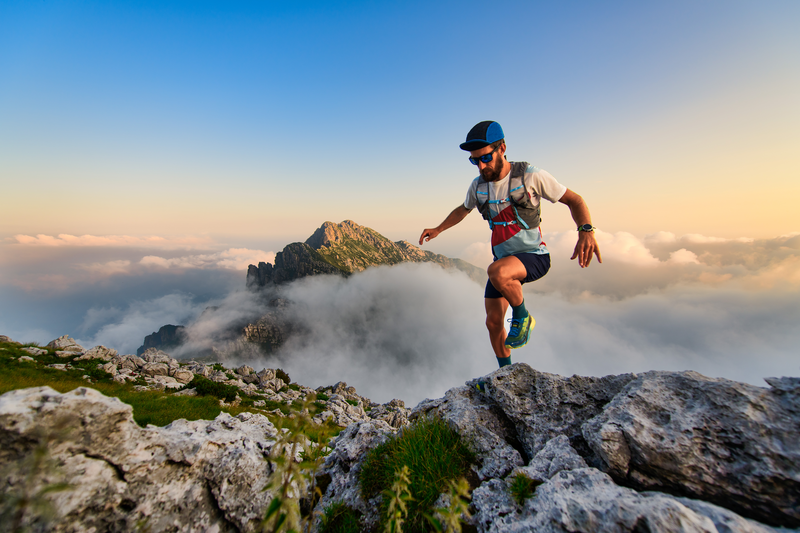
95% of researchers rate our articles as excellent or good
Learn more about the work of our research integrity team to safeguard the quality of each article we publish.
Find out more
HYPOTHESIS AND THEORY article
Front. Neurol. , 27 November 2024
Sec. Autonomic Disorders
Volume 15 - 2024 | https://doi.org/10.3389/fneur.2024.1430390
This article is part of the Research Topic Neurology and Connective Tissue View all 18 articles
Ligamentous cervical instability, especially ligamentous upper cervical instability, can be the missing structural cause and/or co-morbidity for many chronic disabling brain and systemic body symptoms and diagnoses. Due to the forward head-facedown lifestyle from excessive computer and cell phone usage, the posterior ligament complex of the cervical spine undergoes a slow stretch termed “creep” which can, over time, lead to cervical instability and a breakdown of the cervical curve. As this degenerative process continues, the cervical curve straightens and ultimately becomes kyphotic, a process called cervical dysstructure; simultaneously, the atlas (C1) moves forward, both of which can lead to encroachment of the structures in the carotid sheath, especially the internal jugular veins and vagus nerves. This obstruction of fluid flow can account for many brain diseases, and compression and stretch of the vagus nerve for body diseases, including dysautonomia. This article describes the consequences of impaired fluid flow into and out of the brain, especially venous flow through the internal jugular veins, leading to intracranial hypertension (formerly called pseudotumor cerebri). Cervical structural, internal jugular vein, and optic nerve sheath measurements are presented from a retrospective chart review of 227 consecutive patients with no obvious cause for 1 of 8 specific brain or mental health symptoms—anxiety, brain fog, concentration difficulty, depression/hopelessness, headaches, obsessive thoughts, panic attacks, and rumination on traumatic events. A case example is given to demonstrate how cervical structural treatments can open up internal jugular veins and improve a patient’s chronic symptoms.
Many chronic brain disorders are thought to occur primarily because of brain physiology, including brain fog, fatigue, cognitive decline, emotional lability, and mental health conditions such as depression, generalized anxiety disorder, bipolar disorder, personality disorders, depersonalization, and dissociative disorders. There is a mental health crisis in the United States that continues to worsen. The percentage of American adults that suffer from a mental illness has increased from 17.7% (40.7 million people) in 2008 to 20% (57.8 million people) in 2021 (1, 2). According to the World Health Organization, it expects depression, which is the most common mental disorder, to become the largest single healthcare burden by 2030 (3). The meteoric rise of cognitive and mental health disorders may be explained by many factors, but one potential cause could be a breakdown of the cervical neck curve and ligamentous cervical instability due to the forward head-facedown lifestyle (FH-FDL) from poor posture while using electronic devices, leading to impairment of fluid flow out of the brain via compression of the internal jugular veins in the carotid sheath.
The body craves a balance between stability and mobility. When the body is exposed to trauma or chronic low-grade abnormal forces, it leads to ligamentous cervical instability (LCI) and ultimately broken neck structure, termed “cervical dysstructure”: 2 common conditions that are often overlooked or missed by current static supine diagnostic testing methods. Its incidence is increasing with the current ever-prevalent FH-FDL of looking at computers and down at cell phones and tablets. This practice is especially damaging for children, who have a heavier head-to-neck strength ratio than adults. While looking at electronic devices can appear innocuous, it is not so. As the posterior ligamentous complex of the neck stretches, LCI can develop, which is a progressive condition that can lead to cervical dysstructure. Simply put, LCI can lead to a broken neck structure.
LCI may have humble beginnings with prolonged stretching from FH-FDL posture, and the first sign may be neck tightness. If left unchecked, it can progress into neurocatastrophic consequences as the cervical supporting structures weaken and the carotid sheath and/or spinal cord, and the fluids contained within them, become compressed, torqued, and stretched. The 2 most common mechanisms by which severe symptoms arise are venolymphatic drainage compression—leading to intracranial hypertension/increased brain pressure (IBP, formerly referred to in medical literature as pseudotumor cerebri) and alterations of brain function—and cervicovagopathy (cervical-induced vagus nerve injury or signal interference), causing dysautonomia and systemic inflammation, both of which lead to dysfunctional body homeostasis. The most common spinal instability involves the spine’s most mobile segment, the atlantoaxial joint (C1-C2), whose pathophysiology may unlock the cause and solution to neurodegenerative conditions, including dementia.
Mechanical stability of the spine depends on its ability to maintain alignment and provide protection to the neural, vascular, and other structures it encloses during physiological loading so that there is no harm to any of these tissues and no symptoms are produced (4, 5). Clinical ligamentous cervical instability is an inability of the cervical ligaments to maintain individual, adjacent, or global vertebral alignment when subjected to increased forces by various postures, positions, and/or motions that alter bony, soft tissue, and/or neurovascular alignment and function such that symptoms result. LCI causes the bones to move in such a way that destructive forces are placed on adjacent tissues and joints, but also on the myriad vital structures that run through the neck. It is to be distinguished from hypermobility, which entails increased motion of bony and/or soft tissue structures without tissue injury or symptoms.
The potential seriousness of LCI is amplified when one considers that all of the major neurovascular structures from the body that enter and leave the brain and brainstem, including the autonomic ganglia, do so through the neck. The neck is thus a conduit for fluid and nerve flow that run the body through the brain and brainstem. LCI can disrupt this fluid and nerve flow and can explain many resulting chronic symptoms, disorders, syndromes, and diagnoses that plague humanity.
The cervical spine consists of 7 vertebrae and may be considered as 2 distinct regions: upper cervical (C0-C2), also known as the craniocervical junction (CCJ), and lower cervical (C3-C7). The lower cervical spine is connected by intervertebral discs and is thus inherently more stable than the CCJ. The C0-C1 joint is relatively stable, whereas the most mobile section of the spinal column is the C1-C2 joint because of the peg-like structure—the dens of C2—around which the arch of C1 rotates, giving it enormous flexibility. The C1-C2 joint sits between 2 more stable areas (C0-C1 and C2-C7), contributing to its being the most commonly unstable joint in the cervical spine. This precariousness is compounded by the fact that the average head weighs 10–12 pounds and sits on the 2-ounce C1 (atlas).
It is important to remember that ligaments are the fasteners of the bones, the connective tissues that hold adjacent bones together. Most ligaments of the body are capsular ligaments, meaning they are the primary structures of the joint capsule that stabilizes the joint. Ligaments can weaken over time if too much force is put upon them. The degeneration of ligaments (ligamentosis) is common in osteoarthritis (6, 7).
The major cervical ligaments that are overstretched by the FH-FDL in the process known as “creep” are those of the posterior ligament complex (PLC), especially the capsular ligaments of the facet (zygapophyseal) joints, the major joints in the cervical spine. All ligaments posterior to the vertebral body, including the capsular ligaments, ligamentum flavum, posterior longitudinal, interspinous, intertransverse, and supraspinous ligaments, are considered part of the PLC and are stretched during flexion, the very motion in FH-FDL (see Figure 1). Conversely, any ligaments located anterior to the vertebral body, including the anterior longitudinal ligament, are stretched during extension. In regard to the 4 motions of the cervical spine—flexion, extension, axial rotation, and lateral flexion—it is only the capsular ligaments of the facet (apophyseal) joints that inhibit all 4 motions (8, 9). Since the facet joint is the fulcrum of all motions of the cervical spine (except rotation, in which it is the atlanto-dens joint), when the cervical capsular ligaments of the facet joints weaken, it is potentially possible that all neck motions are increased, which becomes evident when motion diagnostic scans are performed.
Figure 1. Forward head posture from hours of computer work and texting, resulting in cervical ligament laxity. “Creep,” which is a term signifying the slow stretching of ligaments, most commonly occurs by a forward head posture from computer work or looking at a smartphone.
The neck, primarily the upper cervical spine, must support the head and keep it balanced and safe at all times, for bodily and brain functions. The CCJ (occiput to C2) is without intervertebral discs and relies primarily on ligamentous stabilizers for support. The atlantoaxial is the most mobile joint in the vertebral column. It is also the most vulnerable to FH-FDL, as well as injury during whiplash and the quick head turning that occurs during head trauma when the skull hits another object (10).
The fulcrum of head rotation is the atlantoaxial joint (C1-C2) (11). The primary ligamentous supports of the lateral facet joints of C1-C2 are the capsular ligaments, whereas the atlanto-dens joint is held together by the transverse ligament and alar ligaments. The C1-C2 atlantoaxial joint provides 50% of cervical spine rotational mobility as the arch of C1 rotates around the dens of C2 (12). When stable, C1-C2 allows some flexion, but almost no lateral flexion. Lateral flexion in this joint is one of the hallmarks of facet atlantoaxial instability. In the medical literature, atlantoaxial instability generally refers to the connection between the atlas and the dens of axis, which can be described as dens atlantoaxial instability. This is an important distinction because dens atlantoaxial instability is more of a surgical lesion, whereas facet atlantoaxial instability can often be treated by conservative measures such as chiropractic care, physiotherapy, and Prolotherapy (13, 14).
Protection against anterior translation of the atlas comes from the capsular ligaments posteriorly and the transverse ligament anteriorly. Capsular ligament injury of C1-C2 results in facet atlantoaxial instability, whereas injury of the transverse and alar ligaments causes dens atlantoaxial instability. Injury to the atlantoaxial capsular ligaments causes a dramatic increase in lateral bending and axial rotation motion (43 and 159%, respectively), whereas transverse ligament disruption significantly increases the anterior atlanto-dens interval (15).
The remaining cervical vertebrae are the more typical vertebrae of the spine, each of which consists of a body, transverse processes and pedicles, and adjoining intervertebral discs. The discs make this part of the cervical spine inherently more stable. As with the CCJ, the fulcrum of motion is at the facet, with the C4-C5 facet joints being the fulcrum for neck flexion (16). The superior and inferior articular processes articulate bilaterally to form the facet joints at each level, which are true synovial joints. The lower cervical segments are chiefly supported by the intervertebral discs, anterior longitudinal ligament, posterior longitudinal ligament, ligamentum flavum, and the facet capsular ligaments (see Figure 2).
Figure 2. Ligament anatomy of the neck. (A) Lateral view. (B) Posterior view. (C) Upper cervical posterior view.
Cervical lordosis is the anterior convexity of the cervical spine from the foramen magnum to the thoracic spine and is caused by its wedge-shaped vertebrae. Cervical lordosis maximizes the spine’s ability to handle forces, maintains global spinal alignment, and supports and balances the head. The ideal sagittal alignment of the cervical spine is lordotic as measured in the sagittal plane by the C2-C7 Cobb angle of 20–35° (17–20). The cervical lordosis depth is normally between 7 and 17 mm as measured by the Borden method (21, 22). This keeps the center of mass of the cranium posterior of the lordotic curve, reducing the forces on the structures in the neck.
The neurovascular and soft tissue support in the neck is best in a relaxed (innate) configuration with minimum tension, which optimizes function. If there is a disruption of cervical lordosis, the axial load (cranial center of motion) shifts anteriorly and will continue to do so until cervical kyphosis results (23). This kyphosis can lead to stretching and lengthening of the spinal cord and other nerves that travel in the neck, including the vagus nerve, affecting nerve impulse conduction (24). This shift in axial load will cause cervical posterior ligamentous creep, further stretching the facet capsular ligaments, explaining why LCI is a progressive disorder.
