- 1Department of Neurology, Danish Headache Center, Copenhagen University Hospital- Rigshospitalet, Copenhagen, Denmark
- 2Department of Clinical Medicine, Faculty of Health and Medical Sciences, University of Copenhagen, Copenhagen, Denmark
- 3Department of Neurology, Nordsjaellands Hospital- Hilleroed, Hilleroed, Denmark
Cumulative evidence suggests that ATP-sensitive potassium (KATP) channels act as a key regulator of cerebral blood flow (CBF). This implication seems to be complicated, since KATP channels are expressed in several vascular-related structures such as smooth muscle cells, endothelial cells and pericytes. In this systematic review, we searched PubMed and EMBASE for preclinical and clinical studies addressing the involvement of KATP channels in CBF regulation. A total of 216 studies were screened by title and abstract. Of these, 45 preclinical and 6 clinical studies were included. Preclinical data showed that KATP channel openers (KCOs) caused dilation of several cerebral arteries including pial arteries, the middle cerebral artery and basilar artery, and KATP channel inhibitor (KCI) glibenclamide, reversed the dilation. Glibenclamide affected neither the baseline CBF nor the baseline vascular tone. Endothelium removal from cerebral arterioles resulted in an impaired response to KCO/KCI. Clinical studies showed that KCOs dilated cerebral arteries and increased CBF, however, glibenclamide failed to attenuate these vascular changes. Endothelial KATP channels played a major role in CBF regulation. More studies investigating the role of KATP channels in CBF-related structures are needed to further elucidate their actual role in cerebral hemodynamics in humans.
Systematic review registration: Prospero: CRD42023339278 (preclinical data) and CRD42022339152 (clinical data).
Introduction
Cerebral hemodynamics including cerebral blood flow (CBF) and cerebral vascular tone are vital parameters contributing to brain homeostasis (1). Dysregulation of cerebrovascular hemodynamics is involved in the pathogenesis of several neurological disorders such as stroke and migraine (2, 3). The molecular mechanisms involved in the modulation of cerebral hemodynamics are complex and not entirely comprehended.
Evidence from preclinical and clinical studies implicates ATP-sensitive potassium (KATP) channels in the regulation of CBF and the cerebral vascular tone (4–6). KATP channels are vastly expressed at several structures of the vasculature such as arteries, penetrating arterioles and the complex mesh of capillaries. Specifically, KATP channels are present in smooth muscle cells (SMCs), endothelial cells (ECs) and pericytes (7–12) (Figure 1). KATP channels link the cellular metabolic state to the plasmalemma’s electrophysiology. They are activated during ischemia and hypoxia, causing potassium efflux, hyperpolarization and subsequently vasodilation (17–19) (Figure 2).
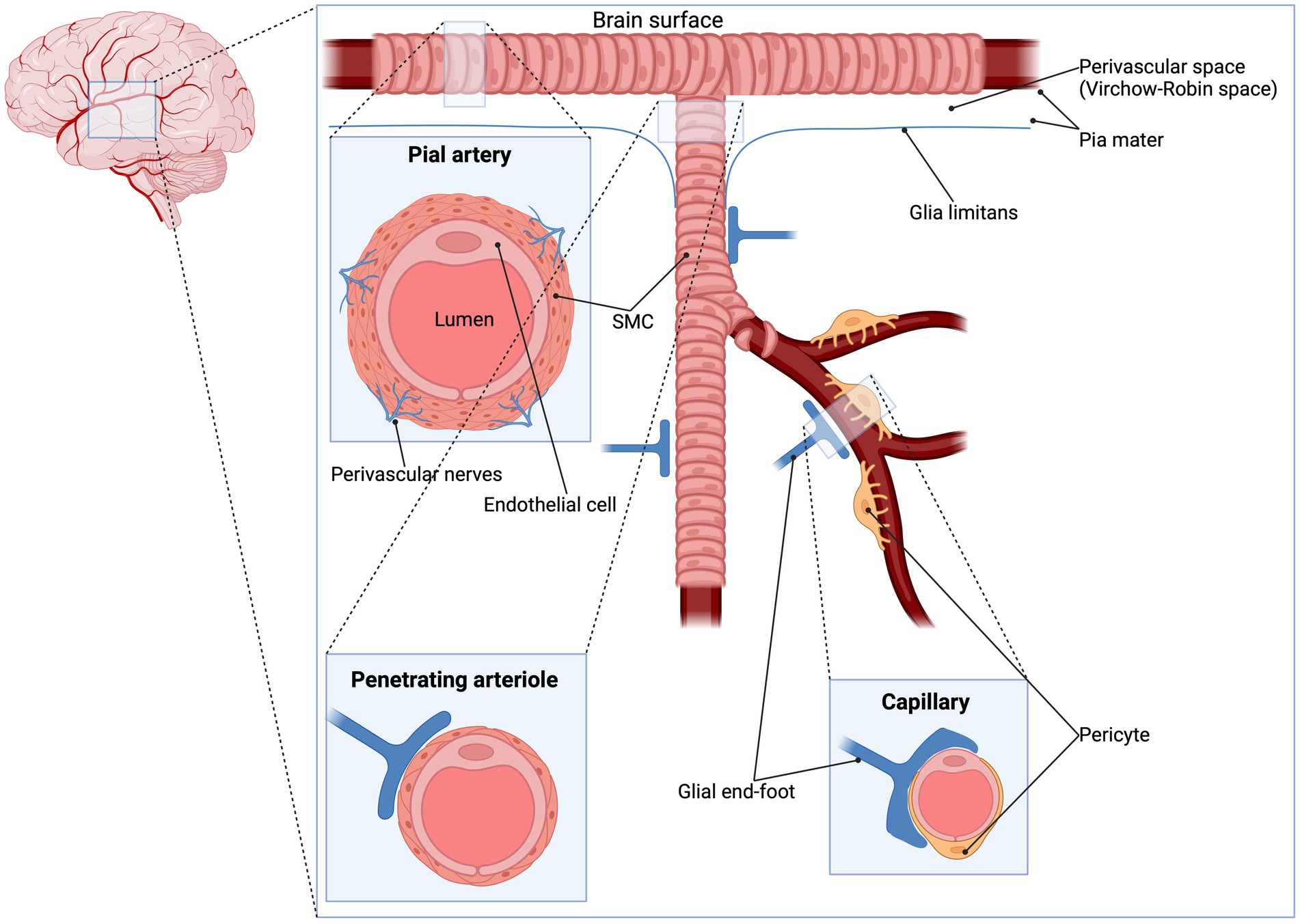
Figure 1. Pial artery, penetrating arteriole and capillary. The pial arterial vasculature (also known as pial collaterals or leptomeningeal anastomoses) consists of smaller arteries and arterioles that connects the three major supplying the arteries of the cerebrum: the anterior cerebral artery, the middle cerebral artery and the posterior cerebral artery (13). The pial arteries are intracranial arteries on the surface of the brain within the pia-arachnoid (leptomeninges) or glia limitans (the outmost layer of the cortex composed of glial end-feet), surrounded by cerebrospinal fluid (14) and give rise to smaller penetrating arterioles (15). An important difference in vessel architecture which might influence the CBF regulation is the number of SMC layers: penetrating arterioles contain one layer of smooth muscle while smaller pial arteries contains two to three layers of smooth muscle (16). Since KATP channels are expressed in SMC, it is expected that these channels have a higher impact in pial arteries. To date, no studies did compare the effect of KCO/KCI between these types of vessels. CBF, cerebral blood flow; KATP, ATP-sensitive potassium; KCI; KATP channel inhibitor KCO; KATP channel opener; SMC, smooth muscle cell.
