- 1Department of Neurology, Brigham MS Center, Brigham and Women’s Hospital, Boston, MA, United States
- 2Harvard Medical School, Boston, MA, United States
The Neuromyelitis Optica Spectrum Disorders (NMOSD) constitute a spectrum of rare autoimmune diseases of the central nervous system characterized by episodes of transverse myelitis, optic neuritis, and other demyelinating attacks. Previously thought to be a subtype of multiple sclerosis, NMOSD is now known to be a distinct disease with unique pathophysiology, clinical course, and treatment options. Although there have been significant recent advances in the diagnosis and treatment of NMOSD, the field still lacks clinically validated biomarkers that can be used to stratify disease severity, monitor disease activity, and inform treatment decisions. Here we review many emerging NMOSD biomarkers including markers of cellular damage, neutrophil-to-lymphocyte ratio, complement, and cytokines, with a focus on how each biomarker can potentially be used for initial diagnosis, relapse surveillance, disability prediction, and treatment monitoring.
Introduction
Neuromyelitis Optica Spectrum Disorders occur in 1–10 per 100,000 people worldwide across ages and ethnicities, with females and people of Asian or African ancestry being most often affected (1). The vast majority of NMOSD patients have a relapsing disease course and accumulate disability over time due to incomplete recovery after recurrent attacks (2). Despite recent breakthroughs in treatment options for NMOSD, there are no established guidelines on whom to treat with immunosuppressive medications and for how long, as relapses can occur even after prolonged periods of remission (3). There is a critical need for clinically validated biomarkers that can be used to guide care and ultimately improve the lives of NMOSD patients.
Based on 2015 international consensus diagnostic criteria, patients can be diagnosed with NMOSD if they have a positive AQP4-IgG test, at least one core clinical characteristic (including optic neuritis, acute myelitis, area postrema syndrome, acute brainstem syndrome, or symptomatic cerebral or diencephalic MRI lesions), and reasonable exclusion of alternative diagnoses (4). Over 80% of NMOSD patients fulfill these criteria with positive AQP4-IgG serology (5). A smaller fraction of NMOSD patients have AQP4-IgG-negative disease, and some of these patients are instead found to have anti-MOG antibodies. For purposes of this review, we will discuss biomarkers of AQP4-IgG-seropositive and seronegative NMOSD excluding cases with positive anti-MOG serology, now known as MOG-associated disease (MOGAD).
An ideal disease biomarker is sensitive and specific, easily sampled via non-invasive techniques, and affordable to assay. Valuable roles for biomarkers include aiding in diagnostic accuracy, predicting relapse, monitoring treatment response, and assessing safety of treatment discontinuation. Though strongly involved in the pathophysiology of NMOSD, AQP4-IgG is not a useful biomarker for monitoring disease activity or treatment response. Laboratories employ different methods of detecting AQP4-IgG and though a live cell-based assay is the gold standard, sensitivity and specificity vary considerably across other assays, leading to difficulty in standardizing the interpretation of titer results (6). While some studies have shown a strong correlation between serum AQP4-IgG titer and disease activity (7, 8), others have failed to replicate these findings (9–11). Some patients relapse despite negative seroconversion (12), whereas others do not relapse despite very high AQP4-IgG titers (10). For this reason, monitoring of serum AQP4-IgG has not been adopted as a standard practice in NMOSD patient care, and the field must instead turn to other biomarkers.
Markers of cellular damage
Glial fibrillary acidic protein (GFAP) is an intermediate filament protein found in the cytoskeletons of astrocytes (13) and neurofilament light chain (NfL) is a scaffolding protein located primarily within neuronal axons (14). Since NMOSD is predominately an astrocytopathy with secondary neuronal damage, both GFAP and NfL are released into CSF due to cellular damage and can then be detected in serum, with serum levels tightly correlated to CSF levels (15). GFAP and NfL have emerged as promising biomarkers in many neurologic diseases including NMOSD.
GFAP and NfL as diagnostic biomarkers
Serum GFAP (sGFAP) and serum NfL (sNfL) are elevated at baseline in NMOSD compared to healthy controls, and sGFAP is higher in AQP4-IgG-positive NMOSD when compared to both multiple sclerosis (MS) and to a lesser extent MOGAD (15–17), implying that sGFAP, but not sNfL, may assist in distinguishing NMOSD from other autoimmune CNS diseases. Notably, sGFAP does not appear to be as elevated in AQP4-seronegative NMOSD (18, 19), suggesting that the subset of patients with clinical NMOSD who test negative for AQP4-IgG may have a unique disease pathology which does not involve a primary astrocytopathy. Conversely, sNfL is elevated in both seropositive and seronegative NMOSD along with other demyelinating conditions, supporting a common role for secondary neuronal damage in all of these diseases (19).
Although a majority of studies find sGFAP to be significantly higher in AQP4-IgG-positive NMOSD compared to MS, there is overlap in the concentrations reported, and therefore no validated numeric sGFAP cutoff has been universally established to support NMOSD diagnosis. The ratio of sGFAP/sNfL is especially promising for differentiating seropositive NMOSD from MOGAD and MS (15, 16, 20), with a sGFAP/sNfL quotient above 5.71 being 73% sensitive and 75.8% specific for AQP4-IgG-positive NMOSD compared to MS (15). Importantly, the timing of serum sampling relative to most recent relapse must be carefully considered when interpreting cellular damage biomarkers. Both sNfL and sGFAP can remain elevated in remitted NMOSD compared to healthy controls, but sGFAP declines after relapse more quickly and to a greater extent than sNfL, which can remain quite elevated in NMOSD patients for many months to years after relapse (15, 16, 21), suggesting ongoing secondary neuronal damage long after the acute attack.
GFAP and NfL as attack biomarkers
Another possible role for biomarkers of cellular damage is in monitoring for NMOSD attacks and distinguishing between true attacks vs. pseudo-attacks, or worsening symptoms in the absence of new MRI-visible disease activity, especially since a serum test would be timelier and more cost-efficient than repeated MRIs. In general, AQP4-IgG-positive NMOSD patients with higher baseline sGFAP have a shorter interval to the next attack compared to NMOSD patients with normal sGFAP (hazard ratio 3–11 within 0.5–2 years) (17, 22). The sGFAP level may then increase between 4-20x above an individual’s recent baseline at the time of an attack or within <1 week preceding an attack (15, 17, 21, 23, 24). Moreover, higher peak sGFAP levels correlate with attack severity, suggesting that more extensive astrocytic damage underlies more disabling disease (17). Beyond acute attacks, robust evidence has shown that higher sGFAP correlates with higher clinical disability scores in NMOSD (15, 16, 20, 22, 24), though it is unclear if this is due to ongoing immune-mediated astrocyte damage vs. chronic astrogliosis (25). Even in clinically stable patients, higher sGFAP correlates with retinal neuraxonal loss and worsening afferent visual function, suggesting a greater degree of subclinical chronic optic nerve damage (26).
