYY, Keatch C, Lambert E, Woods W, Stoddart PR, Kameneva T. Critical review of transcutaneous vagus nerve stimulation: challenges for translation to clinical practice. Front Neurosci. (2020) 14:284. doi: 10.3389/fnins.2020.00284
15. Lundstrom BN, Osman GM, Starnes K, Gregg NM, Simpson HD. Emerging approaches in neurostimulation for epilepsy. Curr Opin Neurol. (2023) 36:69–76. doi: 10.1097/WCO.0000000000001138
16. Espinoza Lopez DA, San Juan Orta D, Morales Quezada L, Orozco Garduño A, Alonso Vanegas MA, Fernandez M, et al. A randomized controlled clinical trial of cathodal transcraneal direct current stimulation in patients with temporal lobe epilepsy with hippocampal sclerosis refractory to pharmacological treatment. Epilepsia. (2015) 56:24–5. doi: 10.1111/epi.13241
17. Fregni F, El-Hagrassy MM, Pacheco-Barrios K, Carvalho S, Leite J, Simis M, et al. Evidence-based guidelines and secondary meta-analysis for the use of transcranial direct current stimulation in neurological and psychiatric disorders. Int J Neuropsychopharmacol. (2021) 24:256–313. doi: 10.1093/ijnp/pyaa051
18. Lee JH, Lee TL, Kang N. Transcranial direct current stimulation decreased cognition-related reaction time in older adults: a systematic review and meta-analysis. Ageing Res Rev. (2021) 70:101377. doi: 10.1016/j.arr.2021.101377
19. Müller D, Habel U, Brodkin ES, Weidler C. High-definition transcranial direct current stimulation (HD-tDCS) for the enhancement of working memory—a systematic review and meta-analysis of healthy adults. Brain Stimul. (2022) 15:1475–85. doi: 10.1016/j.brs.2022.11.001
20. Mutz J. Brain stimulation treatment for bipolar disorder. Bipolar Disord. (2023) 25:9–24. doi: 10.1111/bdi.13283
21. Sun CH, Jiang WL, Cai DB, Wang ZM, Sim K, Ungvari GS, et al. Adjunctive multi-session transcranial direct current stimulation for neurocognitive dysfunction in schizophrenia: a meta-analysis. Asian J Psychiatr. (2021) 66:102887. doi: 10.1016/j.ajp.2021.102887
22. Kamida T, Kong SQ, Eshima N, Abe T, Fujiki M, Kobayashi H. Transcranial direct current stimulation decreases convulsions and spatial memory deficits following pilocarpine-induced status epilepticus in immature rats. Behav Brain Res. (2011) 217:99–103. doi: 10.1016/j.bbr.2010.08.050
23. Regner GG, Torres ILS, De Oliveira C, Pflüger P, Da Silva LS, Scarabelot VL, et al. Transcranial direct current stimulation (tDCS) affects neuroinflammation parameters and behavioral seizure activity in pentylenetetrazole-induced kindling in rats. Neurosci Lett. (2020) 735:135162. doi: 10.1016/j.neulet.2020.135162
24. Kronberg G, Bridi M, Abel T, Bikson M, Parra LC. Direct current stimulation modulates LTP and LTD: activity dependence and dendritic effects. Brain Stimul. (2017) 10:51–8. doi: 10.1016/j.brs.2016.10.001
25. Van Boekholdt L, Kerstens S, Khatoun A, Asamoah B, Mc Laughlin M. tDCS peripheral nerve stimulation: a neglected mode of action? Mol Psychiatry. (2021) 26:456–61. doi: 10.1038/s41380-020-00962-6
26. Sudbrack-Oliveira P, Barbosa MZ, Thome-Souza S, Razza LB, Gallucci-Neto J, da Costa Lane Valiengo L, et al. Transcranial direct current stimulation (tDCS) in the management of epilepsy: a systematic review. Seizure. (2021) 86:85–95. doi: 10.1016/j.seizure.2021.01.020