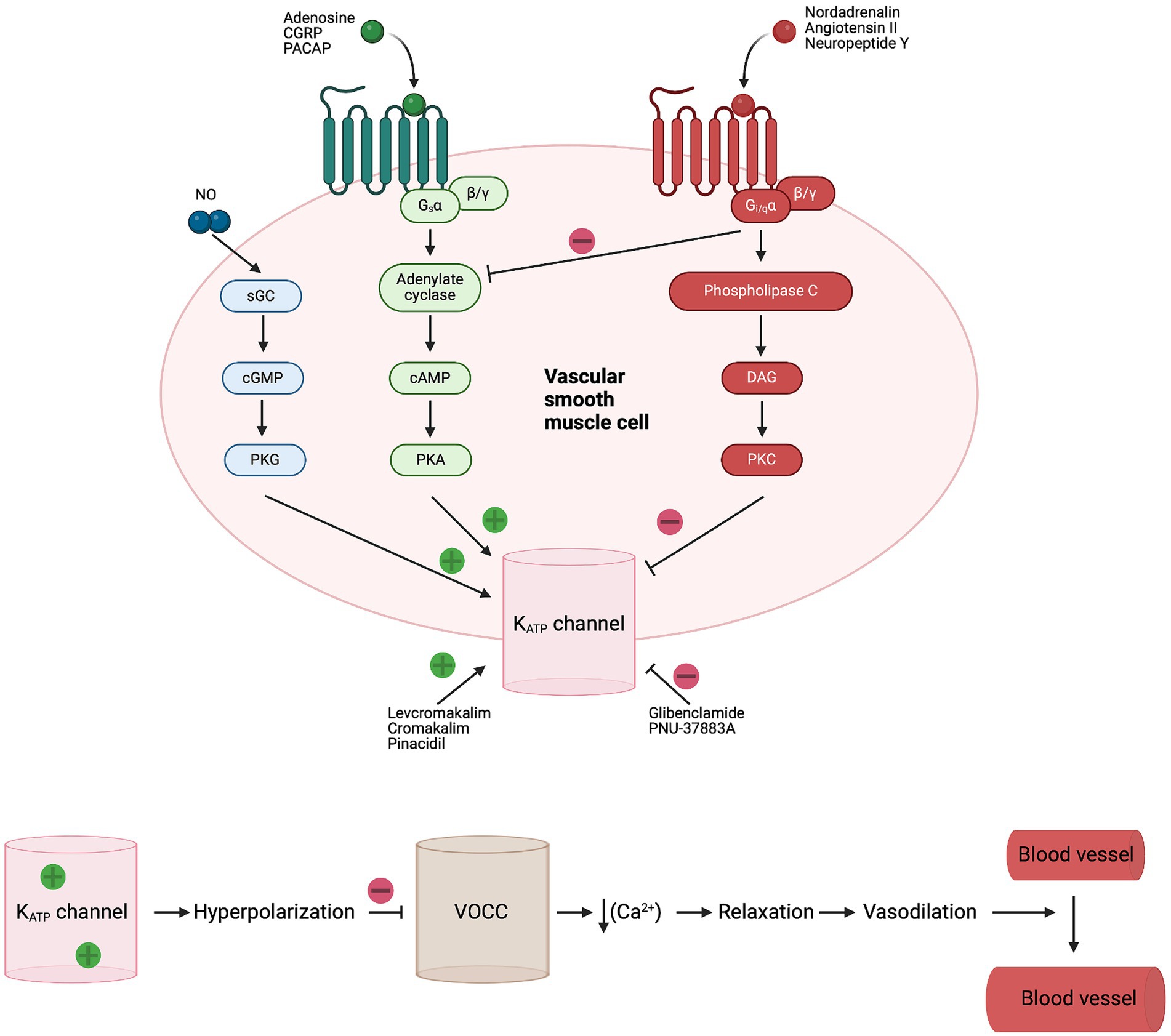
Figure 2. Signaling pathway and opening of KATP channels in vascular SMC. Numerous endogenous vasodilators activate KATP channels in SMC through adenylate cyclase and PKA phosphorylation. While endogenous vasoconstrictors inhibit KATP channels in SMC through DAG and PKC phosphorylation. Activation of KATP leads to hyperpolarization and closing of voltage-operated Ca2+ channels (VOCC), followed by relaxation of SMC and increased blood flow (17). CGRP, calcitonin gene-related peptide; DAG, diacylglycerol; Gs, G-protein-coupled receptor alpha s; Gi/q, G-protein-coupled receptor alpha i/q; sGC, soluble guanylate cyclase; KATP, ATP-sensitive potassium; NO, nitric oxide; PACAP, pituitary adenylate cyclase activating polypeptide; PKA, protein kinase A; PKC, protein kinase C; PKG, protein kinase G; SMC, smooth muscle cell; VOCC, voltage-operated Ca2+ channels.
The intricate mechanisms underpinning the involvement of KATP channels in the regulation of cerebral hemodynamics have not been systematically reviewed. Here, we systemically review preclinical and clinical studies addressing the expression of KATP channel in the cerebral vasculature, and their involvement in CBF regulation and cerebral vasodilation.
Methods
We searched PubMed and EMBASE for articles assessing the role of KATP channel in the cerebral vasculature. The search was conducted on 29 January 2024, and the search string was (“KATP channels” [MeSH Terms] OR “KATP channel” [All Fields] OR “ATP sensitive potassium channel” [All Fields] OR “KATP channel expression” [All Fields] OR “KATP channel knockout” [All Fields] OR “ATP sensitive potassium channel expression” [All Fields] OR “ATP sensitive potassium channel knockout” [All Fields] AND “cerebral blood flow” [MeSH Terms] OR “cerebral blood flow” [All Fields] OR “brain blood flow” [All Fields] OR “blood flow, brain” [All Fields] OR “cerebral circulation” [All Fields] OR “cerebral circulations” [All Fields] OR “flow, brain blood” [All Fields] OR “circulation, cerebrovascular” [All Fields] OR “cerebrovascular circulation” [All Fields]).