While some studies have shown significant elevations in sNfL during NMOSD relapse compared to remission (24, 27), most evidence suggests that sNfL is not a useful biomarker for predicting relapse (15, 16, 22, 27). However, sNfL may be the strongest cellular damage biomarker for predicting accumulated disability (27). Other markers of CNS cellular damage including neuron-specific ubiquitin C-terminal hydrolase L1 (UCHL1), tau, neurofilament heavy chain (NfH), and astrocytic protein S100B have also been studied in NMOSD, though to a much lesser extent than GFAP and NfL. CSF S100B is elevated during acute attacks of NMOSD compared to MS and other neurologic diseases, is significantly higher in AQP4-IgG-positive NMOSD compared to seronegative disease, and correlates with Expanded Disability Status Scale (EDSS) during both attack and remission (28). CSF S100B also correlates with the number of spinal segments involved in acute myelitis associated with NMOSD, but is generally less predictive than CSF GFAP (29). Serum and CSF levels of S100B appear to be closely correlated (30), though insufficient studies have been conducted on serum S100B as a biomarker in NMOSD. CSF NfH is elevated in NMOSD compared to MS, but does not correlate with attack severity or disability (31). Serum levels of tau and UCHL1 are also elevated during attacks and are higher in AQP4-IgG-positive patients than in seronegative NMOSD (19), but do not predict relapse as well as sGFAP and do not predict disability as well as sNfL (27).
GFAP and NfL in treatment response
In addition to aiding in diagnosis and attack monitoring, markers of cellular damage have a potential role in assessing response to treatment. Treatment with anti-CD20 agent rituximab has been shown to either stabilize (20) or decrease sGFAP over time (24) when compared to other immunosuppressive agents. Similarly, treatment with anti-IL-6 agent tocilizumab leads to slightly decreased levels of sGFAP over a period of 12 months when compared to prednisolone (24), and treatment with anti-CD19 drug inebilizumab results in significantly decreased sGFAP levels compared to placebo in as little as 12 weeks (17). Interestingly, in those patients who did experience clinical attacks while on inebilizumab there was no concomitant increase in sGFAP (17). This may indicate that the symptoms experienced by those patients were in fact pseudo-relapses without new disease activity, or that the biomarker profile of attacks is altered by anti-CD19 therapy. Most studies also show a significant decline in sNfL with immunosuppressive treatments (24, 27, 32). Additional research is needed to define sGFAP cutoff values that stratify relapse risk in NMOSD patients and to better understand the utility of monitoring GFAP, NfL and other cellular injury biomarkers in patients on immunosuppressive therapies.
Neutrophil-to-lymphocyte ratio
Histopathologic samples of NMOSD lesions have abundant neutrophils, in contrast to MS lesions which have more macrophages and T-lymphocytes, and the CSF neutrophil count is elevated in approximately 60% of NMOSD patients during acute attacks (33). The neutrophil-to-lymphocyte ratio (NLR) in blood has emerged as a biomarker in numerous diseases including NMOSD (34). Though NLR is elevated in many inflammatory diseases, a small study including 15 MOGAD patients and 28 NMOSD patients showed that NLR > 2.86 is 75% sensitive and 86.7% specific for a diagnosis of NMOSD rather than MOGAD in patients who present with similar initial symptoms (35). Another study of 50 patients suggested that NLR >2.04 is 73% sensitive and 70.8% specific for diagnosing NMOSD over MS and MOGAD (36).
Results are mixed regarding the utility of NLR for stratifying NMOSD severity. One adult study found that at the time of hospitalization with first presentation of NMOSD, elevated NLR above 2.54 is an independent predictor of higher initial disability, though with a small odds ratio of 1.08 (37). NLR also predicts EDSS at 6 and 12 months post-attack in pediatric patients (38). Additionally, at the time of admission with first attack, NLR >2.38 may be up to 81.8% sensitive and 64.7% specific for upcoming relapse over an average follow-up time of 44 months, and NLR > 2.63 is up to 76.3% sensitive and 68% specific for poor functional recovery from the initial attack (39). Meanwhile, other studies have shown that while NLR is indeed elevated in NMOSD during both relapse and remission, it is not an independent predictor of outcome (40).
Larger studies will be necessary to confirm whether NLR is a reliable biomarker for distinguishing NMOSD from disease mimics and to determine if and when NLR rises prior to attacks. More work is also needed to determine how NLR is affected by treatments, apart from corticosteroids which cause increased NLR via neutrophil demargination. At present, data suggest that a very high NLR in a patient meeting diagnostic criteria for NMOSD may prompt consideration of aggressive treatment options for a presumably more severe form of the disease.
Complement
The activation of complement has an established role in NMOSD pathophysiology (41, 42) and provides a promising target for drug discovery, with C5 cleavage inhibitor eculizumab showing excellent clinical efficacy in preventing NMOSD relapse (43). Multiple studies have shown that serum levels of C3 and C4 are lower in NMOSD during acute attacks when compared to controls and patients with MOGAD (44, 45). However, in clinically stable patients C3 and C4 trends are inconsistent, with one study showing lower C4 but not C3 (46), another showing lower C3 but not C4 (47), and another showing no difference in C3 or C4 when comparing remitted NMOSD patients to controls (48). Notably, these studies were performed on small cohorts and inconsistent results may be attributable to differences in assay techniques, duration of remission, and disease-modifying therapies. In a cohort of mixed relapse and remission states serum C1-inhibitor and C5 were both elevated in NMOSD compared to controls (49), whereas in a separate cohort of remitted patients there was no difference between NMOSD and controls in either of these biomarkers (47). Data on complement activation products are similarly inconsistent, with varying reports suggesting that serum sC5b-9 may be higher during relapse (50, 51), equivalent during relapse (52), or lower during remission (47) when compared to controls. In CSF samples sC5b-9, C5a, C10-inhibitor, and C1q are all elevated in NMOSD patients, though not all complement biomarkers can differentiate NMOSD from MS (53–55).
