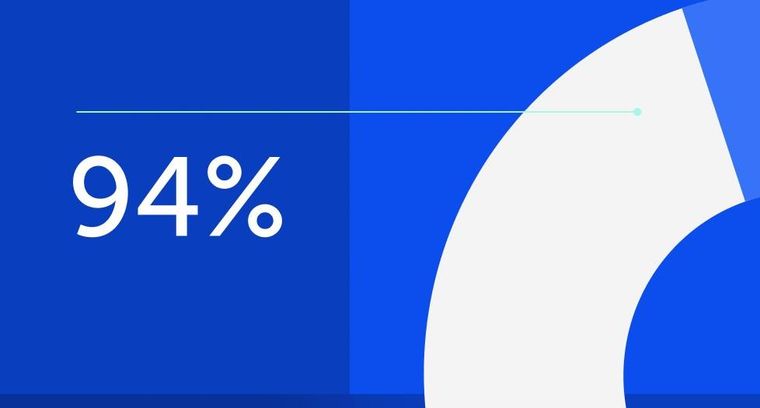
94% of researchers rate our articles as excellent or good
Learn more about the work of our research integrity team to safeguard the quality of each article we publish.
Find out more
ORIGINAL RESEARCH article
Front. Neurol., 01 October 2024
Sec. Movement Disorders
Volume 15 - 2024 | https://doi.org/10.3389/fneur.2024.1451177
Introduction: Cortical thinning is well-documented in individuals with amyotrophic lateral sclerosis (ALS), yet its association with speech deterioration remains understudied. This study characterizes anatomical changes in the brain within the context of speech impairment patterns in individuals with ALS, providing insight into the disease's multiregional spread and biology.
Methods: To evaluate patterns of cortical thickness in speakers with ALS with and without functional speech changes compared to healthy controls (HCs) using whole-brain and region of interest (ROI) analyses. Forty individuals with ALS and 22 HCs underwent a T1-weighted 3-Tesla magnetic resonance imaging (MRI). Individuals with ALS were divided into two groups based on the preserved speech [ps-ALS] (n = 18) or deteriorated speech [ds-ALS] (n = 22) as measured by the ALSFRSF-R speech subscore (=4 or <4 points, respectively). Sixteen a priori-defined and automatically segmented cortical and subcortical brain ROIs were selected based on their previously documented roles in speech production. Two cortical thickness analyses were performed: (1) group-level whole-brain surface-based analyses and (2) group-level ROI analyses. A case study of 6 ALS individuals examined the cortical thickness, and their speech was characterized using quantitative and qualitative measures.
Results: Based on the group-level whole-brain surface-based analyses, the ds-ALS group demonstrated significant cortical thinning compared to HCs in the left primary motor and somatosensory cortices and the right inferior parietal lobe with its adjacent lateral occipital cortical regions. The ps-ALS group demonstrated no significant cortical thinning compared to HCs. Based on the group-level ROI analyses, the ds-ALS group demonstrated significant cortical thinning compared to HCs in bilateral middle motor cortices, right posterior dorsal premotor cortex, and left anterior cingulate cortex. The case study analysis revealed that ALS speakers with speech features characteristic of spastic dysarthria exhibited cortical thinning, while those with speech features characteristic of flaccid dysarthria did not.
Discussion: Individuals with ALS have anatomical changes involving multiregional neocortical areas beyond the primary motor cortex that may manifest as subjective (i.e., clinical judgment) and objective (i.e., speaking rate) changes in speech production. Further longitudinal work in ALS is needed to better understand the link between MRI cortical thickness changes and bulbar dysfunction.
Amyotrophic lateral sclerosis (ALS) is a neurodegenerative disease affecting both upper and lower motor neurons (UMNs and LMNs, respectively). Over the progression of the disease, impairment of the bulbar muscles often results in dysarthria, which is a neuromotor disorder of speech execution that often leads to significantly reduced speech intelligibility (i.e., the ability to be understood). Dysarthria in ALS is typically one of three types: spastic, flaccid, or mixed spastic-flaccid, all of which can have a devastating impact on quality of life (1). Flaccid dysarthria tends to be characterized by weakened, atrophied muscles, leading to slurred speech and difficulty articulating sounds, while spastic dysarthria tends to be characterized by increased muscle tone, causing strained and effortful speech due to involuntary muscle contractions (2, 3). While the different types of speech impairment secondary to ALS are known to result from UMN and LMN damage (2, 3), there is still a paucity of knowledge regarding the specific brain regions that underlie speech deterioration in ALS.
A number of studies have examined neural correlates of bulbar deficits (i.e., impairments in salivation, swallowing, and speech) in ALS and found associations with cortical thinning in the ventral motor cortex (4), premotor and supplementary motor areas (5, 6), and superior temporal gyrus (7, 8). The largest study to date relating bulbar function to brain imaging examined 192 individuals with ALS and 314 controls longitudinally (9). The authors found that cortical thinning in motor regions was detectable earlier and progressed more rapidly than clinical UMN symptoms (9). These findings suggest that cortical thinning may serve as predictive marker for clinical UMN damage and tracks disease biology in the brain better than clinical symptoms (9). Another recent study correlated speech performance, as indexed by syllable production rate task, with structural connectivity among six extramotor regions in 19 individuals with ALS (7). The authors found that degeneration of in the left speech network may relate to bulbar severity (7). While these studies provide crucial knowledge regarding the role of extramotor regions in bulbar function, they either focus on bulbar deficits more broadly, which precludes the identification of regions underlying speech disturbances, specifically, or they are limited in sample size and consequently investigate a limited number of brain regions.