While there are many causes of cervical ligament injuries, including whiplash and head traumas, by far the most common today is due to the FH-FDL from too much time in flexion (25, 26). This constant head and neck flexion places enormous forces on the neck-supporting structures. A neck flexed at 40° while looking at a cell phone triples the forces at C2 (27). In one study, researchers found that the forces on the cervical nervous tissue increased from 2 pounds per square inch in the neutral posture to 40 pounds per square inch in flexion (28). An adult head weights 10 to 12 pounds in the neutral position. As the head tilts forward, the forces seen by the neck surge progressively up to 60 pounds at 60° (29). With the neck flexed, the maximum load the neck can handle drops drastically (25–50%) (30, 31). Eventually, the neck structure becomes broken, and the person loses their normal lordotic curve.
LCI is a progressive disorder leading to a breakdown of the cervical lordotic curve, a condition we call cervical dysstructure (see Figure 3). Cervical instability causes many symptoms, including cracking, clicking, and grinding in the neck with movement, as well as neck stiffness, muscular tension and weakness (feeling of the head being too heavy), and headache. What is not as well appreciated is that it can potentially cause a host of other disabling symptoms, including vertigo, dizziness, tinnitus, migraine headaches, and brain fog (32). LCI causes human disease by 2 primary mechanisms: it interrupts nerve signals (i.e., spinal cord and vagus nerves) and obstructs fluid flow to and from the brain and body. Following Occam’s razor, we know that the simplest solution is normally the correct one, so for a complicated patient with myriad unexplained symptoms, ligamentous cervical joint instability could be the best explanation (33) (see Figure 4).
Figure 3. Ligamentous cervical instability is a progressive disorder causing a normal lordotic curve to break down, ultimately leading to an “S” or “sigmoid” curve and potentially compressing the structures in the carotid sheath, including the vagus nerves and internal jugular veins.
In the cervical spine, the body will always choose stability over motion to protect the adjacent neurovascular structures. This is why long-term osteophytes form, and eventually, osteoarthritis causes a limiting of motion. When cervical ligament injury is present, the body has 3 main mechanisms to try limiting motion to protect the neurovascular structures: muscle tension or spasms, joint swelling, and osteophyte formation (34). Through the ligament-muscular reflex, muscle spasms protect neurovascular structures when ligament injury is present. When the facet capsular ligaments undergo a noxious stretch, research suggests a strain threshold as low as 10% stretch for activation. The nerve afferents that innervate it are also stretched, triggering neuronal signaling to the central nervous system and many of the 30+ stabilizing cervical muscles, as muscle insertions have been found to cover nearly 23% of the capsule area in the cervical spine (35–37). A hallmark of ligamentous upper cervical instability (LUCI) is tension or tenderness in the suboccipital and posterior neck muscles, as they are activated by the ligament-muscular reflex (38, 39). The neuronal signaling from the ligaments and tight muscles can also cause referral pain to the head and arm.
The degenerative cascade will proceed if the forces continue to exceed the cervical spine’s ability to handle them. As the FH-FDL progresses, so do the LCI and cervical dysstructure. Eventually, the flexion of the lower cervical spinal segments (C2–C7) causes compensatory hyperextension of the suboccipital (C0–C2) to maintain horizontal gaze (40, 41). It is also possible for bony hypertrophy to occur, leading to spinal stenosis and possible myelopathic or radicular symptoms. Other possible consequences of the continual forces and destabilization of the cervical spine are torsion, stretch, and compression of the vital neurovascular structures of the central and autonomic nervous system nerves/ganglia and blood vessels on their way to or from the brain.
The Gestalt of cervical spine destabilization and resultant kyphotic curve and tissue destruction is degenerative disc disease and cervical spondylosis, causing traction on the cervical spinal cord and brainstem, potential stretch and compression of the vagus nerves, and restrictions of the fluid flow into and out of the brain from the carotid and vertebral arteries, internal jugular vein (IJV), and cerebrospinal fluid (CSF) (42–44) (see Figure 5). The detrimental consequences of cervical dysstructure can be many, and include increased forces on the PLC, fixation or fusions of vertebral motion segments, increased forces on vertebral segments that still move, dysfunctional vertebral motions, significant muscle tightness and shortening, altered mechanical tension on neurovascular structures, and narrowing of vital spaces all throughout the neck.
Humans spend the larger part of each day in the upright position, the very position that causes the majority of symptoms, yet most diagnostic tests are performed with the patient in the supine, resting position, the very position that provides relief. Scanning in the upright position can show the brain, brainstem, and cervical spine under the effects of gravity, and its alterations in blood flow, venous drainage, and CSF flow can also be seen while the person is upright. Many times, symptomology occurs with a specific head/neck position, such as flexion. Thus, while scanning the person (preferably during motion) when they are upright or in their symptomatic position, upright x-rays, MRI, or positional computerized tomography (CT) scans may be preferred, as static or supine scan abnormalities often do not correlate with symptoms (45–47). Dynamic MRI in the CCJ was found to be able to detect cases of cord compression that were not seen by static supine MRI (48). In a study involving 1,200 patients, cerebellar tonsillar descent (Chiari) of at least 1.0 mm was 4 times more likely to be diagnosed by an upright MRI vs. one supine (49). Cerebellar tonsillar ectopia was found 2.5 times more often in whiplash patients when an upright MRI was done vs. one that was supine. In this study and others, soft tissue lesions are found in the upper cervical region about 3–5 times more often when an upright (especially with flexion/extension views) vs. recumbent scan is performed (50–53).
Cervical structural analysis in the upright position and/or with motion allows the clinician to see the motion of vertebral bones in real time and discover previously hidden pathology. The 2 modalities that can be utilized for this imaging are digital motion x-ray (DMX) and upright cone beam CT scanning. Digital motion x-ray (videofluoroscopic) examination of the cervical spine has been shown to provide a high degree of diagnostic accuracy for the identification of vertebral instability in patients with chronic pain stemming from whiplash trauma (54). CT scanning of the cervical spine with 3-D reconstruction in various positions, including flexion, extension, and/or rotation, is another way to document cervical instability and misalignments that are missed by more traditional means (55). Cone beam CT scanning can document elongated styloid bones, which can compress the carotid sheath, and thus the IJVs, with head rotation and flexion (see Figure 6).
Figure 6. Common pathology seen by upright cone beam CT scan of head and neck. (A) CO-C1 instability. (B) Cervical spine misalignments. (C) Elongated styloid. (D) Ponticulus posticus.
DMX of the spine allows for a continuous and detailed examination of cervical spinal movement and allows unrestricted assessment of C0-C7 motion in multiple dimensions, including the sagittal, rotational, and frontal planes. DMX studies typically include actively moving the head and neck through protraction, retraction, flexion, extension, rotation, and lateral flexion. They show the functional integrity of the ligaments in the cervical spine, specifically the anterior and posterior longitudinal, supraspinous, interspinous, ligamentum flavum, transverse, alar, and facet capsular ligaments. The analysis of over 8,000 DMXs has found 4 common patterns1 (see Figure 7).
1. Extreme overall instability throughout the cervical spine from patients, typically females, with congenital hypermobility syndromes such as Ehlers-Danlos syndrome.
2. Instability above and below the level of prior surgical fusions or degenerative fusions, the latter being more prevalent in older males where the degeneration fusions (body fused or fixed the area by bridging osteophytes and disc degeneration) are in the lower segments, commonly C5-C7 and the unstable segments above it.
3. Severe upper cervical instability, especially at the C1-C2 level, can occur from a car accident, head trauma, birth trauma, or high-velocity rotational injury.
4. Cervical dysstructure: breakdown of the cervical curve with a very forward C1.
Figure 7. Common cervical digital motion (fluoroscopy) findings. (A) Multilevel cervical instability. (B) Instability above the level of a surgical or degenerative fusion. (C) Upper cervical instability at C1-C2 facet joints. (D) Cervical dysstructure.
Severe anterior spondylolisthesis is generally recognized when horizontal translation is greater than 3.0–3.5 mm (56). While there are radiographic diagnostic criteria for lower cervical instability and extreme cases of upper cervical instability, especially those involving the transverse ligament where neurologic insult is current or imminent and surgery is warranted, the accepted diagnostic criteria for chronic upper cervical instability not requiring surgery are not so clear-cut (57–60). These classification systems are well-suited for medial atlanto-dens instability but do not evaluate in-depth lateral posterior LUCI involving the C0-C1 and C1-C2 facet joints and capsular ligaments. Many of these diagnostic criteria involve non-moving and non-weight-bearing imaging. Upright and motion scanning allows changes to be seen between positions and motions. Cervical ligamentous injury is documented when adjacent vertebral alignments are no longer maintained in certain positions or with certain motions.
Once the diagnosis of LCI, LUCI, or cervical dysstructure has been made by upright/motion radiography, ancillary diagnostic testing is performed to discover which pathophysiology is causing the most serious symptoms. We term the overall composition of pathophysiological tests that we perform the “neck vitals.” These tests include ocular and neck ultrasound, tonometry, pupillometry, and extracranial and transcranial Doppler ultrasound. They are used in conjunction with traditional testing methods such as brain, orbit, and cervical MRI, which typically have findings for conditions such as intracranial hypertension. Many of the MRI findings can be seen on ultrasound of the eye, including increased diameter of the optic nerve sheath, optic nerve sheath protrusion (length), retinal vein distention, and flattening of the posterior globe, which are correlated with intracranial pressure (ICP), intraocular pressure, and severity of papilledema (61–63). As MRI only shows the results of some cases of intracranial hypertension, the diagnosis can be missed (64). While obstruction of the various vascular fluid flows can be determined by MRI or CT angiogram, venogram, or CSF flow studies, as well as digital subtraction angiography, the main in-office methods we perform are transcranial and extracranial color duplex imaging. The net effect of CSF or venous flow obstruction can be intracranial hypertension (increased brain pressure), which can be found indirectly by various noninvasive testing methods, such as increased optic nerve sheath diameter. The neck vitals that pertain mainly to fluid flow analysis are discussed here.
Normal pressure within the lumbar and brain CSF is noted to be 6–15 mmHg in the supine and lateral decubitus position, and slightly lower while upright, because of gravity (65). Of interest is that in neurological patients, ICP positively correlates with intraocular pressure, remembering that the eye is within the central nervous system (66).
Intracranial pressure monitoring using invasive methods (lumbar, brain, or cervical spine puncture) has been the gold standard for the evaluation of IBP, defined as a cranial pressure of greater than 20 mmHg, but limitations include its invasiveness and its potential complications, such as hemorrhage and infection (67). The optic nerves, as well as the other cranial nerves and spinal nerve roots, are surrounded by CSF in the subarachnoid space. As ICP rises, especially from CSF stasis, the CSF looks for a path of least resistance, one of which surrounds the optic nerve and can be evaluated by optic nerve sheath diameter measurements. Pressure on the optic nerve from CSF within the sheath and increased intraocular pressure can explain many of the visual disturbances, including loss of visual field, diplopia, and blurry vision symptoms with LCI and LUCI (24, 68).
The optic nerve emerges from the posterior part of the globe and appears as a hypoechoic linear structure with a hyperechoic border (nerve sheath). The outer rim should be included in optic nerve sheath measurements and should be measured 3.0 mm behind the posterior rim of the globe (where standard measurements are made.) Measurements in adults and children without IBP are typically around 3.0 mm+/−1.0 mm (69). While some studies in adults use a cutoff as low as 4.5 mm and as high as 6.0 mm as evidence for increased ICP, emergency rooms and most others use an optic nerve sheath diameter measurement of greater than 5.5–6.0 mm to diagnose intracranial hypertension or IBP (70–74).
The optic nerve is well-suited for noninvasive evaluation of its diameter, as an ultrasound beam can travel unimpeded through the eyeball, which is mostly liquid. Historically, the optic nerve and its sheath diameter were noninvasively assessed by MRI and ophthalmoscopy, but transorbital ultrasound has recently emerged as a promising assessment tool (75). Ultrasound measurements of the optic nerve sheath diameter closely correlate with MRI measurements from in vivo and cadaver studies, and are reproducible (76, 77). Optic nerve sheath diameter has been shown to correlate with instantaneous assessments of ICP, including states of traumatic brain injury, and is even correlated with mortality (78–81). Typically, optic nerve sheath diameters over 6.0 mm are considered abnormal, and one of the evidences for IBP (see Figure 8).
Figure 8. Ultrasound of eye to measure optic nerve sheath diameter, which is used as a marker for intracranial hypertension (increased brain pressure).
Cervical ultrasound examination can reveal many findings that relate to cervical instability, including differences in size and flow velocities of carotid and vertebral arteries, and jugular veins, as well as evidence for dilated collateral vessels, lymphadenopathy, and measurements of pertinent nerve cross-sectional areas, including the vagus nerves (82–85). Vagus nerve degeneration (smaller cross-sectional areas) correlates with the amount of IJV compression. The differences between right and left sides are compared so the patient can be their own control and deviations from known normal values evaluated. Vascular obstructions are visualized in real time, including those in the carotid and vertebral arteries, and jugular veins, in various neck positions.