Multiple reports have shown correlations between components of the complement pathway and NMOSD disease severity. Higher serum C3a predicts a higher EDSS for a given attack, with elevated levels of C3a and elevated C3a:C3 ratio in patients who have relapsed within the past 6 months (50), despite other studies showing overall low C3 during active disease. Serum sC5b-9 is also significantly elevated during relapse compared to remission (52), and CSF sC5b-9 correlates with higher EDSS (54). Though CSF C5a is not significantly different between relapsing NMOSD and relapsing MS, in patients with NMOSD it does significantly correlate with the number of enhancing lesions seen on MRI (53).
To date only one small study of 3 NMOSD patients has examined the effect of treatment on complement, showing that eculizumab leads to a reduction in serum CH50 but no change in C3 or C4 (56). More studies with uniform metrics including remission vs. relapse states, CSF vs. serum testing, and treated vs. untreated patients will be needed in order to establish complement proteins as reliable disease activity biomarkers.
Cytokines, chemokines, and T-lymphocytes
Many cellular and humoral immune mediators are important in NMOSD pathogenesis and are now being studied as potential biomarkers. IL-6 plays several roles in NMOSD, including promoting the survival of plasmablasts that secrete AQP4-IgG, and has proven to be an important drug target with the success of both tocilizumab and satralizumab (57). IL-6 is significantly increased in the CSF of NMOSD patients during initial attack compared to controls (58, 59) and in relapse compared to remission (60), and can be used to distinguish NMOSD from MS (61). CSF IL-6 also correlates with EDSS (62). The role of serum IL-6 as a biomarker is less clear. Some studies have identified elevated serum IL-6 in relapsing NMOSD patients compared to remission and controls (63), while others have failed to show a significant difference between NMOSD and controls (59, 64). Other reports suggest that serum IL-6 significantly correlates with risk of relapse, disease activity and brain atrophy (63, 65). More work is needed to determine whether serum IL-6 can be used as a biomarker of disease activity and to characterize the effect of treatment on both serum and CSF IL-6.
Beyond IL-6, there is also evidence for elevation of other TH17-related cytokines in NMOSD CSF including IL-17A, IL-8, IL-13, TGF-β1, IL-10, BAFF, and APRIL (59, 60, 66, 67). Serum data is less consistent, with significant elevations in serum TGF-β1 and IL-10 in AQP4-IgG-positive NMOSD patients compared to other groups, but no elevation in serum IL-17A (59). In terms of chemokines, CXCL13 is elevated in both CSF (68) and serum (69) during NMOSD attacks, reliably distinguishes NMOSD from MS, and correlates with EDSS (68, 69). Meanwhile, CSF elevations in CXCL1, CXCL5, and CXCL7 can distinguish NMOSD from MS, but do not correlate with disease severity (70). Serum CXCL5 is lower in NMOSD patients than in controls, but does not predict severity nor differentiate from MS (71).
Though NMOSD was initially thought to be mediated primarily by B cells, a prominent role of T cells has emerged. Granzyme B-expressing CD8+ T cells are markedly elevated in blood of NMOSD patients both in relapse and recovery when compared to controls, and can also be used to predict response to immunotherapy, with higher proportions of granzyme B expression correlating with inadequate clinical response to azathioprine, mycophenolate, or rituximab (72). CD4 + CXCR5 + PD-1 + T follicular helper cells are also elevated during NMOSD relapse and predict disability (69). T cell subsets are an intriguing potential drug target and biomarker that merit attention in future research.
Other biomarkers
In addition to the large classes of biomarkers previously discussed, several other NMOSD biomarkers have been proposed. Adhesion molecules, which play a key role in breakdown of the blood–brain barrier leading to migration of peripheral immune cells and antibodies into CSF, are dysregulated in NMOSD. Specifically, elevated serum ICAM-2 and decreased serum PECAM-1 differentiate NMOSD from MS, and PECAM-1 negatively predicts EDSS (73). Neopterin, a nonspecific marker of cellular immune activation, is elevated in the CSF of patients with NMOSD compared to MS, and also predicts current relapse as opposed to remission with greater accuracy than other standard CSF tests including protein, cell count, and IgG index (74).
AQP4 is abundant in the medullary segment of renal collecting ducts, and though overt renal failure is uncommon in patients with NMOSD, markers of renal function may correlate with disease status. During acute attacks urine pH is significantly higher and urine specific gravity is lower in NMOSD compared to MS (75). Additionally, elevated GFR is an independent predictor of relapse in patients with AQP4-IgG-positive NMOSD (76). Due to ease of access, urine biomarkers of relapse and treatment response should be further investigated.
Conclusion
There are many promising biomarkers that have the potential to meaningfully improve the care of NMOSD patients throughout the course of the disease (Figure 1). Although many of the biomarker results discussed here are encouraging, much work remains to be done before these biomarkers will be widely accepted into clinical practice. Some biomarkers have been studied using older detection techniques including immunoblot and ELISA, whereas more recent studies have used newer technologies such as single-molecule arrays (SIMOA) (77), and it is not clear how results will translate across detection methods. Furthermore, as treatment options for NMOSD expand, studies will need to carefully consider the effects of different treatments on biomarker interpretation.
