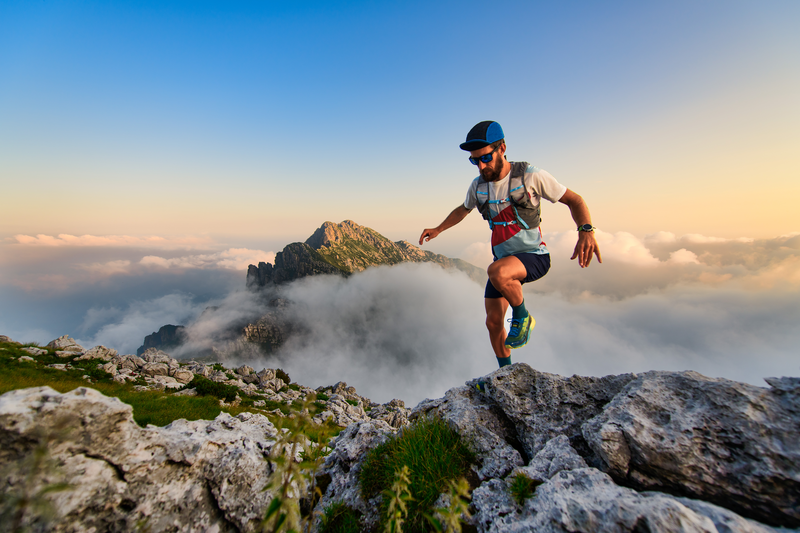
95% of researchers rate our articles as excellent or good
Learn more about the work of our research integrity team to safeguard the quality of each article we publish.
Find out more
OPINION article
Front. Neurol. , 21 June 2024
Sec. Neurotrauma
Volume 15 - 2024 | https://doi.org/10.3389/fneur.2024.1420324
We read with interest the review article by Zou et al. (1) entitled “Techniques and graft materials for repairing peripheral nerve defects”. Here, the Authors discuss current approaches to peripheral nerve injury (PNI) with substance loss (autograft/allograft/nerve conduit), also highlighting methods' advantages and disadvantages, following our previous contribution (2). In addition, appealing materials for nerve conduits (NC) fabrication were overviewed too (1), highlighting that their intrinsic characteristics can affect the regeneration outcome (1, 2).
Certainly, while looking for the ideal device, we believe that together with a focus on conduit material, conduit topography must be considered too, as significantly modulating nerve regeneration across the gap. Based on that, design strategies providing nerve guides with specific topographic cues will be described and discussed below.
According to size, biomaterials pores can be classified as macropores (100–500 μm), micropores (< 100 μm), and nanopores (< 1,000 nm). Surely, wall pore dimension affects the device inner environment; acting as a strain, a porous wall can select the molecules that can be exchanged between the regenerated peripheral nerve and the surrounding environment, thus influencing cell viability/proliferation/blood vessels infiltration (3, 4).
Fully permeable (pore diameter: >50 μm), semi-permeable (pore diameter: < 10 μm), and asymmetric (external surface pore size > lumen surface pore size) conduits have been developed to overcome autograft issues in case of severe PNI. These nerve guides showed to improve the neuro-regenerative process under different aspects. Specifically, semi-permeable conduits can avoid fibrous scar formation; fully-permeable conduits can promote direct signal communication between cells; asymmetric structures-based conduits can assure for efficient removal of metabolic waste. Nevertheless, some intrinsic disadvantages related to their use still need to be addressed, including no direct signal communication between cells affecting semi-permeable and asymmetric structures and the risk of fibrous infiltration concerning fully-permeable devices (3).
Porosity and pore size are often dependent on scaffold fabrication method (4); conduits fabricated using traditional methods are mainly characterized by pores with random morphology. Hence, lithography processes, electrospinning, and 3D printing technology are gaining researchers' attention, distinguishing as promising techniques in exerting control over wall porosity dimension/organization (2).
Schwann cells (SCs) growth direction and migration in the postinjury microenvironment exert a fundamental role in promoting axon growth. Thus, the development of conduits displaying a regular topology can discourage random distribution of SCs (5). NC eventually endowed by anisotropic topographical cues, consisting in micro- and nanogroove patterns in the luminal wall, have received extensive attention. In fact, patterns can trigger a contact guidance effect, thus instructing cells to align along a preferential direction and significantly influencing neurite outgrowth, cellular alignment, elongation and differentiation (6–8).