Transcranial Doppler (TCD) ultrasound provides real-time measurements of blood flow in the arteries that go to the brain, whereas extracranial Doppler (ECD) examines the veins and arteries in the neck as they go into and out of the brain. TCD has been called the “stethoscope for the brain” and ECD the “stethoscope for the neck.” These instruments can track moment-to-moment changes in blood flow to the brain from the vessels in the neck. They can assess the effect of interventions such as changes in neck position on brain blood flow (86–88) (see Figure 9). While the carotid and vertebral arteries can deform somewhat with neck motions such as rotation, there is not normally a significant change in carotid and/or vertebral artery blood flow with the upright position or neck rotation in patients with a cervical lordotic curve that is stable (89–92). TCD and ECD can be used to monitor the brain and neck blood flow for extended periods of time while holding a certain head/neck position. The blood flow in the brain, brainstem, neck, head, eye, and face can be compared in the supine, upright, and in various neck positions. A difference greater than 20% is considered significant to diagnose dynamic carotid artery stenosis or vertebrobasilar insufficiency, but changes in the waveform and pulsatility index (resistance to flow) can also be significant (93–96).
Figure 9. Motion transcranial Doppler monitoring. (A) Watching monitor while patient moves their neck. (B) Blood flow noted to be stopped in the middle cerebral artery. (C) Patient shown shortly after having a dystonic storm.
Blood flow constriction in the upper cervical spine is probable when LUCI is present due to the unique path the vertebral artery takes to go from the skull to the brain, where it is especially vulnerable to compression and stretch in the posterior neck at the level of the C1 and C2 vertebrae. The internal carotid artery is subject to compression and stretch, as it lies just anterior to the transverse processes of the C1, C2, and C3 vertebrae before entering the skull via the carotid canal.
While the real-time blood flow through the middle cerebral artery, the largest distal branch of the carotid artery, is measured by transcranial Doppler, so is pulsatility index, another noninvasive measurement for IBP (97, 98). Pulsatility index is a noninvasive method of assessing vascular resistance in the brain’s blood vessels, and it increases as intracranial pressure increases (99). Pulsatility index is defined as the difference between the peak systolic flow and minimum diastolic flow velocity, divided by the mean velocity recorded throughout the cardiac cycle, and a measurement greater than 1.20 typically means a brain pressure greater than 20 mmHg (100).
The cervical spine structural anatomy affects brain health in many ways. LUCI can affect the 3 major fluid flows through the neck on their way to and from the brain: venous, arterial, and CSF. The more acute and serious of the fluid flows to be altered is arterial, as obstruction of blood flow to the brain will cause immediate ischemia and can be life-threatening. Venolymphatic and cerebrospinal fluid flow obstructions are generally intermittent, lead to IBP, and are more chronic and subtle. Sometimes there are both obstructions, as the carotid artery pulsations can encroach on the IJVs (101).
Dynamic carotid artery and vertebral compression are identified by significant changes in arterial velocities, compared to baseline readings (102). They can be documented by transcranial and extracranial Doppler examinations, and the exact place of compression can be identified in the process. The brain receives 20% of the cardiac output, with the internal carotid artery supplying the anterior 75–80% of the brain, and the vertebral arteries supplying the posterior 20–25%. Just 5 s of cerebral ischemia causes a reactive hyperemia via cerebral autoregulation, and reperfusion after 30 s of ischemia causes an increase in cerebral circulation by 3- to 4-fold (103). Cervical dysstructure has been shown to cause a decline in cerebral blood flow, which improved as lordosis was restored (104). Standard static tests, including CT and MR angiogram, and even extracranial ultrasound, can miss dynamic carotid artery compression unless a person’s neck is flexed and/or rotated (105, 106). Carotid artery compression symptoms can range from intermittent dizziness, vertigo, and lightheadedness to transient ischemic episodes and full strokes (107). This condition is diagnosed via dynamic transcranial Doppler and extracranial ultrasound evaluations, showing significant changes in the middle and anterior cerebral artery (end-branches of the internal carotid artery), and internal carotid artery blood flows with neck posture and upright position (105).
Arterial flow obstruction can be analyzed in a similar manner as disruption of nerve signals. In LUCI cases, the most commonplace obstruction of vertebral arterial blood flow is at and around the atlas, and the most common area of instability is the atlantoaxial joint (108). The vertebral artery most often gets compressed near the lateral mass of C1 on its way to penetrate the atlanto-occipital ligament and enter the skull through the foramen magnum.
While there are many causes of cervicogenic dizziness, perhaps the most underdiagnosed is vertebrobasilar insufficiency (109). Bow hunter’s syndrome is quoted in the medical literature as being rare, but it may just be rare because physicians do not suspect it can occur in little children as well (110, 111). Again, arteries, veins, nerves, and other structures around bones move with the bones. If the atlas is malrotated or unstable, adverse mechanical tension can occur on the vertebral artery. As the vertebral arteries supply the posterior brain, brainstem, and upper spinal cord, symptoms can be acute from posterior circulation ischemia and include vertigo, dizziness, drop attacks, dystonic storms, loss of vision, double vision, numbness, tingling, confusion, cognitive deficits, trouble swallowing, or nausea/vomiting. If the brainstem or posterior brain blood flow only becomes ischemic with rotational (or other) head motions, then CT angiogram or Doppler ultrasound examinations must be done in the symptomatic position.
Loss of cervical lordosis is associated with decreased vertebral artery values in lumen diameter, flow volume, and peak systolic velocity (112). Another study evaluated 256 patients with radiologically detected cervical instability, looking at its effects on vertebrobasilar blood flow. In 80% of the patients with instability of the cervical spine of >3.0 mm, there was cerebral circulation dysfunction (113, 114). A decrease in vertebral artery blood flow has been significantly correlated with a loss of cervical lordosis, while improvements in cerebral blood flow with cervical curve adjustments have also been documented to restore lordosis (104, 112).
The IJVs, also within the carotid sheath, exit the jugular foramen and run adjacent to the anterior border of the cervical vertebral bodies, especially the atlas, as fluid drains from the brain. The IJV is the main drainage port for the brain, especially in the supine position. Normal IJV cross-sectional area is 100 mm2 in the supine position and collapses to about 25 mm2 in the upright position because of negative pressure in the thoracic cavity with respiration (115–117). This IJV collapse increases cerebral pressure, helping maintain ICP in the upright position (118). The IJV cross-sectional area is easily measured under ultrasound.
Internal jugular venous compression is quite common and is one of the causes of chronic cerebrospinal venous insufficiency (119). In a study of 108 consecutive patients undergoing CT angiography for presumed arterial obstruction, 50% of them had IJV compression and 93% of the compressions were at the level of C1 (120). The IJV can also become compressed because of cervical instability, mandibular malposition, weary muscles, dysstructure, styloid bones, and even cervical collars (121, 122). Jugular venous outflow compromise is associated with many symptoms, as it can cause IBP, whose classic symptoms include head pressure, lightheadedness, headache, nausea, vomiting, visual loss, and sixth cranial nerve palsy, as well as swallowing difficulties, brain fog, photophobia, pulsatile tinnitus, and vertigo (123, 124).
ICP is normally kept in a very narrow range of 5–15 mmHg, with intracranial hypertension being a pressure greater than 20 mmHg. Intracranial hypertension is simply hypertension of the brain, or IBP. It is important to understand that just as high blood pressure is the silent killer of the heart, high brain pressure is the silent killer of the brain. Left untreated, IBP can have serious repercussions, including brain neuronal cell death and brain tissue atrophy (125, 126). The most likely cause of so-called idiopathic intracranial hypertension is venous hypertension from LCI, LCUI, and cervical dysstructure (127). Compression of the IJV is typically seen at the level of the atlas. Sometimes it is a combination of the atlas being too far forward from cervical dysstructure or moving too much because of LUCI, or because there are styloids also compressing the IJVs from the front. Symptoms of IJV compression from a styloid bone are worse with flexion and rotation to the side of the styloid.
At any one time, most of the volume of blood in the brain is in the venous system, trying to get out (128). While 32 quarts of fluids flow through the brain each day, 70–80% of them are contained in the brain’s venous system (128). In 94% of healthy people, the IJV is the main venous system through which blood exits the brain, with 6% of people draining less than one-third of the brain through the IJV (129). When the IJV is blocked by LUCI, especially in the upright position (for example, if C1 is unstable and sliding too far forward, compressing the IJV), the brain drains via vertebral veins, external jugular veins, as well as the deep cervical veins and lymphatics (130, 131).
Internal jugular venous compression can occur from something as simple as turning one’s head and wearing a cervical collar. Several animal and human studies have documented that by turning one’s head, the ipsilateral jugular vein can be significantly compressed (132–134). Besides causing compression of the IJV, head/neck rotation has been shown to block CSF flow and raise ICP (135, 136). The increase in ICP with neck motion was maximal with flexion and rotation, with ICP in 26% of the patients going over 50 mmHg when that position was held for 1–2 min (137).
Extracranial IJV compression is increasingly recognized as a cause of IBP, and occurs in the J3 segment at the level of the lateral mass (transverse process) of C1 and styloid process in up to 96% of cases (129, 138, 139). Styloid elongation greater than 30 mm is a risk factor for compression (140). This latter condition is known as Eagle syndrome, whose symptoms relate to compression of the carotid sheath contents (141, 142). Eagle syndrome can occur when extra tension is put on the stylomandibular and stylohyoid ligaments from FH-FDL and calcifies by pressure traction (143). The calcified styloid then compresses the carotid sheath, especially if it angles medially between it and the lateral mass of C1. It is easily seen by CT venogram with 3-D reconstruction of the cervical spine. This explains why lying down, mastication, laughing, and head retraction—all of which change the position of the mandible and C1 or a styloid bone, thus putting tension (stretching) on the carotid sheath—can worsen symptoms due to carotid sheath compression (144).
Compression of the carotid sheath, which contains the IJV, can be caused by a narrowing of the space between the lateral mass of C1 and the styloid (3.7 mm of narrowing, in one study) known as the atlantostyloid interval (145). The diameter of the IJV correlating with reduced size of the atlantostyloid interval and the asymmetric larger area of the lateral mass of the atlas on the side of compression (by 40%) suggests LUCI as a reason for the bony size discrepancy (146). Since the styloid is anterior to the carotid sheath, retraction of the head should increase symptoms if an enlarged styloid is the cause of IJV compression; protraction of the head/jaw therefore relieves symptoms, as the atlantostyloid interval increases with that motion. While the gold standard to diagnose IJV compression has been CT venograms, in many cases it is possible for CT or MR venograms done in the supine neck neutral position to miss the IJV compression, as the atlantostyloid interval is narrowed maximally by contralateral head rotation (132). Static neutral scans do not consider compression of the carotid sheath with rotation, flexion, or extension of the neck. Ultrasound evaluation while moving the head in different positions can show the narrowing of the IJV at the site of compression (see Figure 10).
Figure 10. Degrees of internal jugular vein compression as seen on ultrasound examination of the neck. (A) Normal, “open” internal jugular vein. (B) Slightly compressed. (C) Moderately compressed. (D) Severely compressed. (E) Completely closed. It is internal jugular vein compression (arrows), especially with upright posture and neck motions, that leads to intracranial hypertension (increased brain pressure).
A proposed cervical ligament etiology of IBP is the 3:1 to 18:1 female preponderance, often relieved by lying down, and the fact that neck flexion and rotation can increase ICP by almost 9 mmHg (147–149). In fact, 90% of the patients are females of childbearing age (the most flexible adults), again possibly pointing to cervical instability as a cause (150).
Successful treatment is determined in large part by correctly identifying the underlying cause of symptoms and diseases. When the etiology is presumed to be structural in nature, treatment outcomes directed at the dysfunctional structure (dysstructure) will likely be more successful than chemical ones (medications). In regard to treatment of ICP, the main target of lowering the pressure in IBP is currently reached by medications such as acetazolamide, which acts as an inhibitor of the sulfonamide-sensitive carbonic anhydrases and reduces CSF secretion by approximately 50% (151). Weight loss combined with acetazolamide can be used, as well as ventricular shunting when ICP becomes severely elevated with significant symptoms (152, 153). The prognosis with these approaches is limited, as they do not resolve the condition (154).
As the most likely cause of ICP as presented here is structural, treatments should be directed at improving the structure. Brain fluid outflow is compromised by venous obstruction from both the immediate and long-term effects of LCI, which include vertebral malalignment, curve breakdown, and instability. A structural treatment program would therefore be necessary. Components of this program could include dynamic orthoneurological (chiropractic) adjustments for vertebral subluxations, curve correction by chest and head weights and therapeutic exercises, and Prolotherapy to tighten injured incompetent ligaments. It should be noted that while vertebral subluxations or misalignments—structural asymmetries in vertebral anatomy causing clinical symptoms—can be found objectively on radiographic or physical exam assessments, their clinical significance, though recognized by the World Health Organization, is not universally accepted (155, 156). When clinically significant, the correction is often performed by improving the biomechanics of the cervical curve and its structure, so the fluid flow and nerve signal transmission to and from the brain are improved, along with proprioception. This is confirmed by a lowering of the optic nerve sheath diameter and pulsatile indexes, documentation improvement of ICP, and thus brain health parameters.