The current study seeks to examine the relationship between cortical thinning and functional speech deterioration in a larger sample with a more comprehensive network of speech-related brain regions. For a precise yet comprehensive characterization of the neural substrates involved in speech production, we used a new segmentation cortical map: the SpeechLabel Parcellation Atlas (10). This atlas delineates several regions of interest (ROIs) and their specific roles in speech production based on speech motor impairment patterns seen across a variety of neurological disorders. While it has been increasingly used for studying speech production in speech motor-impaired populations (11–13), to date, this atlas has not yet been used to examine specific regional imaging differences as they relate to speech motor deficits in ALS.
A single-center cross-sectional 3T MRI study of forty individuals with ALS was conducted at Massachusetts General Hospital (MGH) in Boston, USA. The study was approved by the institutional review board, and all eligible participants provided written informed consent before joining the study.
Forty individuals diagnosed with ALS and 22 sex- and age-matched healthy controls were pooled from a larger prospective multimodal neuroimaging study cohort previously published by our group (14) between May 22, 2012 and May 24, 2017. Diagnosis of possible or probable ALS was made based on the El Escorial criteria (15). Participants were excluded if they were diagnosed with other motor neuron diseases including primary lateral sclerosis, hereditary spastic paraplegia, and pure LMN phenotypes; had incomplete or missing clinical data; or did not pass MRI scan data quality check due to motion artifacts or an incomplete scan.
Standard ALS clinical assessments included participant demographics, ALS disease history, and the revised ALS Functional Rating Scale (ALSFRS-R) (16). The disease progression pre-slope, delta (Δ)ALSFRS-R was defined as [(48-ALSFRS-R score at scan)/disease duration (in months)] (16).
The ALSFRS-R speech item subscore (0–4) was used to dichotomize ALS participants into those with preserved speech (ps-ALS; ALSFRS-R speech item score = 4) and those with deteriorated speech (ds-ALS; ALSFRS-R speech item score < 4). The latter group includes individuals who reported one of the following on the ALSFRS-R speech item: detectable speech disturbance (score = 3), intelligible speech with repeating (score = 2), speech combined with nonvocal communications (score = 1), or loss of useful communication (score = 0). While all 18 participants in the ps-ALS group had preserved speech function, four participants endorsed other bulbar symptoms (1 participant rated swallow/dysphagia 2 points out of 4, and 3 participants rated drooling/sialorrhea 3 out of 4 points). All 22 participants in the ds-ALS group reported bulbar dysfunction in multiple bulbar functions (16 participants rated swallow/dysphagia 2 or 3 points out of 4, and 15 participants rated drooling/sialorrhea 0, 1, 2, or 3 out of 4). Dichotomization based on ALSRFRS-R score is a commonly used method for grouping ALS participants (17). Detailed demographic information of the participants is displayed in Table 1.
Six participants with ALS were co-enrolled in a quantitative and qualitative speech assessment case study conducted by two licensed speech-language pathologists (SLPs). These participants were selected given the data we had available with speech samples. For the quantitative speech assessment, speaking rate was used given its role as one of the most widely used objective measures of bulbar impairment (18). Speaking rate was indexed by the number of words produced per minute during the Sentence Intelligibility Test (SIT), which consists of 11 randomly-generated sentences that vary from 5 to 15 words in length (19). Recent work has demonstrated an average of 198.55 (SD = 21.80) words produced per minute for typical speakers during the SIT (20). For the qualitative speech assessment, two experienced SLPs, who were blinded to imaging findings and ALSFRS-R scores, independently rated overall speech severity using a five-point equal interval scale (i.e., normal, mild, moderate, severe, and profound) and described each participant's speech based on the 38 perceptual features in the Darley, Aronson, and Brown paradigm (2, 3). The full list of features is reported in the Supplementary material.
All eligible participants underwent a brain scan at the Martinos Center for Biomedical Imaging in Boston with Siemens 3 Tesla TrioTim MRI scanner equipped with an 8-channel head coil. The images were acquired using a multiple gradient echo 3-dimensional (3D) magnetization-prepared rapid acquisition (ME-MPRAGE) scan (multi-echo time TEs = [1.63, 3.49, 5.35, 7.21]; repetition time TR = 1,200; flip angle = 7 degrees; field of view FOV = 280; slice orientation = PSL; and 1 mm isotropic voxel).
Automated segmentation of cortical and subcortical structures was performed with the FreeSurfer version 6.0.26 image analysis pipeline (21). Each structural image underwent spatial and intensity normalization, skull stripping, 10-mm spatial smoothing, Talairach transformation, surface extraction, spherical registration, and cortical parcellation. The model was fitted to every vertex using Freesurfer's mri_glmfit function. Cortical thickness was then measured from the distance between the gray-white boundary and the pial surface across the entire cortical surface. The generated masks of all participants were inspected individually for accurate cortical measurement. Data were registered to FreeSurfer's standard space (fsaverage space) for conducting higher-level group analyses.
Two approaches were used to measure cortical thinning: (1) whole-brain surface-based analyses with cluster correction, which is a powerful approach for quantifying group-level structural differences between HCs vs. each of the ALS groups (ps-ALS and ds-ALS); and (2) ROI analyses with family-wise error correction, which we used to investigate potential clinical phenotypes (as indexed by brain changes) in participants with ALS (ps-ALS and ds-ALS).
To investigate the brain regions associated with speech function in participants with ALS, 16 regions of interest (ROIs) were defined apriori. The selection of the ROIs was based on the involvement in speech motor control in healthy individuals and individuals with other neurodegenerative disorders as demonstrated in prior MRI and fMRI studies (11, 22). The complete Speech Label Atlas parcellate each cortical hemisphere into 64 ROIs, including a fine-grained subdivision of regions relevant to speech motor control (23). The atlas space for the extraction of the cortical thickness ROIs followed the pipeline developed by Tourville and colleagues (10).