Thin microgrooves (5–10 μm) confine the growth of cells and axon elongation; grooves with widths and spacings of 10–20 μm favorably support SC adhesion and proliferation; patterns comparable with cell dimensions (10–50 μm) are appealing too. The narrower microgrooves were found to improve axonal alignment, diminish the number of axon branches per cell, and decrease incorrect distal re-connection (2, 9). Groove depth can also affect surface/cells interaction. To support neurite alignment or outgrowth, depths ranging from 1 to 4 μm are adequate; conversely, sizes inferior to 300 nm revealed to be less favorable. Difficulties in fabrication are the main critical issue in the development of grooved conduits (2, 10). Various approaches have been developed to produce scaffolds with anisotropic structure, including electrospinning, lithography, and 3D bioprinting (7).
A multichannel conduit may be more likely to preserve the linear organization of regenerating axons in the peripheral nerve (10).
Introduction of macroscale, longitudinally oriented multichannels within NC can guarantee a permissive pathway for axon growth, favoring SCs' attachment, as well as growth factors' release. Contextually, they can reduce the dispersion of regenerating axons avoiding their own consequent misdirection, eventually associated with different target polyinnervation (11, 12). According to the literature, the number/diameter of the channels within a single conduit may vary, ranging from 1 channel/conduit (13) to over 100 (14). Certainly, the presence of multichannels within the conduits provides for a regenerative environment which better mimics the native nerve fascicular structure (2).
Different methods have been adopted for multichannel conduit fabrication, including solvent or thermally induced phase separation, injection molding, electrospinning, and 3D printing (15).
Introducing luminal fillers into conduits aims not only to enhance conduit-associated outcomes at shorter distances but also to improve nerve reconstruction in case of wide gaps (16). Several studies report the incorporation of macro-, micro-, and nanoscale filler materials within the NC to encourage axon regeneration (12). Different natural (e.g., fibrin, collagen, laminin, and agarose) and synthetic polymers [e.g., polyamide, polyacrylonitrile-co-methylacrylate, polyglycolic acid, poly-L-lactic acid, and poly(lactic-co-glycolic acid)] have been used as solutions, hydrogel filaments, porous sponges or films (2, 9, 17, 18).
Recently, attention has been directed to Self-Assembling Peptides (SAPs), mimicking ECM organization. SAPs consist in short peptide molecules self-organizing into stable secondary structures (α-helix, β-sheet, or random coil) and further forming various aggregation states (fibrils, fibril networks, membranes, and gels) under in vivo pH and ion concentration (2, 19–25). Additionally, SAPs functionalization with the laminin-derived peptide IKVAV (sequence: isoleucine–lysine–valine–alanine–valine) (19–21, 23), or brain-derived neurotrophic factor (BDNF) (21) have been also used to improve outcomes of chitosan or poly(L-lactide) (PLLA) conduits.
Intense efforts are dedicated toward the fabrication of the ideal NC. Certainly, the biomaterial adopted for devices' fabrication has a fundamental role over their outcomes; interestingly, a blend of synthetic and natural polymers can assure for appropriate structural/mechanical support (synthetic polymer) and biomimicry (natural polymer) (26). However, providing topographic cues is essential too. Porous and grooved walls, multi-channel conduits, and NC with fillers (in the form of fibers or hydrogels) are among the most appealing strategies for conduits structure/ultrastructure bioactivation (2, 27). The selected biomaterial and the desired topographical cues to be introduce will critically guide toward the fabrication technique to be adopted (12).
ES: Writing – original draft, Conceptualization. SB: Writing – original draft. AE: Writing – review & editing. CT: Writing – review & editing. RDC: Writing – review & editing, Supervision. VM: Writing – review & editing, Supervision. AP: Writing – review & editing, Conceptualization.
The author(s) declare financial support was received for the research, authorship, and/or publication of this article. Open Access funding provided by Università degli Studi di Padova | University of Padua, Open Science Committee.
The authors declare that the research was conducted in the absence of any commercial or financial relationships that could be construed as a potential conflict of interest.
All claims expressed in this article are solely those of the authors and do not necessarily represent those of their affiliated organizations, or those of the publisher, the editors and the reviewers. Any product that may be evaluated in this article, or claim that may be made by its manufacturer, is not guaranteed or endorsed by the publisher.
