- 1Department of Anesthesiology, Guangxi Medical University Cancer Hospital, Nanning, China
- 2Guangxi Clinical Research Center for Anesthesiology (GKAD22035214), Nanning, China
- 3Guangxi Engineering Research Center for Tissue and Organ Injury and Repair Medicine, Nanning, China
- 4Guangxi Health Commission Key Laboratory of Basic Science and Prevention of Perioperative Organ Dysfunction, Nanning, China
- 5Department of Anesthesiology, First Affiliated Hospital of Guangxi Medical University, Nanning, China
Background: Dexmedetomidine (DEX) has demonstrated potential as an effective agent for enhancing early postoperative cognitive function. However, there is ongoing debate regarding its optimal dosage and impact on early postoperative inflammatory response. This study aimed to assess and prioritize the effects of varying doses of DEX on early postoperative cognitive function and inflammatory response, in order to identify the most effective intervention dosage.
Methods: Randomised controlled trials (RCTs) and retrospective cohort studies (RCS) from PubMed, Embase, and Cochrane Library up to January 28, 2024, were included. The Mini-Mental State Examination (MMSE) was utilized to assess the impact of varying doses of DEX on cognitive function during the early postoperative period as the primary outcome, peripheral blood levels of IL-6 and TNF-α were considered as secondary outcomes. Meta-analysis and Bayesian Network Meta-Analysis (NMA) were conducted using R. Funnel plots were generated using Stata 15.0.
Results: A total of 29 studies involving 2,807 patients and 25 different doses of DEX were included. DEX was given at a loading dose of 0.3–1.0 μg/kg followed by a maintenance dose of 0.1–0.5 μg/kg/h, or at a uniform intraoperative dose of 0.4–0.7 μg/kg/h. Network meta-analysis revealed most doses of DEX were significantly more effective than normal saline (NS) in improving postoperative MMSE scores (on days 1, 3, and 7) and lowering IL-6 and TNF-α levels. Probability results showed that a 1 μg/kg loading dose followed by a 0.6 μg/kg/h maintenance dose was the best dosing regimen for improving MMSE scores on postoperative days 1 (97.3%), 3 (100%), and 7 (99.9%), as well as for reducing postoperative blood IL-6 levels (1.3%). On the other hand, 0.3 μg/kg followed by 0.2 μg/kg/h was the optimal dosing regimen for reducing postoperative blood TNF-α levels (6.6%).
Conclusion: Compared with NS, intraoperative intravenous DEX improved early postoperative cognitive function and postoperative inflammatory response in patients undergoing elective surgery. In particular, a 1 μg/kg loading dose and a 0.6 μg/kg/h maintenance dose resulted in the best improvement in postoperative MMSE scores and blood IL-6 levels, while a 0.3 μg/kg loading dose followed by a 0.2 μg/kg/h maintenance dose is the optimal regimen for lowering postoperative blood TNF-α levels.
Systematic review registration: https://www.crd.york.ac.uk/PROSPERO/display_record.php?RecordID=433932, identifier CRD42023433932.
1 Introduction
Abnormalities in postoperative cognitive function can manifest in the anaesthesia recovery room, during hospitalization, or up to 1 year after surgery. These abnormalities may include memory loss, poor concentration, and delayed thinking. Notably, postoperative cognitive abnormalities can result in long-term cognitive impairment, extended hospital stays, increased mortality, and negative socioeconomic consequences. The efficacy and safety of dexmedetomidine (DEX) in treating postoperative cognitive abnormalities and inflammatory response have been supported by numerous randomised, double-blind, placebo-controlled trials (1, 2). DEX has been shown to impact cognitive function and inflammatory outcomes through various mechanisms. Zhao et al. (3) found that DEX inhibited PSD95-NMDA receptor interactions in mice with traumatic brain injury (TBI), leading to improved functional recovery after TBI. Chen et al. (4) reported that DEX enhanced mitochondrial autophagy via PINK1, attenuated hippocampal focal neuroapoptosis, and improved postoperative cognitive dysfunction. Research on epigenetic mechanisms of postoperative cognitive impairment has also indicated that DEX is linked to a lower risk of cognitive decline compared to other substances (5). Kho et al. (6) examined the effects of DEX on autophagic flux, microRNA, and cholinergic anti-inflammatory pathways in LPS-treated rats. The authors revealed that DEX prevented impairment of autophagic flux and reduced apoptosis-associated proteins in the spleen. This suggests that DEX may modulate inflammation through multiple pathways, potentially contributing to its cognitive benefits (6). Furthermore, DEX has been found to play an important role in improving neuroinflammation-induced cognitive dysfunction. Recent studies indicate that DEX ameliorates postoperative cognitive dysfunction (POCD) by activating the c-Jun N-terminal kinase (JNK)/p-38 signaling pathway through CEBPB as a pharmacological target to inhibit M1-mediated inflammation in CNS microglia (7). In a retrospective propensity score study involving patients undergoing lobectomy, DEX prevented cognitive dysfunction and delirium by attenuating neuroinflammation (8). Additionally, a meta-analysis of 17 randomised-controlled trials (RCTs) with 1,619 patients demonstrated that DEX not only ameliorates perioperative immune dysfunction but also reduces POCD and associated neuroinflammation. These findings demonstrate that DEX can enhance postoperative recovery and clinical outcomes in patients undergoing gastrointestinal cancer surgery (9). Clinical guidelines recommend intraoperative DEX infusion for high-risk patients or continuous postoperative infusion for ICU patients to reduce postoperative cognitive abnormalities. However, high doses of DEX as an anaesthetic adjuvant to general intravenous anaesthesia can lower blood pressure and heart rate due to its sympathetic effects. Recovery from these effects may be slow and persistent even after general anaesthesia. Furthermore, there is limited research directly comparing the effects of different DEX doses on postoperative cognitive function and inflammation.
We therefore performed network meta-analyses (NMA) of different doses of DEX. NMA is an extension of traditional meta-analysis, a technique that combines direct and indirect evidence in a network of trials comparing multiple treatments or different doses. In the absence of head-to-head trials, these indirect comparisons provided insights into the optimal dose of DEX to mitigate postoperative cognitive abnormalities and early inflammatory responses.
2 Methods
The analyses were conducted in accordance with a pre-specified protocol registered in PROSPERO (CRD42023433932), and the results were reported following the Preferred Reporting Items for Systematic Evaluation and Meta-Analysis (PRISMA) extension statement for NMA (10).
2.1 Search strategy and eligibility criteria
Relevant studies were systematically search in Embase, Pubmed, and the Cochrane Library from inception to January 28, 2024 using free-text keywords and MeSH related to DEX and postoperative cognitive disorder without language restrictions (see Appendix A). The retrieved articles were identified and screened by two independent reviewers (RMY and XLX). The relevant procedures for systematic appraisal were in accordance with the PRISMA guidelines. The articles were further selected to assess the expression of postoperative inflammatory markers. The inclusion criteria for this study were: (1) Prospective or retrospective studies of patients undergoing elective surgery with intraoperative intravenous DEX; (2) Patients received general anesthesia, intrathecal anesthesia, nerve block, or other anesthesia modalities; (3) Control group received intraoperative intravenous saline equivalents; (4) Outcomes measured were postoperative MMSE scores and/or postoperative blood levels of IL-6 and/or TNF-α. Studies with the following characteristics were excluded: (1) Lacked a detailed description of the experimental protocol; (2) Abstracts or conference proceedings; (3) Unavailable or untranslated relevant data or unreasonable or seriously flawed study designs.
2.2 Data extraction
Data were extracted independently by two authors (CFH, XLX) and entered into a standardized Excel spreadsheet (Microsoft, Redmond, WA, United States). In cases where trials were published in languages other than English, Google Translate was utilized. The extracted trial characteristics included the number of patients in each study group, age, surgical procedure, anesthesia, DEX dose, usage, and duration. The primary endpoints were postoperative MMSE scores on postoperative days 1, 3, and 7, and the secondary endpoints were postoperative blood IL-6 and TNF-α levels. The results were extracted as means and standard deviations. Data presented in graphical form were converted to numerical format using Plot Digitizer (version 2.1, Free Software Foundation, Boston, MA, United States). The median was assumed to be equal to the mean, and the standard deviation was calculated based on the interquartile spacing and extreme deviation (1.35 and 4, respectively) (11). In cases where information was unclear or incomplete, clarification was sought by sending an email to the authors of the included trials.
The quality of all included studies was independently assessed by the investigators (RMY and HJD) according to the Cochrane risk-of-bias tool for randomized trials (RoB2) and Newcastle-Ottawa Scale (NOS). The quality of randomized-controlled trials (RCTs) was assessed using the RoB2 tool in five domains, namely randomization process, deviation from intended intervention, missing outcome data, measurement of outcomes, and selection of reported outcomes. Each domain was scored low risk, some level of concern, or high risk. Any disagreements were resolved through discussion. The NOS scale was used to rate the quality of retrospective cohort studies (RCS), with scores ranging from 1 to 3 indicating low quality, 4 to 6 indicating moderate quality, and 7 to 9 indicating high quality.