Whole-brain surface-based analyses were performed to detect group-level differences in cortical thickness. To correct for multiple testing, we applied a cluster-wise correction with a cluster-forming threshold of 1.3 (p < 0.05) and a cluster-wise threshold of p = 0.05. A general linear model (GLM) was used for pairwise comparisons between ps-ALS vs. HC groups, and ds-ALS vs. HC groups. Age and sex were added to the model as covariates. We compared each ALS group (ps-ALS and ds-ALS) to controls separately to explore the specific effects of speech performance and cortical thickness in persons with ALS.
Region of interest (ROI) analysis was performed using a linear regression model to detect group-level differences (ps-ALS vs. HCs, and ds-ALS vs. HC) in 16 cortical thickness ROIs. Age and sex were added to the model as covariates. To correct for multiple comparisons, we used Romano-Wolf Multiple Hypothesis Correction step-down adjusted p-values with 100 permutations. The uncorrected and corrected p-values of the ROI analyses are reported in the Supplementary material.
For the case study speech analyses for the six pre-identified ALS participants, a whole-brain surface-based analysis was used in a singleton approach by comparing each participant's image against HCs (n = 22). To correct for multiple comparisons, we applied a cluster-wise correction with a cluster-forming threshold of 1.3 (p < 0.05) and a cluster-wise threshold of p = 0.05. The cluster-forming threshold was obtained from the z-score sampling distribution of the largest null hypothesis cluster size, and the cluster-wise p-value was computed based on the number of clusters that exceeded the threshold. Age and sex were added to the model as covariates.
No group differences were observed in baseline demographic characteristics. There were no significant differences between the ps-ALS and ds-ALS groups in their disease characteristics, except region of disease onset. The ds-ALS group had significantly more individuals with bulbar onset, as would be expected from the presence of speech deterioration. All participants exhibited a slow rate of progression, except for two participants in the ds-ALS group (ALSFRS-R pre-slope of >-2 points/month). The ds-ALS group had a significantly higher proportion of bulbar onset and lower bulbar ALSFRS-R subscores compared to the ps-ALS group (Table 1).
Among all brain regions analyzed on whole-brain surface-based analyses, the ds-ALS group showed significant (p < 0.05) cortical thinning compared to HCs, surviving multiple comparisons, in left pre-central cortex (or primary motor cortex), left post-central cortex (or primary somatosensory cortex), and right inferior parietal lobe with its adjacent lateral occipital cortical regions (Figure 1). There were no detectable differences in cortical thinning between the ps-ALS group and HCs.
Figure 1. Whole-brain surface-based analysis depicting significant cortical thinning in ds-ALS group compared to HCs in left primary motor and somatosensory cortices (peak MNI coordinates X = −59.9, Y = −14.4, Z = 32.4) (A) [see anatomical subdivisions in (C)] and right inferior parietal lobe with its adjacent lateral occipital cortical regions (peak MNI coordinates X = 49.5, Y = −57.3, Z = 18.2) (B) [see anatomical subdivisions in Panel (D)]. Values indicated on the color bar are –log(p). ds-ALS, deteriorated speech in amyotrophic lateral sclerosis; HCs, healthy controls; RH, right hemisphere; LH, left hemisphere.
Compared to HCs, the ds-ALS group showed significant cortical thinning in right posterior dorsal premotor cortex, left anterior cingulate cortex, and right and left middle motor cortices after adjusting for multiple comparisons (Figure 2). The ps-ALS group, in contrast, showed no regional differences compared to HCs in any of the 16 ROIs after multiple comparison testing.
Figure 2. ROI analysis with anatomical maps depicting significant cortical thinning in ds-ALS group compared to HCs in right posterior dorsal premotor cortex (A), left anterior cingulate gyrus (B), right middle motor cortex (C), and left middle motor cortex (D). ROI, region of interest; ds-ALS, deteriorated speech in amyotrophic lateral sclerosis; HCs, healthy controls; RH, right hemisphere; LH, left hemisphere.
Qualitative and quantitative speech phenotyping was performed at a matching timepoint to the scan for six ALS participants (Table 2). Four of the six participants experienced speech difficulties as reported by the ALSFRS-R as well as by the SLP-administered speech assessments (Table 2).
Based on the qualitative assessment conducted by two SLPs, two of the four participants with speech difficulties (#4 and #5) exhibited predominantly spastic dysarthria symptoms characterized by slow speaking rate on the SIT and strained quality voice; one (#4) also exhibited mild combined UMN-LMN features characterized by articulatory imprecision. The remaining two participants with speech difficulties (#1 and #3) exhibited predominantly flaccid dysarthria speech patterns; one (#1) presented with only articulatory imprecision, while the other (#3) presented with articulatory imprecision, hypernasality, and breathy voice, all of which are characteristic of flaccid dysarthria. Participants #2 and #6 did not exhibit speech symptoms.
Cortical thinning was observed in two of the four participants with speech difficulties and one of the participants with no speech difficulties (Figure 3). Participant #4 (spastic-dominant) had significant cortical thinning in the right precentral, right superior frontal, and right caudal middle frontal regions, which are involved in speech planning and initiation (Figure 3A) and the right superior parietal, precuneus, and cuneus regions, which are involved in speech motor perception and rhythm regulation (Figure 3B) (54). Participant #5 (spastic-dominant) had significant cortical thinning in the right precuneus and isthmus cingulate (Figure 3C). Participant #6 (no clinical speech deficits) had significant cortical thinning in the left supramarginal (inferior parietal) and superior temporal regions. Neither participants with flaccid-dominant dysarthria (#1 and #3) nor participant #2 (no clinical speech deficits) had significant cortical thinning compared to HCs.
