- 1Department of Physiology and Biomedical Engineering, Mayo Clinic, Rochester, MN, United States
- 2School of Biomedical Sciences, St Lucia, QLD, Australia
- 3Queensland Brain Institute, St Lucia, QLD, Australia
In amyotrophic lateral sclerosis (ALS) postmortem tissue and the SOD1 mouse model at mid-disease, death of hypoglossal motor neurons (XII MNs) is evident. These XII MNs innervate the intrinsic and extrinsic tongue muscles, and despite their importance in many oral and lingual motor behaviours that are affected by ALS (e.g., swallowing, speech, and respiratory functions), little is known about the timing and extent of tongue muscle denervation. Here in the well-characterised SOD1G93A (high-copy) mouse model, we evaluated XII MN numbers and intrinsic tongue muscle innervation using standard histopathological approaches, which included stereological evaluation of Nissl-stained brainstem, and the presynaptic and postsynaptic evaluation of neuromuscular junctions (NMJs), using synapsin, neurofilament, and α-bungarotoxin immunolabelling, at presymptomatic, onset, mid-disease, and endstage timepoints. We found that reduction in XII MN size at onset preceded reduced XII MN survival, while the denervation of tongue muscle did not appear until the endstage. Our study suggests that denervation-induced weakness may not be the most pertinent feature of orolingual deficits in ALS. Efforts to preserve oral and respiratory functions of XII MNs are incredibly important if we are to influence patient outcomes.
Introduction
Death of corticospinal neurons and the motor neurons (MNs) that innervate skeletal muscle is the pathognomic feature of amyotrophic lateral sclerosis (ALS) (1, 2). In ALS, disruption of the neuromotor system and muscle denervation leads to weakness and eventual death by respiratory insufficiency within 3 years of diagnosis (3, 4). Hence, respiratory neuromotor impairments have a strong association with disease morbidity and mortality, with ALS having effects on ventilatory (breathing) and non-ventilatory (cough and sneeze) respiratory behaviours, as well as orolingual and aerodigestive behaviours including speech and swallowing (3, 5). All of these behaviours involve some aspects of tongue musculature (6–8). These deficits (including insufficient cough and dysphagia) result in an increased risk of airway obstruction and aspiration pneumonia (4).
In patients, hypoglossal (XII) MN loss is apparent in human postmortems (2). In the most widely used murine model of ALS, hSOD1G93A (SOD1) hypoglossal MN death is observed at postnatal (P) day 90 (early-disease) and endstage (~P140) in SOD1 mice (9) and rats (10). Across multiple avenues of investigation, fast fatigueable (type FF) motor units, comprising larger MNs and higher force-producing type IIx/IIb muscle fibres they innervate are vulnerable to ALS (11–14). By contrast, slow and fast fatigue-resistant (type S and FR units, respectively) motor units, comprising smaller MNs innervating low-force producing type I and IIa muscle fibres, are relatively resilient. These findings are consistent with the survival of smaller XII MNs in postmortem patients (2) and murine brainstem (9). The pattern of FF-type MN death and muscle fibre denervation underpins the specific behavioural vulnerability of higher-force requiring behaviours (e.g., cough) observed in ALS (4).
At presymptomatic ages [prior to MN death (9)], XII MNs of SOD1 mice have altered intrinsic and extrinsic excitability (15) and dendritic abnormalities (16) that were exclusive to larger XII MNs (17). However, it is unknown whether this early pathophysiology is matched by presymptomatic changes in XII MN survival or tongue muscle innervation, as evident in Tibialis anterior motor units in these SOD1G93A mice (18). In this study, we assessed XII MN survival and intrinsic tongue muscle innervation at presymptomatic, onset, mid-disease, and endstage. We hypothesise that there will be a loss of larger XII MN concomitant with tongue NMJ denervations at disease onset in SOD1G93A mice.
Methods
Ethics approval and experimental animals
All procedures were performed in accordance with national and international guidelines and were approved by the University of Queensland Animal Ethics Committee. Female and male age and litter-matched wild-type (WT; n = 25 for the fixed brainstem histology and n = 16 for frozen tongue evaluations) and high-copy number heterozygote hSOD1G93A (SOD1; n = 24 for the fixed brainstem histology and n = 16 for frozen tongue evaluations) were used at four pre-defined disease stages; presymptomatic (P30), onset (P70), mid-disease (P100–120), and endstage (P130–150) (16, 19). Endstage and humane endpoints for euthanasia were determined by a paralysis of the hindlimbs and the lack of righting reflex. Animals were obtained from our female WT x male SOD1 heterozygote breeding scheme, with pups genotyped and then randomly assigned to each experimental group. Animals were maintained in filtered cages under a 12:12-h light–dark cycle with ad libitum access to chow and water. In all experiments, mice were deeply anaesthetised with 60–80 mg/kg of pentobarbitone (Vetcare), with levels verified by the loss of palpebral and pain reflexes.
XII MN histology
Following deep anaesthesia, mice were intracardially perfused with phosphate-buffered saline pH 7.4 (PBS, 0.1 M) and then 4% paraformaldehyde in PBS. The brainstem was removed, sunk in 30% sucrose, subsequently frozen in liquid nitrogen, sectioned into 16 μm serial transverse cryosections, stained with 0.1% thionin (v/v in an acetic acid buffer), and imaged using a Zeiss Axioskop II microscope. XII MNs were quantified bilaterally with counts of every 5th section (20–22) with anatomical regions located with the aid of a brain atlas (23) and the 4th ventricle and central canal used as key landmarks. To estimate the XII MN volumes, the x, y, and z diameter of every 10th XII MN was provided for the volume of an ellipsoid.