2.3 Statistical analysis
Meta-analysis and Bayesian NMA were performed using the ‘gemtc’ and ‘rjags’ packages of R v4.3.1 (12). Network graphs were generated for each outcome displaying different DEX doses as nodes and direct comparisons as connecting lines. Indirect comparisons were derived from direct comparison estimates of a common comparison dose, and the results of both direct and indirect comparisons were summarized in a network leaderboard. MMSE scores (postoperative days 1, 3, 7) and postoperative blood IL-6 and TNF-α levels are expressed as ratios (OR) and 95% confidence intervals (CI) (13). The advantages and disadvantages of different DEX doses were ranked according to the surface under the cumulative ranking curve (SUCRA). The intervention dose is absolutely effective if SUCRA is ≥0 and ≤ 1, and absolutely ineffective if SUCRA is 0. For IL-6 and TNF-α, a larger area under the curve (AUC) indicates a more detrimental effect of postoperative inflammatory markers on recovery. Heterogeneity among the results of the included studies was determined by the χ2 test (significance level α = 0.1) and quantitated by I2. The fixed effects model was used if there was no heterogeneity between studies (I2 ≤ 50%); otherwise, a random effects model was used (I2 > 50%). Significant clinical heterogeneity was addressed through methods such as subgroup analysis, sensitivity analysis, or descriptive analysis only.
The robustness of results can be influenced by the size of the study and the likelihood of publishing negative results. In addition, we conducted one-way sensitivity analyses to evaluate the influence of the included studies on the combined results, considering the significantly heterogeneous outcomes. Funnel plots were created using Stata version 15.0 (Stata Corp, College Station, TX, United Sates), and Egger’s test were conducted to visually assess publication bias for outcomes that included 10 or more studies.
3 Results
3.1 Characteristics of included studies
A total of 1,484 relevant articles were collected from the three databases. After removing duplications, the title of the remaining 667 articles was screened by two independent reviewers and 495 studies were excluded due to inappropriate study type, including animal studies, case reports, reviews, protocols, and studies unrelated to postoperative cognition. Upon further examination of the abstracts, 126 studies were excluded due to failure to meet the inclusion criteria, including 61 with unrelated study outcomes, 33 with unrelated study interventions, and 32 with unrelated study controls. Following a thorough review of the full text, 17 studies were eliminated, comprising 9 articles with unextractable data, 6 articles lacking raw data, and 2 retracted articles. Ultimately, 29 studies involving 2,807 patients were included. The included studies had comparable baseline characteristics, such as sex, age, and sample size. The screening process is depicted in Figure 1.
Of these 29 studies, 23 RCTs and 3 RCSs reported postoperative cognitive function outcomes (MMSE), 10 RCTs and 1 RCS reported postoperative blood IL-6 expression, and 8 RCTs and 1 RCS reported postoperative blood TNF-α level. The mean age of the participants ranged from 40 to 75 years (Table 1). Most trials were placebo-controlled (89%) and had two treatment groups (72.4%). The endpoints assessed were postoperative cognitive function (assessed by MMSE) and postoperative inflammatory markers (IL-6 and TNF-α). General anesthesia was used in 25 studies, nerve block in 1 study, epidural block in 2 studies, and lumbar nerve block in 1 study. MMSE was assessed at 3 time points, namely postoperative days 1 (n = 20), 3 (n = 13), and 7 (n = 10), with postoperative day 1 being the predominant time point. Inflammatory markers (IL-6 and TNF-α) were assessed at 1 day or 1 h post-surgery, most commonly on postoperative day 1. A total of 24 DEX doses were identified. Four studies (16, 17, 23, 34) used a 1 μg/kg loading dose (LD) followed by a 0.4 μg/kg/h maintenance dose (MD); two studies (15, 42) used 1 μg/kg LD then 0.3 μg/kg/h MD; two studies (18, 29) used 0.5 μg/kg LD then 0.2 μg/kg/h MD; two studies (25, 26) used 0.3 μg/kg LD then 0.2 μg/kg/h MD; two studies (25, 26) used 0.3 μg/kg LD then 0.5 μg/kg/h MD; two studies (25, 26) used 0.3 μg/kg LD then 0.8 μg/kg/h MD; two studies (28, 31) used an intraoperative dose (IOD) of 0.5 μg/kg/h; two studies (30, 33) used 0.4 μg/kg/h IOD; two studies (36, 40) used 0.6 μg/kg/h IOD; one study (14) used 0.7 μg/mL IOD; one study (19) used 0.6 μg/kg LD then 0.2 μg/kg/h MD; one study (20) used 1 μg/kg LD then 0.3–0.5 μg/kg/h MD; one study (21) used 0.3 μg/kg LD then 0.3 μg/kg/h MD; one study (22) used 1 μg/kg LD then 0.2 μg/kg/h MD; one study (24) used 0.5 μg/kg LD then 0.1 μg/kg/h MD; one study (27) used 0.5 μg/kg LD then 0.6 μg/kg/h MD; one study (41) used 0.8 μg/kg LD then 0.5 μg/kg/h MD; one study (32) used 1 μg/kg LD then 0.5 μg/kg/h MD; one study (35) used 0.6 μg/kg LD only; one study (37) used 0.5 μg/kg for 30 min before the end of surgery; one study (38) used 0.5 μg/kg LD then 0.4 μg/kg/h MD; one study (39) used 0.8 μg/kg LD then 0.2 μg/kg/h MD; one study (40) used 0.3 μg/kg/h IOD; and one study (42) used 1 μg/kg LD then 0.6 μg/kg/h MD.
3.2 Results of risk of bias, heterogeneity and sensitivity analysis
Of the 26 RCTs, 17 (65.3%) utilized a method to generate a randomized sequence of appropriate methods, 3 (11.5%) deviated from the intervention, and 3 (11.5%) experienced loss of data. Additionally, 19 (26.9%) RCTs had outcome measurement bias and 2 (7.6%) reported selection bias in the outcome. Quality assessment of the included studies revealed that 38.5% were of low risk, 23.1% were of unknown risk of bias, and 38.5% were of high risk (Figure 2). The overall quality of the included studies ranged from low to moderate. In addition, quality assessment of the 3 RCSs using the NOS scale showed that they were all high-quality (Table 2).
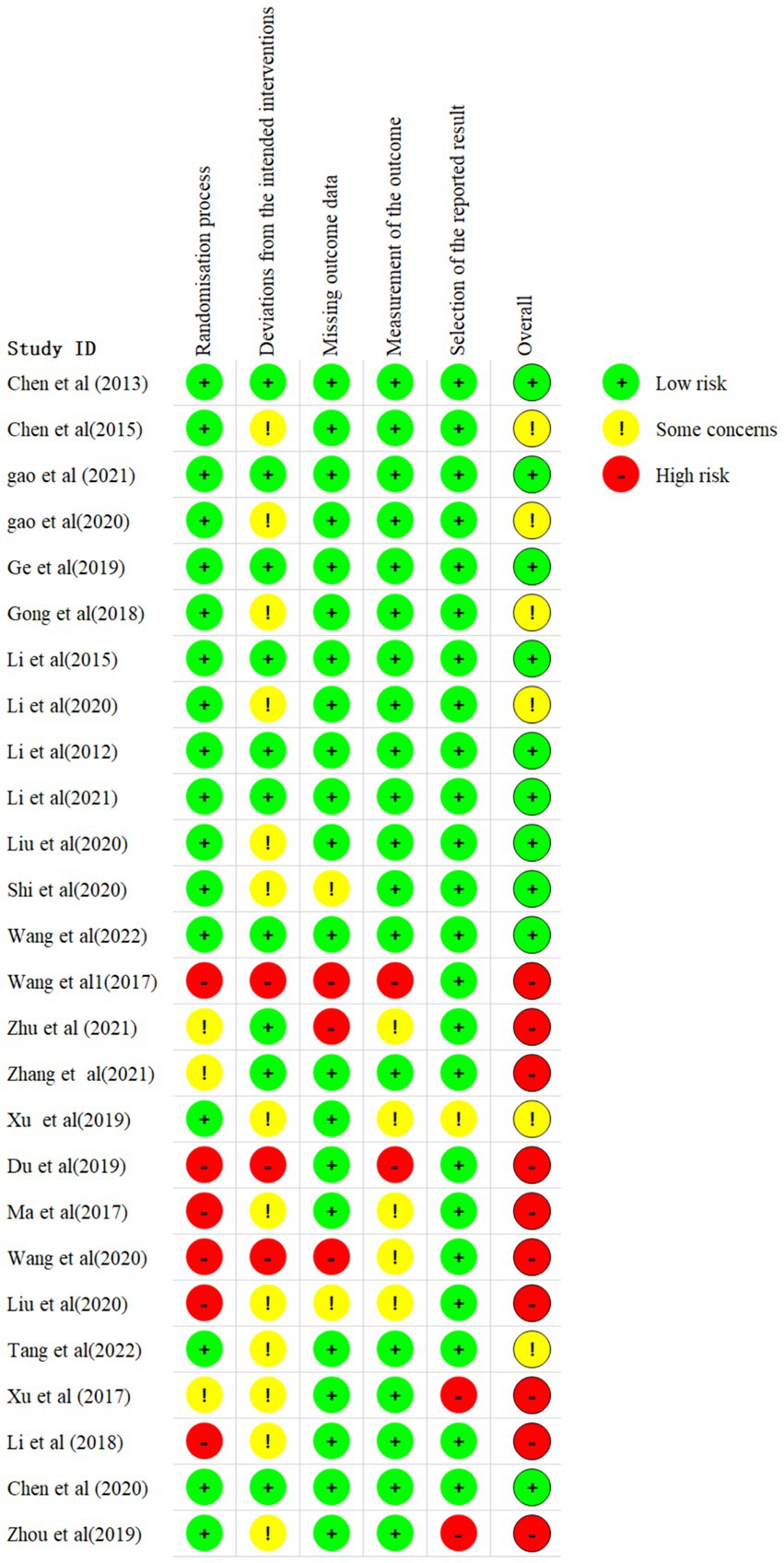
Figure 2. Assessments of every risk of bias item for eligible studies. The green, yellow, and red circle indicate “low, ““unclear,” and “high” risk of bias, respectively.