Figure 3. Whole-brain surface-based analysis depicting significant cortical thinning in the ALS participants included in the case study compared to HCs. Participant #4, who presented with moderate to severe speech disturbances and predominant spastic dysarthria, exhibited cortical thinning in right precentral, right superior frontal, and right caudal middle frontal regions (A) and in right superior parietal, precuneus, and cuneus regions (B). Participant #5, who presented with severe speech disturbances and predominant spastic dysarthria, exhibited cortical thinning in right precuneus and isthmus cingulate (C). Participant #6, who presented with no speech disturbances, exhibited cortical thinning in left supramarginal (inferior parietal) and superior temporal regions (D). Values indicated on the color bar are –log(p). ALS, amyotrophic lateral sclerosis; HCs, healthy controls; RH, right hemisphere; LH, left hemisphere.
Based on the quantitative speech rate analyses, we found a large effect size difference (Cohen's d = 1.41) between the three ALS participants (#4, #5, and #6) with cortical thinning in speech areas and the three ALS participants (#1, #2, and #3) without cortical thinning changes in speech areas on singleton analyses against pooled HCs (Figure 4). Due to our small sample size, we did not examine statistical differences between the groups.
Figure 4. Speaking rate of the ALS participants included in the case study analysis based on presence of cortical thinning (participants #4, #5, and #6) or absence of cortical thinning (participants #1, #2, and #3). ALS, amyotrophic lateral sclerosis.
Our findings provide early insights into the multifocal involvement of neocortical brain regions (beyond primary motor cortices) in speech dysfunction in individuals with ALS. Compared to HCs, the ds-ALS group exhibited cortical thinning in multiple specific neocortical regions known to be involved in speech motor production, while the ps-ALS group exhibited no changes in cortical thickness in any speech-related regions. The group-level whole-brain surface-based analyses revealed significant cortical thinning in only the ds-ALS group compared to HCs in (1) left primary motor and somatosensory cortices and (2) right inferior parietal lobe with its adjacent lateral occipital cortical regions. Among 16 prespecified speech motor control ROIs analyzed, cortical thinning in the ds-ALS group was observed in (1) bilateral middle motor cortices, (2) right posterior dorsal premotor cortex, and (3) left anterior cingulate cortex.
Our group-level whole-brain surface-based results revealed significant cortical thinning in left primary motor and somatosensory cortices in the ds-ALS group. This finding aligns with previously published in vivo neuroimaging and post-mortem studies in ALS (24, 25) and with those of Ferrea et al. (26), who found cortical thinning in the bulbar subregion of the precentral gyri in UMN-predominant ALS participants. The primary motor cortex is responsible for precise control and coordination of speech movements (27), and our results suggest a higher yield of focal cortical thinning in the somatotopically relevant facial-tongue-lip-laryngeal homuncular subregions of the sensorimotor cortex. Given that accurate and well-timed articulatory movements are critical for producing intelligible and fluent speech, cortical thinning in this region may contribute to well-documented deficits in intelligibility in speakers with ALS (28). While thinning in the motor cortex is an expected finding, deficits in the somatosensory cortex are less expected in the ALS population, as it has been long referred to as a “pure motor neuron disease.” Yet our finding of thinning in somatosensory cortex aligns with postmortem studies showing that in later stages of ALS, the pTDP43 burden spreads beyond the precentral gyrus to the postcentral gyrus and other neocortical regions including the cingulate gyrus (29–31). Furthermore, a growing amount of clinical evidence has supported the coexistence of sensory disorders in ALS (32, 33). This work highlights that the disease biology in ALS is multifocal and that the circuitries between the bulbar subregion of the primary motor and somatosensory cortices are anatomically and functionally connected.
In the right hemisphere, our group-level whole-brain surfaced-based results revealed thinning in inferior parietal lobe and lateral occipital lobe. Anatomically, there is no clear sulcal demarcation between the inferior parietal and the adjacent lateral occipital regions on the lateral cortical surface. It is, therefore, likely that there may be histological and functional overlaps between these two adjacent regions forming a composite region rather than interpreting these signals as arising from two functionally discrete regions. Previous studies investigating ALS have shown cortical thinning in the left homologs of these regions, which have been implicated in sensorimotor integration and identifying postures and gestures (26, 34, 35). Similarly, fMRI task activation studies have demonstrated the importance of bilateral inferior parietal lobe for integrating spoken and written language (36) and disambiguating speech signals (37).
Our apriori defined ROI analyses showed significant thinning in bilateral middle motor cortices in the ds-ALS group compared to HCs, which have been implicated in speech planning and initiation (27, 38). Difficulties in speech planning have been previously demonstrated in ALS, as prior work found significant more variability in phrase durations during reading compared to HCs (39).
Our ROI analyses also demonstrated cortical thinning in right posterior dorsal premotor cortex in the ds-ALS group. Of note, the premotor cortical area lies anterior to the primary motor cortex and its topographical organization is roughly the same as the primary motor cortex, with the facial-tongue-lips-laryngeal areas located on the dorsal/lateral aspect (40). The bulbar subregion of the premotor cortex has white matter connections with the superior longitudinal fasciculus and consistent connections with the pyramidal tracts (41), which are associated with more complex aspects of speech articulation (42) and motor planning of language function (43). Cortical thinning in these areas in the ds-ALS group may, therefore, underlie articulatory difficulties seen in this population, such as imprecise articulation and slow rate.
Our group-level ROI analyses lastly revealed cortical thinning in anterior cingulum gyrus in the ds-ALS group. Seminal work by Jürgens and Ploog (44) highlighted the role of anterior cingulum gyrus in the motivation to vocalize (or volitional speech initiation) in monkeys. More recent work conducted in humans similarly implicated the role of anterior cingulate cortex in modulating motor behavior during different affective states or levels of motivation (45). While the current study did not address motivation or affect in our participants, prior work has documented a lack of motivation to self-generate thoughts in individuals with ALS (46) and impairments in executive functions, emotion processing, and social cognition (47, 48).