1. Zou X, Dong Y, Alhaskawi A, Zhou H, Ezzi SHA, Kota VG, et al. Techniques and graft materials for repairing peripheral nerve defects. Front Neurol. (2024) 14:1307883. doi: 10.3389/fneur.2023.1307883
2. Stocco E, Barbon S, Emmi A, Tiengo C, Macchi V, De Caro R, et al. Bridging gaps in peripheral nerves: from current strategies to future perspectives in conduit design. Int J Mol Sci. (2023) 24:9170. doi: 10.3390/ijms24119170
3. Wan T, Wang YL, Zhang FS, Zhang XM, Zhang YC, Jiang HR, et al. The porous structure of peripheral nerve guidance conduits: features, fabrication, and implications for peripheral nerve regeneration. Int J Mol Sci. (2023) 24:14132. doi: 10.3390/ijms241814132
4. Apablaza JA, Lezcano MF, Lopez Marquez A, Godoy Sánchez K, Oporto GH, Dias FJ. Main morphological characteristics of tubular polymeric scaffolds to promote peripheral nerve regeneration-A scoping review. Polymers. (2021) 13:2563. doi: 10.3390/polym13152563
5. Zhang D, Wu S, Feng J, Duan Y, Xing D, Gao C. Micropatterned biodegradable polyesters clicked with CQAASIKVAV promote cell alignment, directional migration, and neurite outgrowth. Acta Biomater. (2018) 74:143–55. doi: 10.1016/j.actbio.2018.05.018
6. Jeon J, Lee MS, Lim J, Park S, Kim SM, Kim D, et al. Micro-grooved nerve guidance conduits combined with microfiber for rat sciatic nerve regeneration. J Ind Eng Chem. (2020) 2020:214–23. doi: 10.1016/j.jiec.2020.07.014
7. Hu Y, Zhang H, Wei H, Cheng H, Cai J, Chen X, et al. Scaffolds with anisotropic structure for neural tissue engineering. Eng Regener. (2022) 3:154–62. doi: 10.1016/j.engreg.2022.04.001
8. Zhang D, Li Z, Shi H, Yao Y, Du W, Lu P, et al. Micropatterns and peptide gradient on the inner surface of a guidance conduit synergistically promotes nerve regeneration in vivo. Bioact Mater. (2021) 9:134–46. doi: 10.1016/j.bioactmat.2021.07.010
9. Carvalho CR, Oliveira JM, Reis RL. Modern trends for peripheral nerve repair and regeneration: beyond the hollow nerve guidance conduit. Front Bioeng Biotechnol. (2019) 7:337. doi: 10.3389/fbioe.2019.00337
10. Hoffman-Kim D, Mitchel JA, Bellamkonda RV. Topography, cell response, and nerve regeneration. Annu Rev Biomed Eng. (2010) 12:203–31. doi: 10.1146/annurev-bioeng-070909-105351
11. de Ruiter GC, Spinner RJ, Malessy MJ, Moore MJ, Sorenson EJ, Currier BL, et al. Accuracy of motor axon regeneration across autograft, single-lumen, and multichannel poly(lactic-co-glycolic acid) nerve tubes. Neurosurgery. (2008) 63:144–55. doi: 10.1227/01.NEU.0000319521.28683.75
12. Sarker M, Naghieh S, McInnes AD, Schreyer DJ, Chen X. Strategic design and fabrication of nerve guidance conduits for peripheral nerve regeneration. Biotechnol J. (2018) 13:e1700635. doi: 10.1002/biot.201700635
13. Yao L, de Ruiter GC, Wang H, Knight AM, Spinner RJ, Yaszemski MJ, et al. Controlling dispersion of axonal regeneration using a multichannel collagen nerve conduit. Biomaterials. (2010) 31:5789–97. doi: 10.1016/j.biomaterials.2010.03.081
14. Sundback C, Hadlock T, Cheney M, Vacanti J. Manufacture of porous polymer nerve conduits by a novel low-pressure injection molding process. Biomaterials. (2003) 24:819–30. doi: 10.1016/S0142-9612(02)00409-X
15. Wang J, Cheng Y, Wang H, Wang Y, Zhang K, Fan C, et al. Biomimetic and hierarchical nerve conduits from multifunctional nanofibers for guided peripheral nerve regeneration. Acta Biomater. (2020) 117:180–91. doi: 10.1016/j.actbio.2020.09.037
16. Chen MB, Zhang F, Lineaweaver WC. Luminal fillers in nerve conduits for peripheral nerve repair. Ann Plast Surg. (2006) 57:462–71. doi: 10.1097/01.sap.0000237577.07219.b6
17. Wang S, Cai L. Polymers for fabricating nerve conduits. Int J Polym Sci. (2010) 2010:138686. doi: 10.1155/2010/138686