27. Lima AE, Telles JP, Dantas J, Fernandes AC, Ribeiro GBS, Barbosa VL, et al. Transcranial direct current stimulation improves seizures frequency in drug-resistant epilepsy: a systematic-review and meta-analysis of randomized controlled trials. Epilepsy Behav. (2024) 159:109974. doi: 10.1016/j.yebeh.2024.109974
28. Shamseer L, Moher D, Clarke M, Ghersi D, Liberati A, Petticrew M, et al. Preferred reporting items for systematic review and meta-analysis protocols (PRISMA-P) 2015: elaboration and explanation. Br Med J. (2015) 350:g7647. doi: 10.1136/bmj.g7647
29. Sterne JAC, Savović J, Page MJ, Elbers RG, Blencowe NS, Boutron I, et al. RoB 2: a revised tool for assessing risk of bias in randomised trials. Br Med J. (2019) 366:l4898. doi: 10.1136/bmj.l4898
30. Yang D, Wang Q, Xu C, Fang F, Fan J, Li L, et al. Transcranial direct current stimulation reduces seizure frequency in patients with refractory focal epilepsy: a randomized, double-blind, sham-controlled, and three-arm parallel multicenter study. Brain Stimul. (2020) 13:109–16. doi: 10.1016/j.brs.2019.09.006
31. Tecchio F, Cottone C, Porcaro C, Cancelli A, Di Lazzaro V, Assenza G. Brain functional connectivity changes after transcranial direct current stimulation in epileptic patients. Front Neural Circuits. (2018) 12:44. doi: 10.3389/fncir.2018.00044
32. Assenza G, Cottone C, Tecchio F, Pellegrino G, Campana C, Porcaro C, et al. Cathodal transcranial direct current stimulation reduces seizure frequency and modulates brain functional connectivity with drug-resistant temporal lobe epilepsy: a Sham controlled study. Clin Neurophysiol. (2017) 128:e416–7. doi: 10.1016/j.clinph.2017.09.014
33. San-Juan D, Espinoza López DA, Vázquez Gregorio R, Trenado C, Fernández-González Aragón M, Morales-Quezada L, et al. Transcranial direct current stimulation in mesial temporal lobe epilepsy and hippocampal sclerosis. Brain Stimul. (2017) 10:28–35. doi: 10.1016/j.brs.2016.08.013
34. Rezakhani S, Amiri M, Weckhuysen S, Keliris GA. Therapeutic efficacy of seizure onset zone-targeting high-definition cathodal tDCS in patients with drug-resistant focal epilepsy. Clin Neurophysiol. (2022) 136:219–27. doi: 10.1016/j.clinph.2022.01.130
35. Liu A, Bryant A, Jefferson A, Friedman D, Minhas P, Barnard S, et al. Exploring the efficacy of a 5-day course of transcranial direct current stimulation (TDCS) on depression and memory function in patients with well-controlled temporal lobe epilepsy. Epilepsy Behav. (2016) 55:11–20. doi: 10.1016/j.yebeh.2015.10.032
36. Mota SM, De Castro LA, Ríedel PG, Torres CM, Bragatti JA, Brondani R, et al. Home-based transcranial direct current stimulation for the treatment of symptoms of depression and anxiety in temporal lobe epilepsy: a randomized, double-blind, sham-controlled clinical trial. Front Integr Neurosci. (2021) 15:753995. doi: 10.3389/fnint.2021.753995
37. Luo WHL, Zhang P, Ding J. Efficacy of cathodal transcranial direct current stimulation on brain networks in patients with focal epilepsy. Brain Stimul. (2019) 12:413. doi: 10.1016/j.brs.2018.12.335