The cervical structural, IJV, and optic nerve sheath diameter measurements for 227 consecutive patients aged 20–50 from January 1, 2022 to June 30, 2022, with no obvious cause for at least 1 of 8 specific brain or mental health symptoms—anxiety, brain fog, concentration difficulty, depression/hopelessness, headaches, obsessive thoughts, panic attacks, and rumination on traumatic events—were taken (see Figure 11). Some 96.2% of patients had an abnormal optic nerve sheath diameter (>6.1 mm). The cervical instability and dysstructure found are presumed to “simply” be from FH-FDL with computer and cell phone usage.
Figure 11. Summary of cervical structural analysis and ultrasound measurements of internal jugular veins (IJVs) and optic nerve sheath diameters (ONSDs) in 227 consecutive patients aged 20–50 who came to an outpatient neck center and had at least 1 of the 8 symptoms above, with no history of trauma or etiology of symptoms. * Mean bilateral total internal jugular vein cross-sectional areas (CSAs), ONSDs, and C1-C2 facet joint instability in patients with various brain/mental health symptoms. Normal for IV CSA >180 mm2, ONSD is <12.2 mm, and optimal C1 overhang on C2 in lateral flexion, open mouth is <2–4 mm. ** C6A1 = C6-atlas interval, horizontal distance in the sagittal plane of the posterior inferior C6 vertebra to anterior atlas (optimal is <10 mm). Measures how far forward the atlas (C1) is in relation to lower cervical vertebra (C6).
Body mechanics in health and disease are an integral part of medical specialties such as orthopedic surgery, physical medicine and rehabilitation, neurology, and osteopathic and chiropractic medicine. It involves how the human frame moves in 3-D space and its effect on joint and neurological structures, as well as organ systems and their functions. A more modern term to describe this field is “dynamic structural medicine,” defined as how human structure influences health and disease. “Dynamic” embodies the constant state of change that occurs in the human body to maintain homeostasis in various postures, positions, and motions. A coordinated and balanced effort between the musculoskeletal, neurologic, and vascular systems is required to maintain a healthy upright posture. It is underappreciated how subtle changes in spinal curvature, posture, and stability affect this delicate balance.
Without normal spinal alignment and movement, neurologic structures that traverse through the neck are at risk. Once alignment, curve, or stability are compromised, the body starts making compensatory changes down the spinal kinetic chain, with changes typically progressing from the neck down (157). Compared to people with normal lordotic curves, those with kyphosis note greater incidence of neck pain and scoliosis, disc herniations that are more severe, increased incidence of spinal stenosis with and without myelopathy, and spinal cord tensions associated with elevated intramedullary pressures (158–161). Missed dynamic cervical spine instability is associated with spinal cord compression, most commonly at the C5-C6 segment and cervical segments with greater disc bulge, more severe disc degeneration, greater angular motion, segmental kyphosis, and developmental stenosis (162).
Static and dynamic DMXs help determine which of the 3 variables (alignment, curve, and/or stability) are involved in a patient’s pathology, but typically one needs motion to show instability. By taking static and motion x-rays in the various neck planes, a picture of the 3-D cervical anatomy is revealed. The primary structural lesion is identified as causing the patient’s symptoms, and then the correct therapeutic remedy is applied. In cases of forward head posture or poor cervical curve in the absence of instability, restoration of the curve is crucial for overall health.
Ideal structural integrity of the cervical spine involves 3 primary parameters: alignment, curve, and stability. LCI can result in misalignment (subluxation), curve deformation, and ligamentous instability. In many ways, they are a continuum of the progressive nature of LCI, so treatment is directed at each part of the triad (see Figure 12).
Figure 12. Cervical treatment recommendations based on dynamic upright radiographic studies. Patients often have a combination of cervical instability, cervical dysstructure (breakdown of cervical curve), and misalignments, the 3 pillars of cervical structural health. * Some extreme cases of instability require surgery or other methods. ** Optimizing cervical curve is multifaceted and can include ergonomics, physical therapy and exercise, and many other physical medicine techniques.
Specific spinal manipulations (or adjustments) are the basis for much of the chiropractic profession (163). Traditional subluxations are diagnosed via static x-rays and physical examination, while dynamic orthoneurological corrections put heavy emphasis on dynamic 3-D anatomy, including spinal curves, utilizing motion x-rays, and taking into account the diagnostic neck vital tests to determine the primary lesion. Corrections are of little force, especially for the upper cervical region, with an activator or adjustment tool that an upper cervical chiropractic specialist would use, and not with high-velocity rotational forces.
Misalignments, especially of the atlas and axis, can have significant effects on compressing the carotid sheath. They are easily diagnosed on DMX and can compress the carotid sheath on the side of the rotation (with specific head positions and motions), causing characteristic pathophysiology and symptoms. The correction is often performed from a mostly anterior to posterior approach, as the cervical deformation with LCI is anterior.
While a study published in 1960 demonstrated that only 3 out of 180 patients (<1.7%) had cervical kyphosis, comparing it to recent studies shows that 30–40% of the general population has cervical kyphosis (21, 164). As those who have kyphosis are more prevalent in younger groups than those with straight or lordotic necks, and the fulcrum of the kyphosis is at C4-C5—the same fulcrum as neck flexion—clearly, the FH-FDL is involved (165, 166). One can then infer some connection between the alarmingly rising rates of cervical kyphosis and the increased use of cell phones and computers, especially in younger populations.
When cervical sagittal anatomy is optimized, symptoms including dizziness and neck pain resolve (167). Cervical curve correction may be initiated for many reasons, including structural, physiological, and symptomatologic (168). Indications that a patient may require cervical curve correction therapy are if observation and/or imaging show a significant forward head posture, or if the patient exhibits significant reversal of their cervical lordosis (169, 170). Other less obvious indicators that curve correction will be required for a successful treatment regimen are things like pooling of CSF at a certain level in the cervical spine with associated structural origin (as visualized on MRI) or flattening of the IJV in front of the upper cervical vertebrae.
Cervical curve correction can be as simple as changing one’s computer setup to be looking up, doing various postural exercises, or changing their sleeping position to one that opens up the jugular veins maximally (171). In our opinion, lying on a cervical orthotic such as the Denneroll® or using patient-specific weights in a standing or weight-bearing position can also help (see Figure 13). The patient can be x-rayed periodically to make sure the cervical curve is correcting, and the opening of the jugular veins can be verified with ultrasound.
Figure 13. Methods to improve one’s cervical curve. (A) Proper workstation. (B) Proper looking-down posture. (C) Postural exercises. * (D) Laying on Denneroll®. (E) Proper sleep position. (F) Pillow with cut-out. (G) Wearing neck weights. * Postural restoration institute.
Prolotherapy is often referred to as a regenerative injection technique that is used to stimulate repair of injured soft tissues, including ligaments. Our definition of “Prolotherapy” is: proliferative injections onto the bone at ligament attachments that strengthen and tighten ligaments to resolve joint instability. It is our opinion that the progress of Prolotherapy can be shown by a decrease or resolution of the instability on repeat DMXs; what can also be shown is an improvement in the neck vitals.2
Ligamentous tissue is often slow to heal on its own due to a lack of blood supply, which explains why people can develop chronic cervical instability that worsens over time. Prolotherapy to the cervical spine involves injecting an irritating solution (often dextrose) at ligament and tendon attachment sites that creates a mild inflammatory response, initiating the body’s natural healing cascade targeted at poorly vascularized tissue (172, 173). Load-bearing capacity, mass, tensile strength, and elasticity of collagenous connective tissues are increased. This initiates responses such as fibroblast and platelet activation, which repair and reinforce injured connective tissue.
Prolotherapy was developed by George Hackett, MD in the 1930s with the primary target to treat potential pain sources within connective tissue by either proliferation of new cells or the improvement in the health of existing cells (174). The most studied type of Prolotherapy utilizes dextrose, but other solutions may be used, such as platelet rich plasma, adipose tissue, bone marrow aspirate, or other minerals or fatty acids. Evidence that Prolotherapy induces the repair of ligaments and other soft tissue structures, including tendons, has been reported in both animal and human studies (175–177). Prolotherapy injections in the neck are directed at the posterior ligament complex, especially the capsular ligaments surrounding the facet joints (see Figure 14).
Figure 14. 3-D rendition showing upper cervical instability with motion and treatment by prolotherapy. (A) Upper cervical instability. (B) Prolotherapy to the C1-C2 facet joints.
In independent studies, Hackett and others reported significant successful outcomes in using Prolotherapy to treat ligament injuries, especially in those with cervical ligament injury-related symptoms, such as headache or whiplash-associated disorder (178–180). Additional clinicians documented significant symptom relief with cervical Prolotherapy directed at the posterior ligamentous complex, including the capsular ligaments (14, 181–183). Prolotherapy, in the absence of dynamic orthoneurological correction and cervical curve correction, is promising for those suffering from LCI. When coupled with loss of the lordotic curve and cervical malrotations, LCI could be reversed using proper structural treatment aimed at resolving targeted structural defects.
A 51-year-old man with a long history of anxiety, depression, dissociative disorder (depersonalization/derealization), panic attacks, brain fog, memory decline, concentration difficulty, chronic fatigue, low stress tolerance, chronic neck pain, and head pressure has worked as an engineer for 30 years, putting in long hours hunched in front of a computer screen. He has seen many healthcare providers, including psychiatrists, psychologists, and chiropractors, but his symptoms continue to progress. He admits to self-manipulating his neck many times a day. Initial cervical structural testing revealed severe cervical dysstructure and upper cervical instability, causing compression of the carotid sheath. He was told to stop self-manipulating his neck and was educated on improved ergonomics for his workstation, so he bought a rocker-bottom chair and standing desk and raised his computer height significantly. His best positions to sleep in were on his left side with his head extended or with a cut-out (Denneroll®) pillow, as they opened his IJVs compared to his normal chin-down sleeping position. He was initially told to lay on a Denneroll® for 5 min every hour (with improvements in curve, this time went down to 20 min per day), started exercises to strengthen his neck musculature, and was given Prolotherapy for his LCI. Over the course of 1 year, during which he received 6 Prolotherapy sessions, we noted a significant increase in his IJV cross-sectional areas and improvement in his neck curve (see Figure 15). Upon his last visit to the clinic in June 2024, he noted no more dissociative episodes nor panic attacks, and approximately 80–90% subjective improvement in his brain and mental health symptoms. He continues his cervical curve correction program.
Figure 15. Improvement in patient’s cervical curve structure correlates with opening of his internal jugular veins and reduction in optic verve sheath diameter. Over the course of a year, his brain and mental health symptoms improved significantly. * Mean bilateral total internal jugular vein (IJV) cross-sectional areas (CSAs), optic nerve sheath diameters (ONSDs), and C1-C2 facet joint instability in patients with various brain/mental health symptoms. Normal for IJV CSA is 180 mm2, ONSD is <12.2 mm, and optimal C1 overhang on C2 in lateral flexion, open mouth is <2–4 mm. ** C6A1 = C6-atlas interval, horizontal distance in the sagittal plane of the posterior inferior C6 vertebra to anterior atlas (optimal is <10 mm). This measurement shows how far forward the atlas (C1) is in relation to the lower cervical vertebra (C6).
The FH-FDL from excessive time spent looking at computers, laptops, and cell phones causes the slow stretching of posterior ligaments in the cervical spine, especially the capsular ligaments. As cervical ligaments stretch out, they can no longer handle the forces of the head on the neck and the normal cervical curve starts to break down, a process called cervical dysstructure. Clinical ligamentous cervical instability results, which is an inability of the cervical ligaments to maintain individual, adjacent, or global vertebral alignment when subjected to increased forces by various postures, positions, and/or motions that alter bony, soft tissue, and/or neurovascular alignment and function such that symptoms result. The cervical lordotic curve becomes straight and then kyphotic as head forces go in front of the cervical vertebrae, causing the condition to be progressive. This change in cervical curve can affect everything that traverses the neck, including vital neurovascular structures. Cervical muscle spasms, facet joint swelling, and degeneration occur to try and limit destructive neck motions. When the body runs out of compensatory mechanisms to limit forces and destruction, neurologic and vascular compromise and injury occur, causing symptoms.
Dynamic structural medicine explains how human posture and motion affect body and brain health and disease. Detrimental changes in the neck curve—cervical dysstructure from ligamentous cervical instability—cause compression of the carotid sheath, narrowing the jugular vein and stretching and putting pressure on the vagus nerve, potentially causing brain and body disease, respectively. The most serious symptoms occur when brain health is affected by the IJVs and the carotid and vertebral arteries becoming compressed, causing intracranial hypertension and arterial insufficiency or ischemia, respectively. These conditions can cause myriad symptoms from neck tension, headache, blurry vision, dizziness, tinnitus, and ringing in the ear, to personality changes and many others. Because the neurovascular compression often occurs in the upright position or with specific neck motions, traditional MRI or CT scans in the supine position can miss it. Cervical structural analysis is performed in the upright position in different head and neck positions with DMX and cone beam CT. A neck vitals analysis is done to document the pathophysiology caused by the LCI.