Finally, our quantitative speech assessment in the six individuals with ALS included in our case analysis revealed that speaking rate was able to distinguish between those with and without cortical thinning. This finding is consistent with prior work reporting speaking rate as a key characteristic differentiating spastic dysarthria (relating to cortical/UMN deficits) from flaccid dysarthria (relating to LMN deficits) (49). While several speech characteristics, such as imprecision, are common in both spastic and flaccid dysarthria, slow speaking rate has consistently been associated with spastic dysarthria (49). Nevertheless, it is typically the combination of features that ultimately distinguishes between different dysarthria types. Indeed, in addition to imprecision, individuals with spastic dysarthria tend to present with strained voice and slow speaking rate (50). Similarly, individuals with flaccid dysarthria tend to also present with breathy voice and hypernasality (50). This result is consistent with prior work reporting speaking rate as a key characteristic differentiating spastic and flaccid dysarthria (49). Indeed, prior work posits that spastic dysarthria is related to cortical/UMN deficits, which contributes to speech characteristics such as strained voice and slow speaking rate (50). In contrast, flaccid dysarthria is related to LMN deficits, which contributes to speech characteristics such as imprecision, breathy voice, and hypernasality (50). Interestingly, our qualitative speech assessment found that two of the three individuals with cortical thinning (or potential UMN deficits) presented with spastic-dominant speech characteristics, while two of the three individuals with no cortical thinning (or potential LMN deficits) presented with flaccid-dominant speech characteristics. These results should be interpreted with caution, however, and they were based on a small case analysis.
Our study has limitations that are acknowledge here. The small sample size limits the generalizability of our findings. A larger sample size would provide more robust data and allow for more comprehensive statistical analyses, potentially revealing significant differences that our current study could not detect. For example, the small sample size might have contributed to our finding of no significant differences in cortical thinning between the ps-ALS group and HCs, as prior research has demonstrated such differences (51). However, we believe that the absence of thinning in the cortex in ps-ALS individuals is unclear and could be hypothesized to be multifactorial and may be explained by unanticipated sampling bias of participants showing either absence of UMN pathology and the presence of brainstem and spinal cord pathology only, or the microscopic reduction of Betz cells that is early and not easily visualized using current MRI morphology techniques. The authors acknowledge that using ALSFRS-R to define the ds-ALS and ps-ALS subgroups is a limitation of this study but that this is a preliminary data generating study. Future studies in a larger and longitudinal ALS cohort with deep phenotyping of speech, integrating more specific and sensitive tools for dysarthria assessment (e.g., voice signal analysis, artificial intelligence, precision medicine) would be logical expansion of this study as next steps (52). The small sample size introduces risk of unintended sampling bias and limits the generalizability of our findings. A larger and longitudinal sample would provide more robust data and allow for more comprehensive statistical analyses, potentially revealing significant differences that our current study could not detect. We did not conduct comparative analyses between ps-ALS and ds-ALS because of the small sample size and the pre-test risk of anatomical abnormalities in brain regions in both groups that could cancel some of the positive findings and confound the interpretation of results.
Overall, our findings provide evidence of the involvement of multiple cortical regions in speech deterioration in individuals with ALS, which is consistent with recent fMRI studies in healthy adults and clinical populations demonstrating the involvement of several neocortical pathways beyond the primary speech motor circuit system in speech production. A recent review by Riancho et al. (53) stated, “The elucidation of these ‘non-motor' systems involvement, which might also be part of the degeneration process, should prompt the scientific community to re-consider ALS as a pure motor neuron disease, which may in turn result in more holistic research approaches.” Genotypic information and other confounding variables, such as mood and cognition, should also be considered for all participants. Deep phenotyping of speech abnormalities in future longitudinal early and presymptomatic ALS cohorts will further our understanding of the multiregional spreading pattern of ALS and its association with speech deterioration.
The raw data supporting the conclusions of this article will be made available by the authors, without undue reservation.
The studies involving humans were approved by Mass General Brigham Human Research Office/Institutional Review Board. The studies were conducted in accordance with the local legislation and institutional requirements. The participants provided their written informed consent to participate in this study. Written informed consent was obtained from the individual(s) for the publication of any potentially identifiable images or data included in this article.
AZ: Conceptualization, Formal analysis, Methodology, Writing – original draft, Writing – review & editing. MM: Data curation, Writing – review & editing. HR: Formal analysis, Writing – review & editing. ME: Writing – review & editing. C-ET: Writing – review & editing. JC: Writing – review & editing. NZ: Writing – review & editing. JH: Writing – review & editing. AL: Writing – review & editing. MK: Writing – review & editing. RG: Writing – review & editing. JG: Funding acquisition, Methodology, Resources, Writing – review & editing. SB: Conceptualization, Investigation, Methodology, Resources, Supervision, Funding acquisition, Writing – review & editing.
The authors declare financial support was received for the research, authorship, and/or publication of this article. All funding sources related to each author: AZ—NIDCD R01DC017291-1, MIMH 5T32MH016259-42; HR—T32DC013017-07; Eshghi-NICDC K23DC019179-01; RG—NIDCD R21 DC016664, NIDCD R01 DC017291, NIDCD R01 DC013547, NIDCD K24 DC016312; SB—NINDS 1UF1NS131791-01, 1U01NS136020-01, 1U01NS136021-01, 1OT2NS136938-01, AANEM Foundation, American Academy of Neurology, ALS Association, Muscular Dystrophy Association, Byrne and Pape Family Foundation and Chuck & Terry Abdalian's family philanthropic support.