The included studies were heterogeneous (I2>50%), except for the MMSE scores on postoperative days 3 and 7 (Supplementary Figures 1a–e). The heterogeneity test indicated an I2 value greater than 50%, with no significant variations observed in study design, region, participant characteristics, interventions, or methodology. Subsequent sensitivity analyses identified the Li et al. (25) and Li et al. (26) studies as the primary sources of heterogeneity (Supplementary Figure 1). Upon re-evaluation, it was discerned that both studies exhibited bias due to small sample sizes in each group. After excluding these studies, the I2 value dropped below 50%, leading to enhanced consistency and stability in the results of the net meta-analysis.
The pooled results of our network meta-analyses may have been influenced by several confounding factors, including the type of surgery, age, and method of assessment. To ensure the reliability of our findings, we performed a series of sensitivity analyses. The results of these analyses, presented in Supplementary Figures 2a–e, did not reveal any significant changes in the pooled results for all comparisons.
3.3 Primary outcome: MMSE scores on postoperative days 1, 3 and 7
A total of 20 studies (14, 15, 17, 21–28, 30–32, 34–38, 42) involving 2041 patients assessed MMSE scores on postoperative day 1. There was heterogeneity among the studies (I2 = 84%) and a random effects model was used for meta-analysis. A total of 18 intervention doses were involved in the NMA, and the evidence relationships are shown in Figure 3A. Compared with the placebo, four DEX dosing regimens (1 μg/kg LD followed by 0.3 μg/kg/h MD, 0.3 μg/kg LD followed by 0.5 μg/kg/h MD, 0.3 μg/kg LD followed by 0.8 μg/kg/h MD, and 1 μg/kg LD followed by 0.6 μg/kg/h MD) significantly improved postoperative MMSE scores (SMD 5.9, 95%CI 2.3 to 9.6; SMD 4.9, 95%CI 1.2 to 8.6; SMD 5, 95%CI 1.3 to 8.7; SMD 9.3, 95%CI 4.4 to 14, respectively) (Figure 3B). When comparing the effects of different DEX doses on improving MMSE scores, 1 μg/kg LD then 0.6 μg/kg/h MD was significantly better than 0.7 μg/mL IOD, 1 μg/kg LD then 0.4 μg/kg/h MD, 0.5 μg/kg LD then 0.1 μg/kg/h MD, 0.5 μg/kg/h IOD, 0.4 μg/kg/h IOD, 1 μg/kg LD then 0.5 μg/kg/h MD and 0.6 μg/kg/h IOD; 1 μg/kg LD then 0.3 μg/kg/h MD was significantly better than 1 μg/kg LD then 0.4 μg/kg/h MD and 0.5 μg/kg/h IOD; 1 μg/kg LD then 0.4 μg/kg/h MD was significantly worse than 0.3 μg/kg LD then 0.5 μg/kg/h MD and 0.3 μg/kg LD then 0.8 μg/kg/h MD (Supplementary Table 1a). The probability of improvement in MMSE scores on postoperative day 1 by different DEX doses versus NS was analyzed by determining the SUCRA (Figure 3C). Refer to Figure 3D for abbreviations and descriptions of the 18 DEX intervention doses and the control group. Our data showed that 1 μg/kg LD then 0.6 μg/kg/h MD resulted in the best MMSE score improvement (97.3%), followed by 1 μg/kg LD then 0.6 μg/kg/h MD (85.1%). An IOD of 0.5 μg/kg/h DEX (13.1%) resulted in the worst MMSE score improvement.
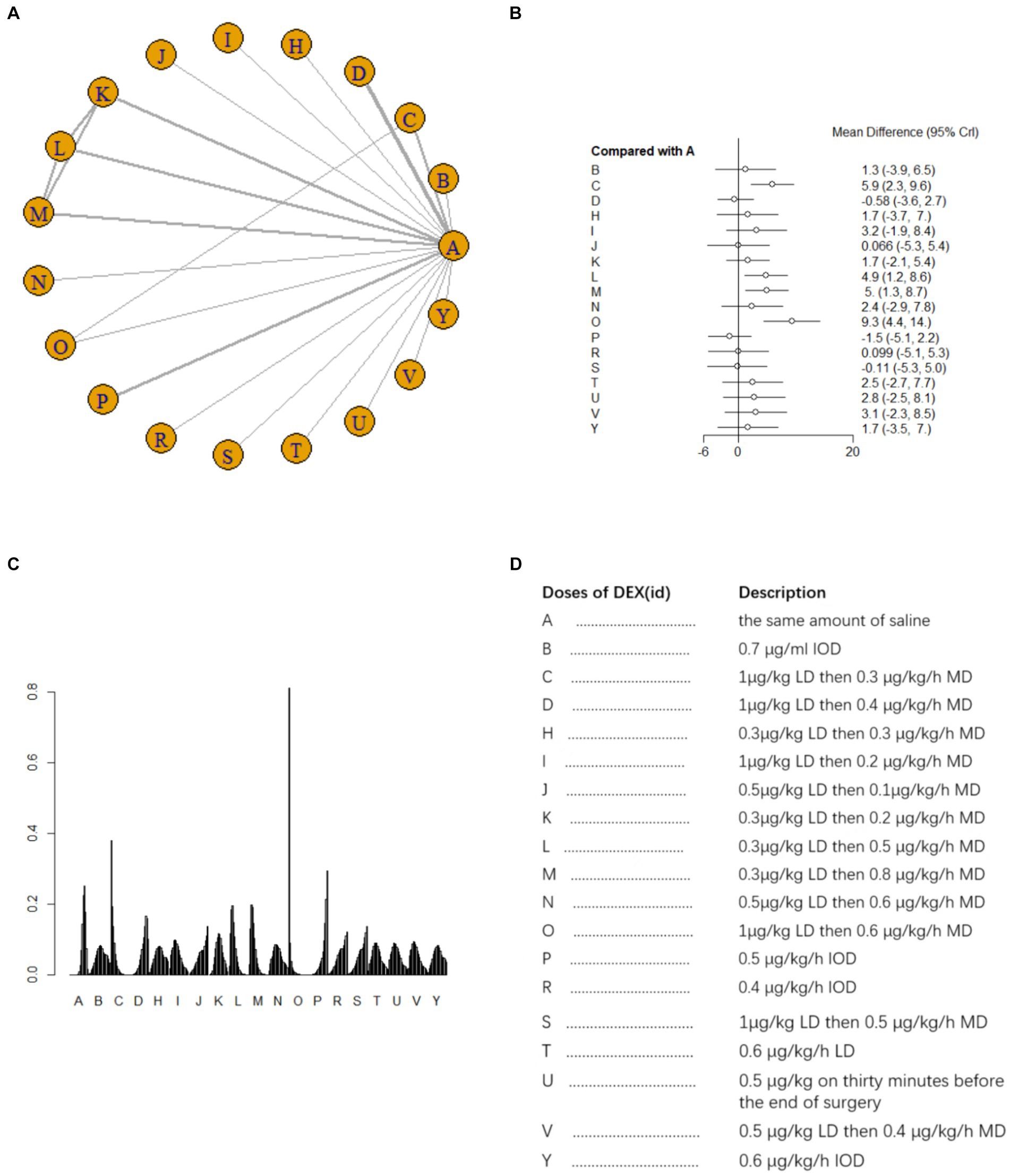
Figure 3. Network estimates of total MMSE score on day 1 after surgery. (A) Network plot showing direct comparisons between nodes. (B) Forest plot of different DEX doses compared with NS in NMA. (C) Estimated probability (%) of each dose level. (D) Description of individual DEX doses in this analysis. LD, Load dose; MD, Maintenance dose; IOD, Intraoperative dose.
Thirteen studies (15, 18, 20, 22, 25, 26, 28–30, 32, 37, 39, 42) involving 1,279 patients assessed MMSE scores on postoperative day 3. A fixed effects model was utilized for meta-analysis due to the presence of low heterogeneity (I2 = 23%). A total of 13 intervention doses were involved in the NMA, and the evidence relationships are shown in Figure 4A. All 11 doses of dexmedetomidine significantly improved postoperative MMSE scores compared with placebo, except for two doses (0.4μg/kg/h IOD and 0.5 μg/kg/h IOD) (Figure 4B). In the NMA, there were significant differences among different doses of DEX (Supplementary Table 1b). The cumulative ranking of different DEX doses versus NS for improvement in MMSE score on postoperative day 3 is shown in Figure 4C. Figure 4D for abbreviations and descriptions of the 13 DEX intervention doses and the control group. SUCRA analysis showed that the improvement in MMSE score was the greatest with 1 μg/kg LD then 0.4 μg/kg/h MD (100%), followed by 1 μg/kg LD then 0.3 μg/kg/h, and the least with 0.5 μg/kg/h IOD (7.4%) (Figure 4C).