Selection criteria and study inclusion
An a priori systematic review protocol was developed. The full protocol can be obtained from the corresponding author upon reasonable request. Two study protocols were registered in Prospero [ID-numbers: CRD42023339278 (preclinical data) and CRD42022339152 (clinical data)]. We followed the Preferred Reporting Items for Systematic Reviews and Meta-analyses (PRISMA) reporting guidelines and the recommendations from the Cochrane Collaboration (20). The population, intervention, comparison, outcome, and study design (PICOS) approach was chosen as follows: study design, sample characteristics of the sample, intervention, comparator and outcomes.
After removing duplicates, two investigators (HASD and LK) independently screened articles, first by title and abstract and then full text to confirm eligibility for this review. The references of the included studies were also screened. Any disagreements between the investigators were resolved through discussion. If the conflict remained, a third investigator (MMK) made the final decision. Studies were restricted to English language and both preclinical and clinical studies investigating KATP channel opener (KCO) or KATP channel inhibitor (KCI; Table 1) and their effects on CBF and the diameter of cerebal arteries were included. Reviews, meta-analysis, conference proceedings and case reports were excluded. For each included study, the following data were extracted: article information (title, authors, and journal), study design, characteristics of the sample intervention, technique, substances used, and outcomes. No formal meta-analysis was planned.
Results
The database search identified 294 citations of which 78 were duplicates. A total of 216 studies were screened by title and abstract and 91 were full text screened. Of these, 51 studies were included, 45 preclinical (35 studies in vivo, seven studies ex vivo, two studies in vivo and ex vivo and one study in vivo and in vitro) and six clinical studies (Figure 3). Preclinical and clinical data are summarized in Tables 2, 3, respectively.
Summary of preclinical studies
KATP channels are expressed in SMCs (50, 54), ECs (11, 52–54), and pericytes (11, 43, 51, 58, 62). In-vivo studies showed that KATP channel openers (KCOs) dilated pial arteries and pial arterioles measured using a video microscaler through a cranial window in cats (4), rats (35), and pigs/piglets (5, 22–25, 28, 31). The basilar artery was also dilated upon administration of KCOs in rats (44, 45). CBF measured by laser-Doppler flowmeter through a cranial window over the region supplied by the middle cerebral artery (MCA) was increased upon administration of KCOs in mice (46–48). Using patch-clamp electrophysiology, ex-vivo studies showed that application of KCOs led to hyperpolarization of pericytes in mice (11) and rats (58), which was inhibited by KATP channel inhibitor (KCI), glibenclamide. In rats, endothelium removal from cerebral arterioles resulted in decreased dilation in response to administration of KCOs (52) and reduced the vasoconstrictive effect of glibenclamide (53). The majority of preclinical studies showed that glibenclamide reduced the increase in CBF upon KCO administration without altering the baseline CBF nor the baseline vascular tone (11, 28, 29, 31, 34, 35, 40, 53, 54).
Summary of clinical studies
KCOs have been used in clinical trials for the treatment of angina pectoris, asthma and hypertension. The most common adverse event mentioned during treatment with KCOs was headache (3, 68, 69).
Clinical studies assessed the effect of KATP channels in cerebral hemodynamic in healthy participants and individuals with migraine using magnetic resonance (MR) angiography and transcranial Doppler. Intravenous infusion of KCO, levcromakalim increased CBF and dilated the MCA, the middle meningeal artery (MMA) and the superficial temporal artery (STA) (3, 6, 70). Glibenclamide did not affect the baseline diameter of intra- and extracerebral arteries (6). In contrast to preclinical studies, glibenclamide failed to attenuate the vasodilation induced by levcromakalim (6) or by other potent endogenous vasodilators including the calcitonin gene-related peptide (CGRP) (67, 71) and the pituitary adenylate cyclase-activating polypeptide (PACAP-38) (64).
Discussion
The aim of the present study is to systematically review the involvement of KATP channels in the cerebral vasculature and the contribution of these channels in cerebrovascular hemodynamics. The main findings are that KATP channels are expressed in cerebral vascular SMCs, ECs and pericytes and play a key role in the regulation of CBF across species (7–12, 72).
The KATP channel is a hetero-octameric complex consisting of four regulatory sulfonylurea receptor (SUR1, SUR2A or SUR2B) subunits and four pore-forming K+ inwardly rectifying (Kir6.1 or Kir6.2) subunits (73). Different compositions of KATP channel subunits lead to unique functions in distinct tissues (74, 75) (Table 4). KATP channels, depending on their different subunit composition, are expressed in vascular SMCs and neurons. Of note, in this systematic review, a frequently used KCO, levcromakalim, has a high affinity to the Kir6.1/SUR2B subunit in the vessels (76), while glibenclamide, a non-specific KCI, has a higher affinity to the Kir6.2/SUR1 subunit which is not present in vessels (77).
Expression of KATP channels
KATP channels are expressed in SMCs, ECs and pericytes. The latter are contractile cells found on the abluminal surface of the endothelial wall of capillaries (78). Two ex-vivo studies using patch-clamp electrophysiology to measure whole cell currents in brain pericytes showed that activation of KATP channels led to hyperpolarization of pericytes, and this effect was inhibited by glibenclamide (11, 58). KATP channels expressed in the endothelium of cerebral arteries might be a key component in the regulation of CBF. Endothelium removal of cerebral arterioles significantly affected the response to KATP channel modulators (52, 53). Endothelium produces numerous vasoactive mediators, including nitric oxide (NO) that influences CBF (10). Impaired endothelial function associated with hypertension (40), diabetes mellitus (35, 52), and aging (45, 46) reduced the impact of KCOs/KCIs. These findings indicate that KATP channel-induced vasodilation is endothelium-dependent. However, Janigro et al. (54) demonstrated that KCOs caused a pronounced vascular SMC-mediated and a lesser endothelium-dependent vasodilation in rats.