We thank all the participants and their caregivers for their selfless participation and support for this observational study. Our sincere gratitude to Dr. Nazem Atassi for providing the imaging dataset used in this study. We also thank Dr. Marco Loggia for his insights and suggestions on imaging analyses, the Martinos Center technical support and staff involved in this project, and the clinical research coordinators and study staff at the MGH Healey & AMG Center for ALS.
The authors declare that the research was conducted in the absence of any commercial or financial relationships that could be construed as a potential conflict of interest.
All claims expressed in this article are solely those of the authors and do not necessarily represent those of their affiliated organizations, or those of the publisher, the editors and the reviewers. Any product that may be evaluated in this article, or claim that may be made by its manufacturer, is not guaranteed or endorsed by the publisher.
The Supplementary Material for this article can be found online at: https://www.frontiersin.org/articles/10.3389/fneur.2024.1451177/full#supplementary-material
1. Hartelius L, Elmberg M, Holm R, Lövberg AS, Nikolaidis S. Living with dysarthria: evaluation of a self-report questionnaire. Folia Phoniatr Logop. (2008) 60:11–9. doi: 10.1159/000111799
2. Darley FL, Aronson AE, Brown JR. Clusters of deviant speech dimensions in the dysarthrias. J Speech Hear Res. (1969) 12:462–96. doi: 10.1044/jshr.1203.462
3. Darley FL, Aronson AE, Brown JR. Differential diagnostic patterns of dysarthria. J Speech Hear Res. (1969) 12:246–69. doi: 10.1044/jshr.1202.246
4. Schuster C, Kasper E, Dyrba M, Machts J, Bittner D, Kaufmann J, et al. Cortical thinning and its relation to cognition in amyotrophic lateral sclerosis. Neurobiol Aging. (2014) 35:240–6. doi: 10.1016/j.neurobiolaging.2013.07.020
5. de Marco M, Merico A, Berta G, Segato N, Citton V, Baglione A, et al. Morphometric correlates of dysarthric deficit in amyotrophic lateral sclerosis. Amyotroph Lateral Scler Frontotemporal Degener. (2015) 16:464–72. doi: 10.3109/21678421.2015.1056191
6. Christidi F, Karavasilis E, Riederer F, Zalonis I, Ferentinos P, Velonakis G, et al. Gray matter and white matter changes in non-demented amyotrophic lateral sclerosis patients with or without cognitive impairment: a combined voxel-based morphometry and tract-based spatial statistics whole-brain analysis. Brain Imaging Behav. (2018) 12:547–63. doi: 10.1007/s11682-017-9722-y
7. Shellikeri S, Myers M, Black SE, Abrahao A, Zinman L, Yunusova Y, et al. Speech network regional involvement in bulbar ALS: a multimodal structural MRI study. Amyotroph Lateral Scler Frontotemporal Degener. (2019) 20:385–95. doi: 10.1080/21678421.2019.1612920
8. Schuster C, Kasper E, Machts J, Bittner D, Kaufmann J, Benecke R, et al. Focal thinning of the motor cortex mirrors clinical features of amyotrophic lateral sclerosis and their phenotypes: a neuroimaging study. J Neurol. (2013) 260:2856–64. doi: 10.1007/s00415-013-7083-z
9. Nitert AD, Tan HHG, Walhout R, Knijnenburg NL, van Es MA, Veldink JH, et al. Sensitivity of brain MRI and neurological examination for detection of upper motor neurone degeneration in amyotrophic lateral sclerosis. J Neurol Neurosurg Psychiatry. (2022) 93:82–92. doi: 10.1136/jnnp-2021-327269
10. Tourville JA, Nieto-Castañón A, Heyne M, Guenther FH. Functional parcellation of the speech production cortex. J Speech Lang Hear Res. (2019) 62:3055–70. doi: 10.1044/2019_JSLHR-S-CSMC7-18-0442
11. Cordella C, Quimby M, Touroutoglou A, Brickhouse M, Dickerson BC, Green JR, et al. Quantification of motor speech impairment and its anatomic basis in primary progressive aphasia. Neurology. (2019) 92:E1992–2004. doi: 10.1212/WNL.0000000000007367
12. Peeva MG, Tourville JA, Agam Y, Holland B, Manoach DS, Guenther FH, et al. White matter impairment in the speech network of individuals with autism spectrum disorder. Neuroimage Clin. (2013) 3:234–41. doi: 10.1016/j.nicl.2013.08.011
13. Cai S, Beal DS, Ghosh SS, Guenther FH, Perkell JS. Impaired timing adjustments in response to time-varying auditory perturbation during connected speech production in persons who stutter. Brain Lang. (2014) 129:24–9. doi: 10.1016/j.bandl.2014.01.002
14. Zürcher NR, Loggia ML, Lawson R, Chonde DB, Izquierdo-Garcia D, Yasek JE, et al. Increased in vivo glial activation in patients with amyotrophic lateral sclerosis: Assessed with [11C]-PBR28. Neuroimage Clin. (2015) 7:409–14. doi: 10.1016/j.nicl.2015.01.009
15. Brooks BR, Miller RG, Swash M, Munsat TL. El Escorial revisited: revised criteria for the diagnosis of amyotrophic lateral sclerosis. Amyotroph Lateral Scler Other Motor Neuron Disord. (2000) 1:293–99. doi: 10.1080/146608200300079536
16. Cedarbaum JM, Stambler N, Malta E, Fuller C, Hilt D, Thurmond B, et al. The ALSFRS-R: a revised ALS functional rating scale that incorporates assessments of respiratory function. J Neurol Sci. (1999) 169:13–21. doi: 10.1016/S0022-510X(99)00210-5
17. Barp A, Lizio A, Gerardi F, Tarlarini C, Mauro L, Sansone VA, et al. Neurophysiological indices in amyotrophic lateral sclerosis correlate with functional outcome measures, staging and disease progression. Clin Neurophysiol. (2021) 132:1564–71. doi: 10.1016/j.clinph.2021.02.394
18. Yunusova Y, Plowman EK, Green JR, Barnett C, Bede P. Clinical measures of bulbar dysfunction in ALS. Front Neurol. (2019) 10:106. doi: 10.3389/fneur.2019.00106
19. Yorkston K, Beukelman D, Hakel M. Speech Intelligibility Test for Windows. Lincoln: Copyright Communication Disorders Software (1996), p. 1–34.
