- 1Department of Neurology and Experimental Neurology, Charité-Universitätsmedizin Berlin, Corporate Member of Freie Universität Berlin and Humboldt-Universität zu Berlin, Berlin, Germany
- 2German Center for Neurodegenerative Diseases (DZNE) Berlin, Berlin, Germany
- 3Institute of Integrative Neuroanatomy Berlin, Charité-Universitätsmedizin Berlin, Corporate Member of Freie Universiät Berlin and Humboldt-Universität zu Berlin, Berlin, Germany
- 4Clinical Immunological Laboratory Prof. Stöcker, Groß Grönau, Germany
Autoantibodies against proteins in the brain are increasingly considered as a potential cause of cognitive decline, not only in subacute autoimmune encephalopathies but also in slowly progressing impairment of memory in patients with classical neurodegenerative dementias. In this retrospective cohort study of 161 well-characterized patients with different forms of dementia and 34 controls, we determined the prevalence of immunoglobulin (Ig) G and IgA autoantibodies to brain proteins using unbiased immunofluorescence staining of unfixed murine brain sections. Autoantibodies were detected in 21.1% of dementia patients and in 2.9% of gender-matched controls, with higher frequencies in vascular dementia (42%), Alzheimer’s disease (30%), dementia of unknown cause (25%), and subjective cognitive impairment (16.7%). Underlying antigens involved glial fibrillary acidic protein (GFAP), glycine receptor, and Rho GTPase activating protein 26 (ARHGAP26), but also a range of yet undetermined epitopes on neurons, myelinated fiber tracts, choroid plexus, glial cells, and blood vessels. Antibody-positive patients were younger than antibody-negative patients but did not differ in the extent of cognitive impairment, epidemiological and clinical factors, or comorbidities. Further research is needed to understand the potential contribution to disease progression and symptomatology, and to determine the antigenic targets of dementia-associated autoantibodies.
1 Introduction
Detection of anti-neuronal autoantibodies in clinical neurology has markedly changed routine assessment of patients with subacute neuropsychiatric abnormalities in the context of autoimmune encephalopathies. Lately, autoantibody diagnostics has been expanded to more chronic, slowly progressing changes of cognition, mood and behavior—where it allowed the recognition of treatment-responsive clinical entities previously thought to be classical neurodegenerative diseases (1).
For several of these autoantibodies, the direct pathogenicity has already been proven, such as for antibodies targeting the N-methyl-D-aspartate (NMDA)-receptor, ℽ-aminobutyric acid A (GABAA) and GABAB receptors, α-amino-3-hydroxy-5-methyl-4-isoxazolepropionic acid (AMPA) receptor, Contactin-associated protein-like 2 (Caspr2) or leucine rich, glioma activated-1 (LGI1) (2). While many of them cause a broader clinical phenotype including epileptic seizures, psychosis or movement disorders, cognitive impairment and amnesia are common features and can predominate, which then overlaps with the clinical presentation of neurodegenerative dementias (3, 4). For example, patients with LGI1 autoantibodies can present to memory clinics with anterograde amnesia and behavioral abnormalities suggestive of Alzheimer’s disease (AD) or frontotemporal dementia (FTD) (5, 6). These patients often have post-inflammatory rapidly progressing atrophy in the mesiotemporal lobes (7), the predominant area of neuronal loss also in AD.
Another example is autoantibodies against the neuronal cell adhesion molecule Ig family containing LAMP, OBCAM, and NTM 5 (IgLON5), which causes a subacute encephalopathy with behavioral abnormalities and sleep disorder, but also cognitive impairment in up to 40% of affected patients (8, 9). Patients can have depositions of hyperphosphorylated Tau protein in hippocampus and brainstem, indicative of a primary neurodegenerative disease (tauopathy) (10). As clinical symptoms can respond to immunotherapy, the autoantibodies may be directly involved in the initiation or propagation of neurodegenerative processes, which is currently under intensive investigation.