As presented here, the most likely cause of IBP is structural. This reaffirms that a structural treatment program should be prescribed that is aimed at correcting incompetent structures of the cervical spine. Ligamentous cervical instability is often the missing structural cause for many chronic conditions associated with the cervical spine and neck’s relevant anatomy. Instability, along with loss of the cervical lordotic curve, can be the cause of increased brain pressure and many associated chronic conditions. Treatments directed at cervical vertebral malalignments (subluxations), curve dysstructure (breakdown), and instability include dynamic orthoneurological (chiropractic) low-force adjustments, curve correction and therapeutic exercises, and Prolotherapy, respectively.
The original contributions presented in the study are included in the article/supplementary material, further inquiries can be directed to the corresponding author.
Written informed consent was obtained from the individuals for the publication of any identifiable images or data included in this article.
RH: Conceptualization, Investigation, Writing – original draft. DM: Writing – original draft. BR: Conceptualization, Project administration, Resources, Supervision, Visualization, Writing – review & editing.
The author(s) declare that no financial support was received for the research, authorship, and/or publication of this article.
The authors declare that the research was conducted in the absence of any commercial or financial relationships that could be construed as a potential conflict of interest.
All claims expressed in this article are solely those of the authors and do not necessarily represent those of their affiliated organizations, or those of the publisher, the editors and the reviewers. Any product that may be evaluated in this article, or claim that may be made by its manufacturer, is not guaranteed or endorsed by the publisher.
1. ^Analysis of patients coming to our outpatient Hauser Neck Center. As of November 1, 2024, final statistical analysis is being completed for scientific publication.
2. ^Opinion based on 8,000 DMXs and data that are forthcoming in subsequent articles after this overview article.
1. US Department of Health and Human Services. (2024) Results from the 2012 National Survey on drug use and health. Mental Health Findings. Available at: https://www.samhsa.gov/data/sites/default/files/2k12MH_Findings/2k12MH_Findings/NSDUHmhfr2012.htm (Accessed August 27, 2024).
2. National Institute of Mental Health. (2024). Available at: https://www.nimh.nih.gov/health/statistics/mental-illness (Accessed August 27, 2024).
3. World Health Organization. WHO global burden of disease: 2004 update. Geneva: World Health Organization (2008) Available at: http://www.who.int/healthinfo/global_burden_disease/GBD_report_2004update_full.pdf (Accessed April 22, 2024).
4. Panjabi, MM. The stabilizing system of the spine. Part I. Function, dysfunction, adaptation, and enhancement. J Spinal Disord. (1992) 5:383–9. doi: 10.1097/00002517-199212000-00001
5. Kim, CW, Perry, A, and Garfin, SR. Spinal instability: the orthopedic approach. Semin Musculoskelet Radiol. (2005) 9:77–87. doi: 10.1055/s-2005-867098
6. Kwee, RM, Hafezi-Nejad, N, Roemer, FW, Zikria, BA, Hunter, DJ, Guermazi, A, et al. Association of Mucoid Degeneration of the anterior cruciate ligament at MR imaging with medial tibiofemoral osteoarthritis progression at radiography: data from the osteoarthritis initiative. Radiology. (2018) 287:912–21. doi: 10.1148/radiol.2018171565
7. Hasegawa, A, Otsuki, S, Pauli, C, Miyaki, S, Patil, S, Steklov, N, et al. Anterior cruciate ligament changes in the human knee joint in aging and osteoarthritis. Arthritis Rheumatol. (2012) 64:696–704. doi: 10.1002/art.33417
8. Bogduk, N. Functional anatomy of the spine. Handb Clin Neurol. (2016) 136:675–88. doi: 10.1016/B978-0-444-53486-6.00032-6
9. Steilen, D, Hauser, R, Woldin, B, and Sawyer, S. Chronic neck pain: making the connection between capsular ligament laxity and cervical instability. Open Orthop J. (2014) 8:326–45. doi: 10.2174/1874325001408010326
10. Myers, BS, Ejima, S, Ono, K, Kamiji, K, Yasuki, T, and Winkelstein, BA. Head-turned postures increase the risk of cervical facet capsule injury during whiplash. Spine (Phila Pa 1976). (2008) 33:1643–9. doi: 10.1097/BRS.0b013e31817b5bcf
11. Visocchi, M. Why the Craniovertebral junction? Acta Neurochir Suppl. (2019) 125:3–8. doi: 10.1007/978-3-319-62515-7_1
12. Klimo, P Jr, Rao, G, and Brockmeyer, D. Congenital anomalies of the cervical spine. Neurosurg Clin N Am. (2007) 18:463–78. doi: 10.1016/j.nec.2007.04.005
13. Katz, EA, Katz, SB, and Freeman, MD. Non-surgical Management of Upper Cervical Instability via improved cervical lordosis: a case series of adult patients. J Clin Med. (2023) 12:1797. doi: 10.3390/jcm12051797
14. Hauser, RA, Steilen, D, and Gordin, K. The biology of Prolotherapy and its application in clinical cervical spine instability and chronic neck pain: a retrospective study. Eur J Prev Med. (2015) 3:85–102. doi: 10.11648/j.ejpm.20150304.11
15. Phuntsok, R, Ellis, BJ, Herron, MR, Provost, CW, Dailey, AT, and Brockmeyer, DL. The occipitoatlantal capsular ligaments are the primary stabilizers of the occipitoatlantal joint in the craniocervical junction: a finite element analysis. J Neurosurg Spine. (2019) 30:593–601. doi: 10.3171/2018.10.SPINE181102
16. Swartz, EE, Floyd, RT, and Cendoma, M. Cervical spine functional anatomy and the biomechanics of injury due to compressive loading. J Athl Train. (2005) 40:155–61.
17. Yoon, SY, Moon, HI, Lee, SC, Eun, NL, and Kim, YW. Association between cervical lordotic curvature and cervical muscle cross-sectional area in patients with loss of cervical lordosis. Clin Anat. (2018) 31:710–5. doi: 10.1002/ca.23074
18. Been, E, Shefi, S, and Soudack, M. Cervical lordosis: the effect of age and gender. Spine J. (2017) 17:880–8. doi: 10.1016/j.spinee.2017.02.007
19. Radcliff, K, Rubin, T, and Reitman, CA. Normal cervical alignment. Semin Spine Surg. (2011) 23:159–64. doi: 10.1053/j.semss.2011.04.006
20. Öğrenci, A, Koban, O, Yaman, O, Dalbayrak, S, and Yılmaz, M. The effect of technological devices on cervical lordosis. Open Access Maced J Med Sci. (2018) 6:467–71. doi: 10.3889/oamjms.2018.107
21. Borden, AGB, Rechtman, AM, and Gershon-Cohen, J. The normal cervical lordosis. Radiology. (1960) 74:806–9. doi: 10.1148/74.5.806
22. Hou, SB, Sun, XZ, Liu, FY, Gong, R, Zhao, ZQ, Lu, K, et al. Relationship of change in cervical curvature after laminectomy with lateral mass screw fixation to spinal cord shift and clinical efficacy. J Neurol Surg A Cent Eur Neurosurg. (2022) 83:129–34. doi: 10.1055/s-0041-1723807
23. Tan, LA, Riew, DK, and Traynelis, VC. Cervical spine deformity-part 1: biomechanics, radiographic parameters, and classification. Neurosurgery. (2017) 81:197–203. doi: 10.1093/neuros/nyx249
24. Kamel, I, and Barnette, R. Positioning patients for spine surgery: avoiding uncommon position-related complications. World J Orthop. (2014) 5:425–43. doi: 10.5312/wjo.v5.i4.425
25. Norasi, H, Tetteh, E, Sarker, P, Mirka, GA, and Hallbeck, MS. Exploring the relationship between neck flexion and neck problems in occupational populations: a systematic review of the literature. Ergonomics. (2022) 65:587–603. doi: 10.1080/00140139.2021.1976847
26. Barrett, JM, McKinnon, C, and Callaghan, JP. Cervical spine joint loading with neck flexion. Ergonomics. (2020) 63:101–8. doi: 10.1080/00140139.2019.1677944
27. Anderst, W. (2014). In vivo cervical spine kinematics arthrokinematics and disc loading in asymptomatic control subjects and anterior fusion patients. Pittsburgh (PA): University of Pittsburgh. Available at: http://d-scholarship.pitt.edu/22226/1/anderst_edt2014.pdf (Accessed July 6, 2023).
28. Reid, JD. Effects of flexion-extension movements of the head and spine upon the spinal cord and nerve roots. J Neurol Neurosurg Psychiatry. (1960) 23:214–21. doi: 10.1136/jnnp.23.3.214
29. Hansraj, KK. Assessment of stresses in the cervical spine caused by posture and position of the head. Surg Technol Int. (2014) 25:277–9.
30. Coakwell, MR, Bloswick, DS, and Moser, R Jr. High-risk head and neck movements at high G and interventions to reduce associated neck injury. Aviat Space Environ Med. (2004) 75:68–80.
31. Maiman, DJ, Sances, A Jr, Myklebust, JB, Larson, SJ, Houterman, C, Chilbert, M, et al. Compression injuries of the cervical spine: a biomechanical analysis. Neurosurgery. (1983) 13:254–60. doi: 10.1227/00006123-198309000-00007
32. Russek, LN, Block, NP, Byrne, E, Chalela, S, Chan, C, Comerford, M, et al. Presentation and physical therapy management of upper cervical instability in patients with symptomatic generalized joint hypermobility: international expert consensus recommendations. Front Med (Lausanne). (2023) 9:1072764. doi: 10.3389/fmed.2022.1072764
34. Solomonow, M. Ligaments: a source of musculoskeletal disorders. J Bodyw Mov Ther. (2009) 13:136–54. doi: 10.1016/j.jbmt.2008.02.001
35. Lee, KE, Davis, MB, Mejilla, RM, and Winkelstein, BA. In vivo cervical facet capsule distraction: mechanical implications for whiplash and neck pain. Stapp Car Crash J. (2004) 48:373–95. doi: 10.4271/2004-22-0016
36. Lu, Y, Chen, C, Kallakuri, S, Patwardhan, A, and Cavanaugh, JM. Neural response of cervical facet joint capsule to stretch: a study of whiplash pain mechanism. Stapp Car Crash J. (2005) 49:49–65. doi: 10.4271/2005-22-0003
37. Winkelstein, BA, McLendon, RE, Barbir, A, and Myers, BS. An anatomical investigation of the human cervical facet capsule, quantifying muscle insertion area. J Anat. (2001) 198:455–61. doi: 10.1046/j.1469-7580.2001.19840455.x
38. Cook, C, Brismée, JM, Fleming, R, and Sizer, PS Jr. Identifiers suggestive of clinical cervical spine instability: a Delphi study of physical therapists. Phys Ther. (2005) 85:895–906. doi: 10.1093/ptj/85.9.895
39. Olson, KA, and Joder, D. Diagnosis and treatment of cervical spine clinical instability. J Orthop Sports Phys Ther. (2001) 31:194–206. doi: 10.2519/jospt.2001.31.4.194
40. Patwardhan, AG, Havey, RM, Khayatzadeh, S, Muriuki, MG, Voronov, LI, Carandang, G, et al. Postural consequences of cervical sagittal imbalance: a novel laboratory model. Spine (Phila Pa 1976). (2015) 40:783–92. doi: 10.1097/BRS.0000000000000877
41. Wang, K, Deng, Z, Li, Z, Wang, H, and Zhan, H. The influence of natural head position on the cervical sagittal alignment. J Healthc Eng. (2017) 2017:1–7. doi: 10.1155/2017/2941048
42. Stemper, BD, Yoganandan, N, and Pintar, FA. Effects of abnormal posture on capsular ligament elongations in a computational model subjected to whiplash loading. J Biomech. (2005) 38:1313–23. doi: 10.1016/j.jbiomech.2004.06.013
43. Miyazaki, M, Hong, SW, Yoon, SH, Zou, J, Tow, B, Alanay, A, et al. Kinematic analysis of the relationship between the grade of disc degeneration and motion unit of the cervical spine. Spine (Phila Pa 1976). (2008) 33:187–93. doi: 10.1097/BRS.0b013e3181604501
44. Dai, L. Disc degeneration and cervical instability. Correlation of magnetic resonance imaging with radiography. Spine (Phila Pa 1976). (1998) 23:1734–8. doi: 10.1097/00007632-199808150-00005
45. Mayer, M, Zenner, J, Auffarth, A, Blocher, M, Figl, M, Resch, H, et al. Hidden discoligamentous instability in cervical spine injuries: can quantitative motion analysis improve detection? Eur Spine J. (2013) 22:2219–27. doi: 10.1007/s00586-013-2854-x
46. Kim, JH, Sharan, A, Cho, W, Emam, M, Hagen, M, and Kim, SY. The prevalence of asymptomatic cervical and lumbar facet Arthropathy: a computed tomography study. Asian Spine J. (2019) 13:417–22. doi: 10.31616/asj.2018.0235
47. Kato, F, Yukawa, Y, Suda, K, Yamagata, M, and Ueta, T. Normal morphology, age-related changes and abnormal findings of the cervical spine. Part II: magnetic resonance imaging of over 1,200 asymptomatic subjects. Eur Spine J. (2012) 21:1499–507. doi: 10.1007/s00586-012-2176-4
48. Gupta, V, Khandelwal, N, Mathuria, SN, Singh, P, Pathak, A, and Suri, S. Dynamic magnetic resonance imaging evaluation of craniovertebral junction abnormalities. J Comput Assist Tomogr. (2007) 31:354–9. doi: 10.1097/01.rct.0000238009.57307.26
49. Freeman, MD, Rosa, S, Harshfield, D, Smith, F, Bennett, R, Centeno, CJ, et al. A case-control study of cerebellar tonsillar ectopia (Chiari) and head/neck trauma (whiplash). Brain Inj. (2010) 24:988–94. doi: 10.3109/02699052.2010.490512
50. Suzuki, F, Fukami, T, Tsuji, A, Takagi, K, and Matsuda, M. Discrepancies of MRI findings between recumbent and upright positions in atlantoaxial lesion. Report of two cases. Eur Spine J. (2008) 17:304–S307. doi: 10.1007/s00586-008-0595-z
51. Michelini, G, Corridore, A, Torlone, S, Bruno, F, Marsecano, C, Capasso, R, et al. Dynamic MRI in the evaluation of the spine: state of the art. Acta Biomed. (2018) 89:89–101. doi: 10.23750/abm.v89i1-S.7012
52. Gilbert, JW, Wheeler, GR, Lingreen, RA, and Johnson, RK. Upright weight-bearing cervical flexion/extension dynamic magnetic resonance imaging: case report and review of the literature. Eur J Radiol Extra. (2006) 60:121–4. doi: 10.1016/j.ejrex.2006.09.007