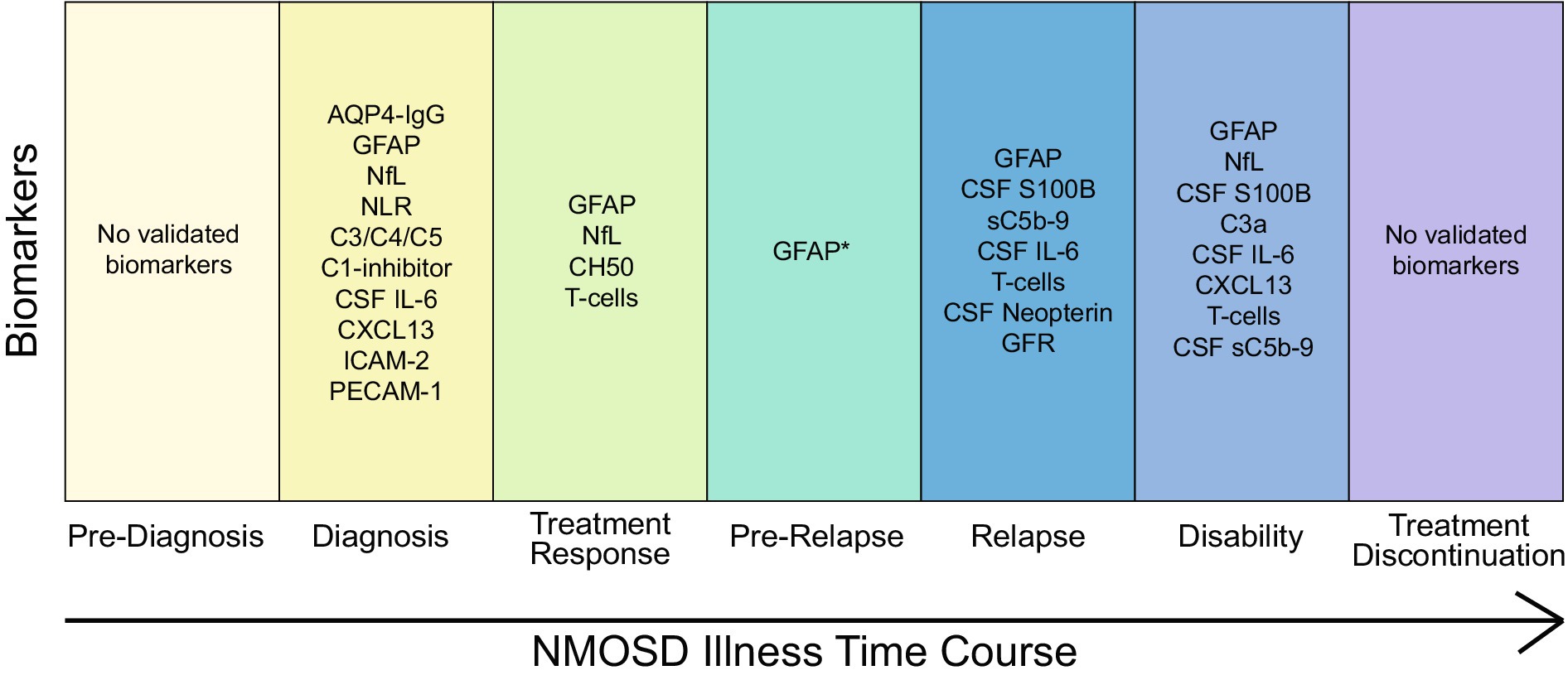
Figure 1. Summary of key NMOSD biomarkers and their clinical utility during various stages of disease. Except where indicated, all referenced biomarkers are from serum. *Relapse prediction for serum GFAP has only been shown within <1 week preceding relapse.
Nearly all statistically significant findings discussed in this review reflect comparisons between groups of patients, often with substantial overlap in numeric biomarker values. With the possible exception of sGFAP as a relapse biomarker when compared to an individual’s own baseline, no other biomarker is yet robust enough to be used at the individual patient level. One potential way to increase biomarker precision is by using z-scores. Particularly for NfL and GFAP, recent studies on other diseases have found that the use of z-scores, rather than raw biomarker values, can correct for variability within populations and enhance the determination of pathologic biomarker cutoff values (78, 79). Another possible way to increase the clinical applicability of NMOSD biomarkers could be via a combined disease severity or relapse risk score calculated from multiple biomarkers, which could have higher sensitivity and specificity compared to any single biomarker.
Author contributions
RR: Writing – review & editing, Writing – original draft. TC: Writing – review & editing, Writing – original draft.
Funding
The author(s) declare that no financial support was received for the research, authorship, and/or publication of this article.
Conflict of interest
TC has received compensation for consulting from Bristol Myers Squibb, Cabaletta Bio, Cycle Pharmaceuticals*, Genentech, Janssen, Merck KGaA, MJH Life Sciences, Novartis Pharmaceuticals AG, Novartis Pharmaceuticals KK, Octave Bioscience, F. Hoffmann-La Roche Ltd., Sanofi, Siemens*, and UCB Biopharma*; has received compensation for speaking engagements from Intellisphere, LLC* and Prime Education, LLC*; and has received research support from the BrightFocus Foundation, Bristol Myers Squibb, Genentech, EMD Serono, I-Mab Biopharma, Massachusetts Life Sciences Center, National Institutes of Health, National MS Society, Novartis Pharmaceuticals, Octave Bioscience, Sanofi Genzyme, Tiziana Therapeutics, US Department of Defense, and Wesley Clover International. All activities and funding have occurred within the past 24 months (*relationship has since ended) and disclosures do not conflict with the work being presented.
The remaining author declares that the research was conducted in the absence of any commercial or financial relationships that could be construed as a potential conflict of interest.
Publisher's note
All claims expressed in this article are solely those of the authors and do not necessarily represent those of their affiliated organizations, or those of the publisher, the editors and the reviewers. Any product that may be evaluated in this article, or claim that may be made by its manufacturer, is not guaranteed or endorsed by the publisher.