The list of potential “dementia autoantibodies” is continuously growing and further contains autoantibodies against GFAP (11, 12), alpha1-adrenergic receptors (13), NMDA receptors (14–17), and multiple neuronal antigens in cancer patients with cognitive impairment (18). Initiation of immunotherapy in patients with LGI1 and IgLON5 autoantibodies can partially reverse the cognitive impairment, underscoring both, the need for and the potential of early autoantibody diagnostics in presumed neurodegenerative diseases. To further understand the role of such autoantibodies in dementia, detailed analyses of the frequencies, titers, kinetics, and pathogenicity of the antibodies are needed. Here, we systematically searched for established and novel anti-neuronal autoantibodies in cerebrospinal fluid (CSF) and serum of patients with different types of dementia using indirect immunofluorescence on unfixed murine brain sections.
2 Methods
2.1 Study population
For anti-neuronal autoantibody testing, 195 patients with different forms of dementia and controls were recruited for this study from the memory clinic of the Department of Neurology at Charité Berlin, Campus Mitte (Figure 1). Patients were diagnosed according to current clinical guidelines (19) and assigned to one of the following diagnostic groups: (1) AD, (2) mild cognitive impairment due to AD (MCI), (3) FTD, (4) other [including Lewy body dementia (LBD), mixed dementia, and cerebral amyloid angiopathy], (5) vascular dementia, (6) cognitive impairment of unknown cause, and (7) subjective cognitive impairment (SCI). Patients with cognitive impairment due to depression or with neurological autoimmune disease, e.g., multiple sclerosis, were excluded. The control group consisted of 34 patients (Table 1) without neurodegenerative disease, including patients with depression and other psychiatric disorders (n = 19), patients with concerns of memory impairment but excluded neurodegenerative disease (n = 8), headache (n = 4), syncope (n = 1), minor stroke (n = 1), and paresthesia (n = 1).
Testing for an autoantibody panel using cell-based assays was available for the serum of 71 patients and the CSF of 36 patients. Serum from 189 and CSF from 34 patients was available for indirect immunofluorescence staining on murine unfixed brain sections. Serum and CSF were stored at −80°C until further use. Samples were pseudonymized and handled blinded to the status of the patients during the assessment and evaluation described below. The study was approved by the Charité Universitätsmedizin Berlin Institutional Review Board (Berlin, Germany, #EA1/258/18).
2.2 Tissue reactivity screening (indirect immunofluorescence)
To determine the prevalence of IgG and IgA isotype autoantibodies, serum and CSF were screened for tissue reactivity on 20 μm cryostat-cut unfixed sagittal mouse brain sections (C57BL/6 mice) as previously described (20–23). Briefly, sections were blocked for 1 h at room temperature in blocking solution [phosphate-buffered saline (PBS), pH 7.4, supplemented with 2% bovine serum albumin and 5% normal goat serum]. Serum (200 μL, diluted 1:400 in blocking solution) or CSF (200 μL, undiluted) were applied to the brain sections for 16 h at 4°C and washed with PBS. Bound antibodies were detected with goat anti-human IgG [Alexa Fluor®488 AffiniPure Goat Anti-Human IgG (H + L), Jackson ImmunoResearch, #109-545-003, dilution 1:1,000] or goat anti-human IgA (Fluorescein AffiniPure Goat Anti-Human IgA, Jackson ImmunoResearch #109-095-011, dilution 1:200). After 2 h of incubation at room temperature, sections were rinsed again and mounted with Immumount (Shandon, #9990402). Images were taken with fluorescence microscopes (Olympus CKX41, Leica DMI8/SPE, Nikon Scanning Confocal A1Rsi+).