NMJ assessment
Following deep anaesthesia, the tongue blade was removed, and 40 μm transverse vibratome sections were cut and stained for presynaptic and postsynaptic elements of the NMJ (24). Acetylcholine receptors (AChRs) were located using Alexa-555 conjugated α-bungarotoxin [BTX; diluted 1:500 in PBS; Sigma MO, USA], and motor nerve endings were located with rabbit anti-SV2 1 [1:50, Sigma] and anti-neurofilament [1:200, Sigma], with secondary Alexa-488 goat anti-rabbit [1:500, Invitrogen] as previously described (25). All antibodies were diluted in a blocking buffer. Intrinsic tongue muscles were imaged using a Zeiss LSM 900 confocal microscope using Airyscan detectors in multiplex 4y mode using a 63x oil objective N.A. 1.4. Approximately 25 tongue NMJs were imaged per mouse, across the proximal middle and distal regions of the tongue blade. The percentage overlap between presynaptic synapsin-NF and BTX-labelled postsynaptic AChRs was used to classify innervated, partially, or fully denervated muscle fibres (18).
Statistical analysis
Prism 9 was used for all analyses (GraphPad, Carlsbad, CA), which were performed blind to genotype. For all comparisons, two-way ANOVAs with Bonferroni post-hoc tests were performed, with statistical significance established at a p-value of <0.05. All data were reported as the mean ± 95% CI of the mean.
Results
Loss of bodyweight by endstage in SOD1 mice
Both female and male mice gained weight until mid-disease, where endstage SOD1 mice (19.3 ± 1.2 g, n = 13) weighed ~12% less than WT controls (22.1 ± 1.3 g, n = 13; p = 0.0123, unpaired t-test).
Loss of larger XII MNs in SOD1 mice
XII MN numbers were evaluated bilaterally in the entire XII nucleus in WT and SOD1 mice (Figure 1). XII MN loss was dependent on genotype (F (1.41) = 16.5, p = 0.0002) and genotype–age interactions (F (3.41) = 4.0, p = 0.014; two-way ANOVA), with significant reductions (~25%) at mid-disease (WT: 2247 ± 330, n = 7; SOD1: 1678 ± 336, n = 6; p = 0.023) and endstage (~41%; WT: 2236 ± 304, n = 9; SOD1: 1328 ± 294, n = 8; p < 0.0001) in SOD1 mice (Bonferroni post-tests; Figure 1B). No differences in XII MN number were apparent at presymptomatic (WT: 2144 ± 429, n = 4; SOD1: 2123 ± 336, n = 5; p > 0.99) or onset ages (WT: 2136 ± 314, n = 5; SOD1: 1945 ± 550, n = 5; p > 0.99; Bonferroni post-tests; Figure 1B). In SOD1 mice at mid-disease stages, extensive vacuolation of the hypoglossal nucleus was apparent, similar to that previously observed at endstage (26).
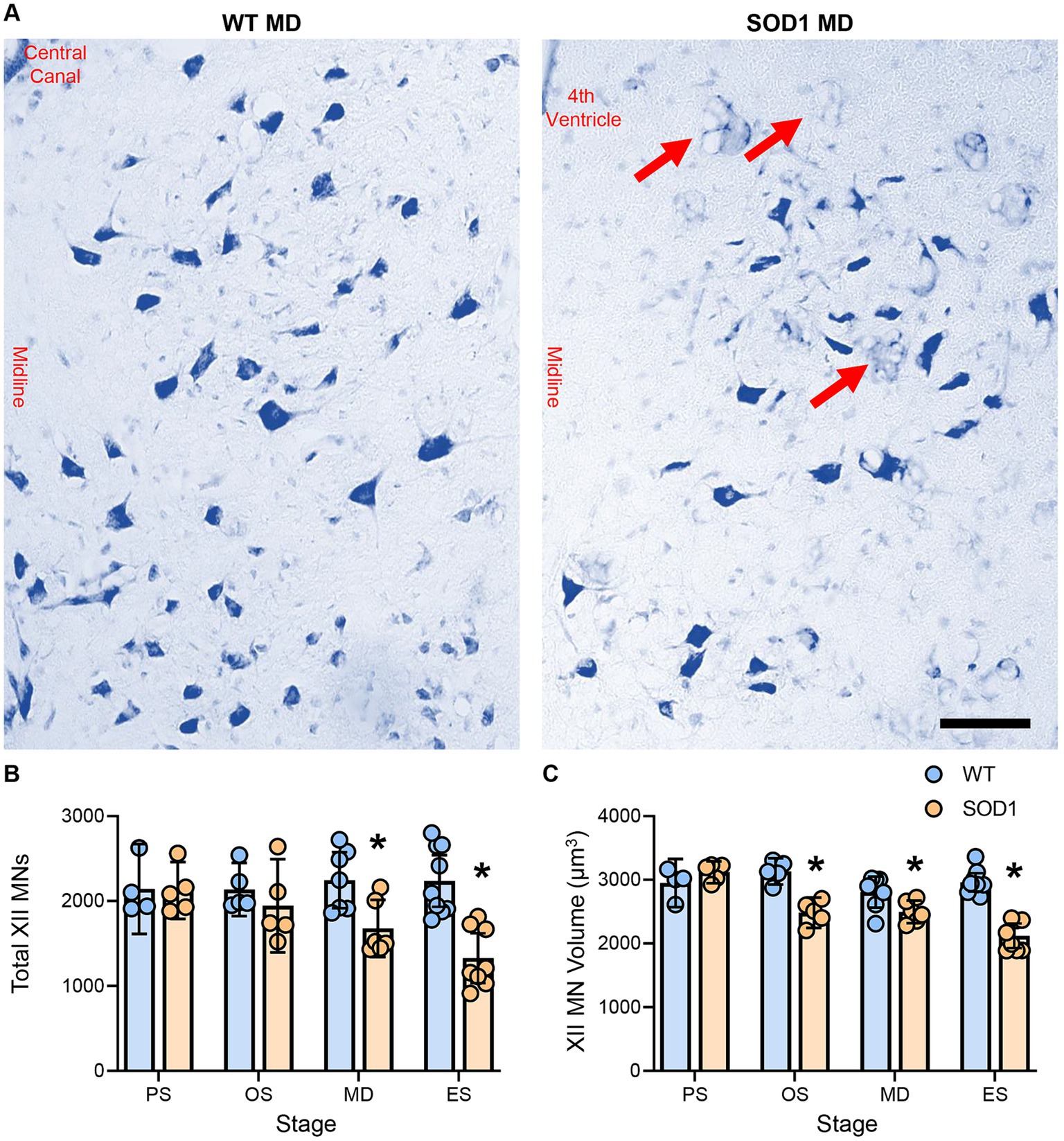
Figure 1. (A) Photomicrographs of the Nissl-stained brainstem and XII MNs in WT and SOD1 mice at mid-disease (MD). (B) Plot showing a reduced number of XII MNs in SOD1 mice at mid-disease and endstage (ES) but not presymptomatic (PS) or onset (OS). Note that the XII MN number is relatively stable until mid-disease, where ~25% of XII MNs have perished. Red arrows indicate vacuolated regions within the hypoglossal nucleus from mid-disease in SOD1 mice. (C) Scatterplot showing reduced XII MN volume in SOD1 mice from the onset. These somal changes are not the earliest alterations in XII MN morphology, but they are consistent with the resilience of type S and FR motor units. Two-way ANOVAs with Bonferroni post-tests, * indicates p < 0.05 between genotypes within an age group. Each symbol represents one mouse (i.e., the n). Scale bar = 100 μm.