53. Smith, FW, and Dworkin, JS eds. The Craniocervical syndrome and MRI. Basel, Switzerland: Karger (2015).
54. Freeman, MD, Katz, EA, Rosa, SL, Gatterman, BG, Strömmer, EMF, and Leith, WM. Diagnostic accuracy of Videofluoroscopy for symptomatic cervical spine injury following whiplash trauma. Int J Environ Res Public Health. (2020) 17:1693. doi: 10.3390/ijerph17051693
55. Dvorak, J, Hayek, J, and Zehnder, R. CT-functional diagnostics of the rotatory instability of the upper cervical spine. Part 2. An evaluation on healthy adults and patients with suspected instability. Spine (Phila Pa 1976). (1987) 12:726–31. doi: 10.1097/00007632-198710000-00002
56. Kawasaki, M, Tani, T, Ushida, T, and Ishida, K. Anterolisthesis and retrolisthesis of the cervical spine in cervical spondylotic myelopathy in the elderly. J Orthop Sci. (2007) 12:207–13. doi: 10.1007/s00776-007-1122-5
57. Daffner, RH, Brown, RR, and Goldberg, AL. A new classification for cervical vertebral injuries: influence of CT. Skeletal Radiol. (2000) 29:125–32. doi: 10.1007/s002560050582
58. Liao, S, Jung, MK, Hörnig, L, Grützner, PA, and Kreinest, M. Injuries of the upper cervical spine-how can instability be identified? Int Orthop. (2020) 44:1239–53. doi: 10.1007/s00264-020-04593-y
59. van Middendorp, JJ, Audigé, L, Hanson, B, Chapman, JR, and Hosman, AJ. What should an ideal spinal injury classification system consist of? A methodological review and conceptual proposal for future classifications. Eur Spine J. (2010) 19:1238–49. doi: 10.1007/s00586-010-1415-9
60. Maeda, FL, Formentin, C, de Andrade, EJ, Rodrigues, PAS, Goyal, DKC, Shroeder, GD, et al. Reliability of the new AOSpine classification system for upper cervical traumatic injuries. Neurosurgery. (2020) 86:E263–70. doi: 10.1093/neuros/nyz464
61. Chang, YC, Alperin, N, Bagci, AM, Lee, SH, Rosa, PR, Giovanni, G, et al. Relationship between optic nerve protrusion measured by OCT and MRI and papilledema severity. Invest Ophthalmol Vis Sci. (2015) 56:2297–302. doi: 10.1167/iovs.15-16602
62. Degnan, AJ, and Levy, LM. Pseudotumor cerebri: brief review of clinical syndrome and imaging findings. AJNR Am J Neuroradiol. (2011) 32:1986–93. doi: 10.3174/ajnr.A2404
63. Wall, M. The morphology of visual field damage in idiopathic intracranial hypertension: an anatomic region analysis. In: RP Mills and A Heijl, editors. Perimetry update 1990/1991. Amsterdam, Netherlands: Kugler Publications (1991). 20–7.
64. Barkatullah, AF, Leishangthem, L, and Moss, HE. MRI findings as markers of idiopathic intracranial hypertension. Curr Opin Neurol. (2021) 34:75–83. doi: 10.1097/WCO.0000000000000885
65. Norager, NH, Olsen, MH, Pedersen, SH, Riedel, CS, Czosnyka, M, and Juhler, M. Reference values for intracranial pressure and lumbar cerebrospinal fluid pressure: a systematic review. Fluids Barriers CNS. (2021) 18:19. doi: 10.1186/s12987-021-00253-4
66. Garg, P, Diwedi, S, and Mullick, R. To evaluate the correlation between intracranial pressure and intraocular pressure in neurological patients: a prospective cohort study. Int J Ophthalmol Clin Res. (2019) 6:104. doi: 10.23937/2378-346X/1410104
67. Geeraerts, T, Launey, Y, Martin, L, Pottecher, J, Vigué, B, Duranteau, J, et al. Ultrasonography of the optic nerve sheath may be useful for detecting raised intracranial pressure after severe brain injury. Intensive Care Med. (2007) 33:1704–11. doi: 10.1007/s00134-007-0797-6
68. Manfré, L, Lagalla, R, Mangiameli, A, Lupo, F, Giuffré, G, Ponte, F, et al. Idiopathic intracranial hypertension: orbital MRI. Neuroradiology. (1995) 37:459–61. doi: 10.1007/BF00600093
69. Naldi, A, Provero, P, Vercelli, A, Bergui, M, Mazzeo, AT, Cantello, R, et al. Optic nerve sheath diameter asymmetry in healthy subjects and patients with intracranial hypertension. Neurol Sci. (2020) 41:329–33. doi: 10.1007/s10072-019-04076-y
70. Lochner, P, Czosnyka, M, Naldi, A, Lyros, E, Pelosi, P, Mathur, S, et al. Optic nerve sheath diameter: present and future perspectives for neurologists and critical care physicians. Neurol Sci. (2019) 40:2447–57. doi: 10.1007/s10072-019-04015-x
71. Ussahgij, W, Toonpirom, W, Munkong, W, and Ienghong, K. Optic nerve sheath diameter cutoff point for detection of increased intracranial pressure in the emergency room. Maced. J Med Sci. (2020) 8:62–5. doi: 10.3889/oamjms.2020.3272
72. Kishk, NA, Ebraheim, AM, Ashour, AS, Badr, NM, and Eshra, MA. Optic nerve sonographic examination to predict raised intracranial pressure in idiopathic intracranial hypertension: the cut-off points. Neuroradiol J. (2018) 31:490–5. doi: 10.1177/1971400918789385
73. Lee, SJ, Choi, MH, Lee, SE, Park, JH, Park, B, Lee, JS, et al. Optic nerve sheath diameter change in prediction of malignant cerebral edema in ischemic stroke: an observational study. BMC Neurol. (2020) 20:354. doi: 10.1186/s12883-020-01931-w
74. Dubourg, J, Javouhey, E, Geeraerts, T, Messerer, M, and Kassai, B. Ultrasonography of optic nerve sheath diameter for detection of raised intracranial pressure: a systematic review and meta-analysis. Intensive Care Med. (2011) 37:1059–68. doi: 10.1007/s00134-011-2224-2
75. Tawfik, EA, Walker, FO, and Cartwright, MS. Neuromuscular ultrasound of cranial nerves. J Clin Neurol. (2015) 11:109–21. doi: 10.3988/jcn.2015.11.2.109
76. Steinborn, M, Fiegler, J, Kraus, V, Denne, C, Hapfelmeier, A, Wurzinger, L, et al. High resolution ultrasound and magnetic resonance imaging of the optic nerve and the optic nerve sheath: anatomic correlation and clinical importance. Ultraschall Med. (2011) 32:608–13. doi: 10.1055/s-0029-1245822
77. Bäuerle, J, Schuchardt, F, Schroeder, L, Egger, K, Weigel, M, and Harloff, A. Reproducibility and accuracy of optic nerve sheath diameter assessment using ultrasound compared to magnetic resonance imaging. BMC Neurol. (2013) 13:187. doi: 10.1186/1471-2377-13-187
78. Robba, C, Donnelly, J, Cardim, D, Tajsic, T, Cabeleira, M, Citerio, G, et al. Optic nerve sheath diameter ultrasonography at admission as a predictor of intracranial hypertension in traumatic brain injured patients: a prospective observational study. J Neurosurg. (2019) 132:1279–85. doi: 10.3171/2018.11.JNS182077
79. Shrestha, B, Shrestha, P, Ghale, P, and Lakshmipathy, G. Correlation between invasive intracranial pressure monitoring and optic nerve sheath diameter in patients with traumatic brain injury. Kathmandu Univ Med J (KUMJ). (2021) 19:221–4. doi: 10.3126/kumj.v19i2.49650
80. Robba, C, Santori, G, Czosnyka, M, Corradi, F, Bragazzi, N, Padayachy, L, et al. Optic nerve sheath diameter measured sonographically as non-invasive estimator of intracranial pressure: a systematic review and meta-analysis. Intensive Care Med. (2018) 44:1284–94. doi: 10.1007/s00134-018-5305-7
81. Wang, LJ, Chen, HX, Chen, Y, Yu, ZY, and Xing, YQ. Optic nerve sheath diameter ultrasonography for elevated intracranial pressure detection. Ann Clin Transl Neurol. (2020) 7:865–8. doi: 10.1002/acn3.51054
82. Giovagnorio, F, and Martinoli, C. Sonography of the cervical vagus nerve: normal appearance and abnormal findings. AJR Am J Roentgenol. (2001) 176:745–9. doi: 10.2214/ajr.176.3.1760745
83. Chen, HH, Chen, TC, Yang, TL, and Wang, CP. Transcutaneous sonography for detection of the cervical Vagus nerve. Ear Nose Throat J. (2021) 100:155–9. doi: 10.1177/0145561319875432
84. Norin, H, Pikwer, A, Fellert, F, and Åkeson, J. Internal jugular dimensions and common carotid overlapping assessed in a cross-sectional study by ultrasonography at three neck levels in healthy volunteers. J Vasc Access. (2017) 18:69–72. doi: 10.5301/jva.5000619
85. Ahuja, AT, and Ying, M. Sonographic evaluation of cervical lymph nodes. AJR Am J Roentgenol. (2005) 184:1691–9. doi: 10.2214/ajr.184.5.01841691
86. White, RP, and Markus, HS. Impaired dynamic cerebral autoregulation in carotid artery stenosis. Stroke. (1997) 28:1340–4. doi: 10.1161/01.str.28.7.1340
87. Mitchell, J. Vertebral artery blood flow velocity changes associated with cervical spine rotation: a Meta-analysis of the evidence with implications for professional practice. J Man Manip Ther. (2009) 17:46–57. doi: 10.1179/106698109790818160
88. D'Andrea, A, Conte, M, Cavallaro, M, Scarafile, R, Riegler, L, Cocchia, R, et al. Transcranial Doppler ultrasonography: from methodology to major clinical applications. World J Cardiol. (2016) 8:383–400. doi: 10.4330/wjc.v8.i7.383
89. Mitchell, J, and Kramschuster, K. Real-time ultrasound measurements of changes in suboccipital vertebral artery diameter and blood flow velocity associated with cervical spine rotation. Physiother Res Int. (2008) 13:241–54. doi: 10.1002/pri.400
90. Beigelman, R, Izaguirre, A, Robles, M, Grana, D, Ambrosio, G, and Milei, J. Kinking of carotid arteries is not a mechanism of cerebral ischemia: a functional evaluation by Doppler echography. Int Angiol. (2011) 30:342–8.