Cerebrospinal fluid samples were additionally screened for established autoantibodies using commercial panel tests (Euroimmun AG, Lübeck, Germany), including Hu, Ri, anti-neuronal nuclear antibodies 3 (ANNA3), Yo, Anti-Tr/anti-Delta/Notch-like epidermal growth factor-related receptor (Tr/DNER), myelin, Ma/Ta, glutamate decarboxylase 65 (GAD65), amphiphysin, glutamate receptor type AMPA, GABAB receptor, LGI1, Caspr2, zinc finger protein 4 (ZIC4), dipeptidyl aminopeptidase-like protein (DPPX), carbonic anhydrase related protein VIII (CARPVIII), glycine receptor (GlyR), metabotropic glutamate receptor 1 and 5 (mGluR1, mGluR5), GABAA receptor, ARHGAP26, inositol 1,4,5-trisphosphate receptor type 1 (ITPR1), homer3, potassium voltage-gated channel subfamily A member 2 (KCNA2), myelin oligodendrocyte glycoprotein (MOG), recoverin, neurochondrin, glutamate receptor δ2 (GluRD2), flotillin-1/2, and IgLON5.
2.3 Evaluation criteria
Indirect immunofluorescence sections were evaluated according to a modified semi-quantitative fluorescence score ranging from 0 to 3, as previously described (24). “0” defined the absence of any fluorescence signal, “1” the faint “background” intensity commonly seen with serum of healthy controls, “2” a clearly visible fluorescence patterns with reproducible anatomical distribution, and “3” a high-intensity fluorescent staining as in positive controls. Consistent intensities ≥2 in repeated experiments were considered positive staining.
The frequently observed staining of neuronal nuclei often corresponded to established anti-nuclear antibodies (ANA, e.g., finely speckled nuclear ANA) (25) and was considered positive only if it was detected with an intensity of ≥2 in CSF. Co-staining with an anti-GFAP antibody (NeuroMab, Cat# 75–240, RRID:AB 10672299; dilution 1:1,000) was performed when a glia-like pattern was observed.