References
1. Papp, V, Magyari, M, Aktas, O, Berger, T, Broadley, SA, Cabre, P, et al. Worldwide incidence and prevalence of Neuromyelitis Optica: a systematic review. Neurology. (2021) 96:59–77. doi: 10.1212/WNL.0000000000011153
2. Duchow, A, Bellmann-Strobl, J, Friede, T, Aktas, O, Angstwurm, K, Ayzenberg, I, et al. Time to disability milestones and annualized relapse rates in NMOSD and MOGAD. Ann Neurol. (2023) 95:720–32. doi: 10.1002/ana.26858
3. Kim, SH, Jang, H, Park, NY, Kim, Y, Kim, SY, Lee, MY, et al. Discontinuation of immunosuppressive therapy in patients with Neuromyelitis Optica Spectrum disorder with Aquaporin-4 antibodies. Neurol Neuroimmunol Neuroinflamm. (2021) 8:e947. doi: 10.1212/NXI.0000000000000947
4. Wingerchuk, DM, Banwell, B, Bennett, JL, Cabre, P, Carroll, W, Chitnis, T, et al. International consensus diagnostic criteria for neuromyelitis optica spectrum disorders. Neurology. (2015) 85:177–89. doi: 10.1212/WNL.0000000000001729
5. Jarius, S, Paul, F, Weinshenker, BG, Levy, M, Kim, HJ, and Wildemann, B. Neuromyelitis optica. Nat Rev Dis Prim. (2020) 6:85. doi: 10.1038/s41572-020-0214-9
6. Waters, P, Reindl, M, Saiz, A, Schanda, K, Tuller, F, Kral, V, et al. Multicentre comparison of a diagnostic assay: aquaporin-4 antibodies in neuromyelitis optica. J Neurol Neurosurg Psychiatry. (2016) 87:1005–15. doi: 10.1136/jnnp-2015-312601
7. Takahashi, T, Fujihara, K, Nakashima, I, Misu, T, Miyazawa, I, Nakamura, M, et al. Anti-aquaporin-4 antibody is involved in the pathogenesis of NMO: a study on antibody titre. Brain. (2007) 130:1235–43. doi: 10.1093/brain/awm062
8. Jarius, S, Aboul-Enein, F, Waters, P, Kuenz, B, Hauser, A, Berger, T, et al. Antibody to aquaporin-4 in the long-term course of neuromyelitis optica. Brain. (2008) 131:3072–80. doi: 10.1093/brain/awn240
9. Kessler, RA, Mealy, MA, Jimenez-Arango, JA, Quan, C, Paul, F, López, R, et al. Anti-aquaporin-4 titer is not predictive of disease course in neuromyelitis optica spectrum disorder: a multicenter cohort study. Mult Scler Relat Disord. (2017) 17:198–201. doi: 10.1016/j.msard.2017.08.005
10. Jitprapaikulsan, J, Fryer, JP, Majed, M, Smith, CY, Jenkins, SM, Cabre, P, et al. Clinical utility of AQP4-IgG titers and measures of complement-mediated cell killing in NMOSD. Neurol Neuroimmunol Neuroinflamm. (2020) 7:e727. doi: 10.1212/NXI.0000000000000727
11. Akaishi, T, Takahashi, T, Nakashima, I, Abe, M, Ishii, T, Aoki, M, et al. Repeated follow-up of AQP4-IgG titer by cell-based assay in neuromyelitis optica spectrum disorders (NMOSD). J Neurol Sci. (2020) 410:116671. doi: 10.1016/j.jns.2020.116671
12. Zabala-Ángeles, I, García-Estrada, C, Gómez-Figueroa, E, Rodriguez-Rivas, R, Higuera-Gonzalez, E, Casallas-Vanegas, A, et al. Anti-aquaporin-4 positive-to-negative seroconversion in Latin American patients with neuromyelitis optica spectrum disorder treated with rituximab. Clin Exp Neuroim. (2021) 12:248–52. doi: 10.1111/cen3.12664
13. Kim, H, Lee, EJ, Lim, YM, and Kim, KK. Glial fibrillary acidic protein in blood as a disease biomarker of Neuromyelitis Optica Spectrum disorders. Front Neurol. (2022) 13:865730. doi: 10.3389/fneur.2022.865730
14. Gaetani, L, Blennow, K, Calabresi, P, Di Filippo, M, Parnetti, L, and Zetterberg, H. Neurofilament light chain as a biomarker in neurological disorders. J Neurol Neurosurg Psychiatry. (2019) 90:870–81. doi: 10.1136/jnnp-2018-320106
15. Watanabe, M, Nakamura, Y, Michalak, Z, Isobe, N, Barro, C, Leppert, D, et al. Serum Gfap and Neurofilament light as biomarkers of disease activity and disability in Nmosd. Neurology. (2019) 93:e1299–311. doi: 10.1212/WNL.0000000000008160
16. Chang, X, Huang, W, Wang, L, ZhangBao, J, Zhou, L, Lu, C, et al. Serum Neurofilament light and GFAP are associated with disease severity in inflammatory disorders with Aquaporin-4 or myelin oligodendrocyte glycoprotein antibodies. Front Immunol. (2021) 12:647618. doi: 10.3389/fimmu.2021.647618
17. Aktas, O, Smith, MA, Rees, WA, Bennett, JL, She, D, Katz, E, et al. Serum glial fibrillary acidic protein: a Neuromyelitis Optica Spectrum disorder biomarker. Ann Neurol. (2021) 89:895–910. doi: 10.1002/ana.26067
18. Hyun, JW, Kim, Y, Kim, KH, Kim, SH, Olesen, MN, Asgari, N, et al. CSF GFAP levels in double seronegative neuromyelitis optica spectrum disorder: no evidence of astrocyte damage. J Neuroinflammation. (2022) 19:86. doi: 10.1186/s12974-022-02450-w
19. Carta, S, Dinoto, A, Capobianco, M, Valentino, P, Montarolo, F, Sala, A, et al. Serum biomarker profiles discriminate AQP4 seropositive and double seronegative Neuromyelitis Optica Spectrum disorder. Neurol Neuroimmunol Neuroinflamm. (2024) 11:e200188. doi: 10.1212/NXI.0000000000200188
20. Liu, C, Lu, Y, Wang, J, Chang, Y, Wang, Y, Chen, C, et al. Serum neurofilament light chain and glial fibrillary acidic protein in AQP4-IgG-seropositive neuromyelitis optica spectrum disorders and multiple sclerosis: a cohort study. J Neurochem. (2021) 159:913–22. doi: 10.1111/jnc.15478
21. Hyun, JW, Kim, Y, Kim, SY, Lee, MY, Kim, SH, and Kim, HJ. Investigating the presence of Interattack astrocyte damage in Neuromyelitis Optica Spectrum disorder: longitudinal analysis of serum glial fibrillary acidic protein. Neurol Neuroimmunol Neuroinflamm. (2021) 8:e965. doi: 10.1212/NXI.0000000000000965