91. Zaina, C, Grant, R, Johnson, C, Dansie, B, Taylor, J, and Spyropolous, P. The effect of cervical rotation on blood flow in the contralateral vertebral artery. Man Ther. (2003) 8:103–9. doi: 10.1016/s1356-689x(02)00155-8
92. Ohya, T, Iwai, T, Luan, K, Kato, T, Liao, H, Kobayashi, E, et al. Analysis of carotid artery deformation in different head and neck positions for maxillofacial catheter navigation in advanced oral cancer treatment. Biomed Eng Online. (2012) 11:65. doi: 10.1186/1475-925X-11-65
93. Gunabushanam, G, Kummant, L, and Scoutt, LM. Vertebral artery ultrasound. Radiol Clin North Am. (2019) 57:519–33. doi: 10.1016/j.rcl.2019.01.011
94. Ginat, DT, Bhatt, S, Sidhu, R, and Dogra, V. Carotid and vertebral artery Doppler ultrasound waveforms: a pictorial review. Ultrasound Q. (2011) 27:81–5. doi: 10.1097/RUQ.0b013e31821c7f6a
95. Neto, ACL, Bor-Seng-Shu, E, Oliveira, ML, Macedo-Soares, A, Topciu, FR, and Bittar, RSM. Magnetic resonance angiography and transcranial Doppler ultrasound findings in patients with a clinical diagnosis of vertebrobasilar insufficiency. Clinics (Sao Paulo). (2020) 75:e1212. doi: 10.6061/clinics/2020/e1212
96. Sultan, MJ, Hartshorne, T, and Naylor, AR. Extracranial and transcranial ultrasound assessment in patients with suspected positional “vertebrobasilar ischaemia”. Eur J Vasc Endovasc Surg. (2009) 38:10–3. doi: 10.1016/j.ejvs.2008.12.006
97. Kaloria, N, Panda, NB, Bhagat, H, Kaloria, N, Soni, SL, Chauhan, R, et al. Pulsatility index reflects intracranial pressure better than resistive index in patients with clinical features of intracranial hypertension. J Neurosci Rural Pract. (2020) 11:144–50. doi: 10.1055/s-0039-3399477
98. Bellner, J, Romner, B, Reinstrup, P, Kristiansson, KA, Ryding, E, and Brandt, L. Transcranial Doppler sonography pulsatility index (PI) reflects intracranial pressure (ICP). Surg Neurol. (2004) 62:45–51. doi: 10.1016/j.surneu.2003.12.007
99. Bouguetof, H, Negadi, M, Halimi, KE, and Boumendil, D. Correlation between intracranial pressure and pulsatility index measured by transcranial Doppler in children with severe trauma brain injury. Crit Care. (2015) 19:449. doi: 10.1186/cc14529
100. Pinillos, OM, Rodríguez, CN, and Hakimi, R. Transcranial Doppler ultrasound Pulsatility index: utility and clinical interpretation. In: CN Rodríguez, C Baracchini, JH Mejia-Mantilla, M Czosnyka, JI Suarez, and L Csiba, et al., editors. Neurosonology in critical care. Cham: Springer (2022). 357–76.
101. Li, M, Su, C, Fan, C, Chan, CC, Bai, C, and Meng, R. Internal jugular vein stenosis induced by tortuous internal carotid artery compression: two case reports and literature review. J Int Med Res. (2019) 47:3926–33. doi: 10.1177/0300060519860678
102. Sturzenegger, M, Newell, DW, Douville, C, Byrd, S, and Schoonover, K. Dynamic transcranial Doppler assessment of positional vertebrobasilar ischemia. Stroke. (1994) 25:1776–83. doi: 10.1161/01.str.25.9.1776
103. Gourley, JK, and Heistad, DD. Characteristics of reactive hyperemia in the cerebral circulation. Am J Phys. (1984) 246:H52–8. doi: 10.1152/ajpheart.1984.246.1.H52
104. Katz, EA, Katz, SB, Fedorchuk, CA, Lightstone, DF, Banach, CJ, and Podoll, JD. Increase in cerebral blood flow indicated by increased cerebral arterial area and pixel intensity on brain magnetic resonance angiogram following correction of cervical lordosis. Brain Circ. (2019) 5:19–26. doi: 10.4103/bc.bc_25_18
105. Santirso, D, Garami, Z, Diaz, O, and Lumsden, A. Ultrasound during neck rotation to reveal a case of positional occlusion of the internal carotid artery. J Ultrasound. (2022) 25:297–300. doi: 10.1007/s40477-020-00490-7
106. Brassart, N, Deforche, M, Goutte, A, and Wery, D. A rare vascular complication of eagle syndrome highlight by CTA with neck flexion. Radiol Case Rep. (2020) 15:1408–12. doi: 10.1016/j.radcr.2020.05.052
107. Kan, P, Srivatsan, A, Johnnson, JN, and Chen, SR. Republished: rotational carotid insufficiency: an unusual cause of bow hunter's syndrome. J Neurointerv Surg. (2019) 11:e9. doi: 10.1136/neurintsurg-2018-014210.rep
108. Rastogi, V, Rawls, A, Moore, O, Victorica, B, Khan, S, Saravanapavan, P, et al. Rare etiology of Bow Hunter's syndrome and systematic review of literature. J Vasc Interv Neurol. (2015) 8:7–16.
109. Devaraja, K. Approach to cervicogenic dizziness: a comprehensive review of its aetiopathology and management. Eur Arch Otorrinolaringol. (2018) 275:2421–33. doi: 10.1007/s00405-018-5088-z
110. Jadeja, N, and Nalleballe, K. Pearls & oy-sters: Bow hunter syndrome: a rare cause of posterior circulation stroke: do not look the other way. Neurology. (2018) 91:329–31. doi: 10.1212/WNL.0000000000006009
111. Cohen, NT, Harrar, DB, Diab, YA, Pearl, MS, and Murnick, JG. Atlanto-occipital ligament calcification: a novel imaging finding in pediatric rotational vertebral artery occlusion. Pediatr Radiol. (2020) 50:137–41. doi: 10.1007/s00247-019-04515-0
112. Bulut, MD, Alpayci, M, Şenköy, E, Bora, A, Yazmalar, L, Yavuz, A, et al. Decreased vertebral artery hemodynamics in patients with loss of cervical lordosis. Med Sci Monit. (2016) 22:495–500. doi: 10.12659/msm.897500
113. Grinenko, EA, Kul'chikov, AE, Musin, RS, and Morozov, SG. The effect of the instability of cervical spine on the hemodynamics in the vertebrobasilar system. Zh Nevrol Psikhiatr Im S S Korsakova. (2014) 114:75–5. doi: 10.17116/jnevro201411412175-77
114. Grinenko, EA, Kulchikov, AE, Musin, RS, and Morozov, SG. Pathogenesis of the vascular consequences caused by cervical instability and their algorithm for pharmacotherapy. Patol Fiziol Eksp Ter. (2015) 59:29–38.
115. Gisolf, J, van Lieshout, JJ, van Heusden, K, Pott, F, Stok, WJ, and Karemaker, JM. Human cerebral venous outflow pathway depends on posture and central venous pressure. J Physiol. (2004) 560:317–27. doi: 10.1113/jphysiol.2004.070409
116. Tartière, D, Seguin, P, Juhel, C, Laviolle, B, and Mallédant, Y. Estimation of the diameter and cross-sectional area of the internal jugular veins in adult patients. Crit Care. (2009) 13:R197. doi: 10.1186/cc8200
117. Yoon, HK, Lee, HK, Jeon, YT, Hwang, JW, Lim, SM, and Park, HP. Clinical significance of the cross-sectional area of the internal jugular vein. J Cardiothorac Vasc Anesth. (2013) 27:685–9. doi: 10.1053/j.jvca.2012.10.007
118. Holmlund, P, Eklund, A, Koskinen, LD, Johansson, E, Sundström, N, Malm, J, et al. Venous collapse regulates intracranial pressure in upright body positions. Am J Physiol Regul Integr Comp Physiol. (2018) 314:R377–85. doi: 10.1152/ajpregu.00291.2017
119. Rangel-Castilla, L, Gasco, J, Nauta, HJ, Okonkwo, DO, and Robertson, CS. Cerebral pressure autoregulation in traumatic brain injury. Neurosurg Focus. (2008) 25:E7. doi: 10.3171/FOC.2008.25.10.E7
120. Jayaraman, MV, Boxerman, JL, Davis, LM, Haas, RA, and Rogg, JM. Incidence of extrinsic compression of the internal jugular vein in unselected patients undergoing CT angiography. AJNR Am J Neuroradiol. (2012) 33:1247–50. doi: 10.3174/ajnr.A2953
121. Sparke, A, Voss, S, and Benger, J. The measurement of tissue interface pressures and changes in jugular venous parameters associated with cervical immobilisation devices: a systematic review. Scand J Trauma Resusc Emerg Med. (2013) 21:81. doi: 10.1186/1757-7241-21-81
122. Farina, R, Foti, PV, Pennisi, I, Conti, A, Meli, GA, Vasile, T, et al. Stylo-jugular venous compression syndrome: lessons based on a case report. Am J Case Rep. (2021) 22:e932035. doi: 10.12659/AJCR.932035
123. Ball, AK, and Clarke, CE. Idiopathic intracranial hypertension. Lancet Neurol. (2006) 5:433–42. doi: 10.1016/S1474-4422(06)70442-2
124. Giuseffi, V, Wall, M, Siegel, PZ, and Rojas, PB. Symptoms and disease associations in idiopathic intracranial hypertension (pseudotumor cerebri): a case-control study. Neurology. (1991) 41:239–44. doi: 10.1212/wnl.41.2_part_1.239
125. Wostyn, P, Audenaert, K, and Deyn, D. Alzheimer's disease-related changes in diseases characterized by elevation of intracranial or intraocular pressure. Clin Neurol Neurosurg. (2008) 110:101–9. doi: 10.1016/j.clineuro.2007.10.011
126. Wostyn, P. Can chronic increased intracranial pressure or exposure to repetitive intermittent intracranial pressure elevations raise your risk for Alzheimer's disease? Med Hypotheses. (2004) 62:925–30. doi: 10.1016/j.mehy.2004.01.013
127. Mangalore, S, Rakshith, S, and Srinivasa, R. Solving the riddle of "idiopathic" in idiopathic intracranial hypertension and Normal pressure hydrocephalus: an imaging study of the possible mechanisms – Monro-Kellie 3.0. Asian J Neurosurg. (2019) 14:440–52. doi: 10.4103/ajns.AJNS_252_18
128. Chen, S, Chen, Y, Xu, L, Matei, N, Tang, J, Feng, H, et al. Venous system in acute brain injury: mechanisms of pathophysiological change and function. Exp Neurol. (2015) 272:4–10. doi: 10.1016/j.expneurol.2015.03.007
129. Doepp, F, Schreiber, SJ, von Münster, T, Rademacher, J, Klingebiel, R, and Valdueza, JM. How does the blood leave the brain? A systematic ultrasound analysis of cerebral venous drainage patterns. Neuroradiology. (2004) 46:565–70. doi: 10.1007/s00234-004-1213-3
130. Rasmussen, JC, Kwon, S, Pinal, A, Bareis, A, Velasquez, FC, Janssen, CF, et al. Assessing lymphatic route of CSF outflow and peripheral lymphatic contractile activity during head-down tilt using near-infrared fluorescence imaging. Physiol Rep. (2020) 8:e14375. doi: 10.14814/phy2.14375
131. Shapiro, R, Youngberg, AS, and Rothman, SL. The differential diagnosis of traumatic lesions of the occipito-atlanto-axial segment. Radiol Clin North Am. (1973) 11:505–26. doi: 10.1016/S0033-8389(22)01669-4
132. Gooding, CA, and Stimac, GK. Jugular vein obstruction caused by turning of the head. AJR Am J Roentgenol. (1984) 142:403–6. doi: 10.2214/ajr.142.2.403
133. Watson, GH. Effect of head rotation on jugular vein blood flow. Arch Dis Child. (1974) 49:237–9. doi: 10.1136/adc.49.3.237
134. Fischer, GW, and Scherz, RG. Neck vein catheters and pericardial tamponade. Pediatrics. (1973) 52:868–71. doi: 10.1542/peds.52.6.868
135. Potts, DG, and Deonarine, V. Effect of positional changes and jugular vein compression on the pressure gradient across the arachnoid villi and granulations of the dog. J Neurosurg. (1973) 38:722–8. doi: 10.3171/jns.1973.38.6.0722
136. Højlund, J, Sandmand, M, Sonne, M, Mantoni, T, Jørgensen, HL, Belhage, B, et al. Effect of head rotation on cerebral blood velocity in the prone position. Anesthesiol Res Pract. (2012) 2012:647258. doi: 10.1155/2012/647258
137. Hulme, A, and Cooper, R. The effect of head position and jugular vein compression on intracranial pressure: a clinical study. In: JWF Beks, DA Bosch, and M Brock, editors. International symposium on intracranial pressure III. Berlin: Springer-Verlag (1978). 259–63.