XII MN volume was dependent on genotype (F (1.41) = 45.0, p < 0.0001) and genotype–age interactions (F (3.41) = 12.4, p < 0.0001; two-way ANOVA), with significant reductions at onset (~21%; WT: 3132 ± 207 μm2, n = 5; SOD1: 2484 ± 238 μm2, n = 5; p < 0.0001), mid-disease (~11%; WT: 2808 ± 244 μm2, n = 7; SOD1: 2498 ± 178 μm2, n = 6; p = 0.0386), and endstage (~28%; WT: 2960 ± 145 μm2, n = 9; SOD1: 2121 ± 194 μm2, n = 8; p < 0.0001) in SOD1 mice (Bonferroni post-tests; Figure 1C). No difference in XII MN volume was apparent in presymptomatic mice (WT: 2950 ± 389 μm2, n = 4; SOD1: 3120 ± 171 μm2, n = 5; p > 0.99; Bonferroni post-test; Figure 1C).
Tongue NMJ denervation occurs subsequent to XII MN loss in SOD1 mice
Intrinsic tongue muscle NMJs were evaluated whole-mount within 40-μm transverse cryosections of the tongue blade (Figure 2). The % of NMJ endplates that were innervated was dependent on genotype (F (1.24) = 17.6, p = 0.0003) and genotype–age interactions (F (3.24) = 7.1, p < 0.0014; two-way ANOVA). We did not observe any denervated fibres in WT mice, and denervation was rare in SOD1 mice at presymptomatic (100% innervated), onset (~99% innervated), and mid-disease (~97% innervated). However, at endstage, there was an 11% reduction of innervated fibre SOD1 mice, compared to WT (SOD1: 89 ± 10%; p < 0.0001, Bonferroni post-test; n = 4 all ages/groups; Figure 2B).
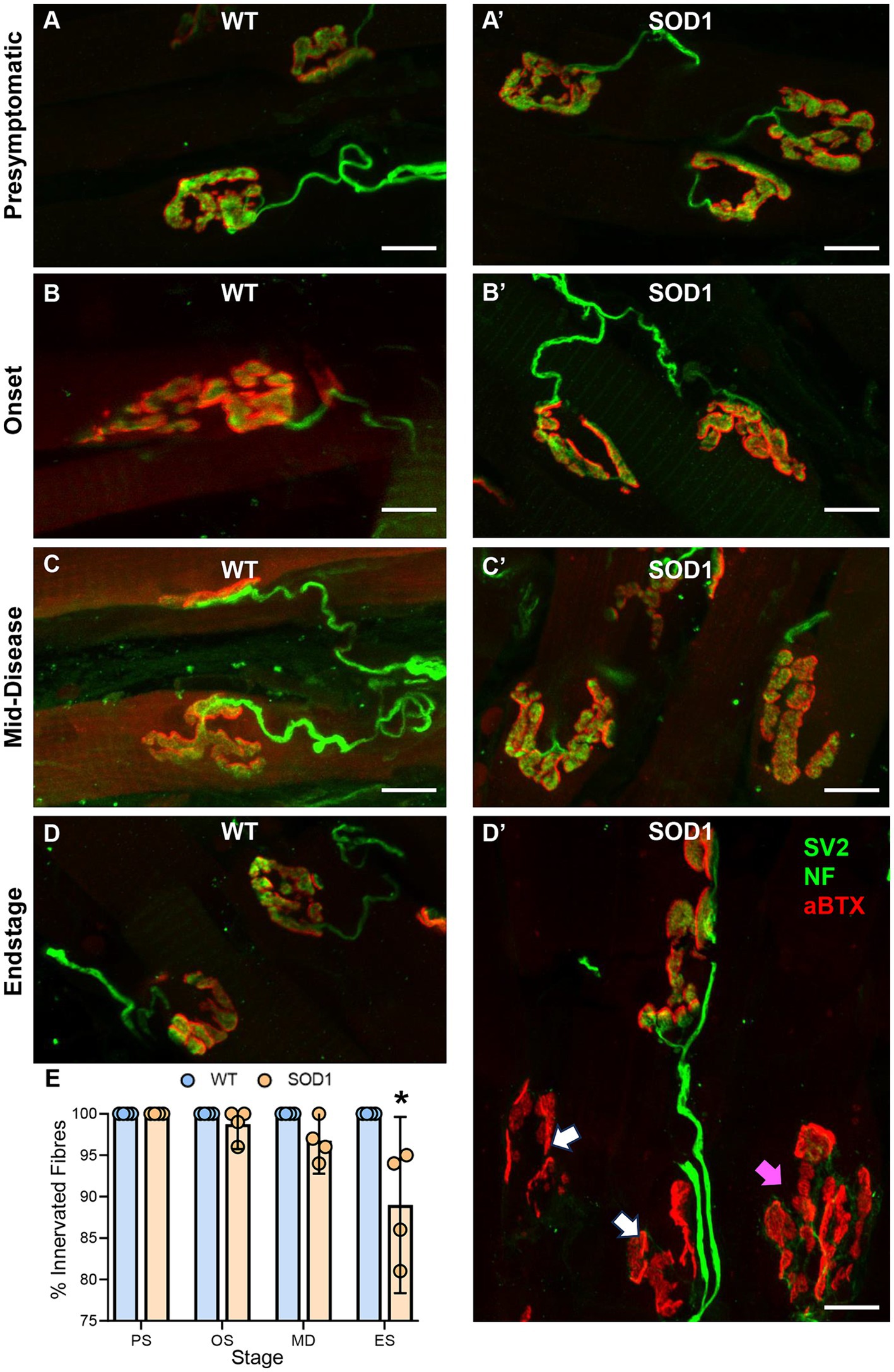
Figure 2. (A–D’) Show flattened z-stack projections of NMJs from wild-type (WT, left column A–D) to aged match SOD1G93A (SOD1, right column, A’–D’), at all stages of ALS-like disease progression, including, presymptomatic (PS), onset (OS), mid-disease (MD), and end-stage (ES). In panel (D’), white arrows indicate denervated endplates at ES, and purple arrows indicate partially denervated endplates. In all panels, presynaptic components of the NMJ (green) are labelled with antibodies to SV2 and neurofilament (NF), while postsynaptic acetylcholine receptors (red) are located with αBTX. (E) Scatterplot shows a reduction in the percentage (%) of innervated muscle fibres in SOD1G93A mice at endstage, following XII MN loss, with innervation unchanged at presymptomatic, onset, and mid-disease. Two-way ANOVA with Bonferroni post-tests. * indicates p < 0.05 between genotypes within an age group. Each symbol represents one animal (i.e., the n) with data based on ~25 NMJs per tongue. Scale bars = 10 μm.
Discussion
In the present study, we report the first longitudinal study of XII MN size-dependent survival and tongue blade innervation in SOD1 mice. This study has important implications regarding the interpretation of pathophysiological changes in young SOD1 XII MNs, and in relation to the natural history of MN death and denervation in SOD1 mice more generally. Notably, our current findings suggest that in the case of orolingual and aerodigestive behaviours, disordered neural networks altering the pattern of MN activation may be a more salient feature of dysfunction than muscle denervation and weakness per se, as maximal tongue strength seems maintained in SOD1 rats (27) and when corrected for baseline values in SOD1 mice (28). For behaviours such as swallowing, where multiple brainstem MN pools are activated, swallow deficits occur in SOD1 mice (26, 29) and rats (27) in the absence of reduced nucleus ambiguus MN density counts (10), although stereological assessments of MN numbers and detailed assessment of the computational capacity of these nucleus ambiguus MNs have not been comprehensively assessed [(see 30)]. How aberrant brainstem neural circuitry and synaptic transmission very early in the pathogenesis of SOD1 mice (15–17) influences orolingual and aerodigestive activities at later disease stages (26, 29) remains unclear. Exploring these nuances may yield sensitive diagnostic hallmarks and widen therapeutic windows.