38. Assenza G, Cottone C, Tecchio F, Pellegrino G, Campana C, Porcaro C, et al. Cathodal transcranial direct current stimulation reduces seizure frequency and modulates brain functional connectivity in drug-resistant temporal lobe epilepsy: a Sham controlled study. Epilepsia. (2017) 58:S129–30. doi: 10.1016/j.clinph.2017.07.029
39. Ashrafzadeh F, Akhondian J, Hashemi N, Esmaeilzadeh M, Ghanaee A, Yavarzadeh H, et al. Therapeutical impacts of transcranial direct current stimulation on drug-resistant epilepsy in pediatric patients: a double-blind parallel-group randomized clinical trial. Epilepsy Res. (2023) 190:107074. doi: 10.1016/j.eplepsyres.2022.107074
40. Assenza G, Campana C, Assenza F, Pellegrino G, Di Pino G, Fabrizio E, et al. Cathodal transcranial direct current stimulation reduces seizure frequency in adults with drug-resistant temporal lobe epilepsy: a Sham controlled study. Brain Stimul. (2017) 10:333–5. doi: 10.1016/j.brs.2016.12.005
41. Fregni F, Thome-Souza S, Nitsche MA, Freedman SD, Valente KD, Pascual-Leone A. A controlled clinical trial of cathodal DC polarization in patients with refractory epilepsy. Epilepsia. (2006) 47:335–42. doi: 10.1111/j.1528-1167.2006.00426.x
42. Auvichayapat N, Rotenberg A, Gersner R, Ngodklang S, Tiamkao S, Tassaneeyakul W, et al. Transcranial direct current stimulation for treatment of refractory childhood focal epilepsy. Brain Stimul. (2013) 6:696–700. doi: 10.1016/j.brs.2013.01.009
43. Auvichayapat N, Sinsupan K, Tunkamnerdthai O, Auvichayapat P. Transcranial direct current stimulation for treatment of childhood pharmacoresistant Lennox-Gastaut syndrome: a pilot study. Front Neurol. (2016) 7:66. doi: 10.3389/fneur.2016.00066
44. San-Juan D, Morales-Quezada L, Orozco Garduño AJ, Alonso-Vanegas M, González-Aragón MF, Espinoza López DA, et al. Transcranial direct current stimulation in epilepsy. Brain Stimul. (2015) 8:455–64. doi: 10.1016/j.brs.2015.01.001
45. Yang Y, Shangguan Y, Wang X, Liu R, Shen Z, Tang M, et al. The efficacy and safety of third-generation antiseizure medications and non-invasive brain stimulation to treat refractory epilepsy: a systematic review and network meta-analysis study. Front Neurol. (2023) 14:1307296. doi: 10.3389/fneur.2023.1307296
46. Simula S, Daoud M, Ruffini G, Biagi MC, Bénar CG, Benquet P, et al. Transcranial current stimulation in epilepsy: a systematic review of the fundamental and clinical aspects. Front Neurosci. (2022) 16:909421. doi: 10.3389/fnins.2022.909421
47. Kaufmann E, Hordt M, Lauseker M, Palm U, Noachtar S. Acute effects of spaced cathodal transcranial direct current stimulation in drug resistant focal epilepsies. Clin Neurophysiol. (2021) 132:1444–51. doi: 10.1016/j.clinph.2021.03.048
48. De Smet S, Nikolin S, Moffa A, Suen P, Vanderhasselt MA, Brunoni AR, et al. Determinants of Sham response in tDCS depression trials: a systematic review and meta-analysis. Prog Neuropsychopharmacol Biol Psychiatry. (2021) 109:110261. doi: 10.1016/j.pnpbp.2021.110261
49. Nikolin S, Moffa A, Razza L, Martin D, Brunoni A, Palm U, et al. Time-course of the tDCS antidepressant effect: an individual participant data meta-analysis. Prog Neuropsychopharmacol Biol Psychiatry. (2023) 125:110752. doi: 10.1016/j.pnpbp.2023.110752