138. Schreiber, SJ, Lurtzing, F, Gotze, R, Doepp, F, Klingebiel, R, and Valdueza, JM. Extrajugular pathways of human cerebral venous blood drainage assessed by duplex ultrasound. J Appl Physiol (1985). (2003) 94:1802–5. doi: 10.1152/japplphysiol.00782.2002
139. Ding, JY, Zhou, D, Pan, LQ, Ya, JY, Liu, C, Yan, F, et al. Cervical spondylotic internal jugular venous compression syndrome. CNS Neurosci Ther. (2020) 26:47–54. doi: 10.1111/cns.13148
140. Ghosh, LM, and Dubey, SP. The syndrome of elongated styloid process. Auris Nasus Larynx. (1999) 26:169–75. doi: 10.1016/s0385-8146(98)00079-0
141. Custodio, ALN, MRM, A e S, and Abreu, MH. Styloid process of the temporal bone: morphometric analysis and clinical implications. Biomed Res Int. (2016) 2016:8792725. doi: 10.1155/2016/8792725
142. Piagkou, M, Anagnostopoulou, S, Kouladouros, K, and Piagkos, G. Eagle's syndrome: a review of the literature. Clin Anat. (2009) 22:545–58. doi: 10.1002/ca.20804
143. Zamboni, P, Scerrati, A, Menegatti, E, Galeotti, R, Lapparelli, M, Traina, L, et al. The eagle jugular syndrome. BMC Neurol. (2019) 19:333. doi: 10.1186/s12883-019-1572-3
144. de Andrade, KM, Rodrigues, CA, Watanabe, PC, and Mazzetto, MO. Styloid process elongation and calcification in subjects with tmd: clinical and radiographic aspects. Braz Dent J. (2012) 23:443–50. doi: 10.1590/s0103-64402012000400023
145. Frommer, J. Anatomic variations in the stylohyoid chain and their possible clinical significance. Oral Surg Oral Med Oral Pathol. (1974) 38:659–67. doi: 10.1016/0030-4220(74)90382-x
146. Gweon, HM, Chun g, TS, and Suh, SH. Evaluation of the cause of internal jugular vein obstruction of head and neck contrast enhanced 3D MR angiography using contrast enhanced computed tomography. J Magn Reson Imaging. (2011) 15:41–7. doi: 10.13104/jksmrm.2011.15.1.41
147. Bezerra, MLS, Ferreira, ACAF, and de Oliveira-Souza, R. Pseudotumor Cerebri and Glymphatic dysfunction. Front Neurol. (2018) 8:734. doi: 10.3389/fneur.2017.00734
148. Wall, M. Idiopathic intracranial hypertension. Neurol Clin. (2010) 28:593–617. doi: 10.1016/j.ncl.2010.03.003
149. Mavrocordatos, P, Bissonnette, B, and Ravussin, P. Effects of neck position and head elevation on intracranial pressure in anaesthetized neurosurgical patients: preliminary results. J Neurosurg Anesthesiol. (2000) 12:10–4. doi: 10.1097/00008506-200001000-00003
150. Eide, PK, and Kerty, E. Static and pulsatile intracranial pressure in idiopathic intracranial hypertension. Clin Neurol Neurosurg. (2011) 113:123–8. doi: 10.1016/j.clineuro.2010.10.008
151. Vogh, BP, Godman, DR, and Maren, TH. Effect of AlCl3 and other acids on cerebrospinal fluid production: a correction. J Pharmacol Exp Ther. (1987) 243:35–9.
152. Wall, M. Update on idiopathic intracranial hypertension. Neurol Clin. (2017) 35:45–57. doi: 10.1016/j.ncl.2016.08.004
153. Bjornson, A, Tapply, I, Nabbanja, E, Lalou, AD, Czosnyka, M, Czosnyka, Z, et al. Ventriculo-peritoneal shunting is a safe and effective treatment for idiopathic intracranial hypertension. Br J Neurosurg. (2019) 33:62–70. doi: 10.1080/02688697.2018.1538478
154. Lueck, C, and McIlwaine, G. Interventions for idiopathic intracranial hypertension, p. 1–10. The Cochrane Library. Hoboken, New Jersey: John Wiley & Sons, Ltd; (2009).
155. Haavik, H, Kumari, N, Holt, K, Niazi, IK, Amjad, I, Pujari, AN, et al. The contemporary model of vertebral column joint dysfunction and impact of high-velocity, low-amplitude controlled vertebral thrusts on neuromuscular function. Eur J Appl Physiol. (2021) 121:2675–720. doi: 10.1007/s00421-021-04727-z
156. Senzon, SA. The chiropractic vertebral subluxation part 1: introduction. J Chiropr Humanit. (2019) 25:10–21. doi: 10.1016/j.echu.2018.10.002
157. Le Huec, JC, Thompson, W, Mohsinaly, Y, Barrey, C, and Faundez, A. Sagittal balance of the spine. Eur Spine J. (2019) 28:1889–905. doi: 10.1007/s00586-019-06083-1
158. Tang, Y, Xu, X, Zhu, F, Chen, C, Wang, F, Lu, M, et al. Incidence and risk factors of cervical kyphosis in patients with adolescent idiopathic scoliosis. World Neurosurg. (2019) 127:e788–92. doi: 10.1016/j.wneu.2019.03.264
159. Mahmoud, NF, Hassan, KA, Abdelmajeed, SF, Moustafa, IM, and Silva, AG. The relationship between forward head posture and neck pain: a systematic review and Meta-analysis. Curr Rev Musculoskelet Med. (2019) 12:562–77. doi: 10.1007/s12178-019-09594-y
160. Gadia, A, Shah, K, and Nene, A. Cervical kyphosis. Asian Spine J. (2019) 13:163–72. doi: 10.31616/asj.2018.0086
161. Ames, CP, Blondel, B, Scheer, JK, Schwab, FJ, Le Huec, JC, Massicotte, EM, et al. Cervical radiographical alignment: comprehensive assessment techniques and potential importance in cervical myelopathy. Spine (Phila Pa 1976). (2013) 38:S149–60. doi: 10.1097/BRS.0b013e3182a7f449
162. Hayashi, T, Wang, JC, Suzuki, A, Takahashi, S, Scott, TP, Phan, K, et al. Risk factors for missed dynamic canal stenosis in the cervical spine. Spine (Phila Pa 1976). (2014) 39:812–9. doi: 10.1097/BRS.0000000000000289
163. Eriksen, K, Rochester, RP, and Hurwitz, EL. Symptomatic reactions, clinical outcomes and patient satisfaction associated with upper cervical chiropractic care: a prospective, multicenter, cohort study. BMC Musculoskelet Disord. (2011) 12:219. doi: 10.1186/1471-2474-12-219
164. Gerstin, G, Oakley, PA, and Harrison, DE. The treatment of dizziness by improving cervical lordosis: a chiropractic BioPhysics® case report. J Phys Ther Sci. (2020) 32:864–8. doi: 10.1589/jpts.32.864
165. Gao, K, Zhang, J, Lai, J, Liu, W, Lyu, H, Wu, Y, et al. Correlation between cervical lordosis and cervical disc herniation in young patients with neck pain. Medicine (Baltimore). (2019) 98:e16545. doi: 10.1097/MD.0000000000016545
166. Shen, XL, Tian, Y, Zhou, XH, Ren, D, Cao, P, and Yuan, W. A radiographic analysis of cervical sagittal alignment in adolescent idiopathic cervical kyphosis. Clin Spine Surg. (2017) 30:E560–6. doi: 10.1097/BSD.0000000000000257
167. Moustafa, IM, Diab, AA, and Harrison, DE. The effect of normalizing the sagittal cervical configuration on dizziness, neck pain, and cervicocephalic kinesthetic sensibility: a 1-year randomized controlled study. Eur J Phys Rehabil Med. (2017) 53:57–71. doi: 10.23736/S1973-9087.16.04179-4
168. Woodfield, HC 3rd, York, C, Rochester, RP, Bales, S, Beebe, M, Salminen, B, et al. Craniocervical chiropractic procedures – a précis of upper cervical chiropractic. J Can Chiropr Assoc. (2015) 59:173–92.
169. Oakley, PA, Kallan, SZ, and Harrison, DE. Structural rehabilitation of the cervical lordosis and forward head posture: a selective review of chiropractic BioPhysics® case reports. J Phys Ther Sci. (2022) 34:759–71. doi: 10.1589/jpts.34.759
170. Morningstar, M. Cervical curve restoration and forward head posture reduction for the treatment of mechanical thoracic pain using the pettibon corrective and rehabilitative procedures. J Chiropr Med. (2002) 1:113–5. doi: 10.1016/S0899-3467(07)60013-5
171. Moustafa, IM, Diab, AA, and Harrison, DE. The efficacy of cervical lordosis rehabilitation for nerve root function and pain in cervical Spondylotic radiculopathy: a randomized trial with 2-year follow-up. J Clin Med. (2022) 11:6515. doi: 10.3390/jcm11216515
172. Hauser, R, Dolan, E, Phillips, H, Newlin, A, Moore, R, and Woldin, B. Ligament injury and healing: a review of current clinical diagnostics and therapeutics. Open Rehabil J. (2013) 6:1–20. doi: 10.2174/1874943701306010001
173. Hackett, G, Hemwall, GA, and Montgomery, GA. (1993). Ligament and tendon relaxation treated by prolotherapy, 5th ed., p. 94–96. Gustav A. Hemwall. Oak Park, Illinois.
174. Giordano, L, Murrell, WD, and Maffulli, N. Prolotherapy for chronic low back pain: a review of literature. Br Med Bull. (2021) 138:96–111. doi: 10.1093/bmb/ldab004
175. Hackett, GS, and Henderson, DG. Joint stabilization; an experimental, histologic study with comments on the clinical application in ligament proliferation. Am J Surg. (1955) 89:968–73. doi: 10.1016/0002-9610(55)90568-7
176. Liu, YK, Tipton, CM, Matthes, RD, Bedford, TG, Maynard, JA, and Walmer, HC. An in situ study of the influence of a sclerosing solution in rabbit medial collateral ligaments and its junction strength. Connect Tissue Res. (1983) 11:95–102. doi: 10.3109/03008208309004846
177. Klein, R, Dorman, T, and Johnson, C. Proliferant injections for low back pain histologic changes of injected ligaments and objective measurements of lumbar spine mobility before and after treatment. J Neuro Orthop Med Surg. (1989) 10:123–6.
178. Hackett, GS. Prolotherapy in whiplash and low back pain. Postgrad Med. (1960) 27:214–9. doi: 10.1080/00325481.1960.11712804
179. Hackett, GS, Huang, TC, and Raftery, A. Prolotherapy for headache. Pain in the head and neck, and neuritis. Headache. (1962) 2:20–8. doi: 10.1111/j.1526-4610.1962.hed0201020.x
180. Kayfetz, DO, Blumenthal, LS, Hackett, GS, Hemwall, GA, and Neff, FE. Whiplash injury and other ligamentous headache--its management with prolotherapy. Headache. (1963) 3:21–8. doi: 10.1111/j.1526-4610.1963.hed0301021.x
181. Hooper, RA, Frizzell, JB, and Faris, P. Case series on chronic whiplash related neck pain treated with intraarticular zygapophysial joint regeneration injection therapy. Pain Physician. (2007) 10:313–8. doi: 10.36076/ppj.2007/10/313
182. Centeno, CJ, Elliott, J, Elkins, WL, and Freeman, M. Fluoroscopically guided cervical prolotherapy for instability with blinded pre and post radiographic reading. Pain Physician. (2005) 8:67–72.
Keywords: cervical instability, cervical ligament injury, cervical lordosis, dysstructure, jugular vein compression, intracranial hypertension
Citation: Hauser RA, Matias D and Rawlings B (2024) The ligamentous cervical instability etiology of human disease from the forward head-facedown lifestyle: emphasis on obstruction of fluid flow into and out of the brain. Front. Neurol. 15:1430390. doi: 10.3389/fneur.2024.1430390
Received: 09 May 2024; Accepted: 07 November 2024;
Published: 27 November 2024.
Edited by:
David Saperstein, Center for Complex Neurology, EDs & POTS, United StatesReviewed by:
Fraser Henderson, University Maryland CRMC, United StatesCopyright © 2024 Hauser, Matias and Rawlings. This is an open-access article distributed under the terms of the Creative Commons Attribution License (CC BY). The use, distribution or reproduction in other forums is permitted, provided the original author(s) and the copyright owner(s) are credited and that the original publication in this journal is cited, in accordance with accepted academic practice. No use, distribution or reproduction is permitted which does not comply with these terms.
*Correspondence: B. Rawlings, cmF3bGluZ3NiQGNhcmluZ21lZGljYWwuY29t
Disclaimer: All claims expressed in this article are solely those of the authors and do not necessarily represent those of their affiliated organizations, or those of the publisher, the editors and the reviewers. Any product that may be evaluated in this article or claim that may be made by its manufacturer is not guaranteed or endorsed by the publisher.
Research integrity at Frontiers
Learn more about the work of our research integrity team to safeguard the quality of each article we publish.