22. Schindler, P, Grittner, U, Oechtering, J, Leppert, D, Siebert, N, Duchow, AS, et al. Serum GFAP and NfL as disease severity and prognostic biomarkers in patients with aquaporin-4 antibody-positive neuromyelitis optica spectrum disorder. J Neuroinflammation. (2021) 18:105. doi: 10.1186/s12974-021-02138-7
23. Kim, H, Lee, EJ, Kim, S, Choi, LK, Kim, HJ, Kim, HW, et al. Longitudinal follow-up of serum biomarkers in patients with neuromyelitis optica spectrum disorder. Mult Scler. (2022) 28:512–21. doi: 10.1177/13524585211024978
24. Zhang, TX, Chen, JS, Du, C, Zeng, P, Zhang, H, Wang, X, et al. Longitudinal treatment responsiveness on plasma neurofilament light chain and glial fibrillary acidic protein levels in neuromyelitis optica spectrum disorder. Ther Adv Neurol Disord. (2021) 14:175628642110549. doi: 10.1177/17562864211054952
25. Schindler, P, Aktas, O, Ringelstein, M, Wildemann, B, Jarius, S, Paul, F, et al. Glial fibrillary acidic protein as a biomarker in neuromyelitis optica spectrum disorder: a current review. Expert Rev Clin Immunol. (2023) 19:71–91. doi: 10.1080/1744666X.2023.2148657
26. Lin, TY, Schindler, P, Grittner, U, Oertel, FC, Lu, A, Motamedi, S, et al. Serum glial fibrillary acidic protein correlates with retinal structural damage in aquaporin-4 antibody positive neuromyelitis optica spectrum disorder. Mult Scler Relat Disord. (2022) 67:104100. doi: 10.1016/j.msard.2022.104100
27. Aktas, O, Hartung, HP, Smith, MA, Rees, WA, Fujihara, K, Paul, F, et al. Serum neurofilament light chain levels at attack predict post-attack disability worsening and are mitigated by inebilizumab: analysis of four potential biomarkers in neuromyelitis optica spectrum disorder. J Neurol Neurosurg Psychiatry. (2023) 94:757–68. doi: 10.1136/jnnp-2022-330412
28. Wei, Y, Chang, H, Li, X, Du, L, Xu, W, Cong, H, et al. CSF-S100B is a potential candidate biomarker for Neuromyelitis Optica Spectrum disorders. Biomed Res Int. (2018) 2018:1–11. doi: 10.1155/2018/5381239
29. Takano, R, Misu, T, Takahashi, T, Sato, S, Fujihara, K, and Itoyama, Y. Astrocytic damage is far more severe than demyelination in NMO: a clinical CSF biomarker study. Neurology. (2010) 75:208–16. doi: 10.1212/WNL.0b013e3181e2414b
30. Fujii, C, Tokuda, T, Ishigami, N, Mizuno, T, and Nakagawa, M. Usefulness of serum S100B as a marker for the acute phase of aquaporin-4 autoimmune syndrome. Neurosci Lett. (2011) 494:86–8. doi: 10.1016/j.neulet.2011.02.063
31. Miyazawa, I, Nakashima, I, Petzold, A, Fujihara, K, Sato, S, and Itoyama, Y. High CSF neurofilament heavy chain levels in neuromyelitis optica. Neurology. (2007) 68:865–7. doi: 10.1212/01.wnl.0000256820.26489.17
32. Liu, C, Zhao, L, Fan, P, Ko, H, Au, C, Ng, A, et al. High serum neurofilament levels among Chinese patients with aquaporin-4-IgG-seropositive neuromyelitis optica spectrum disorders. J Clin Neurosci. (2021) 83:108–11. doi: 10.1016/j.jocn.2020.11.016
33. Jarius, S, Paul, F, Franciotta, D, Ruprecht, K, Ringelstein, M, Bergamaschi, R, et al. Cerebrospinal fluid findings in aquaporin-4 antibody positive neuromyelitis optica: results from 211 lumbar punctures. J Neurol Sci. (2011) 306:82–90. doi: 10.1016/j.jns.2011.03.038
34. Cabanillas-Lazo, M, Cruzalegui-Bazán, C, Pascual-Guevara, M, Quispe-Vicuña, C, Terry-Escalante, FA, Mori, N, et al. Clinical and imagenologic significance of the neutrophil-to-lymphocyte ratio in neuromyelitis optica spectrum disorder: a systematic review with meta-analysis. PLoS One. (2023) 18:e0281064. doi: 10.1371/journal.pone.0281064
35. Duan, Z, and Feng, J. Comparison of neutrophil-to-lymphocyte ratio between myelin oligodendrocyte glycoprotein antibody-associated disease and aquaporin-4 antibody-positive neuromyelitis optica spectrum disorders in adults. J Clin Neurosci. (2022) 101:89–93. doi: 10.1016/j.jocn.2022.05.002
36. Chen, B, Gui, MC, Ji, SQ, Xie, Y, Tian, DS, and Bu, BT. Distinct immunological features of inflammatory demyelinating diseases of the central nervous system. Neuroimmunomodulation. (2022) 29:220–30. doi: 10.1159/000519835
37. Zhou, Y, Xie, H, Zhao, Y, Zhang, J, Li, Y, Duan, R, et al. Neutrophil-to-lymphocyte ratio on admission is an independent risk factor for the severity of neurological impairment at disease onset in patients with a first episode of Neuromyelitis Optica Spectrum disorder. Neuropsychiatr Dis Treat. (2021) 17:1493–503. doi: 10.2147/NDT.S311942
38. Devlin, L, and Gombolay, G. The neutrophil-to-lymphocyte ratio and the monocyte-to-lymphocyte ratio predict expanded disability status scale score at one year in pediatric Neuromyelitis Optica Spectrum disorder but not in multiple sclerosis. Pediatr Neurol. (2023) 143:84–8. doi: 10.1016/j.pediatrneurol.2023.03.009
39. Xie, H, Zhao, Y, Pan, C, Zhang, J, Zhou, Y, Li, Y, et al. Association of neutrophil-to-lymphocyte ratio (NLR) with the prognosis of first attack neuromyelitis optica spectrum disorder (NMOSD): a retrospective cohort study. BMC Neurol. (2021) 21:389. doi: 10.1186/s12883-021-02432-0