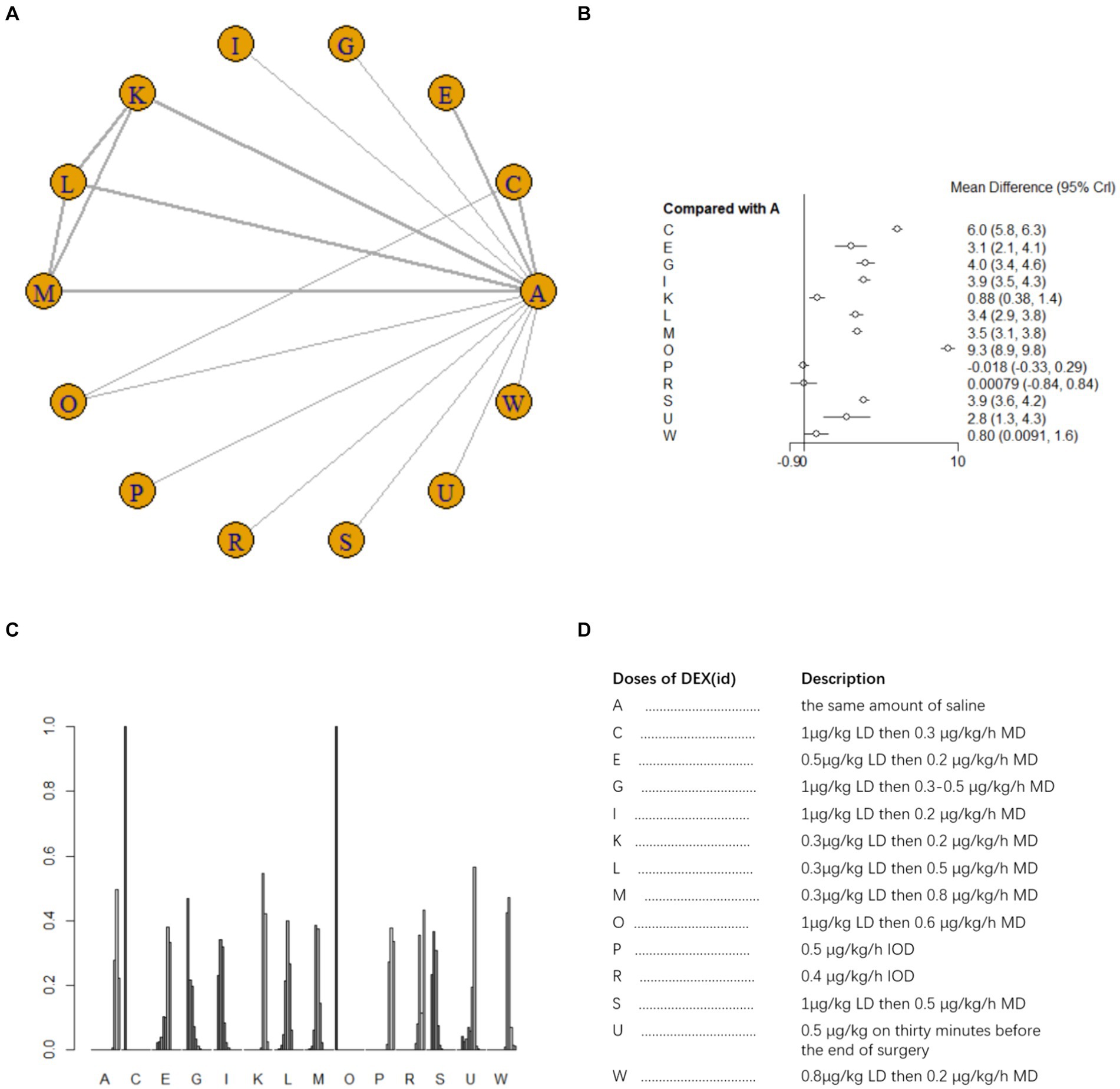
Figure 4. Network estimates of total MMSE score on day 3 after surgery. (A) Network plot showing direct comparisons between nodes. (B) Forest plot of different DEX doses compared with NS in NMA. (C) Estimated probability (%) of each dose level. (D) Description of individual DEX doses in this analysis. LD, Load dose; MD, Maintenance dose; IOD, Intraoperative dose.
Ten studies (16, 19, 20, 22, 25–28, 32, 42) involving 950 patients evaluated MMSE scores on postoperative day 7. A fixed effects model was adopted for meta-analysis owing to the small heterogeneity among studies (I2 = 23%). A total of 12 intervention doses were involved in the NMA, and the evidence relationships are shown in Figure 5A. Compared with the placebo, ten DEX dosing regimens (1 μg/kg LD then 0.3 μg/h MD, 0.6 μg/kg LD then 0.2 μg/kg/h MD, 1 μg/kg LD then 0.3–0.5 μg/kg/h MD, 1 μg/kg LD then 0.2 μg/kg/h MD, 0.3 μg/kg LD then 0.2 μg/kg/h MD, 0.3 μg/kg LD then 0.5 μg/kg/h MD, 0.3 μg/kg LD then 0.8 μg/kg/h MD, 0.5 μg/kg LD then 0.6 μg/kg/h MD, 1 μg/kg then 0.5 μg/kg/h MD, 1 μg/kg LD then 0.6 μg/kg/h MD) significantly improved MMSE scores on postoperative day 7 (SMD 5, 95%CI 4.4 to 5.6; SMD 4.2, 95%CI 3.4 to 5.0; SMD 3.8, 95%CI 3.2 to 4.4; SMD 4.9, 95%CI 4.7 to 5.1; SMD 1.7, 95%CI 1.3 to 2.1; SMD 3.7, 95%CI 3.1 to 4.3; SMD 3.2, 95%CI 2.8 to 3.5; SMD 1.8, 95%CI 0.65 to 3; SMD 6.2, 95%CI 6 to 6.5; SMD 6.9, 95%CI 6.5 to 7.4, respectively) (Figure 5B). In the NMA, there were significant differences among different doses of DEX (Supplementary Table 1c). The cumulative ranking of different DEX doses versus NS on the improvement of MMSE on postoperative day 7 is shown in Figure 5C. Figure 5D for abbreviations and descriptions of the 12 DEX intervention doses and the control group. SUCRA analysis revealed that improvement in MMSE scores was the greatest with 1 μg/kg LD then 0.6 μg/kg/h MD (99.9%), followed by 1 μg/kg LD then 0.5 μg/kg/h MD (91.6%) and 1 μg/kg LD then 0.2 μg/kg/h MD (77.9%), and the least with NS (6.4%) and 0.5 μg/kg/h IOD (2.4%) (Figure 5C).
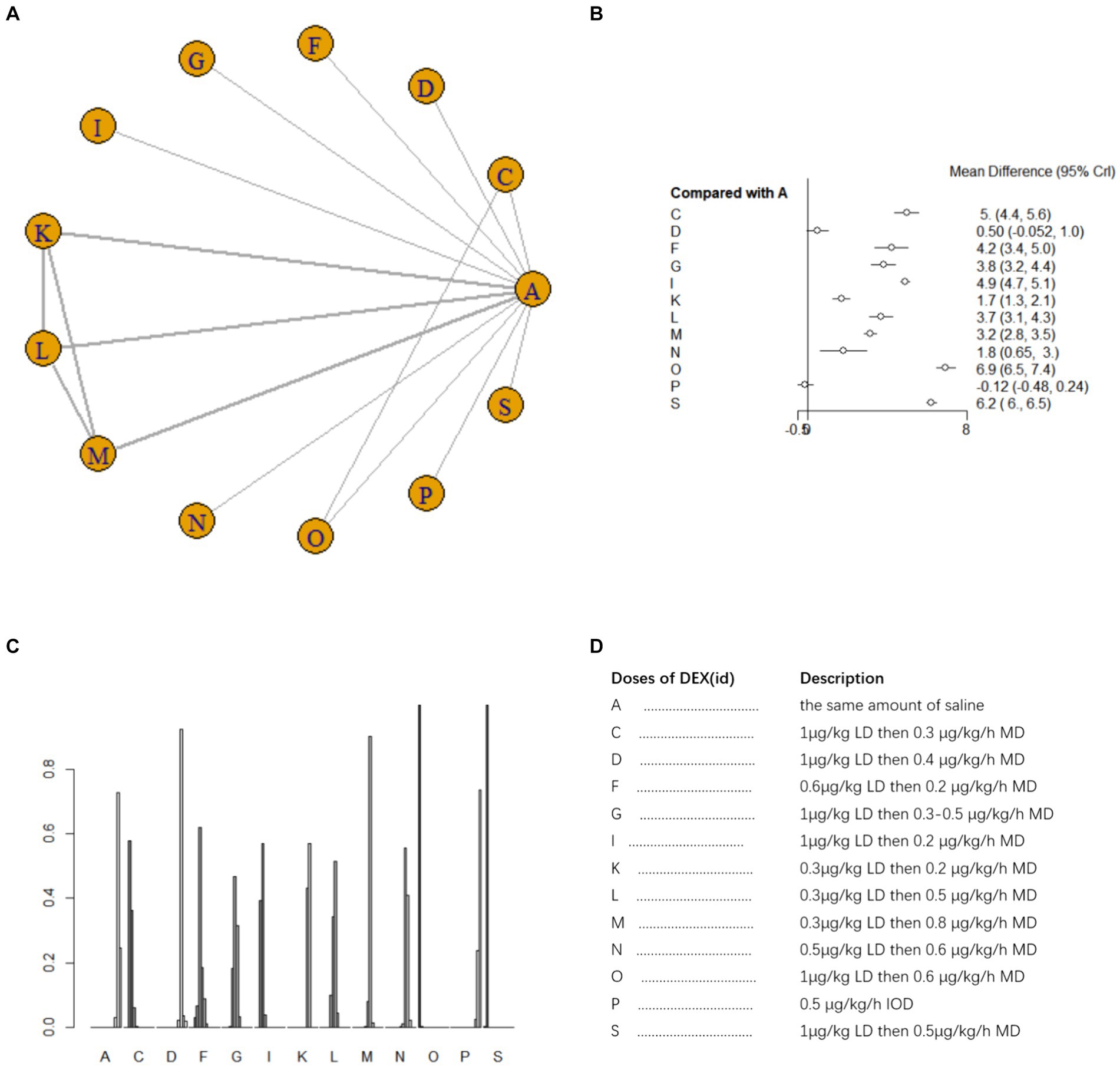
Figure 5. Network estimates of total MMSE score on day 7 after surgery. (A) Network plot showing direct comparisons between nodes. (B) Forest plot of different DEX doses compared with NS in NMA. (C) Estimated probability (%) of each dose level. (D) Description of individual DEX doses in this analysis. LD, Load dose; MD, Maintenance dose; IOD, Intraoperative dose.