20. Barnett C, Green JR, Marzouqah R, Stipancic KL, Berry JD, Korngut L, et al. Reliability and validity of speech and pause measures during passage reading in ALS. Amyotroph Lateral Scler Front Degener. (2020) 21:42–50. doi: 10.1080/21678421.2019.1697888
21. Dale AM, Fischl B, Sereno MI. Cortical surface-based analysis. Neuroimage. (1999) 9:179–94. doi: 10.1006/nimg.1998.0395
22. Golfinopoulos E, Tourville JA, Guenther FH. The integration of large-scale neural network modeling and functional brain imaging in speech motor control. Neuroimage. (2010) 52:862–74. doi: 10.1016/j.neuroimage.2009.10.023
23. Klein A, Tourville J. 101 labeled brain images and a consistent human cortical labeling protocol. Front Neurosci. (2012) 6:171. doi: 10.3389/fnins.2012.00171
24. Agosta F, Valsasina P, Riva N, Copetti M, Messina MJ, Prelle A, et al. The cortical signature of amyotrophic lateral sclerosis. PLoS ONE. (2012) 7:e42816. doi: 10.1371/journal.pone.0042816
25. Bowen BC, Pattany PM, Bradley WG, Murdoch JB, Rotta F, Younis AA, et al. MR imaging and localized proton spectroscopy of the precentral gyrus in amyotrophic lateral sclerosis. AJNR Am J Neuroradiol. (2000) 21:647–58.
26. Ferrea S, Junker F, Korth M, Gruhn K, Grehl T, Schmidt-wilcke T. Cortical thinning of motor and non-motor brain regions enables diagnosis of amyotrophic lateral sclerosis and supports distinction between upper-and lower-motoneuron phenotypes. Biomedicines. (2021) 9:1195. doi: 10.3390/biomedicines9091195
27. Guenther FH. Neural Control of Speech. Cambridge, MA: MIT Press (2016). doi: 10.7551/mitpress/10471.001.0001
28. Kent RD, Kent JF, Weismer G, Sufit RL, Rosenbek JC, Martin RE, et al. Impairment of speech intelligibility in men with amyotrophic lateral sclerosis. J Speech Hear Disord. (1990) 55:721–8. doi: 10.1044/jshd.5504.721
29. Braak H, Brettschneider J, Ludolph AC, Lee VM, Trojanowski JQ, Tredici K, et al. Amyotrophic lateral sclerosis—a model of corticofugal axonal spread. Nat Rev Neurol. (2013) 9:708–14. doi: 10.1038/nrneurol.2013.221
30. Eisen A, Braak H. del Tredici K, Lemon R, Ludolph AC, Kiernan MC. Cortical influences drive amyotrophic lateral sclerosis. J Neurol Neurosurg Psychiatry. (2017) 88:917–24. doi: 10.1136/jnnp-2017-315573
31. Brettschneider J, Arai K., del Tredici K, Toledo JB, Robinson JL, Lee EB, et al. TDP-43 pathology and neuronal loss in amyotrophic lateral sclerosis spinal cord. Acta Neuropathol. (2014) 128:423–37. doi: 10.1007/s00401-014-1299-6
32. Chio A, Moglia C, Canosa A, Manera U, Vasta R, Brunetti M, et al. Cognitive impairment across ALS clinical stages in a population-based cohort. Neurology. (2019) 93:e984–94. doi: 10.1212/WNL.0000000000008063
33. Hammad M, Silva A, Glass J, Sladky JT. Clinical, electrophysiologic, and pathologic evidence for sensory abnormalities in ALS. Neurology. (2007) 69:2236–42. doi: 10.1212/01.wnl.0000286948.99150.16
34. Chipika RH, Mulkerrin G, Murad A, Lope J, Hardiman O, Bede P, et al. Alterations in somatosensory, visual and auditory pathways in amyotrophic lateral sclerosis: an under-recognised facet of ALS. J Integr Neurosci. (2022) 21:88. doi: 10.31083/j.jin2103088
35. Shum M, Shiller DM, Baum SR, Gracco VL. Sensorimotor integration for speech motor learning involves the inferior parietal cortex. Eur J Neurosci. (2011) 34:1817–22. doi: 10.1111/j.1460-9568.2011.07889.x
36. Kilian-Hütten N, Vroomen J, Formisano E. Brain activation during audiovisual exposure anticipates future perception of ambiguous speech. Neuroimage. (2011) 57:1601–7. doi: 10.1016/j.neuroimage.2011.05.043
37. Cusack R. The intraparietal sulcus and perceptual organization. J Cogn Neurosci. (2005) 17:641–51. doi: 10.1162/0898929053467541
38. Hertrich I, Dietrich S, Ackermann H. The role of the supplementary motor area for speech and language processing. Neurosci Biobehav Rev. (2016) 68:602–10. doi: 10.1016/j.neubiorev.2016.06.030
39. Yunusova Y, Graham NL, Shellikeri S, Phuong K, Kulkarni M, Rochon E, et al. Profiling speech and pausing in amyotrophic lateral sclerosis (ALS) and frontotemporal dementia (FTD). PLoS ONE. (2016) 11:e0147573. doi: 10.1371/journal.pone.0147573
40. Guyton AC, Hall JE. Cerebral cortex, intellectual functions of the brain, learning, and memory. In: Guyton AC, Hall JE, , editors. Guyton ad Hall Textbook of Medical Physiology, 12th Edn. Philadelphia, PA: Saunders Elsevier (2011), p. 697–709.