2.4 Statistical analysis and figures
Statistics were conducted in Microsoft Excel (RRID: SCR_016137) including XLSTAT (RRID: SCR_016299). Statistical significance was assumed when p < 0.05. Differences in the prevalence of antibody-positive patients in the dementia cohort compared to controls were calculated using the Chi square test. Clinical parameters, including CSF markers, age and mini-mental state examination (MMSE) were compared using t-test, assuming unequal variances (Welch’s test). ANOVA was used for subgroup analysis. Figure 2 was created in GraphPad Prism (RRID: SCR_002798).
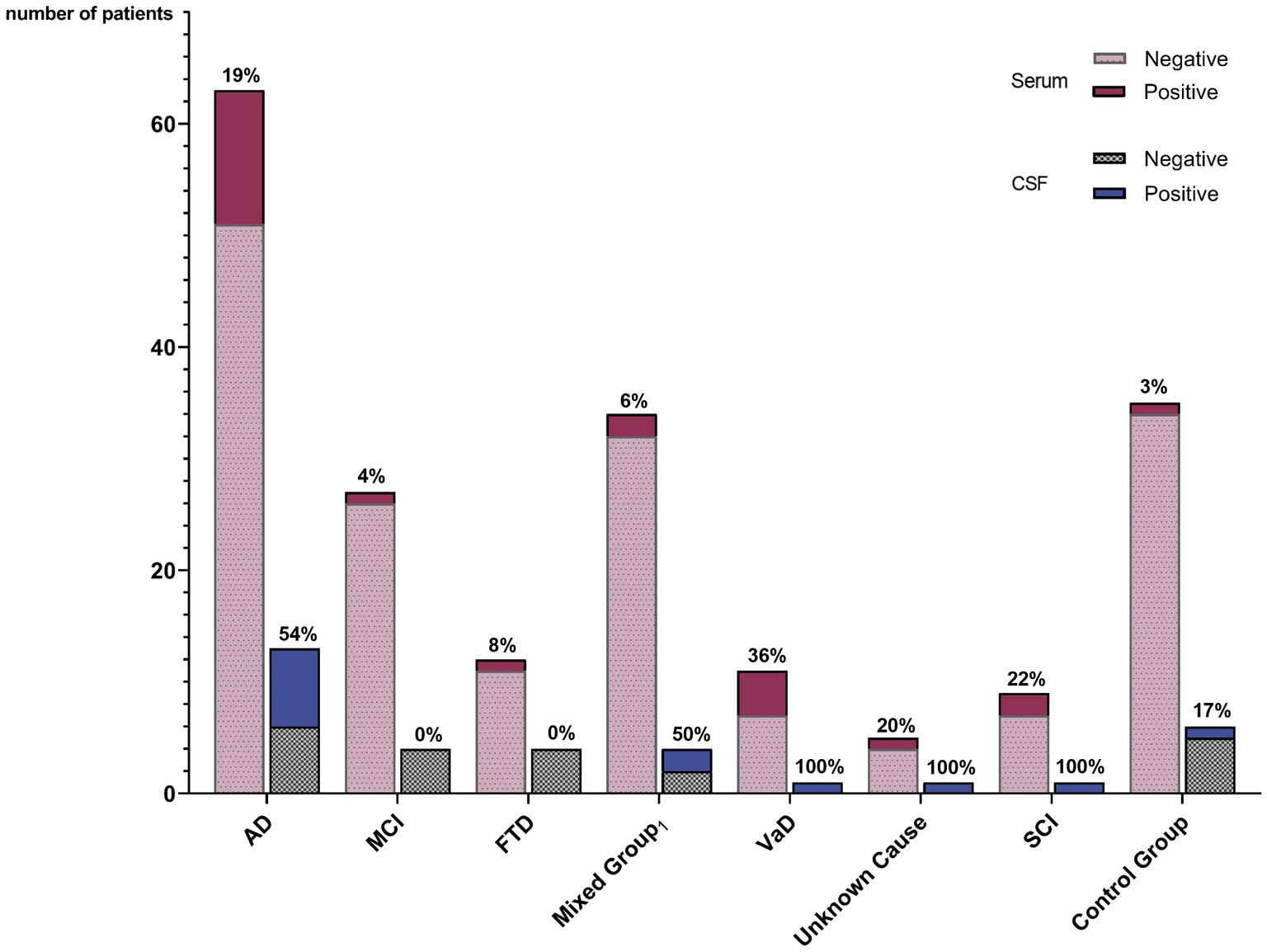
Figure 2. Prevalence of autoantibody-positive patients among different diagnostic groups. The percentage of positive patients within the diagnostic group is given for serum and for CSF, respectively. Lewy body dementia, mixed dementia, and amyloid angiopathy. AD, Alzheimer’s disease; MCI, Mild cognitive impairment; FTD, Frontotemporal dementia; VaD, Vascular dementia; SCI, Subjective cognitive impairment; CG, Control group; and CSF, Cerebrospinal fluid.
3 Results
3.1 Clinical data
Epidemiological and clinical data, CSF and imaging findings of dementia patients and gender-matched controls are shown in Table 1. The control cohort was younger than the dementia group (mean age 60.9 vs. 72.5 years). Basic CSF diagnostics (e.g., cell count, protein) were available for 89 dementia patients (55.3%) and 10 controls (29.4%). MMSE test results were available for 107 dementia patients (66.5%) and eight controls (23.5%) and showed lower values in the dementia group, as expected. Autoimmune diseases were more frequent in the control cohort (17.6 vs. 9.3%), while cancer was more prevalent in the dementia cohort (16.1 vs. 11.8%), not reaching statistical significance.