Our findings of XII MN loss from mid-disease are not surprising given the prior studies in P90 and endstage SOD1 mice (9, 26). Here, we extend that finding by showing that XII MN loss is not apparent at younger ages, despite widespread presymptomatic functional and morphological changes (15–17). In a major advance, we show that at onset, the surviving XII MNs of SOD1 mice are smaller than their WT comparators, consistent with reduced volumes at mid-disease and endstage. This observation suggests that the larger MNs, likely comprising type FF motor units, are vulnerable to loss (2, 9, 12–14). Indeed, the major differences in XII MNs compared to lumbar MNs in SOD1 mice seem to be their capacity to compensate for their altered synaptic milieu which is evident in neonates (15).
In XII MNs, morphological changes consistent with lowered intrinsic MN activity (i.e., dendritic expansion) are present presymptomatically in the larger more vulnerable XII MNs (16, 17). By contrast, in the more rapidly degenerating lumbar MNs and corticospinal neurons, neuronal death is apparent presymptomatically or at onset (18, 31). Notably, corticospinal neurons and lumbar MNs do not exhibit neuroplastic changes consistent with compensation but display degenerative morphologies (16, 32, 33). This compensation is not related to the respiratory neuromotor system in general, as there are marked deficits in respiratory MNs and muscles in SOD1 models (34), including the tongue (35).
Despite evidence of XII MN loss (2, 9), degeneration (26), and a plethora of early pathophysiological changes (15–17), the integrity of tongue NMJs has not been evaluated in ALS. Here, we show that gross NMJ morphology is relatively well preserved in SOD1 mice, with pathology largely absent until endstage, similar to SOD1 rats (10, 27). Importantly, our NMJ assessments do not discriminate the dynamic re-innervation of tongue muscle fibres, where formerly denervated fibres are innervated by nascent axonal projections from surviving XII MNs or fibres that have been totally unaffected. This is a key caveat to our interpretations as it has been shown that dynamic structural and functional NMJ remodelling precedes MN death in the limb muscles of SOD1G93A mice (36, 37). Perhaps most relevant to ALS are recent observations in muscle from early staged ALS patients who show type 1 fibre type grouping is evident during the onset of leg muscle weakness in ALS patients, supporting the idea of re-innervation by resilient type S MNs (11). These observations, along with the inclusion of some type I and IIa fibres in the mouse tongue (38), may confound our NMJ evaluations. Regardless, the timing of pathology, including XII MN loss occurring just prior to NMJ denervation, suggests that the complete denervation of NMJs may be a consequence, not a cause of MN deficits in SOD1G93A mice, consistent with neonatal XII MN dysfunction in this ALS model (15).