41. Kamali A, Flanders AE, Brody J, Hunter J, Hasan KM. Tracing superior longitudinal fasciculus connectivity in the human brain using high resolution diffusion tensor tractography. Brain Struct Funct. (2014) 219:269–81. doi: 10.1007/s00429-012-0498-y
42. Makris N, Kennedy DN, McInerney S, Sorensen AG, Wang R, Caviness VS, et al. Segmentation of subcomponents within the superior longitudinal fascicle in humans: a quantitative, in vivo, DT-MRI study. Cereb Cortex. (2005) 15:854–69. doi: 10.1093/cercor/bhh186
43. Wang X, Pathak S, Stefaneanu L, Yeh FC, Li S, Fernandez-Miranda JC, et al. Subcomponents and connectivity of the superior longitudinal fasciculus in the human brain. Brain Struct Funct. (2016) 221:2075–92. doi: 10.1007/s00429-015-1028-5
44. Jürgens U, Ploog D. Cerebral representation of vocalization in the squirrel monkey. Exp Brain Res. (1970) 10:532–54. doi: 10.1007/BF00234269
45. Paus T. Primate anterior cingulate cortex: where motor control, drive and cognition interface. Nat Rev Neurosci. (2001) 2:417–24. doi: 10.1038/35077500
46. Radakovic R, Stephenson L, Newton J, Crockford C, Swingler R, Chandran S, et al. Multidimensional apathy and executive dysfunction in amyotrophic lateral sclerosis. Cortex J Devoted Study Nerv Syst Behav. (2017) 94:142–51. doi: 10.1016/j.cortex.2017.06.023
47. Abrahams S, Leigh PN, Harvey A, Vythelingum GN, Grisé D, Goldstein LH, et al. Verbal fluency and executive dysfunction in amyotrophic lateral sclerosis (ALS). Neuropsychologia. (2000) 38:734–47. doi: 10.1016/S0028-3932(99)00146-3
48. Murphy J, Henry R, Lomen-Hoerth C. Establishing subtypes of the continuum of frontal lobe impairment in amyotrophic lateral sclerosis. Arch Neurol. (2007) 64:330–4. doi: 10.1001/archneur.64.3.330
49. Duffy JR. Motor Speech Disorders Substrates, Differential Diagnosis, and Management, 4th Edn. Amsterdam: Elsevier (2019).
50. Langmore SE, Lehman ME. Physiologic deficits in the orofacial system underlying dysarthria in amyotrophic lateral sclerosis. J Speech Lang Hear Res. (1994) 37:28–37. doi: 10.1044/jshr.3701.28
51. Agosta F, Spinelli EG, Filippi M. Neuroimaging in amyotrophic lateral sclerosis: current and emerging uses. Expert Rev Neurother. (2018) 18:395–406. doi: 10.1080/14737175.2018.1463160
52. Dubbioso R, Iannotti FA, Senerchia G, Verde R, Iuzzolino VV, Spisto M, et al. Voice signals database of ALS patients with different dysarthria severity and healthy controls. Sci Data. (2024) 11:800. doi: 10.1038/s41597-024-03597-2
53. Riancho J, Paz-Fajardo L, López de Munaín A. Clinical and preclinical evidence of somatosensory involvement in amyo-trophic lateral sclerosis. Br J Pharmacol. (2020) 178:1257–68. doi: 10.1111/bph.15202
54. Sitek KR, Cai S, Beal DS, Perkell JS, Guenther FH, Ghosh SS. Decreased cerebellar-orbitofrontal connectivity correlates with stuttering severity: whole-brain functional and structural connectivity associations with persistent developmental stuttering. Front Hum Neurosci. (2016) 10:190. doi: 10.3389/fnhum.2016.00190
Keywords: amyotrophic lateral sclerosis (ALS), speech motor control, speech impairment, MRI, neurodegeneration, dysarthria, brain atrophy
Citation: Zaninotto AL, Makary MM, Rowe HP, Eshghi M, Tseng C-EJ, Chan J, Zürcher NR, Hooker J, Lewis A, Keegan M, Gifford RF, Green JR and Babu S (2024) Speech motor impairment in ALS is associated with multiregional cortical thinning beyond primary motor cortex. Front. Neurol. 15:1451177. doi: 10.3389/fneur.2024.1451177
Received: 18 June 2024; Accepted: 20 August 2024;
Published: 01 October 2024.
Edited by:
Raffaele Dubbioso, Federico II University Hospital, ItalyReviewed by:
Valentina Virginia Iuzzolino, University of Naples Federico II, ItalyCopyright © 2024 Zaninotto, Makary, Rowe, Eshghi, Tseng, Chan, Zürcher, Hooker, Lewis, Keegan, Gifford, Green and Babu. This is an open-access article distributed under the terms of the Creative Commons Attribution License (CC BY). The use, distribution or reproduction in other forums is permitted, provided the original author(s) and the copyright owner(s) are credited and that the original publication in this journal is cited, in accordance with accepted academic practice. No use, distribution or reproduction is permitted which does not comply with these terms.
*Correspondence: Suma Babu, c2JhYnVAbWdoLmhhcnZhcmQuZWR1
†Present address: Ana Luiza Zaninotto, Arvinas, New Haven, CT, United States
Disclaimer: All claims expressed in this article are solely those of the authors and do not necessarily represent those of their affiliated organizations, or those of the publisher, the editors and the reviewers. Any product that may be evaluated in this article or claim that may be made by its manufacturer is not guaranteed or endorsed by the publisher.
Research integrity at Frontiers
Learn more about the work of our research integrity team to safeguard the quality of each article we publish.