3.2 Prevalence of autoantibodies
IgG isotype autoantibodies were significantly more frequent in dementia patients (34 of 161 = 21.1%) compared to controls (1 of 34 = 2.9%, p = 0.04) (Table 2; Figure 2). Antibody-positive patients were younger compared to antibody-negative patients (p = 0.01; Table 1), while they did not differ significantly in MMSE scores, epidemiological and clinical factors, CSF and imaging parameters, or comorbidities (Table 1).
Statistical comparison of the individual dementia groups showed differences in autoantibody frequencies and clinical characteristics such as age, sex, and comorbidities (Table 2). Age and MMSE varied between groups (ANOVA, p < 0.001). The highest autoantibody prevalence was observed in patients with vascular dementia (41.7%) but was also high in AD (30%) and dementia of unknown cause (25%), while lowest in MCI (2.1%).
The patterns of autoantibody binding on unfixed murine brain sections in the 34 dementia patients ranged from myelin staining in cerebellum and/or thalamus (n = 11, Figure 3C) to astrocytes (n = 2, Figures 3A,B), brain blood vessels and immunostaining of the choroid plexus (n = 5, Figures 3E,F,H). Several of these patients had additional ANA patterns in the CSF (n = 10) and serum (n = 17) (Figures 3G,I), but also various staining patterns of fibers and structures with unknown target antigen (Table 3). ANA patterns included among others “rings and