KATP channels and cerebral hemodynamics
Administration of synthetic KCOs (Table 1) increased the CBF measured through cranial window using a laser-Doppler flowmeter (11, 40, 44, 46, 48). Whereas, glibenclamide and other synthetic KCIs inhibited the effect induced by KCOs (40, 46, 48). The majority of the preclinical studies showed that glibenclamide did not affect the baseline CBF and the vascular tone measured by laser-Doppler flowmeter (11, 40) except one study which reported that glibenclamide injected in the cisterna magna lowered baseline CBF (38). CBF is dependent on cerebral perfusion pressure (CPP) and cerebrovascular resistance (CVR). The diameter of small arteries and pial arterioles contributes to CVR. In particular, dilation of pial arterioles might increase CBF while constriction of these vessels could decrease CBF (1).
KCOs dilated pial arteries (5, 22–25, 79), pial arterioles (4, 28, 31, 35, 61), the basilar artery (44, 45), and the MCA (50, 52). Here, glibenclamide and other synthetic KCIs reversed this dilation (4, 28, 31, 35, 43–45, 61). Glibenclamide did not affect the baseline diameter of these vessels in vivo (28, 29, 31, 34, 35) or ex vivo (53, 54). However, in one study, glibenclamide induced constriction of isolated MMAs in the absence of other vasoactive stimuli but did not alter the diameter of cerebral arteries (59).
Inhalation of anesthetics such as isoflurane/sevoflurane or hypoxia caused dilation of cerebral pial arterioles which was inhibited by glibenclamide (32). Adenosine induced dilation of cerebral arterioles in pigs (29) and hyperpolarized retinal pericytes in mice and rats (11, 58) and capillary ECs in mice (11), and administration of glibenclamide inhibited the effects of adenosine. CGRP in vivo and in vitro induced dilation of dural and pial arteries. Glibenclamide attenuated the effect of CGRP in vivo, but not in vitro (60). In healthy participants, glibenclamide had no effect on CGRP-induced headache (67).
Clinical studies demonstrated that levcromakalim dilated the MMA, the MCA and the STA in healthy humans (6) and individuals with migraine (3). In contrast to the preclinical studies, glibenclamide failed to attenuate the vascular changes induced by levcromakalim (6), PACAP-38 (64), CGRP (67) or hypercapnia (65). Of note, adenosine, CGRP and PACAP-38 are potent endogenous vasodilators which activate KATP channels indirectly through adenylate cyclase and protein kinase A phosphorylation (80–82). One study, however, reported that hypoxia increased the anterior circulation of the brain and this effect was attenuated by KATP channel blockage with glibenclamide (66). The lack of effect of glibenclamide in clinical studies could be attributed to differences in administration routes, metabolic rate and/or tissue expression of KATP channels across species. Basic mathematical modeling of pharmacokinetics and receptor potencies showed that the dose of glibenclamide used in clinical studies had receptor occupancy of 26% at the migraine relevant KATP channel subtype Kir6.1/SUR2B (83).
Limitations and future perspective
The major limitations for the preclinical studies are differences in methodological approaches including subjects, designs, concentrations and formulations of different types of KCOs and KCIs, potentially affecting the reported results (Table 2). Shortcomings of clinical trials assessing the hemodynamics role of KATP channel are (1) the use of low dose of glibenclamide, (2) including individuals from all age groups, and (3) not evaluating the long-term effect of KCOs or KCIs on cerebral hemodynamics and how endothelial dysfunction interferes with this effect. An additional question is whether KATP channels are involved in cerebral angiogenesis.
The KATP channel emerges to be a potential target for numerous pathological conditions such as migraine and ischemic stroke. Recent studies showed that KATP channel activation caused headache and migraine (3), indicating that KCIs might be a novel therapeutic approach for the treatment of headache and migraine. The fact that targeting KATP channels did not affect the baseline hemodynamic state, at least based on preclinical studies, is applicable to avoid serious adverse events. Activation of KATP channels increased CBF after cerebral ischemia in mice (51). More experiments are needed to reveal if KCOs have a clinically meaningful effect on cerebral hypoperfusion during ischemic stroke.
Other findings with direct clinical significance are that glibenclamide attenuated peripheral arterial dilation but failed to affect cerebral hemodynamics indicating an unique biochemical difference between KATP expressed in cerebral circulation and those expressed in peripheral arteries.
Several scenarios might underlie this difference, including expression of different SUR and Kir6 isoforms, different expression levels, post-translational modifications that render cerebral vascular KATP channels less sensitive to KCIs and/or existence of other cerebral regulatory mechanisms with higher impact. Western blotting and quantitative PCR could be used to compare the isoforms, expression within cerebral and peripheral arteries. Patch-clamp electrophysiology on isolated SMCs or ECs from the cerebral and peripheral arteries can assess the functional properties and thereby drug sensitivity.
These studies might allow a possible treatment avenue for individuals with hypertension without altering cerebral hemodynamics. Several clinical studies applied KCO to treat hypertension (68, 84–86). However, a common adverse event was headache, most likely due to changes in cephalic hemodynamics. Yet, more selective agonists are needed to avoid adverse events. The next step is the development of a selective KCO to avoid headache when treating hypertension. An agonist with high affinity to the Kir6.1 isoform of KATP channels could be an applicable candidate.
Conclusion
Preclinical and clinical data from this systematic review demonstrated that KATP channels are implicated in the regulation of cerebral hemodynamic. The main findings are that KATP channels are expressed in cerebral vascular SMCs, ECs and pericytes. KCO increased CBF and dilated cerebral arteries in both preclinical and clinical data. Glibenclamide did not change baseline CBF and cerebral diameter in preclinical studies and did not attenuate the vasodilation induced by KCOs in clinical studies.
Data availability statement
The original contributions presented in the study are included in the article/supplementary material, further inquiries can be directed to the corresponding author.
Author contributions
HASD: Writing – original draft, Writing – review & editing. LK: Writing – review & editing, Writing – original draft. MMK: Writing – original draft, Writing – review & editing.
Funding
The author(s) declare that no financial support was received for the research, authorship, and/or publication of this article.
Conflict of interest
The authors declare that the research was conducted in the absence of any commercial or financial relationships that could be construed as a potential conflict of interest.
Publisher’s note
All claims expressed in this article are solely those of the authors and do not necessarily represent those of their affiliated organizations, or those of the publisher, the editors and the reviewers. Any product that may be evaluated in this article, or claim that may be made by its manufacturer, is not guaranteed or endorsed by the publisher.