40. Carnero Contentti, E, Delgado-García, G, Criniti, J, López, PA, Pettinicchi, JP, Cristiano, E, et al. An abnormally high neutrophil-to-lymphocyte ratio is not an independent outcome predictor in AQP4-IgG-positive NMOSD. Front Immunol. (2021) 12:628024. doi: 10.3389/fimmu.2021.628024
41. Asavapanumas, N, Tradtrantip, L, and Verkman, AS. Targeting the complement system in neuromyelitis optica spectrum disorder. Expert Opin Biol Ther. (2021) 21:1073–86. doi: 10.1080/14712598.2021.1884223
42. Lucchinetti, CF, Mandler, RN, McGavern, D, Bruck, W, Gleich, G, Ransohoff, RM, et al. A role for humoral mechanisms in the pathogenesis of Devic’s neuromyelitis optica. Brain. (2002) 125:1450–61. doi: 10.1093/brain/awf151
43. Pittock, SJ, Berthele, A, Fujihara, K, Kim, HJ, Levy, M, Palace, J, et al. Eculizumab in Aquaporin-4-positive Neuromyelitis Optica Spectrum disorder. N Engl J Med. (2019) 381:614–25. doi: 10.1056/NEJMoa1900866
44. Qin, C, Chen, B, Tao, R, Chen, M, Ma, X, Shang, K, et al. The clinical value of complement proteins in differentiating AQP4-IgG-positive from MOG-IgG-positive neuromyelitis optica spectrum disorders. Mult Scler Relat Disord. (2019) 35:1–4. doi: 10.1016/j.msard.2019.06.035
45. Lin, L, Wu, Y, Hang, H, Lu, J, and Ding, Y. Plasma complement 3 and complement 4 are promising biomarkers for distinguishing NMOSD from MOGAD and are associated with the blood-brain-barrier disruption in NMOSD. Front Immunol. (2022) 13:853891. doi: 10.3389/fimmu.2022.853891
46. Pache, F, Ringelstein, M, Aktas, O, Kleiter, I, Jarius, S, Siebert, N, et al. C3 and C4 complement levels in AQP4-IgG-positive NMOSD and in MOGAD. J Neuroimmunol. (2021) 360:577699. doi: 10.1016/j.jneuroim.2021.577699
47. Veszeli, N, Füst, G, Csuka, D, Trauninger, A, Bors, L, Rozsa, C, et al. A systematic analysis of the complement pathways in patients with neuromyelitis optica indicates alteration but no activation during remission. Mol Immunol. (2014) 57:200–9. doi: 10.1016/j.molimm.2013.09.010
48. Manin, A, Justo, ME, Leoni, J, Paz, ML, and Villa, AM. C5a complement levels in clinical remission AQP4-IgG-positive NMO patients. Acta Neurol Belg. (2023) 123:1447–51. doi: 10.1007/s13760-023-02261-7
49. Hakobyan, S, Luppe, S, Evans, DR, Harding, K, Loveless, S, Robertson, NP, et al. Plasma complement biomarkers distinguish multiple sclerosis and neuromyelitis optica spectrum disorder. Mult Scler. (2017) 23:946–55. doi: 10.1177/1352458516669002
50. Nytrova, P, Potlukova, E, Kemlink, D, Woodhall, M, Horakova, D, Waters, P, et al. Complement activation in patients with neuromyelitis optica. J Neuroimmunol. (2014) 274:185–91. doi: 10.1016/j.jneuroim.2014.07.001
51. Tüzün, E, Kürtüncü, M, Türkoğlu, R, Içöz, S, Pehlivan, M, Birişik, O, et al. Enhanced complement consumption in neuromyelitis optica and Behçet’s disease patients. J Neuroimmunol. (2011) 233:211–5. doi: 10.1016/j.jneuroim.2010.11.010
52. Miyamoto, K, Minamino, M, Kuwahara, M, Tsujimoto, H, Ohtani, K, Wakamiya, N, et al. Complement biomarkers reflect the pathological status of neuromyelitis optica spectrum disorders. Front Immunol. (2023) 14:1090548. doi: 10.3389/fimmu.2023.1090548
53. Kuroda, H, Fujihara, K, Takano, R, Takai, Y, Takahashi, T, Misu, T, et al. Increase of complement fragment C5a in cerebrospinal fluid during exacerbation of neuromyelitis optica. J Neuroimmunol. (2013) 254:178–82. doi: 10.1016/j.jneuroim.2012.09.002
54. Wang, H, Wang, K, Wang, C, Qiu, W, Lu, Z, and Hu, X. Increased soluble C5b-9 in CSF of neuromyelitis optica. Scand J Immunol. (2014) 79:127–30. doi: 10.1111/sji.12132
55. Zelek, WM, Fathalla, D, Morgan, A, Touchard, S, Loveless, S, Tallantyre, E, et al. Cerebrospinal fluid complement system biomarkers in demyelinating disease. Mult Scler. (2020) 26:1929–37. doi: 10.1177/1352458519887905
56. Namatame, C, Misu, T, Takai, Y, Nishiyama, S, Nakashima, I, Fujihara, K, et al. CH50 as a putative biomarker of eculizumab treatment in neuromyelitis optica spectrum disorder. Heliyon. (2021) 7:e05899. doi: 10.1016/j.heliyon.2021.e05899
57. Fujihara, K, Bennett, JL, de Seze, J, Haramura, M, Kleiter, I, Weinshenker, BG, et al. Interleukin-6 in neuromyelitis optica spectrum disorder pathophysiology. Neurol Neuroimmunol Neuroinflamm. (2020) 7:e841. doi: 10.1212/NXI.0000000000000841
58. Uzawa, A, Mori, M, Sawai, S, Masuda, S, Muto, M, Uchida, T, et al. Cerebrospinal fluid interleukin-6 and glial fibrillary acidic protein levels are increased during initial neuromyelitis optica attacks. Clin Chim Acta. (2013) 421:181–3. doi: 10.1016/j.cca.2013.03.020
59. Wei, Y, Chang, H, Li, X, Wang, H, Du, L, Zhou, H, et al. Cytokines and tissue damage biomarkers in first-onset Neuromyelitis Optica Spectrum disorders: significance of Interleukin-6. Neuroimmunomodulation. (2018) 25:215–24. doi: 10.1159/000494976
60. Wang, Y, Zhou, Y, Sun, X, Lu, T, Wei, L, Fang, L, et al. Cytokine and chemokine profiles in patients with Neuromyelitis Optica Spectrum disorder. Neuroimmunomodulation. (2016) 23:352–8. doi: 10.1159/000464135
61. Matsushita, T, Tateishi, T, Isobe, N, Yonekawa, T, Yamasaki, R, Matsuse, D, et al. Characteristic cerebrospinal fluid cytokine/chemokine profiles in neuromyelitis optica, relapsing remitting or primary progressive multiple sclerosis. PLoS One. (2013) 8:e61835. doi: 10.1371/journal.pone.0061835