3.4 Secondary outcomes: postoperative blood IL-6 and TNF-α levels
Ten studies (17, 18, 21, 25, 26, 33–35, 41, 42) involving 1,110 patients examined postoperative IL-6 level. There was high heterogeneity among the studies (I2 = 88%) and a random effects model was utilized. A total of 11 intervention doses were involved in the NMA, and the evidence relationships are shown in Figure 6A. Compared with the placebo, five DEX dosing regimens (1 μg/kg LD then 0.3 μg/kg/h MD, 0.3 μg/kg LD then 0.5 μg/kg/h MD;0.3 μg/kg LD then 0.8 μg/kg/h MD;1 μg/kg LD then 0.6ug/kg/h MD; 0.4 μg/kg/h IOD) significantly decreased postoperative IL-6 level (SMD-60, 95%CI -98 to −22; SMD -26 95%CI -52 to −1.2; SMD -32 95%CI -59 to −7.4; SMD -1.3e+0.2, 95%CI -1.7e to −98; SMD -1.1e+0.2, 95%CI -1.5e+0.2 to −72, respectively) (Figure 6B). Among the different doses, 1 μg/kg LD then 0.6 μg/kg/h led to significantly greater reduction in postoperative blood IL-6 level than most other dosing regimens; 1 μg/kg LD then 0.3 μg/h MD was significantly better than 0.3 μg/kg LD then 0.3 μg/kg/h MD and 0.3 μg/kg LD then 0.2 μg/kg/h MD; 0.4 μg/kg/h IOD was significantly worse than most other dosing regimens (Supplementary Table 1d). The cumulative ranking of different DEX doses versus NS in reducing postoperative blood IL-6 level is shown in Figure 6C, refer to Figure 6D for abbreviations and descriptions of the 11 DEX intervention doses and the control group, a lower SUCRA indicates superior efficacy. SUCRA analysis showed that 1 μg/kg LD then 0.6 μg/kg/h MD (1.4%), 0.4 μg/kg/h IOD (8.5%), and 1 μg/kg LD then 0.3 μg/h MD (20.8%) were the three most effective dosing regimens for lowering postoperative blood IL-6 level, whereas the same volume of NS (89.0%) and 0.3 μg/kg LD then 0.3 μg/kg/h MD (83.5%) were the least effective (Figure 6C).
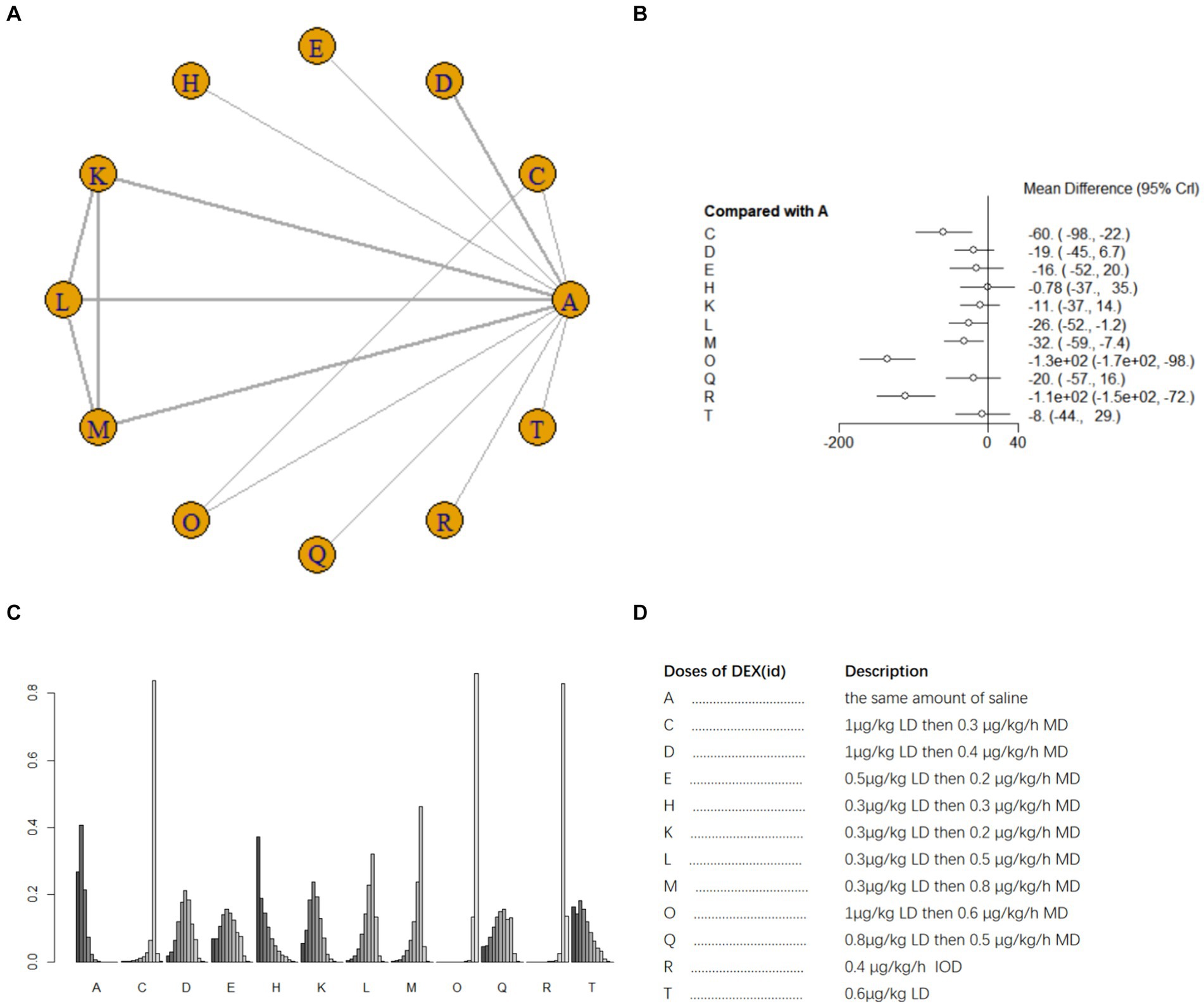
Figure 6. Network estimates of blood IL-6 level after surgery. (A) Network plot showing direct comparisons between nodes. (B) Forest plot of different DEX doses compared with NS in NMA. (C) Estimated probability (%) of each dose level. (D) Description of individual DEX doses in this analysis. LD, Load dose; MD, Maintenance dose; IOD, Intraoperative dose.
Nine studies (17, 18, 21, 25, 26, 33–35, 40) involving 1,073 patients reported postoperative blood TNF-α level. A random effects model was used for meta-analysis due to high heterogeneity among studies (I2 = 87%). A total of 10 intervention doses were involved in the NMA, and the evidence relationships are shown in Figure 7A. Compared with the placebo, three DEX dosing regimens 0.3 μg/kg LD then 0.2 μg/kg/h MD,0.3 μg/kg LD then 0.5 μg/kg/h MD, 0.3 μg/kg LD then 0.8 μg/kg/h MD significantly reduced postoperative blood TNF-α level (SMD-12, 95%CI -23 to −0.37; SMD-20, 95%CI -31 to −8.4; SMD -22, 95%CI -34 to −11, respectively) (Figure 7B). Among the different doses, the reduction in postoperative blood TNF-α level was significantly lower with 0.3 μg/kg LD then 0.3 μg/kg/h MD than with 0.3 μg/kg LD then 0.5 μg/kg/h MD and 0.3 μg/kg LD then 0.8 μg/kg/h MD (Supplementary Table 1e). Cumulative ranking of the 12 intervention doses from most effective to least effective based on the SURCA was 0.3 μg/kg LD then 0.8 μg/kg/h MD (6.6%) > 0.3 μg/kg LD then 0.5 μg/kg/h MD (14.2%) > 0.3 μg/kg LD then 0.2 μg/kg/h MD (42.6%) > 0.4 μg/kg/h IOD (43.1%) > 1 μg/kg LD then 0.4 μg/kg/h MD (43.8%) > 0.6 μg/kg/h IOD (46.5%) > 0.5 μg/kg LD then 0.2 μg/kg/h MD (48.4%) > 0.3 μg/kg/h IOD (54.6%) > 0.6 μg/kg LD (78.9%) > 0.3 μg/kg LD then 0.3 μg/kg/h MD (83.1%) > same volume of NS (87.6%) (Figure 7C), see Figure 7D for abbreviations and descriptions of the 10 DEX intervention doses and the control group.