50. Touma L, Dansereau B, Chan AY, Jetté N, Kwon CS, Braun KPJ, et al. Neurostimulation in people with drug-resistant epilepsy: systematic review and meta-analysis from the ILAE Surgical Therapies Commission. Epilepsia. (2022) 63:1314–29. doi: 10.1111/epi.17243
51. Wang Z, Zhang X, Meiduo G, Song M, Wang S. Time-effectiveness of low-frequency rTMS for epilepsy and improvement in cognitive function in patients: a systematic review and meta-analysis. Epilepsy Res. (2024) 199:107277. doi: 10.1016/j.eplepsyres.2023.107277
52. Boon P, De Cock E, Mertens A, Trinka E. Neurostimulation for drug-resistant epilepsy: a systematic review of clinical evidence for efficacy, safety, contraindications and predictors for response. Curr Opin Neurol. (2018) 31:198–210. doi: 10.1097/WCO.0000000000000534
53. Abuhaiba SI, Duarte IC, Castelhano J, Dionísio A, Sales F, Edden R, et al. The impact of cathodal tDCS on the GABAergic system in the epileptogenic zone: a multimodal imaging study. Front Neurol. (2022) 13:935029. doi: 10.3389/fneur.2022.935029
54. Sun W, Mao W, Meng X, Wang D, Qiao L, Tao W, et al. Low-frequency repetitive transcranial magnetic stimulation for the treatment of refractory partial epilepsy: a controlled clinical study. Epilepsia. (2012) 53:1782–9. doi: 10.1111/j.1528-1167.2012.03626.x
55. Simula S, Makhalova J, Pizzo F, Garnier E, Damiani G, Mercadal B, et al. Impact of transcranial electrical stimulation on simultaneous stereoelectroencephalography recordings: a randomized sham-controlled study. Clin Neurophysiol. (2024) 166:211–22. doi: 10.1016/j.clinph.2024.08.003
56. Del Felice A, Magalini A, Masiero S. Slow-oscillatory transcranial direct current stimulation modulates memory in temporal lobe epilepsy by altering sleep spindle generators: a possible rehabilitation tool. Brain Stimul. (2015) 8:567–73. doi: 10.1016/j.brs.2015.01.410
57. Arzani M, Motamedi M, Karvigh SA, Nia Roshan JH. HD-tDCS in refractory lateral frontal lobe epilepsy patients. Brain Stimul. (2019) 12:394–5. doi: 10.1016/j.brs.2018.12.267
58. Roshan JHN, Chamanabad AG, Mashhadi A, Motamedi M. Cathodal HD-tDCS and attention: a study on patients with intractable left lateral frontal lobe epilepsy. Epilepsy Res. (2024) 199:107265. doi: 10.1016/j.eplepsyres.2023.107265
59. Lefaucheur JP, André-Obadia N, Antal A, Ayache SS, Baeken C, Benninger DH, et al. Evidence-based guidelines on the therapeutic use of repetitive transcranial magnetic stimulation (rTMS). Clin Neurophysiol. (2014) 125:2150–206. doi: 10.1016/j.clinph.2014.05.021
60. Emonson MRL, Fitzgerald PB, Rogasch NC, Hoy KE. Neurobiological effects of transcranial direct current stimulation in younger adults, older adults and mild cognitive impairment. Neuropsychologia. (2019) 125:51–61. doi: 10.1016/j.neuropsychologia.2019.01.003
61. Guo B, Zhang M, Hao W, Wang Y, Zhang T, Liu C. Neuroinflammation mechanisms of neuromodulation therapies for anxiety and depression. Transl Psychiatry. (2023) 13:2297. doi: 10.1038/s41398-022-02297-y
62. Flöel A. tDCS-enhanced motor and cognitive function in neurological diseases. Neuroimage. (2014) 85, 934–947. doi: 10.1016/j.neuroimage.2013.05.098
63. Bergmann TO, Groppa S, Seeger M, Mölle M, Marshall L, Siebner HR. Acute changes in motor cortical excitability during slow oscillatory and constant anodal transcranial direct current stimulation. J Neurophysiol. (2009) 102:2303–11. doi: 10.1152/jn.00437.2009