References
1. Fantini, S, Sassaroli, A, Tgavalekos, KT, and Kornbluth, J. Cerebral blood flow and autoregulation: current measurement techniques and prospects for noninvasive optical methods. Neurophotonics. (2016) 3:031411. doi: 10.1117/1.NPh.3.3.031411
2. Sun, HS, Xu, B, Chen, W, Xiao, A, Turlova, E, Alibraham, A, et al. Neuronal KATP channels mediate hypoxic preconditioning and reduce subsequent neonatal hypoxic-ischemic brain injury. Exp Neurol. (2015) 263:161–71. doi: 10.1016/j.expneurol.2014.10.003
3. Al-Karagholi, MAM, Hansen, JM, Guo, S, Olesen, J, and Ashina, M. Opening of ATP-sensitive potassium channels causes migraine attacks: a new target for the treatment of migraine. Brain. (2019) 142:2644–54. doi: 10.1093/brain/awz199
4. Wei, EP, Kontos, HA, and Beckman, JS. Mechanisms of cerebral vasodilation by superoxide, hydrogen peroxide, and peroxynitrite. Am Physiol Soc. (1996) 271:H1262–6. doi: 10.1152/ajpheart.1996.271.3.H1262
5. Armstead, WM, Riley, J, Cines, DB, and Higazi, AAR. TPA contributes to impairment of ATP and Ca sensitive K channel mediated cerebrovasodilation after hypoxia/ischemia through upregulation of ERK MAPK. Brain Res. (2011) 1376:88–93. doi: 10.1016/j.brainres.2010.12.052
6. Al-Karagholi, MAM, Ghanizada, H, Nielsen, CAW, Ansari, A, Gram, C, Younis, S, et al. Cerebrovascular effects of glibenclamide investigated using high-resolution magnetic resonance imaging in healthy volunteers. J Cereb Blood Flow Metab. (2021) 41:1328–37. doi: 10.1177/0271678X20959294
7. Gozalov, A, Petersen, KA, Mortensen, C, Jansen-Olesen, I, Klaerke, D, and Olesen, J. Role of KATP channels in the regulation of rat dura and pia artery diameter. Cephalalgia. (2005) 25:249–60. doi: 10.1111/j.1468-2982.2004.00848.x
8. Kitazono, T, Faraci, FM, Taguchi, H, and Heistad, DD. Role of potassium channels in cerebral blood vessels. Stroke. (1995) 26:1713–23. doi: 10.1161/01.STR.26.9.1713
9. Janigro, D, Alexander West, G, Gordon, EL, Richard Winn, H, and Richard, H. ATP-sensitive K+ channels in rat aorta and brain microvascular endothelial cells. Am Physiol Soc. (1993) 265:C812–21. doi: 10.1152/ajpcell.1993.265.3.C812
10. Faraci, FM, and Heistad, DD. Regulation of the cerebral circulation: role of endothelium and potassium channels. Physiol Rev. (1998) 78:53–97. doi: 10.1152/physrev.1998.78.1.53
11. Sancho, M, Klug, NR, Mughal, A, Koide, M, Huerta de la Cruz, S, Heppner, TJ, et al. Adenosine signaling activates ATP-sensitive K + channels in endothelial cells and pericytes in CNS capillaries. Sci Signal. (2022) 15:eabl5405. doi: 10.1126/scisignal.abl5405
12. Thomzig, A, Wenzel, M, Karschin, C, Eaton, MJ, Skatchkov, SN, Karschin, A, et al. Kir6.1 is the principal pore-forming subunit of astrocyte but not neuronal plasma membrane K-ATP channels. Mol Cell Neurosci. (2001) 18:671–90. doi: 10.1006/mcne.2001.1048
13. Bollmann, S, Mattern, H, Bernier, M, Robinson, SD, Park, D, Speck, O, et al. Imaging of the pial arterial vasculature of the human brain in vivo using high-resolution 7T time-of-flight angiography. eLife. (2022) 11:1186. doi: 10.7554/eLife.71186
14. Jones, EG. On the mode of entry of blood vessels into the cerebral cortex. J Anat. (1970) 106:507–20.
15. Cohen, Z. Serotonin in the regulation of brain microcirculation. Prog Neurobiol. (1996) 50:335–62. doi: 10.1016/S0301-0082(96)00033-0
16. Neil Granger, ED, Granger, J, Korthuis, R, Pittman, R, David Zawieja, L, Davis, M, et al. Colloquium series on integrated systems physiology: from molecule to function to disease forthcoming titles In: Granger N, Granger JP, editors. Capillary fluid exchange regulation of tissue oxygenation local control of blood flow regulation of arterial pressure. San Rafael (CA): Morgan & Claypool Life Sciences (2010)
17. Chrissobolis, S, and Sobey, CG. Inwardly rectifying potassium channels in the regulation of vascular tone four types of K + channels are functionally important in the vasculature-Ca 2+−activated K + (K Ca) channels, voltage-dependent K + (K V) channels, ATP-sensitive K + (K ATP) channels, and inwardly rectifying K +. Curr Drug Targets. (2003) 4:281–9. doi: 10.2174/1389450033491046
18. Nelson, MT, Patlak, JB, Worley, JF, and Standen, NB. Calcium channels, potassium channels, and voltage dependence of arterial smooth muscle tone. American physiological. Society. (1990) 259:C3–C18. doi: 10.1152/ajpcell.1990.259.1.C3
19. Edwards, G, and Weston, AH. THE PHARMACOLOGY OF ATP-SENSITIVE POTASSIUM CHANNELS [Internet]. Annu Rev Pharmacol Toxicol. (1993) 33:597–637. doi: 10.1146/annurev.pa.33.040193.003121
20. Liberati, A, Altman, DG, Tetzlaff, J, Mulrow, C, Gøtzsche, PC, Ioannidis, JPA, et al. The PRISMA statement for reporting systematic reviews and meta-analyses of studies that evaluate healthcare interventions: explanation and elaboration. BMJ. (2009) 339:b2700. doi: 10.1136/bmj.b2700
21. Ca, A, and Armstead, WM. G protein activation elicits cerebrovasodilation through interaction with K and K channels [internet]. Brain Res. (2002) 957:369–72. doi: 10.1016/S0006-8993(02)03698-3
22. Ann, M, and Armstead, WM. Age-dependent impairment of KAtp Channel function following brain injury [internet]. J Neurotrauma. (1999) 16:391–402. doi: 10.1089/neu.1999.16.391
23. Armstead, WM, and Hecker, JG. Heat shock protein modulation of K ATP and K Ca channel cerebrovasodilation after brain injury. Am J Physiol Heart Circ Physiol. (2005) 289:H1184–90. doi: 10.1152/ajpheart.00276.2005