In the respiratory neuromotor system, expulsive behaviours, such as cough and sneeze, requiring the activation of type FF motor units, are impaired in ALS patients (4), consistent with ALS pathology selectively afflicting larger MNs (2). For the tongue muscles, ballistic-type activations occur during swallowing, which is impaired in ALS (3). There are few ways to directly assess the maximal activations of individual intrinsic and extrinsic tongue muscles. Reduced uniaxial approaches (complicated by the complex interdigitation of tongue musculature) give unequivocal maximum specific forces at the cost of being ex vivo, while in vivo nerve stimulation may be confounded by NMJ or axonal pathology (6). Alternatively, behavioural tasks, such as licking and swallowing, altered in low-copy SOD1G93A mice (29, 39), can be evaluated, although interpretations are couched, as the involvement of other muscle groups is required for these activities (particularly the nucleus ambiguus for swallowing). Regardless, these tongue deficiencies are also a likely cause of the poor grooming and coat conditions of SOD1 mice from both strains.
As ALS is an age-associated neurodegenerative condition, separating the ravages of age from the effects of the disease, particularly in animal models, is often overlooked. In rodents (20, 40–43) and humans (44, 45), ageing-related XII MN loss, tongue weakness, and impaired orolingual and aerodigestive behaviours are readily observed. An important goal is to uncover the precise pathophysiological phenomena differentiating ALS from old age sarcopenia (cf old age vigour, or healthy ageing). To this end, the low-copy number SOD1G93A mice have been employed, with their onset of symptoms is delayed compared to the high-copy number strain used in the present study (46). Although swallowing and licking behavioural deficits are identified (29, 39) and smaller genioglossal muscle fibre cross-sectional areas are evident at endstage (39), corticospinal involvement has not been clarified [(see 47)], and thus, the validity of this strain is unverified. Notably, the endstage in the low-copy strain occurs at ~240 days (29, 46), barely aged enough to be considered “young” in rodent ageing studies (48). Efforts to create animal models where the timing of abnormalities can be controlled (i.e., where MN death can commence in ages approaching higher risk in humans) are likely to be highly informative and are within our grasp (49–51).
In conclusion, we have novel results showing a relative resilience of tongue muscle to denervation in SOD1G93A mice, and XII MN loss consistent with prior neural (9) and behavioural (26, 29, 52) assessments. These results are also largely in agreement with unaltered maximal licking force (27) and absent (27) to mild (10) evidence of tongue denervation in SOD1 rats. In addition, we show altered morphology of XII MNs, consistent with the vulnerability of type FF motor units occurring prior to statistically robust XII MN death and tongue denervation. Whether the relative delay in XII MN and tongue denervation compared to limb MNs and muscle denervation is related to the increased capacity of the XII MNs to effect adaptive compensatory changes, rather than early pathophysiological degenerations, is an exciting new avenue to pursue (53, 54). Therapeutic assessments designed to preserve MNs would do well to include aerodigestive behaviours, particularly of swallows evoked using naturalistic methods [(see 55, 56)], as there is major brainstem sensory degeneration in SOD1 mice (26). Due to their highly relevant implications for both disease morbidity and mortality, efforts assessing the bulbar manifestations of ALS, which occur even in limb-onset patients, are of great importance.
Data availability statement
The original contributions presented in the study are included in the article/supplementary material, further inquiries can be directed to the corresponding author.
Ethics statement
The animal study was approved by the University of Queensland Animal Ethics Committee. The study was conducted in accordance with the local legislation and institutional requirements.
Author contributions
MF: Conceptualization, Data curation, Formal analysis, Funding acquisition, Investigation, Methodology, Supervision, Validation, Visualization, Writing – original draft, Writing – review & editing. JD-T: Conceptualization, Data curation, Formal analysis, Investigation, Writing – original draft, Writing – review & editing. MB: Conceptualization, Data curation, Formal analysis, Funding acquisition, Investigation, Project administration, Supervision, Writing – original draft, Writing – review & editing. PN: Conceptualization, Data curation, Formal analysis, Funding acquisition, Investigation, Project administration, Supervision, Writing – original draft, Writing – review & editing.
Funding
The author(s) declare that financial support was received for the research, authorship, and/or publication of this article. This study was in part supported by Motor Neuron Disease Research Australia (MNDRIA GIA 1842 and IG2326 to PN), National Health and Medical Research Council Australia (NHMRC-2029824, NHMRC 1188169 to PN, and a CJ Martin Fellowship to MF), Fight MND, and the NIH (R56-HL166204 to MF).
Acknowledgments
We thank M Shayegh, M White, and L Hammond for their assistance. We would also like to extend a special thanks to Dr. David Knight who provided invaluable confocal assistance and analysis to PN. We acknowledge the use of the SBMS imaging core facility for confocal microscopy.
Conflict of interest
The authors declare that the research was conducted in the absence of any commercial or financial relationships that could be construed as a potential conflict of interest.
Publisher’s note
All claims expressed in this article are solely those of the authors and do not necessarily represent those of their affiliated organizations, or those of the publisher, the editors and the reviewers. Any product that may be evaluated in this article, or claim that may be made by its manufacturer, is not guaranteed or endorsed by the publisher.