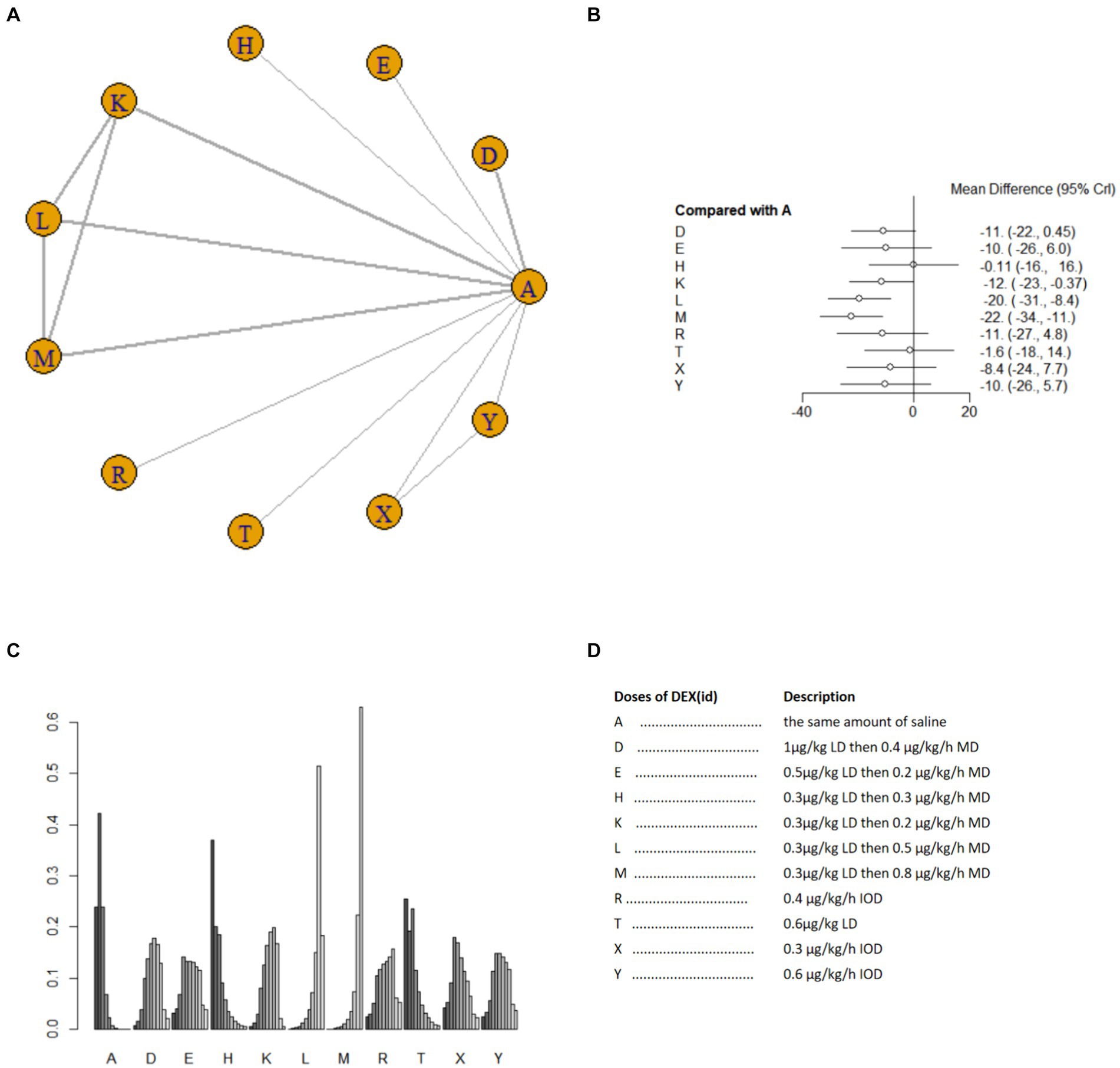
Figure 7. Network estimates of blood TNF-α level after surgery. (A) Network plot showing direct comparisons between nodes. (B) Forest plot of different DEX doses compared with NS in NMA. (C) Estimated probability (%) of each dose level. (D) Description of individual DEX doses in this analysis. LD, Load dose; MD, Maintenance dose; IOD, Intraoperative dose.
Visual inspection of the comparative adjusted funnel plots did not reveal publication bias in the postoperative day 1, 3, 7 MMSE scores and blood IL-6 lever in our network meta-analysis. The results of Egger’s test (p = 0.964, 0.993, 0.339 and 0.126) also negated the presence of a small study effect. Because there were no more than 10 studies on TNF-α, the publication bias analysis did not employ the inverted funnel plot method and the Egger’s test (Supplementary Figures 3a–d).
4 Discussion
The results of this study demonstrated that a 1 μg/kg LD followed by a 0.6 μg/kg/h MD was the best dosing regimen for improving postoperative MMSE scores on days 1, 3, and 7. Conversely, an IOD of 0.5 μg/kg/h had the least favorable effect. Additionally, the 1 μg/kg LD followed by 0.6 μg/kg/h MD regimen exhibited the greatest efficacy in reducing postoperative blood IL-6 level, while the 0.3 μg/kg LD followed by a 0.8 μg/kg/h MD regimen was the best approach for lowering postoperative blood TNF-α level. NS had the last effect on postoperative blood levels of IL-6 and TNF-α. Collectively, our findings demonstrate that a 1 μg/kg LD followed by a 0.6 μg/kg/h MD of DEX is superior for reducing postoperative blood IL-6 level and improving postoperative MMSE scores, while caution should be exercised when considering the 0.5 μg/kg/h IOD or 0.3 μg/kg LD then 0.2 μg/kg/h MD regimens. Although the certainty of evidence was low, these results nonetheless indicated that there is an optimal DEX dosing regimen that can improve postoperative cognitive function and postoperative blood IL-6 level. It is interesting to note that the optimal dose converges while the worst dose does not.
Does DEX exert neuroprotective effects through its anti-inflammatory action? DEX inhibits the production and release of key inflammatory mediators, such as cytokines IL-6 and TNF-α (43). These mediators are crucial in the inflammatory response, and their reduction diminishes the intensity and extent of inflammation. DEX influences immune cell function, such as macrophages and neutrophils (44), reducing their accumulation and activation at the inflammation site, thus mitigating the local inflammatory response. By inhibiting inflammatory mediators and reducing oxidative stress, DEX promotes nerve cell survival and decreases apoptosis rates. Inflammatory responses can harm the blood–brain barrier, increasing its permeability and worsening neurological damage. Dexmedetomidine’s anti-inflammatory effect helps maintain and restore the blood–brain barrier’s integrity (45), preventing harmful substances from entering brain tissue. These pathways demonstrate DEX’s significant neuroprotective and nerve repair effects, with its anti-inflammatory action being a crucial mechanism. Further research could delve into these mechanisms and assess DEX’s therapeutic potential in various neurological disorders.
We included studies in which DEX was used in different surgical and anaesthesia modalities. Our meta-analysis showed that DEX improves neurological outcomes in different surgical and anesthesia approaches, consistent with previous studies. A study comparing pre-anesthetic doses of ketamine and DEX in older patients undergoing emergency surgery showed promising results in reducing cognitive dysfunction (46). In addition, a study assessing the impact of DEX combined with etomidate on postoperative cognitive function in older patients undergoing ureteroscopic holmium laser lithotripsy revealed decreased incidences of cognitive dysfunction, emergent agitation, depression, and anxiety (47). Furthermore, a recent network meta-analysis involving 24 RCTs reported that intravenous DEX infusion during non-cardiac and non-neurosurgical procedures led to significantly reduced incidences of postoperative delirium (POD) and cognitive deficits when compared to alternative interventions (1). DEX has also demonstrated neuroprotective effects across different anesthesia modalities. It was found that the incidence of POD was lower in patients using DEX in spinal anaesthesia than in those using propofol (48). Additionally, a meta-analysis of 18 RCTs suggested that intravenous DEX sedation during surgery could play a crucial role in preventing POD and cognitive dysfunction in older patients (≥ 60 years old) receiving regional anesthesia. Moreover, transnasal administration of DEX has been shown to be effective for improving sleep disturbances, preventing neurocognitive impairment and POD, reducing anxiety, and minimizing adverse effects and complications in older patients undergoing general anaesthesia (49). Taken together, DEX is a promising sedative for enhancing cognitive function in various surgical procedures, anaesthesia modalities, and administration routes.
DEX has demonstrated favourable outcomes in postoperative sedation (50), pain management, anxiety reduction (51), cognitive function, and postoperative inflammation. Additionally, it has a unique and manageable mechanism of action that results in a low risk of respiratory depression (52) compared to other sedatives. However, DEX can also pose several adverse effects, including hypotension (53), bradycardia (54), and over-sedation. These risks may vary between different patient populations and should be closely monitored in the clinical setting.
Due to the presence of potential heterogeneity, the ranking of DEX doses in the NMA may not be directly applicable to future clinical treatment rankings. Therefore, our findings should be interpreted with caution, and randomized-controlled trials will be required to directly compare the effect of different DEX doses on postoperative cognitive function and inflammatory response.
This study has two strengths. First, this is the first and largest systematic review using a NMA approach to determine the effect of different DEX doses on postoperative cognitive function and inflammatory markers. The findings of this work provide valuable insight into the optimal DEX dosing regimen for reducing the incidences of postoperative cognitive dysfunction and postoperative inflammation. Second, the results of this systematic review and NMA can serve as support for future studies on the same topic.
Several limitations should be noted for this study. Differences in study design between RCTs and retrospective comparative studies (RCS) can impact result interpretation, since the latter is prone to selection bias and information bias due to its non-randomized nature. Additionally, high heterogeneity could indicate variations in populations, interventions, and outcome measures, which may impact result stability and interpretation. Lastly, potential limitations in data availability and study quality should be considered. Nonetheless, further large-cohort RCTs are warranted to minimize heterogeneity, improve internal validity, and assess the long-term safety and tolerability of DEX in different patient populations.
5 Conclusion
In conclusion, our NMA demonstrates the benefits of perioperative DEX use in improving postoperative cognitive function and postoperative inflammatory response. In addition, a 1 μg/kg LD then 0.6 μg/kg/h MD of DEX is the optimal dosing regimen for improving postoperative cognitive function and lowering blood IL-6 levels, whereas a 0.3 μg/kg LD then 0.2 μg/kg/h MD is the best regimen for lowering postoperative blood TNF-α level. Due to the limitations of this study and the heterogeneity among the included studies, these findings should be interpreted with caution. Nevertheless, we hope that our findings will provide valuable insights into the selection of appropriate DEX regimens for elective surgery patients.
Data availability statement
The original contributions presented in the study are included in the article/Supplementary material, further inquiries can be directed to the corresponding author.