18. Meyer C, Stenberg L, Gonzalez-Perez F, Wrobel S, Ronchi G, Udina E, et al. Chitosan-film enhanced chitosan nerve guides for long-distance regeneration of peripheral nerves. Biomaterials. (2016) 76:33–51. doi: 10.1016/j.biomaterials.2015.10.040
19. Wu X, He L, Li W, Li H, Wong WM, Ramakrishna S, et al. Functional self-assembling peptide nanofiber hydrogel for peripheral nerve regeneration. Regen Biomater. (2017) 4:21–30. doi: 10.1093/rb/rbw034
20. Yang S, Wang C, Zhu J, Lu C, Li H, Chen F, et al. Self-assembling peptide hydrogels functionalized with LN- and BDNF- mimicking epitopes synergistically enhance peripheral nerve regeneration. Theranostics. (2020) 10:8227–49. doi: 10.7150/thno.44276
21. Yang S, Zhu J, Lu C, Chai Y, Cao Z, Lu J, et al. Aligned fibrin/functionalized self-assembling peptide interpenetrating nanofiber hydrogel presenting multi-cues promotes peripheral nerve functional recovery. Bioact Mater. (2021) 8:529–44. doi: 10.1016/j.bioactmat.2021.05.056
22. Stocco E, Barbon S, Lamanna A, De Rose E, Zamuner A, Sandrin D, et al. Bioactivated oxidized polyvinyl alcohol towards next-generation nerve conduits development. Polymers. (2021) 13:3372. doi: 10.3390/polym13193372
23. Shen X, Qu F, Pei Y, Lei S, Xia S, Liang J, et al. Repairing sciatic nerve injury with self-assembling peptide nanofiber scaffold-containing chitosan conduit. Front Neurol. (2022) 13:867711. doi: 10.3389/fneur.2022.867711
24. Stocco E, Barbon S, Zeni E, Cassari L, Zamuner A, Gloria A, et al. Development of two-layer hybrid scaffolds based on oxidized polyvinyl alcohol and bioactivated chitosan sponges for tissue engineering purposes. Int J Mol Sci. (2022) 23:12059. doi: 10.3390/ijms232012059
25. Stocco E, Barbon S, Faccio D, Petrelli L, Incendi D, Zamuner A, et al. Development and preclinical evaluation of bioactive nerve conduits for peripheral nerve regeneration: a comparative study. Mater Today Bio. (2023) 22:100761. doi: 10.1016/j.mtbio.2023.100761
26. Sionkowska A. Current research on the blends of natural and synthetic polymers as new biomaterials. Prog Polym Sci. (2011) 36:1254–76. doi: 10.1016/j.progpolymsci.2011.05.003
Keywords: peripheral nerve injury, nerve conduits, luminal filler, bioactivation, tissue engineering
Citation: Stocco E, Barbon S, Emmi A, Tiengo C, De Caro R, Macchi V and Porzionato A (2024) Commentary: Techniques and graft materials for repairing peripheral nerve defects. Front. Neurol. 15:1420324. doi: 10.3389/fneur.2024.1420324
Received: 26 April 2024; Accepted: 29 May 2024;
Published: 21 June 2024.
Edited by:
Lingxiao Deng, Indiana University, United StatesReviewed by:
Rodrigo Ramos-Zúñiga, University of Guadalajara, MexicoCopyright © 2024 Stocco, Barbon, Emmi, Tiengo, De Caro, Macchi and Porzionato. This is an open-access article distributed under the terms of the Creative Commons Attribution License (CC BY). The use, distribution or reproduction in other forums is permitted, provided the original author(s) and the copyright owner(s) are credited and that the original publication in this journal is cited, in accordance with accepted academic practice. No use, distribution or reproduction is permitted which does not comply with these terms.
*Correspondence: Raffaele De Caro, cmFmZmFlbGUuZGVjYXJvQHVuaXBkLml0; Veronica Macchi, dmVyb25pY2EubWFjY2hpQHVuaXBkLml0
Disclaimer: All claims expressed in this article are solely those of the authors and do not necessarily represent those of their affiliated organizations, or those of the publisher, the editors and the reviewers. Any product that may be evaluated in this article or claim that may be made by its manufacturer is not guaranteed or endorsed by the publisher.
Research integrity at Frontiers
Learn more about the work of our research integrity team to safeguard the quality of each article we publish.