24. Armstead, WM, Kiessling, JW, Riley, J, Kofke, WA, and Vavilala, MS. Phenylephrine infusion prevents impairment of ATP- and calcium-sensitive potassium channel-mediated cerebrovasodilation after brain injury in female, but aggravates impairment in male, piglets through modulation of ERK MAPK upregulation. J Neurotrauma. (2011) 28:105–11. doi: 10.1089/neu.2010.1581
25. Armstead, WM. Vasopressin induced cyclooxygenase dependent superoxide generation 1 contributes to K channel function impairment after brain injury [internet]. Brain Res. (2001) 910:19–28. doi: 10.1016/S0006-8993(01)02716-0
26. Pastor, P, Curvello, V, Hekierski, H, and Armstead, WM. Inhaled nitric oxide protects cerebral autoregulation through prevention of impairment of ATP and calcium sensitive K channel mediated cerebrovasodilation after traumatic brain injury. Brain Res. (2019) 1711:1–6. doi: 10.1016/j.brainres.2019.01.008
27. Wei, EP, and Kontos, HA. Blockade of ATP-sensitive potassium channels in cerebral arterioles inhibits vasoconstriction from Hypocapnic alkalosis in cats. Stroke. (1999) 30:851–4. doi: 10.1161/01.STR.30.4.851
28. Nnorom, CC, Davis, C, Fedinec, AL, Howell, K, Jaggar, JH, Parfenova, H, et al. Contributions of KATP and KCa channels to cerebral arteriolar dilation to hypercapnia in neonatal brain. Physiol Rep. (2014) 2:e12127. doi: 10.14814/phy2.12127
29. Bari, F, Louis, TM, and Busija, DW. Effects of ischemia on cerebral arteriolar dilation to arterial hypoxia in piglets. Stroke. (1998) 29:222–8. doi: 10.1161/01.STR.29.1.222
30. Patel, S, Fedinec, AL, Liu, J, Weiss, MA, Pourcyrous, M, Harsono, M, et al. H 2 S mediates the vasodilator effect of endothelin-1 in the cerebral circulation. Am J Physiol Heart Circ Physiol. (2018) 315:H1759–64. doi: 10.1152/ajpheart.00451.2018
31. tFerenc, B, Errico, RA, Louis, TM, and Busija, DW. Interaction between ATP-sensitive K+ channels and nitric oxide on Pial arterioles in piglets. J Cereb Blood Flow Metab. (1996) 16:1158–64. doi: 10.1097/00004647-199611000-00010
32. Lida, H. Isoflurane and sevoflurane induce vasodilation of cerebral vessels via ATP-sensitive K+ channel activation. Am Soc Anesthesiol. (1998) 89:954–60. doi: 10.1097/00000542-199810000-00020
33. Faraci, FM, and Heistad, DD. Responses of cerebral arterioles to N-methyl-D-aspartate and activation of ATP-sensitive potassium channels in old rats. Brain Res. (1994) 654:349–51. doi: 10.1016/0006-8993(94)90499-5
34. Parfenova, H, Liu, J, Hoover, DT, and Fedinec, AL. Vasodilator effects of sulforaphane in cerebral circulation: a critical role of endogenously produced hydrogen sulfide and arteriolar smooth muscle KATP and BK channels in the brain. J Cereb Blood Flow Metab. (2020) 40:1987–96. doi: 10.1177/0271678X19878284
35. Mayhan, WG, and Faraci, FM. Responses of cerebral arterioles in diabetic rats to activation of ATP-sensitive potassium channels. Am Physiol Soc. (1993) 265:H152–7. doi: 10.1152/ajpheart.1993.265.1.H152
36. Horinaka, N, Kuang, TY, Pak, H, Wang, R, Jehle, J, Kennedy, C, et al. Blockade of cerebral blood flow response to insulin-induced hypoglycemia by caffeine and glibenclamide in conscious rats. J Cereb Blood Flow Metab. (1997) 17:1309–18. doi: 10.1097/00004647-199712000-00006
37. Horinaka, N, Artz, N, Jehle, J, Takahashi, S, Kennedy, C, and Sokoloff, L. Examination of potential mechanisms in the enhancement of cerebral blood flow by hypoglycemia and pharmacological doses of Deoxyglucose. J Cereb Blood Flow Metab. (1997) 17:54–63. doi: 10.1097/00004647-199701000-00008
38. Takanori, E, Itoh, Y, Shimoji, K, Cook, M, Jehle, J, and Sokoloff, L. Blockade of KATP channels with glibenclamide does not alter functional activation of cerebral blood flow in the unanesthetized rat. Brain Res. (2002) 948:56–63. doi: 10.1016/s0006-8993(02)02948-7
39. Tomiyama, Y, Brian, JE, and Todd, MM. Cerebral blood flow during Hemodilution and hypoxia in rats role of ATP-sensitive potassium channels. Stroke. (1999) 30:1942–8. doi: 10.1161/01.STR.30.9.1942
40. Takaba, H, Nagao, T, Ibayashi, S, Kitazono, T, Fujii, K, and Fujishima, M. Altered cerebrovascular response to a potassium channel opener in hypertensive rats. Hypertension. (1996) 28:143–6. doi: 10.1161/01.HYP.28.1.143
41. Golanov, EV, and Reis, DJ. A role for KATP-channels in mediating the elevations of cerebral blood flow ATP and arterial pressure by hypoxic stimulation of oxygen-sensitive neurons of rostral ventrolateral medulla. Brain Res. (1999) 827:210–4. doi: 10.1016/S0006-8993(99)01256-1
42. Erdos, B, Lacza, Z, Toth, IE, Szelke, E, Mersich, T, Komjati, K, et al. Mechanisms of pain-induced local cerebral blood flow changes in the rat sensory cortex and thalamus. Brain Res. (2003) 960:219–27. doi: 10.1016/s0006-8993(02)03890-8
43. Andreas Zambach, S, Cai, C, Christian Cederberg Helms, H, Olav Hald, B, Dong, Y, Christoffer Fordsmann, J, et al. Precapillary sphincters and pericytes at first-order capillaries as key regulators for brain capillary perfusion. PNAS. (2021) 118:49118. doi: 10.1073/pnas.2023749118
44. Toyoda, K, Fujii, K, Ibayashi, S, and Kitazono, T. Role of ATP-sensitive potassium channels in brain stem circulation during hypotension. Am Physiol Soc. (1997) 273:H1342–6. doi: 10.1152/ajpheart.1997.273.3.H1342
45. Toyoda, K, Fujii, K, Takata, Y, Ibayashi, S, Kitazono, T, Nagao, T, et al. Age-related changes in response of brain stem vessels to opening of ATP-sensitive potassium channels. Stroke. (1997) 28:171–5. doi: 10.1161/01.STR.28.1.171