rods” and fine-speckled patterns. The single autoantibody-positive control patient showed binding to myelin and nerve fibers. Two further controls had ANA in serum, but not in CSF, thus being negative according to our criteria (Figure 3D).
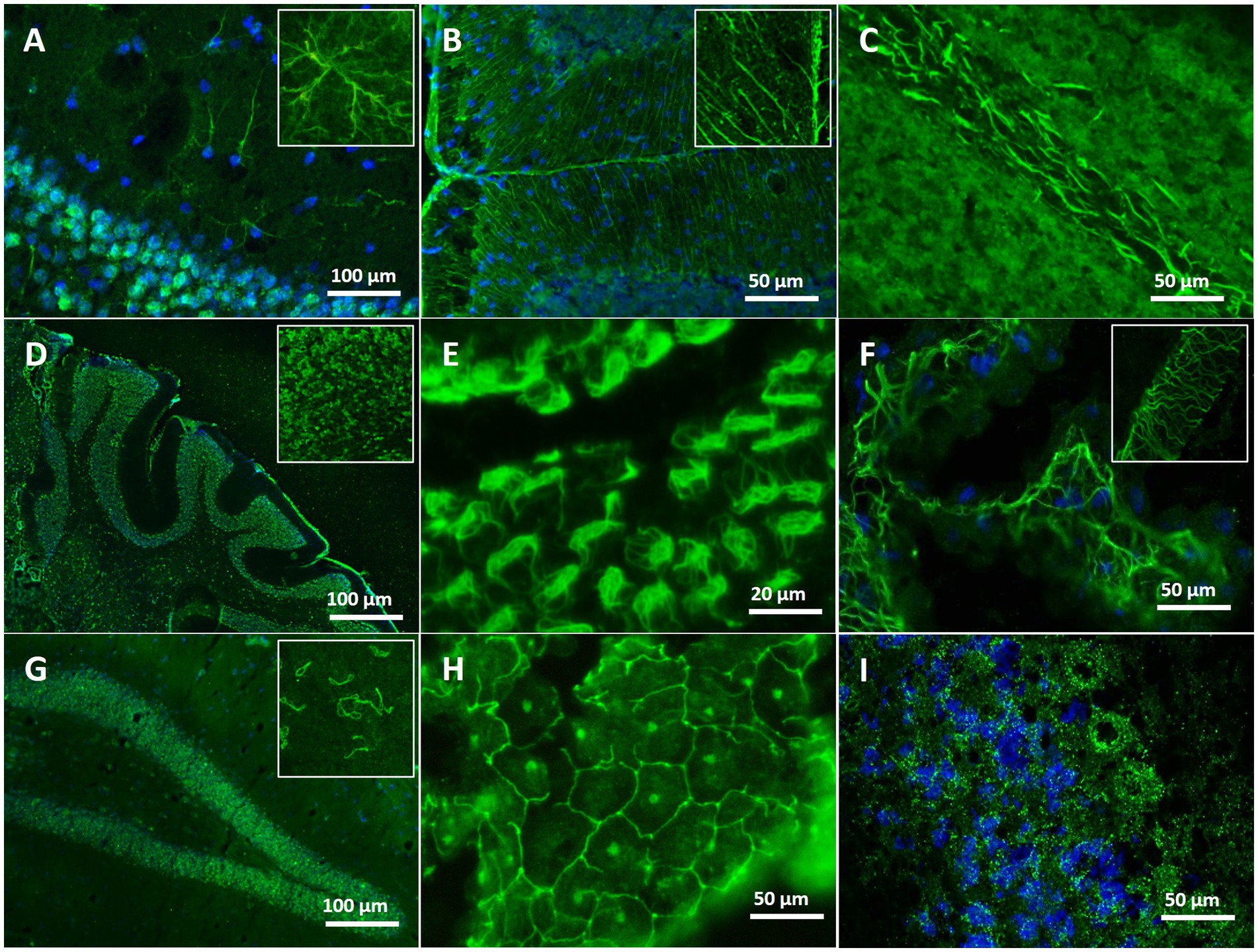
Figure 3. Immunofluorescence staining patterns on unfixed mouse brain with serum/CSF of different patients. (A) Staining of astrocytes in the hippocampal region (blue: co-staining of the nuclei with DAPI) with serum of an AD patient. (B) Distinct labeling of Bergmann glia cells in the cerebellum with serum of an AD patient. (C) Intense myelin staining in the deep white matter of the cerebellum with CSF of an AD patient. (D) Survey micrograph showing ubiquitous ANA staining in the cerebellum using serum of an AD patient. (E) Distinct staining of the ependymal layering of brain ventricles with serum of an AD patient. (F) Net-like staining in the choroid plexus with serum of an AD patient. (G) Intense ANA staining of rings and rod-like structures in hippocampus and cortex using CSF of an AD patient. (H) Reticular labeling of choroid plexus cells resembling a tight junction pattern, in addition staining of nucleoli, using serum of an MCI patient. (I) Finely speckled, granular ANA staining in the cerebellum with serum of an SCI patient (blue: co-staining of the nuclei with DAPI).
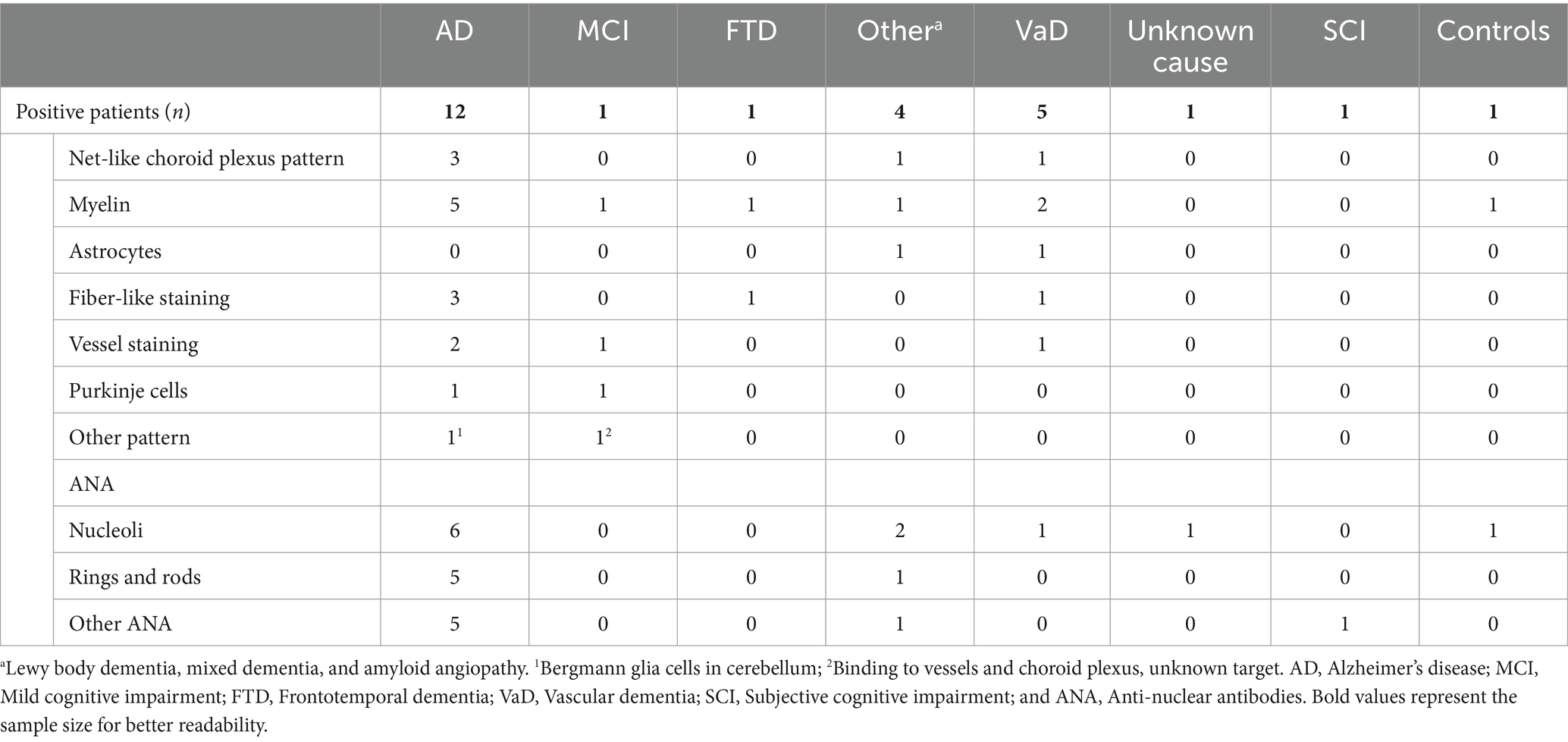