References
1. Hardiman, O, Van Den Berg, LH, and Kiernan, MC. Clinical diagnosis and management of amyotrophic lateral sclerosis. Nat Rev Neurol. (2011) 7:639–49. doi: 10.1038/nrneurol.2011.153
2. Kiernan, JA, and Hudson, AJ. Changes in sizes of cortical and lower motor neurons in amyotrophic lateral sclerosis. Brain. (1991) 114:843–53. doi: 10.1093/brain/114.2.843
3. Garand, KLF, Bhutada, AM, Hopkins-Rossabi, T, Mulekar, MS, and Carnaby, G. Pilot study of respiratory-swallow coordination in amyotrophic lateral sclerosis. J Speech Lang Hear Res. (2022) 65:2815–28. doi: 10.1044/2022_JSLHR-21-00619
4. Tabor-Gray, LC, Gallestagui, A, Vasilopoulos, T, and Plowman, EK. Characteristics of impaired voluntary cough function in individuals with amyotrophic lateral sclerosis. Amyotroph Lateral Scler Frontotemporal Degener. (2019) 20:37–42. doi: 10.1080/21678421.2018.1510011
5. Borges, ALF, Velasco, LC, Ramos, HVL, Imamura, R, Roldao, P, Petrillo, MVB, et al. Association between dysphagia and tongue strength in patients with amyotrophic lateral sclerosis. Braz J Otorhinolaryngol. (2022) 88:752–7. doi: 10.1016/j.bjorl.2020.10.015
6. Fogarty, MJ, and Sieck, GC. Tongue muscle contractile, fatigue, and Fiber type properties in rats. J Appl Physiol. (2021) 131:1043–55. doi: 10.1152/japplphysiol.00329.2021
7. Sawczuk, A, and Mosier, KM. Neural control of tongue movement with respect to respiration and swallowing. Crit Rev Oral Biol Med. (2001) 12:18–37. doi: 10.1177/10454411010120010101
8. Wealing, JC, Cholanian, M, Flanigan, EG, Levine, RB, and Fregosi, RF. Diverse physiological properties of hypoglossal Motoneurons innervating intrinsic and extrinsic tongue muscles. J Neurophysiol. (2019) 122:2054–60. doi: 10.1152/jn.00478.2019
9. Haenggeli, C, and Kato, AC. Differential vulnerability of cranial Motoneurons in mouse models with motor neuron degeneration. Neurosci Lett. (2002) 335:39–43. doi: 10.1016/S0304-3940(02)01140-0
10. Kashlan, ON, Kashlan, BN, Oh, SS, Mcginley, LM, Chen, KS, Kupfer, R, et al. Histological bulbar manifestations in the ALS rat. Neurodegener Dis. (2015) 15:121–6. doi: 10.1159/000377725
11. Ding, Q, Kesavan, K, Lee, KM, Wimberger, E, Robertson, T, Gill, M, et al. Impaired signaling for neuromuscular synaptic maintenance is a feature of motor neuron disease. Acta Neuropathol Commun. (2022) 10:61. doi: 10.1186/s40478-022-01360-5
12. Dukkipati, SS, Garrett, TL, and Elbasiouny, SM. The vulnerability of spinal Motoneurons and Soma size plasticity in a mouse model of amyotrophic lateral sclerosis. J Physiol. (2018) 596:1723–45. doi: 10.1113/JP275498
13. Kaplan, A, Spiller, KJ, Towne, C, Kanning, KC, Choe, GT, Geber, A, et al. Neuronal matrix Metalloproteinase-9 is a determinant of selective neurodegeneration. Neuron. (2014) 81:333–48. doi: 10.1016/j.neuron.2013.12.009
14. Pun, S, Santos, AF, Saxena, S, Xu, L, and Caroni, P. Selective vulnerability and pruning of phasic Motoneuron axons in Motoneuron disease alleviated by CNTF. Nat Neurosci. (2006) 9:408–19. doi: 10.1038/nn1653
15. Van Zundert, B, Peuscher, MH, Hynynen, M, Chen, A, Neve, RL, Brown, RH Jr, et al. Neonatal neuronal circuitry shows Hyperexcitable disturbance in a mouse model of the adult-onset neurodegenerative disease amyotrophic lateral sclerosis. J Neurosci. (2008) 28:10864–74. doi: 10.1523/JNEUROSCI.1340-08.2008
16. Fogarty, MJ, Mu, EWH, Lavidis, NA, Noakes, PG, and Bellingham, MC. Motor areas show altered dendritic structure in an amyotrophic lateral sclerosis mouse model. Front Neurosci. (2017) 11:609. doi: 10.3389/fnins.2017.00609
17. Fogarty, MJ, Mu, EWH, Lavidis, NA, Noakes, PG, and And Bellingham, MC. Size-dependent dendritic Maladaptations of hypoglossal motor neurons in SOD1(G93A) mice. Anat Rec. (2020) 303:1455–71. doi: 10.1002/ar.24255
18. Ngo, ST, Baumann, F, Ridall, PG, Pettitt, AN, Henderson, RD, Bellingham, MC, et al. The relationship between Bayesian motor unit number estimation And histological measurements of motor neurons in wild-type And Sod1(G93A) mice. Clin Neurophysiol. (2012) 123:2080–91. doi: 10.1016/j.clinph.2012.01.028
19. Lee, JD, Kamaruzaman, NA, Fung, JN, Taylor, SM, Turner, BJ, Atkin, JD, et al. Dysregulation of the complement Cascade in the hSOD1G93A transgenic mouse model of amyotrophic lateral sclerosis. J Neuroinflammation. (2013) 10:119. doi: 10.1186/1742-2094-10-119
20. Fogarty, MJ. Loss of larger hypoglossal motor neurons in aged Fischer 344 rats. Respir Physiol Neurobiol. (2023) 314:104092. doi: 10.1016/j.resp.2023.104092