46. Liu, D, Ahmet, I, Griess, B, Tweedie, D, Greig, NH, and Mattson, MP. Age-related impairment of cerebral blood flow response to KATP channel opener in Alzheimer’s disease mice with presenilin-1 mutation. J Cereb Blood Flow Metab. (2021) 41:1579–91. doi: 10.1177/0271678X20964233
47. Liu, D, Pitta, M, Lee, JH, Ray, B, Lahiri, DK, Furukawa, K, et al. The KATP channel activator diazoxide ameliorates amyloid-β and tau pathologies and improves memory in the 3xTgAD mouse model of Alzheimer’s disease. J Alzheimers Dis. (2010) 22:443–57. doi: 10.3233/JAD-2010-101017
48. Kotoda, M, Ishiyama, T, Mitsui, K, Hishiyama, S, and Matsukawa, T. Nicorandil increased the cerebral blood flow via nitric oxide pathway and ATP-sensitive potassium channel opening in mice. J Anesth. (2018) 32:244–9. doi: 10.1007/s00540-018-2471-2
49. Takaba, H, Nagao, T, Yao, H, Kitazono, T, Kanari, KT, Ibayashi, S, et al. An ATP-sensitive potassium channel activator reduces infarct volume in focal cerebral ischemia in rats. Am Physiol Soc. (1997) 273:R583–6. doi: 10.1152/ajpregu.1997.273.2.R583
50. Son, NT, Richard Winn, H, and Janigro, D. ATP-sensitive potassium channels may participate in the coupling of neuronal activity and cerebrovascular tone. Am Physiol Soc. (2000) 278:H878–85. doi: 10.1152/ajpheart.2000.278.3.H878
51. Bing, GR, Feng, DY, Yin, Z, Yu, CZ, Yang, J, Ji, J, et al. Iptakalim improves cerebral microcirculation in mice after ischemic stroke by inhibiting pericyte contraction. Acta Pharmacol Sin. (2021) 43:1349–59. doi: 10.1038/s41401-021-00784-4
52. Zimmermann, PA, Knot, HJ, Stevenson, AS, and Nelson, MT. Increased myogenic tone and diminished responsiveness to ATP-sensitive K+ channel openers in cerebral arteries from diabetic rats. Circ Res. (1997) 81:996–1004. doi: 10.1161/01.RES.81.6.996
53. Horiuchi, T, Dietrich, HH, Hongo, K, Goto, T, and Dacey, RG. Role of endothelial nitric oxide and smooth muscle potassium channels in cerebral arteriolar dilation in response to acidosis. Stroke. (2002) 33:844–9. doi: 10.1161/hs0302.104112
54. Janigro, D, Nguyen, TS, Men, J, Alex West, G, Meno, J, and Richard, H. Endothelium-dependent regulation of cerebrovascular tone by extracellular and intracellular ATP. Am Physiol Soc. (1997) 273:H878–85. doi: 10.1152/ajpheart.1997.273.2.H878
55. Kinoshita, H. Lidocaine impairs vasodilation mediated by adenosine triphosphate-sensitive K+ channels but not by inward rectifier K+ channels in rat cerebral microvessels. Anesth Analg. (2004) 99:904–9. doi: 10.1213/01.ANE.0000133912.54318.0F
56. Nakahata, K, Kinoshita, H, Hirano, Y, Kimoto, Y, Iranami, H, and Hatano, Y. Mild hypercapnia induces vasodilation via adenosine triphosphate-sensitive K channels in parenchymal microvessels of the rat cerebral cortex [internet]. Anesthesiology. (2003) 99:1333–9. doi: 10.1097/00000542-200312000-00014
57. Movahed, P, Högestätt, ED, and Petersson, J. Effect of hypoxia on vasodilator responses to S-nitroso-N-acetylpenicillamine and levcromakalim in guinea pig basilar artery. Naunyn Schmiedeberg's Arch Pharmacol. (2003) 367:532–7. doi: 10.1007/s00210-003-0711-x
58. Li, Q, and Puro, DG. Adenosine activates ATP-sensitive K currents in pericytes of rat retinal microvessels: role of A1 and A2 receptors. Brain Res. (2001) 907:93–9. doi: 10.1016/S0006-8993(01)02607-5
59. Syed, AU, Koide, M, Brayden, JE, and Wellman, GC. Tonic regulation of middle meningeal artery diameter by ATP-sensitive potassium channels. J Cereb Blood Flow Metab. (2019) 39:670–9. doi: 10.1177/0271678X17749392
60. Gozalov, A, Jansen-Olesen, I, Klaerke, D, and Olesen, J. Role of KATP channels in cephalic vasodilatation induced by calcitonin gene-related peptide, nitric oxide, and transcranial electrical stimulation in the rat. Headache. (2008) 48:1202–13. doi: 10.1111/j.1526-4610.2008.01205.x
61. Taguchi, H, Heistad, DD, Kitazono, T, and Faraci, FM. ATP-sensitive K’ channels mediate dilatation of cerebral arterioles during hypoxia. Circ Res. (1994) 74:1005–8. doi: 10.1161/01.RES.74.5.1005
62. Hariharan, A, Robertson, CD, Garcia, DCG, and Longden, TA. Brain capillary Pericytes are metabolic sentinels that control blood flow through KATP Channel activity. Cell Rep. (2022) 41:111872. doi: 10.1016/j.celrep.2022.111872
63. Marc Simard, J, Geng, Z, Kyoon Woo, S, Ivanova, S, Tosun, C, Melnichenko, L, et al. Glibenclamide reduces inflammation, vasogenic edema, and caspase-3 activation after subarachnoid hemorrhage. J Cereb Blood Flow Metab. (2009) 29:317–30. doi: 10.1038/jcbfm.2008.120
64. Kokoti, L, Al-Mahdi Al-Karagholi, M, Elbahi, FA, Coskun, H, Ghanizada, H, Amin, FM, et al. Effect of KATP channel blocker glibenclamide on PACAP38-induced headache and hemodynamic. Cephalalgia. (2022) 42:846–58. doi: 10.1177/03331024221080574
65. Bayerle-eder, M, Wolzt, M, Polska, E, Langenberger, H, Pleiner, J, Teherani, D, et al. Hypercapnia-induced cerebral and ocular vasodilation is not altered by glibenclamide in humans [internet]. Am J Physiol Regul Integr Comp Physiol. (2000) 278:R1667–73. doi: 10.1152/ajpregu.2000.278.6.R1667
66. Rocha, MP, Campos, MO, Mattos, JD, Mansur, DE, Rocha, HNM, Secher, NH, et al. KATP channels modulate cerebral blood flow and oxygen delivery during isocapnic hypoxia in humans. J Physiol. (2020) 598:3343–56. doi: 10.1113/JP279751
67. Coskun, H, Elbahi, FA, Al-Karagholi, MAM, Ghanizada, H, Sheykhzade, M, and Ashina, M. The effect of KATP Channel blocker Glibenclamide on CGRP-induced headache and hemodynamic in healthy volunteers. Front Physiol. (2021) 12:652136. doi: 10.3389/fphys.2021.652136