64. Kuo HI, Bikson M, Datta A, Minhas P, Paulus W, Kuo MF, et al. Comparing cortical plasticity induced by conventional and high-definition 4 × 1 ring tDCS: a neurophysiological study. Brain Stimul. (2013) 6:644–8. doi: 10.1016/j.brs.2012.09.010
65. Moliadze V, Antal A, Paulus W. Electrode-distance dependent after-effects of transcranial direct and random noise stimulation with extracephalic reference electrodes. Clin Neurophysiol. (2010) 121:2165–71. doi: 10.1016/j.clinph.2010.04.033
66. Lefaucheur JP, Antal A, Ayache SS, Benninger DH, Brunelin J, Cogiamanian F, et al. Evidence-based guidelines on the therapeutic use of transcranial direct current stimulation (tDCS). Clin Neurophysiol. (2017) 128:56–92. doi: 10.1016/j.clinph.2016.10.087
67. Regner GG, Pereira P, Leffa DT, De Oliveira C, Vercelino R, Fregni F, et al. Preclinical to clinical translation of studies of transcranial direct-current stimulation in the treatment of epilepsy: a systematic review. Front Neurosci. (2018) 12:189. doi: 10.3389/fnins.2018.00189
68. Liebetanz D, Nitsche MA, Tergau F, Paulus W. Pharmacological approach to the mechanisms of transcranial DC-stimulation-induced after-effects of human motor cortex excitability. Brain. (2002) 125:2238–47. doi: 10.1093/brain/awf238
69. Zoghi M, O'Brien TJ, Kwan P, Cook MJ, Galea M, Jaberzadeh S. Cathodal transcranial direct-current stimulation for treatment of drug-resistant temporal lobe epilepsy: a pilot randomized controlled trial. Epilepsia Open. (2016) 1:130–5. doi: 10.1002/epi4.12020
70. Tekturk P, Erdogan ET, Kurt A, Vanli-Yavuz EN, Ekizoglu E, Kocagoncu E, et al. The effect of transcranial direct current stimulation on seizure frequency of patients with mesial temporal lobe epilepsy with hippocampal sclerosis. Clin Neurol Neurosurg. (2016) 149:27–32. doi: 10.1016/j.clineuro.2016.07.014
Keywords: brain stimulation, transcranial electric stimulation, seizure, temporal lobe epilepsy, nerve stimulation, brain polarization, randomized controlled trial
Citation: Chen Y, Ou Z, Hao N, Zhang H, Zhang E, Zhou D and Wu X (2024) Transcranial direct current stimulation in the management of epilepsy: a meta-analysis and systematic review. Front. Neurol. 15:1462364. doi: 10.3389/fneur.2024.1462364
Received: 10 July 2024; Accepted: 14 October 2024;
Published: 11 November 2024.
Edited by:
Shuli Liang, Capital Medical University, ChinaReviewed by:
Kapil Gururangan, Northwestern University, United StatesJacopo Lanzone, Istituti Clinici Scientifici Maugeri IRCCS, Italy
Copyright © 2024 Chen, Ou, Hao, Zhang, Zhang, Zhou and Wu. This is an open-access article distributed under the terms of the Creative Commons Attribution License (CC BY). The use, distribution or reproduction in other forums is permitted, provided the original author(s) and the copyright owner(s) are credited and that the original publication in this journal is cited, in accordance with accepted academic practice. No use, distribution or reproduction is permitted which does not comply with these terms.
*Correspondence: Xintong Wu, MzY4NTM4MzkmI3gwMDA0MDtxcS5jb20=; Dong Zhou, emhvdWRvbmc2NiYjeDAwMDQwO3lhaG9vLmRl
†These authors have contributed equally to this work