21. Fogarty, MJ, Smallcombe, KL, Yanagawa, Y, Obata, K, Bellingham, MC, and Noakes, PG. Genetic deficiency of GABA differentially regulates respiratory and non-respiratory motor neuron development. Plos One. (2013) 8:E56257. doi: 10.1371/journal.pone.0056257
22. Fogarty, MJ, Yanagawa, Y, Obata, K, Bellingham, MC, and Noakes, PG. Genetic absence of the vesicular inhibitory amino acid transporter differentially regulates respiratory and locomotor motor neuron development. Brain Struct Funct. (2015) 220:525–40. doi: 10.1007/s00429-013-0673-9
23. Paxinos, G., And Franklin, K.B.J. (2001). The mouse brain in stereotaxic coordinates. Academic Press. San Diego, CA, USA
24. Johnson, A.M., And Connor, N.P. (2011). Effects of electrical stimulation on neuromuscular junction morphology in the aging rat tongue. Muscle Nerve 43, 203–211, doi: 10.1002/mus.21819
25. Gautam, M, Noakes, PG, Moscoso, L, Rupp, F, Scheller, RH, Merlie, JP, et al. Defective neuromuscular synaptogenesis in Agrin-deficient mutant mice. Cell. (1996) 85:525–35. doi: 10.1016/S0092-8674(00)81253-2
26. Lever, TE, Simon, E, Cox, KT, Capra, NF, O'brien, KF, Hough, MS, et al. A mouse model of pharyngeal dysphagia in amyotrophic lateral sclerosis. Dysphagia. (2010) 25:112–26. doi: 10.1007/s00455-009-9232-1
27. Smittkamp, SE, Spalding, HN, Brown, JW, Gupte, AA, Chen, J, Nishimune, H, et al. Measures of bulbar and spinal motor function, muscle innervation, and mitochondrial function in ALS rats. Behav Brain Res. (2010) 211:48–57. doi: 10.1016/j.bbr.2010.03.007
28. Smittkamp, S.E., and Brown, J.W., And Stanford, J.A. (2008). Time-course and characterization of Orolingual motor deficits in B6SJL-Tg(SOD1-G93A)1Gur/J mice. Neuroscience 151, 613–621, doi: 10.1016/j.neuroscience.2007.10.017
29. Osman, KL, Kohlberg, S, Mok, A, Brooks, R, Lind, LA, Mccormack, K, et al. Optimizing the translational value of mouse models of ALS for dysphagia therapeutic discovery. Dysphagia. (2020) 35:343–59. doi: 10.1007/s00455-019-10034-9
30. Fogarty, MJ. Dendritic morphology of motor neurons and interneurons within the compact, Semicompact, and loose formations of the rat nucleus Ambiguus. Front Cell Neurosci. (2024) 18:1409974. doi: 10.3389/fncel.2024.1409974
31. Ozdinler, PH, Benn, S, Yamamoto, TH, Guzel, M, Brown, RH Jr, and Macklis, JD. Corticospinal motor neurons and related subcerebral projection neurons undergo early and specific neurodegeneration in hSOD1g(9)(3)A transgenic ALS mice. J Neurosci. (2011) 31:4166–77. doi: 10.1523/JNEUROSCI.4184-10.2011
32. Fogarty, MJ, Noakes, PG, and Bellingham, MC. Motor cortex layer V pyramidal neurons exhibit dendritic regression, spine loss, and increased synaptic excitation in the Presymptomatic hSOD1(G93A) mouse model of amyotrophic lateral sclerosis. J Neurosci. (2015) 35:643–7. doi: 10.1523/JNEUROSCI.3483-14.2015
33. Genc, B, Jara, JH, Lagrimas, AK, Pytel, P, Roos, RP, Mesulam, MM, et al. Apical dendrite degeneration, a novel cellular pathology for Betz cells in ALS. Sci Rep. (2017) 7:41765. doi: 10.1038/srep41765
34. Seven, YB, Nichols, NL, Kelly, MN, Hobson, OR, Satriotomo, I, and Mitchell, GS. Compensatory plasticity in diaphragm and intercostal muscle utilization in a rat model of ALS. Exp Neurol. (2018) 299:148–56. doi: 10.1016/j.expneurol.2017.10.015
35. Smittkamp, SE, Spalding, HN, Brown, JW, Yeh, HW, and Stanford, JA. Relationships between tongue motility, grip force, and survival in SOD1-G93A rats. Physiol Behav. (2014) 125:17–20. doi: 10.1016/j.physbeh.2013.11.010
36. Dobrowolny, G, Martini, M, Scicchitano, BM, Romanello, V, Boncompagni, S, Nicoletti, C, et al. Muscle expression of Sod1(G93A) triggers the dismantlement of neuromuscular junction via PKC-Theta. Antioxid Redox Signal. (2018) 28:1105–19. doi: 10.1089/ars.2017.7054
37. Rocha, MC, Pousinha, PA, Correia, AM, Sebastiao, AM, and Ribeiro, JA. Early changes of neuromuscular transmission in the SOD1(G93A) mice model of ALS start long before motor symptoms onset. PLoS One. (2013) 8:E73846. doi: 10.1371/journal.pone.0073846
38. Abe, S, Maejima, M, Watanabe, H, Shibahara, T, Agematsu, H, Doi, T, et al. Muscle-Fiber characteristics in adult mouse-tongue muscles. Anat Sci Int. (2002) 77:145–8. doi: 10.1046/j.0022-7722.2002.00019.x
39. Mueller, M, Thompson, R, Osman, KL, Andel, E, Dejonge, CA, Kington, S, et al. Impact of limb phenotype on tongue denervation atrophy, dysphagia penetrance, and survival time in a mouse model of ALS. Dysphagia. (2022) 37:1777–95. doi: 10.1007/s00455-022-10442-4
40. Glass, TJ, Figueroa, JE, Russell, JA, Krekeler, BN, and Connor, NP. Progressive protrusive tongue exercise does not alter aging effects in retrusive tongue muscles. Front Physiol. (2021) 12:740876. doi: 10.3389/fphys.2021.740876