68. Al-Karagholi, MAM, Hansen, JM, Severinsen, J, Jansen-Olesen, I, and Ashina, M. The KATP channel in migraine pathophysiology: a novel therapeutic target for migraine. J Headache Pain. (2017) 18:90. doi: 10.1186/s10194-017-0800-8
69. Al-Karagholi, MAM, Ghanizada, H, Nielsen, CAW, Hougaard, A, and Ashina, M. Opening of ATP sensitive potassium channels causes migraine attacks with aura. Brain. (2021) 144:2322–32. doi: 10.1093/brain/awab136
70. Al-Karagholi, MAM, Ghanizada, H, Hansen, JM, Skovgaard, LT, Olesen, J, Larsson, HBW, et al. Levcromakalim, an adenosine triphosphate-sensitive Potassium Channel opener, dilates Extracerebral but not cerebral arteries. Headache. (2019) 59:1468–80. doi: 10.1111/head.13634
71. Al-Karagholi, MAM, Kalatharan, V, Fagerberg, PS, and Amin, FM. The vascular role of CGRP: a systematic review of human studies. Front Neurol. (2023) 14:1204734. doi: 10.3389/fneur.2023.1204734
72. Ploug, KB, Edvinsson, L, Olesen, J, and Jansen-Olesen, I. Pharmacological and molecular comparison of KATP channels in rat basilar and middle cerebral arteries. Eur J Pharmacol. (2006) 553:254–62. doi: 10.1016/j.ejphar.2006.09.053
73. Kokoti, L, Al-Karagholi, MAM, and Ashina, M. Latest insights into the pathophysiology of migraine: the ATP-sensitive potassium channels. Curr Pain Headache Rep. (2020) 24:77. doi: 10.1007/s11916-020-00911-6
74. Clement Iv, JP, Kunjilwar, K, Gonzalez, G, Schwanstecher, M, Panten, U, Aguilar-Bryan, L, et al. Association and stoichiometry of K ATP Channel subunits confirming that these subunits comprise the. Neuron. (1997) 18:827–38. doi: 10.1016/S0896-6273(00)80321-9
75. Shyng, SL, and Nichols, CG. Octameric stoichiometry of the K ATP Channel complex [internet]. J Gen Physiol. (1997) 110:655–64. doi: 10.1085/jgp.110.6.655
76. Ploug, KB, Boni, LJ, Baun, M, Hay-Schmidt, A, Olesen, J, and Jansen-Olesen, I. KATP channel expression and pharmacological in vivo and in vitro studies of the KATP channel blocker PNU-37883A in rat middle meningeal arteries. Br J Pharmacol. (2008) 154:72–81. doi: 10.1038/bjp.2008.86
77. Rubaiy, HN. The therapeutic agents that target ATP-sensitive potassium channels. Acta Pharma. (2016) 66:23–34. doi: 10.1515/acph-2016-0006
78. Kelley, C, Damore, P, Hechtman, HB, and Shepro, D. Microvascular Pericyte contractility in vitro: comparison with other cells of the Vascular Wall. J Cell Biol. (1987) 104:483–90. doi: 10.1083/jcb.104.3.483
79. Luscher, TF, Vanhoutte, PM, and Rau, L. Antihypertensive treatment normalizes decreased endothelium-dependent relaxations in rats with salt-induced hypertension. Hypertension. (1987) 9:193. doi: 10.1161/01.HYP.9.6_Pt_2.III193
80. Kleppisch, T, and Nelson, MT. Adenosine activates ATP-sensitive potassium channels in arterial myocytes via A2 receptors and cAMP-dependent protein kinase (vasodilation/glibenclamide/hypoxia/P1 receptors). Physiology. (1995) 92:12441–5. doi: 10.1073/pnas.92.26.12441
81. Quayle, JM, Bonev, AD, Brayden, JE, and Nelson, MT. Calcitonin gene-related peptide activated ATP-sensitive K+ currents in rabbit arterial smooth muscle via protein kinase a. J Physiol. (1994) 475:9–13. doi: 10.1113/jphysiol.1994.sp020045
82. Bruch, L, Rubel, S, Kästner, A, Gellert, K, Gollasch, M, and Witt, C. Pituitary adenylate cyclase activating peptides relax human pulmonary arteries by opening of K ATP and K Ca channels. Thorax. (1998) 53:586–7. doi: 10.1136/thx.53.7.586
83. Christophersen, P, and Dyhring, T. The shortcoming of using glibenclamide in exploratory clinical headache provocation studies. Cephalalgia. (2023) 43:475. doi: 10.1177/03331024231219475
84. Muiesan, G, Fariello, R, Muiesan, ML, and Christensen, OE. European journal of clinical Pharmacology effect of Pinacidil on blood pressure, plasma Catecholamines and plasma renin activity in essential hypertension. Eur J Clin Pharmacol. (1985) 28:495–9. doi: 10.1007/BF00544057
85. Golberg, MR. Clinical Pharmacology of Pinacidil, a prototype for drugs that affect potassium channels. J Cardiovasc Pharmacol. (1988) 12:S41–7. doi: 10.1097/00005344-198812002-00008
86. Sterndorff, B, and Johansen, P. The antihypertensive effect of Pinacidil versus prazosin in mild to moderate hypertensive patients seen in general practice. Acta Med Scand. (1988) 224:329–36. doi: 10.1111/j.0954-6820.1988.tb19591.x
Glossary
Keywords: CBF, cerebral arteries, ATP-sensitive potassium channels, migraine, stroke
Citation: Daoud HAS, Kokoti L and Al-Karagholi MA-M (2024) KATP channels in cerebral hemodynamics: a systematic review of preclinical and clinical studies. Front. Neurol. 15:1417421. doi: 10.3389/fneur.2024.1417421
Edited by:
Caroline Ran, Karolinska Institutet (KI), SwedenReviewed by:
Cédric Gollion, Centre Hospitalier Universitaire de Toulouse, FranceShow-Ling Shyng, Oregon Health and Science University, United States
Copyright © 2024 Daoud, Kokoti and Al-Karagholi. This is an open-access article distributed under the terms of the Creative Commons Attribution License (CC BY). The use, distribution or reproduction in other forums is permitted, provided the original author(s) and the copyright owner(s) are credited and that the original publication in this journal is cited, in accordance with accepted academic practice. No use, distribution or reproduction is permitted which does not comply with these terms.
*Correspondence: Mohammad Al-Mahdi Al-Karagholi, bWFoZGkuYWxrYXJhZ2hvbGlAZ21haWwuY29t