Author contributions
CH: Conceptualization, Data curation, Formal analysis, Methodology, Software, Visualization, Writing – original draft, Writing – review & editing. RY: Data curation, Methodology, Writing – review & editing, Investigation, Supervision. XX: Investigation, Methodology, Writing – original draft. HD: Conceptualization, Resources, Supervision, Validation, Writing – review & editing. LP: Conceptualization, Data curation, Project administration, Resources, Supervision, Validation, Writing – review & editing.
Funding
The author(s) declare that no financial support was received for the research, authorship, and/or publication of this article.
Acknowledgments
We acknowledge the helpful comments on this article received from our reviewers.
Conflict of interest
The authors declare that the research was conducted in the absence of any commercial or financial relationships that could be construed as a potential conflict of interest.
Publisher’s note
All claims expressed in this article are solely those of the authors and do not necessarily represent those of their affiliated organizations, or those of the publisher, the editors and the reviewers. Any product that may be evaluated in this article, or claim that may be made by its manufacturer, is not guaranteed or endorsed by the publisher.
Supplementary material
The Supplementary material for this article can be found online at: https://www.frontiersin.org/articles/10.3389/fneur.2024.1422049/full#supplementary-material
References
1. Wang, D, Liu, Z, Zhang, W, Zu, G, Tao, H, and Bi, C. Intravenous infusion of dexmedetomidine during the surgery to prevent postoperative delirium and postoperative cognitive dysfunction undergoing non-cardiac surgery: a meta-analysis of randomized controlled trials. Eur J Med Res. (2024) 29:239. doi: 10.1186/s40001-024-01838-z
2. Wang, D, He, X, Li, Z, Tao, H, and Bi, C. The role of dexmedetomidine administered via intravenous infusion as adjunctive therapy to mitigate postoperative delirium and postoperative cognitive dysfunction in elderly patients undergoing regional anesthesia: a meta-analysis of randomized controlled trials. BMC Anesthesiol. (2024) 24:73. doi: 10.1186/s12871-024-02453-5
3. Zhao, Z, Ren, Y, Jiang, H, and Huang, Y. Dexmedetomidine inhibits the PSD95-NMDA receptor interaction to promote functional recovery following traumatic brain injury. Exp Ther Med. (2021) 21:4. doi: 10.3892/etm.2020.9436
4. Chen, Y, Wei, G, Feng, X, Lei, E, and Zhang, L. Dexmedetomidine enhances Mitophagy via PINK1 to alleviate hippocampal neuronal Pyroptosis and improve postoperative cognitive dysfunction in elderly rat. Exp Neurol. (2024) 379:114842. doi: 10.1016/j.expneurol.2024.114842
5. Rump, K, and Adamzik, M. Epigenetic Mechanisms of Postoperative Cognitive Impairment Induced by Anesthesia and Neuroinflammation. Cells. (2022):11. doi: 10.3390/cells11192954
6. Kho, W, von Haefen, C, Paeschke, N, Nasser, F, Endesfelder, S, Sifringer, M, et al. Dexmedetomidine Restores Autophagic Flux, Modulates Associated microRNAs and the Cholinergic Anti-inflammatory Pathway upon LPS-Treatment in Rats. J Neuroimmune Pharmacol. (2022) 17:261–76. doi: 10.1007/s11481-021-10003-w
7. Fu, S, Zhao, X, Li, Y, Fan, X, and Huang, Z. Dexmedetomidine alleviates hippocampal neuronal loss and cognitive decline in rats undergoing open surgery under sevoflurane anaesthesia by suppressing CCAAT/enhancer-binding protein beta. Eur J Neurosci. (2024) 59:36–53. doi: 10.1111/ejn.16193
8. Tang, C, Li, Y, and Lai, Y. Intraoperative Dexmedetomidine for Prevention of Postoperative Cognitive Dysfunction and Delirium in Elderly Patients with Lobectomy: A Propensity Score-Matched, Retrospective Study. Int J Gen Med. (2024) 17:2673–80. doi: 10.2147/ijgm.S456762
9. Xu, W, Zheng, Y, Suo, Z, Fei, K, Wang, Y, Liu, C, et al. Effect of dexmedetomidine on postoperative systemic inflammation and recovery in patients undergoing digest tract cancer surgery: A meta-analysis of randomized controlled trials. Front Oncol. (2022) 12:970557. doi: 10.3389/fonc.2022.970557
10. Hutton, B, Salanti, G, Caldwell, DM, Chaimani, A, Schmid, CH, Cameron, C, et al. The PRISMA extension statement for reporting of systematic reviews incorporating network meta-analyses of health care interventions: checklist and explanations. Ann Intern Med. (2015) 162:777–84. doi: 10.7326/m14-2385
11. Guo, Y, Pan, H, Chen, S, Tian, M, Huang, Y, and Zhou, Y. Effectiveness of acupuncture on urinary retention after radical hysterectomy for cervical cancer in China: a systematic review and meta-analysis. Front Med (Lausanne). (2024) 11:1375963. doi: 10.3389/fmed.2024.1375963
12. Neupane, B, Richer, D, Bonner, AJ, Kibret, T, and Beyene, J. Network meta-analysis using R: a review of currently available automated packages. PLoS One. (2014) 9:e115065. doi: 10.1371/journal.pone.0115065
13. PLOS ONE Staff. Correction: Network meta-analysis using R: a review of currently available automated packages. PLoS One. (2015) 10:e0123364. doi: 10.1371/journal.pone.0123364
14. Ao, CB, Wu, PL, Shao, L, Yu, JY, and Wu, WG. Clinical effect of ultrasound-guided nerve block and dexmedetomidine anesthesia on lower extremity operative fracture reduction. World J Clin Cases. (2022) 10:4064–71. doi: 10.12998/wjcc.v10.i13.4064
15. Chen, H, and Li, F. Effect of Dexmedetomidine with Different Anesthetic Dosage on Neurocognitive Function in Elderly Patients After Operation Based on Neural Network Model. World Neurosurg. (2020) 138:688–95. doi: 10.1016/j.wneu.2020.01.012
16. Chen, J, Yan, J, and Han, X. Dexmedetomidine may benefit cognitive function after laparoscopic cholecystectomy in elderly patients. Exp Ther Med. (2013) 5:489–94. doi: 10.3892/etm.2012.811
17. Chen, R, Kang, Z, Wang, Y, Zhao, J, and Li, S. The Anti-inflammatory Effect of Dexmedetomidine Administration on Patients Undergoing Intestinal Surgery: A Randomized Study. Drugs R D. (2021) 21:445–53. doi: 10.1007/s40268-021-00368-x
18. Chen, W, Liu, B, Zhang, F, Xue, P, Cui, R, and Lei, W. The effects of dexmedetomidine on post-operative cognitive dysfunction and inflammatory factors in senile patients. Int J Clin Exp Med. (2015) 8:4601–5.
19. Gao, Y, Yu, H, Wang, W, Wang, Y, Teng, J, and Li, F. Effect of Dexmedetomidine on The Neuroglobin Expression in Elderly Patients With Minimally Invasive Coronary Artery Bypass Graft Surgery. Heart Surg Forum. (2021) 24:E776–e80. doi: 10.1532/hsf.4073
20. Gao, Y, Zhu, X, Huang, L, Teng, J, and Li, F. Effects of dexmedetomidine on cerebral oxygen saturation and postoperative cognitive function in elderly patients undergoing minimally invasive coronary artery bypass surgery. Clin Hemorheol Microcirc. (2020) 74:383–9. doi: 10.3233/ch-190590
21. Ge, Y, Li, Q, Nie, Y, Gao, J, Luo, K, Fang, X, et al. Dexmedetomidine improves cognition after carotid endarterectomy by inhibiting cerebral inflammation and enhancing brain-derived neurotrophic factor expression. J Int Med Res. (2019) 47:2471–82. doi: 10.1177/0300060519843738
22. Gong, Z, Li, J, Zhong, Y, Guan, X, Huang, A, and Ma, L. Effects of dexmedetomidine on postoperative cognitive function in patients undergoing coronary artery bypass grafting. Exp Ther Med. (2018) 16:4685–9. doi: 10.3892/etm.2018.6778
23. Li, Y, He, R, Chen, S, and Qu, Y. Effect of dexmedetomidine on early postoperative cognitive dysfunction and peri-operative inflammation in elderly patients undergoing laparoscopic cholecystectomy. Exp Ther Med. (2015) 10:1635–42. doi: 10.3892/etm.2015.2726
24. Li, Y, Wang, C, Bi, M, Gao, J, Zhang, X, and Tian, H. Effect of dexmedetomidine on brain function and hemodynamics in patients undergoing lung cancer resection. Oncol Lett. (2020) 20:1077–82. doi: 10.3892/ol.2020.11675
25. Li, Z, Li, H, Yao, S, Cheng, M, and Chen, J. Effects of dexmedetomidine doses on postoperative cognitive dysfunction and serum β- amyloid and cytokine levels in elderly patients after spine surgery: a randomized controlled trial. Nan Fang Yi Ke Da Xue Xue Bao. (2021) 41:600–6. doi: 10.12122/j.issn.1673-4254.2021.04.18
26. Li, Z, Yao, S, Cheng, M, and Chen, J. Evaluation of the Effect of Dexmedetomidine on Postoperative Cognitive Dysfunction through Aβ and Cytokines Analysis. Iran J Pharm Res. (2021) 20:515–22. doi: 10.22037/ijpr.2020.113576.14381
27. Liu, Y, Zhu, X, He, Z, Sun, Z, Wu, X, and Zhong, J. Protective effect of dexmedetomidine infusion combined with epidural blockade on postoperative complications after surgery: A prospective randomized controlled clinical trial. J Int Med Res. (2020) 48:300060520930168. doi: 10.1177/0300060520930168
28. Shi, H, Du, X, Wu, F, Hu, Y, Xv, Z, and Mi, W. Dexmedetomidine improves early postoperative neurocognitive disorder in elderly male patients undergoing thoracoscopic lobectomy. Exp Ther Med. (2020) 20:3868–77. doi: 10.3892/etm.2020.9113
29. Shi, HX, Du, XJ, Wu, F, Hu, YJ, and Mi, WD. Dexmedetomidine for early postoperative cognitive dysfunction after video-assisted thoracoscopic lobectomy in elderly male patients with lung cancer. Medicine (Baltimore). (2020) 99:e21691. doi: 10.1097/md.0000000000021691
30. Wang, F, Xie, D, Xu, H, Ye, Q, Wu, L, and Gao, XP. The effects of remifentanil-propofol combined with dexmedetomidine on cognitive dysfunction in elderly patients after ureteroscopic holmium laser lithotripsy: a double-blind randomized controlled trial. Trials. (2022) 23:192. doi: 10.1186/s13063-022-06121-2
31. Wang, Z, Chen, Q, Guo, H, Li, Z, Zhang, J, Lv, L, et al. Effects of dexmedetomidine on H-FABP, CK-MB, cTnI levels, neurological function and near-term prognosis in patients undergoing heart valve replacement. Exp Ther Med. (2017) 14:5851–6. doi: 10.3892/etm.2017.5265
32. Zhu, Y, and Le, G. The effect of dexmedetomidine combined with epidural anesthesia on post-operative cognitive dysfunction in elderly patients after orthopedic surgery. Am J Transl Res. (2021) 13:12058–64.