41. Kletzien, H., Hare, A.J., and Leverson, G., And Connor, N.P. (2018). Age-related effect of cell death on Fiber morphology and number in tongue muscle. Muscle Nerve 57, E29–E37, doi: 10.1002/mus.25671
42. Nagai, H., Russell, J.A., and Jackson, M.A., And Connor, N.P. (2008). Effect of aging on tongue protrusion forces in rats. Dysphagia 23, 116–121, doi: 10.1007/s00455-007-9103-6
43. Sieck, G.C., Hernandez-Vizcarrondo, G.A., and Brown, A.D., And Fogarty, M.J. (2023). Sarcopenia of the longitudinal tongue muscles in rats. Respir Physiol Neurobiol 319:104180. doi: 10.1016/j.resp.2023.104180
44. Crow, H.C., And Ship, J.A. (1996). Tongue strength and endurance in different aged individuals. J Gerontol A Biol Sci Med Sci 51, M247–M250, doi: 10.1093/gerona/51A.5.M247
45. Youmans, SR, Youmans, GL, and Stierwalt, JA. Differences in tongue strength across age and gender: is there a diminished strength reserve? Dysphagia. (2009) 24:57–65. doi: 10.1007/s00455-008-9171-2
46. Alexander, GM, Erwin, KL, Byers, N, Deitch, JS, Augelli, BJ, Blankenhorn, EP, et al. Effect of transgene copy number on survival in the G93A SOD1 transgenic mouse model of ALS. Brain Res Mol Brain Res. (2004) 130:7–15. doi: 10.1016/j.molbrainres.2004.07.002
47. Fogarty, MJ. Driven to decay: excitability and synaptic abnormalities in amyotrophic lateral sclerosis. Brain Res Bull. (2018) 140:318–33. doi: 10.1016/j.brainresbull.2018.05.023
48. Sturrock, RR. Stability of motor neuron and interneuron number in the hypoglossal nucleus of the ageing mouse brain. Anat Anz. (1991) 173:113–6.
49. Fogarty, M.J., Dasgupta, D., and Khurram, O.U., And Sieck, G.C. (2023). Chemogenetic inhibition of TrkB Signalling reduces phrenic motor neuron survival and size. Mol Cell Neurosci, 125:103847, doi: 10.1016/j.mcn.2023.103847
50. Lind, L.A., Murphy, E.R., and Lever, T.E., And Nichols, N.L. (2018). Hypoglossal motor neuron death via Intralingual CTB-Saporin (CTB-SAP) injections mimic aspects of amyotrophic lateral sclerosis (ALS) related to dysphagia. Neuroscience 390, 303–316, doi: 10.1016/j.neuroscience.2018.08.026
51. Nichols, N.L., Vinit, S., and Bauernschmidt, L., And Mitchell, G.S. (2015). Respiratory function after selective respiratory motor neuron death from intrapleural CTB-Saporin injections. Exp Neurol 267, 18–29, doi: 10.1016/j.expneurol.2014.11.011
52. Lever, TE, Gorsek, A, Cox, KT, O’Brien, KF, Capra, NF, Hough, MS, et al. An animal model of Oral dysphagia in amyotrophic lateral sclerosis. Dysphagia. (2009) 24:180–95. doi: 10.1007/s00455-008-9190-z
53. Filipchuk, A, Pambo-Pambo, A, Gaudel, F, Liabeuf, S, Brocard, C, Patrick Gueritaud, J, et al. Early hypoexcitability in a subgroup of spinal motoneurons in superoxide dismutase 1 transgenic mice, a model of amyotrophic lateral sclerosis. Neuroscience. (2021) 463:337–53. doi: 10.1016/j.neuroscience.2021.01.039
54. Fogarty, MJ. Neuronal Hypoexcitability and dendritic overbranching - the case for failed compensatory mechanisms in ALS Aetiology. Neuroscience. (2021) 465:231–2. doi: 10.1016/j.neuroscience.2021.02.034
55. Bolser, DC, Shen, TY, Musselwhite, MN, Rose, MJ, Hayes, JA, and Pitts, T. Evidence for peripheral and central actions of codeine to dysregulate swallowing in the anesthetized cat. Front Neurol. (2024) 15:1356603. doi: 10.3389/fneur.2024.1356603
Keywords: amyotrophic lateral sclerosis, motor neurons, neuromuscular junctions, hypoglossal, tongue
Citation: Fogarty MJ, Drieberg-Thompson JR, Bellingham MC and Noakes PG (2024) Timeline of hypoglossal motor neuron death and intrinsic tongue muscle denervation in high-copy number SOD1G93A mice. Front. Neurol. 15:1422943. doi: 10.3389/fneur.2024.1422943
Edited by:
Teresa Pitts, University of Missouri, United StatesReviewed by:
Kimberly Iceman, University of Missouri, United StatesDonald C. Bolser, University of Florida, United States
Marlusa Karlen-Amarante, University of Missouri, United States
Copyright © 2024 Fogarty, Drieberg-Thompson, Bellingham and Noakes. This is an open-access article distributed under the terms of the Creative Commons Attribution License (CC BY). The use, distribution or reproduction in other forums is permitted, provided the original author(s) and the copyright owner(s) are credited and that the original publication in this journal is cited, in accordance with accepted academic practice. No use, distribution or reproduction is permitted which does not comply with these terms.
*Correspondence: Matthew J. Fogarty, Rm9nYXJ0eS5tYXR0aGV3QG1heW8uZWR1
†These authors share senior authorship