62. Uzawa, A, Mori, M, Sato, Y, Masuda, S, and Kuwabara, S. CSF interleukin-6 level predicts recovery from neuromyelitis optica relapse. J Neurol Neurosurg Psychiatry. (2012) 83:339–40. doi: 10.1136/jnnp.2011.241760
63. Haramati, A, Rechtman, A, Zveik, O, Haham, N, Brill, L, and Vaknin-Dembinsky, A. IL-6 as a marker for NMOSD disease activity. J Neuroimmunol. (2022) 370:577925. doi: 10.1016/j.jneuroim.2022.577925
64. Hofer, LS, Mariotto, S, Wurth, S, Ferrari, S, Mancinelli, CR, Delogu, R, et al. Distinct serum and cerebrospinal fluid cytokine and chemokine profiles in autoantibody-associated demyelinating diseases. Mult Scler J Exp Transl Clin. (2019) 5:2055217319848463. doi: 10.1177/2055217319848463
65. Barros, PO, Cassano, T, Hygino, J, Ferreira, TB, Centurião, N, Kasahara, TM, et al. Prediction of disease severity in neuromyelitis optica by the levels of interleukin (IL)-6 produced during remission phase. Clin Exp Immunol. (2016) 183:480–9. doi: 10.1111/cei.12733
66. Uzawa, A, Mori, M, Arai, K, Sato, Y, Hayakawa, S, Masuda, S, et al. Cytokine and chemokine profiles in neuromyelitis optica: significance of interleukin-6. Mult Scler. (2010) 16:1443–52. doi: 10.1177/1352458510379247
67. Wang, H, Wang, K, Zhong, X, Qiu, W, Dai, Y, Wu, A, et al. Cerebrospinal fluid BAFF and APRIL levels in neuromyelitis optica and multiple sclerosis patients during relapse. J Clin Immunol. (2012) 32:1007–11. doi: 10.1007/s10875-012-9709-9
68. Zhong, X, Wang, H, Dai, Y, Wu, A, Bao, J, Xu, W, et al. Cerebrospinal fluid levels of CXCL13 are elevated in neuromyelitis optica. J Neuroimmunol. (2011) 240-241:104–8. doi: 10.1016/j.jneuroim.2011.10.001
69. Yang, X, Peng, J, Huang, X, Liu, P, Li, J, Pan, J, et al. Association of Circulating Follicular Helper T Cells and Serum CXCL13 with Neuromyelitis Optica Spectrum disorders. Front Immunol. (2021) 12:677190. doi: 10.3389/fimmu.2021.677190
70. Liu, Z, Chen, J, Wang, Z, Wang, Y, Zheng, D, Wang, H, et al. The CSF levels of neutrophil-related chemokines in patients with Neuromyelitis Optica. Ann Clin Transl Neurol. (2020) 7:1245–51. doi: 10.1002/acn3.51094
71. Karaaslan, Z, Yilmaz, V, Yuceer, H, Sanli, E, Akcay, HI, Kurtuncu, M, et al. Serum CXCL5 as a biomarker in multiple sclerosis and neuromyelitis optica spectrum disorder. North Clin Istanb. (2023) 10:341–4. doi: 10.14744/nci.2022.77861
72. Shi, Z, Du, Q, Wang, X, Wang, J, Chen, H, Lang, Y, et al. Granzyme B in circulating CD8+ T cells as a biomarker of immunotherapy effectiveness and disability in neuromyelitis optica spectrum disorders. Front Immunol. (2022) 13:1027158. doi: 10.3389/fimmu.2022.1027158
73. Chang, BL, Ro, LS, Chen, CM, Lo, YS, Lyu, RK, Kuo, HC, et al. Serum levels of cell adhesion molecules in patients with neuromyelitis optica spectrum disorder. Ann Clin Transl Neurol. (2020) 7:1854–61. doi: 10.1002/acn3.51167
74. Miyaue, N, Hosokawa, Y, Yamanishi, Y, Tada, S, Ando, R, and Nagai, M. Clinical use of CSF neopterin levels in CNS demyelinating diseases. J Neurol Sci. (2022) 441:120385. doi: 10.1016/j.jns.2022.120385
75. Chen, ZG, Huang, J, Fan, R, Weng, RH, Shinohara, RT, Landis, JR, et al. Urinalysis in patients with neuromyelitis optica spectrum disorder. Eur J Neurol. (2020) 27:619–25. doi: 10.1111/ene.14128
76. Chen, Y, Wang, Y, Jin, R, Lv, Z, Fu, Y, Teng, J, et al. Renal dysfunction in AQP4 NMOSD and MS; a potential predictor of relapse and prognosis. Clin Immunol. (2023) 259:109875. doi: 10.1016/j.clim.2023.109875
77. Rissin, DM, Kan, CW, Campbell, TG, Howes, SC, Fournier, DR, Song, L, et al. Single-molecule enzyme-linked immunosorbent assay detects serum proteins at subfemtomolar concentrations. Nat Biotechnol. (2010) 28:595–9. doi: 10.1038/nbt.1641
78. Benkert, P, Meier, S, Schaedelin, S, Manouchehrinia, A, Yaldizli, Ö, Maceski, A, et al. Serum neurofilament light chain for individual prognostication of disease activity in people with multiple sclerosis: a retrospective modelling and validation study. Lancet Neurol. (2022) 21:246–57. doi: 10.1016/S1474-4422(22)00009-6
Keywords: NMO, neurofilament, glial fibrillary acidic protein, neutrophil, lymphocyte
Citation: Rodin RE and Chitnis T (2024) Soluble biomarkers for Neuromyelitis Optica Spectrum Disorders: a mini review. Front. Neurol. 15:1415535. doi: 10.3389/fneur.2024.1415535
Edited by:
Ingo Kleiter, Marianne-Strauss-Klinik, GermanyReviewed by:
Klemens Ruprecht, Charité University Medicine Berlin, GermanyElias S. Sotirchos, Johns Hopkins University, United States
Copyright © 2024 Rodin and Chitnis. This is an open-access article distributed under the terms of the Creative Commons Attribution License (CC BY). The use, distribution or reproduction in other forums is permitted, provided the original author(s) and the copyright owner(s) are credited and that the original publication in this journal is cited, in accordance with accepted academic practice. No use, distribution or reproduction is permitted which does not comply with these terms.
*Correspondence: Tanuja Chitnis, dGNoaXRuaXNAYndoLmhhcnZhcmQuZWR1