33. Zhou, M, Lyu, Y, Zhu, Y, Jiang, T, Wu, C, Yang, J, et al. Effect of Ulinastatin Combined With Dexmedetomidine on Postoperative Cognitive Dysfunction in Patients Who Underwent Cardiac Surgery. Front Neurol. (2019) 10:1293. doi: 10.3389/fneur.2019.01293
34. Zhang, Z, Li, W, and Jia, H. Postoperative Effects of Dexmedetomidine on Serum Inflammatory Factors and Cognitive Malfunctioning in Patients with General Anesthesia. J Healthc Eng. (2021) 2021:7161901. doi: 10.1155/2021/7161901
35. Xu, Y, and Du, X. Application of dexmedetomidine-assisted intravertebral anesthesia in hip replacement and its influence on T-lymphocyte subsets. Exp Ther Med. (2020) 20:1269–76. doi: 10.3892/etm.2020.8869
36. Du, X, Song, F, Zhang, X, and Ma, S. Protective efficacy of combined use of parecoxib and dexmedetomidine on postoperative hyperalgesia and early cognitive dysfunction after laparoscopic cholecystectomy for elderly patients. Acta Cir Bras. (2019) 34:e201900905. doi: 10.1590/s0102-865020190090000005
37. Ma, XD, Li, BP, Wang, DL, and Yang, WS. Postoperative benefits of dexmedetomidine combined with flurbiprofen axetil after thyroid surgery. Exp Ther Med. (2017) 14:2148–52. doi: 10.3892/etm.2017.4717
38. Wang, Z, Shen, Z, Wang, H, Zhang, L, and Dong, R. Effect of dexmedetomidine on the cognitive function of patients undergoing gastric cancer surgery by regulating the PI3K/AKT signaling pathway. Oncol Lett. (2020) 19:1151–6. doi: 10.3892/ol.2019.11224
39. M. L. The effects of dexmedetomidine in general anesthesia on the perioperative hemodynamics and postoperative cognitive functions of patients with sleep apnea syndrome in the perioperative period of uvulopalatopharyngoplasty. Int. J Clin Exp Med. (2020) 13:2086–94.
40. Tang, Y, Wang, Y, Kong, G, Zhao, Y, Wei, L, and Liu, J. Prevention of dexmedetomidine on postoperative delirium and early postoperative cognitive dysfunction in elderly patients undergoing hepatic lobectomy. Zhong Nan Da Xue Xue Bao Yi Xue Ban. (2022) 47:219–25. doi: 10.11817/j.issn.1672-7347.2022.210280
41. Xu, HY, Fu, GH, and Wu, GS. Effect of dexmedetomidine-induced anesthesia on the postoperative cognitive function of elder patients after laparoscopic ovarian cystectomy. Saudi J Biol Sci. (2017) 24:1771–5. doi: 10.1016/j.sjbs.2017.11.010
42. Li, XT, Jiang, XM, Zheng, ZY, and Huang, HS. Effect of dexmedetomidine on inflammatory factors level and cognitive function after femoral head replacement in elderly patients. Zhongguo Gu Shang. (2018) 31:1091–5. doi: 10.3969/j.issn.1003-0034.2018.12.003
43. Hou, M, Chen, F, He, Y, Tan, Z, Han, X, Shi, Y, et al. Dexmedetomidine against intestinal ischemia/reperfusion injury: A systematic review and meta-analysis of preclinical studies. Eur J Pharmacol. (2023) 959:176090. doi: 10.1016/j.ejphar.2023.176090
44. Heil, LBB, Braga, CL, Magalhães, RF, Antunes, MA, Cruz, FF, Samary, CS, et al. Dexmedetomidine compared to low-dose ketamine better protected not only the brain but also the lungs in acute ischemic stroke. Int Immunopharmacol. (2023) 124:111004. doi: 10.1016/j.intimp.2023.111004
45. Liu, S, Jia, X, Liu, B, Liu, Y, and Yin, H. Suppression of cerebral ischemia injury induced blood brain barrier breakdown by dexmedetomidine via promoting CCN1. Aging (Albany NY). (2024) 16:3750–62. doi: 10.18632/aging.205557
46. Ghazaly, HF, Hemaida, TS, Zaher, ZZ, Elkhodary, OM, and Hammad, SS. A pre-anesthetic bolus of ketamine versus dexmedetomidine for prevention of postoperative delirium in elderly patients undergoing emergency surgery: a randomized, double-blinded, placebo-controlled study. BMC Anesthesiol. (2023) 23:407. doi: 10.1186/s12871-023-02367-8
47. Fu, W, Xu, H, Zhao, T, Xu, J, and Wang, F. Effects of dexmedetomidine combined with etomidate on postoperative cognitive function in older patients undergoing total intravenous anaesthesia: a randomized, double-blind, controlled trial. BMC Geriatr. (2024) 24:97. doi: 10.1186/s12877-024-04726-7
48. Shin, HJ, Woo Nam, S, Kim, H, Yim, S, Han, SH, Hwang, JW, et al. Postoperative Delirium after Dexmedetomidine versus Propofol Sedation in Healthy Older Adults Undergoing Orthopedic Lower Limb Surgery with Spinal Anesthesia: A Randomized Controlled Trial. Anesthesiology. (2023) 138:164–71. doi: 10.1097/aln.0000000000004438
49. He, J, Zhang, X, Li, C, Fu, B, Huang, Y, and Li, H. Dexmedetomidine nasal administration improves perioperative sleep quality and neurocognitive deficits in elderly patients undergoing general anesthesia. BMC Anesthesiol. (2024) 24:42. doi: 10.1186/s12871-024-02417-9
50. Bhalotra, AR, Arya, M, Singh, R, and Dhiman, S. Comparative evaluation of nebulized versus intravenous dexmedetomidine on intubating conditions during awake fiberoptic nasotracheal intubation. J Clin Anesth. (2024) 95:111461. doi: 10.1016/j.jclinane.2024.111461
51. Lv, X, Zhang, H, Gao, J, Hou, A, Ma, Y, Zhou, Z, et al. Intraoperative dexmedetomidine on postoperative pain in gastrointestinal surgery: an observational study. Int J Surg. (2023) 109:887–95. doi: 10.1097/js9.0000000000000360
52. Zhang, W, Wang, L, Zhu, N, Wu, W, and Liu, H. A prospective, randomized, single-blinded study comparing the efficacy and safety of dexmedetomidine and propofol for sedation during endoscopic retrograde cholangiopancreatography. BMC Anesthesiol. (2024) 24:191. doi: 10.1186/s12871-024-02572-z
53. Yang, SS, Gelinas, C, Yim, E, Li, MMJ, Kardash, K, Zhang, M, et al. Association of intraoperative dexmedetomidine use with postoperative hypotension in unilateral hip and knee arthroplasties: a historical cohort study. Can J Anaesth. (2022) 69:1459–70. doi: 10.1007/s12630-022-02339-5
Keywords: dexmedetomidine, postoperative cognitive complications, postoperative cognitive disorder, inflammation, systematic review, network meta-analysis
Citation: Huang C, Yang R, Xie X, Dai H and Pan L (2024) Effects of dexmedetomidine on early postoperative cognitive function and postoperative inflammatory response: a systematic review and network meta-analysis. Front. Neurol. 15:1422049. doi: 10.3389/fneur.2024.1422049
Edited by:
Yucheng Xiao, Indiana University, United StatesReviewed by:
Mahmoud A. Ebada, Zagazig University, EgyptHui Zheng, Chinese Academy of Medical Sciences and Peking Union Medical College, China
Copyright © 2024 Huang, Yang, Xie, Dai and Pan. This is an open-access article distributed under the terms of the Creative Commons Attribution License (CC BY). The use, distribution or reproduction in other forums is permitted, provided the original author(s) and the copyright owner(s) are credited and that the original publication in this journal is cited, in accordance with accepted academic practice. No use, distribution or reproduction is permitted which does not comply with these terms.
*Correspondence: Linghui Pan, cGFubGluZ2h1aUBneG11LmVkdS5jbg==