Table 3. Distribution of staining patterns (intensity ≥2) on unfixed murine brain sections among the autoantibody-positive patients of all diagnostic groups.
The search for IgA isotype autoantibodies revealed only one positive patient (0.6%) with ANA reactivity in CSF. This patient was also positive for IgG autoantibodies.
Panel diagnostics for established autoantibodies using a commercial cell-based assay were available in 77 patients (30 CSF plus serum, 41 serum only, and six CSF only). Eleven (14.1%) were autoantibody-positive in serum, and included autoantibodies against myelin (n = 3; titers 1:100 to 1:320), GlyR (n = 2; 1:32), GABABR (n = 2; 1:10 to 1:100), ARHGAP26 (n = 1; 1:32), GFAP (n = 1; 1:100), KCNA2 (n = 1; 1:320), and Caspr2 (n = 1; 1:32).
4 Discussion
In this pilot study, we identified both, well-established and potentially novel autoantibodies in 21.1% of patients with different forms of cognitive impairment and dementia, compared to 2.9% of controls. Antigenic targets included GFAP, ARHGAP26, KCNA2, Caspr2, GlyR, and GABABR, but also various not yet identified antigens on unfixed mouse brain sections, such as myelinated fibers, brain vessels, astroglia, choroid plexus, and antinuclear antigens. Autoantibody prevalence was highest in vascular dementia (41.7%), but also common in AD (30%). Given the large variety of autoantibodies, it is not surprising that autoantibody-positive patients had similar MMSE scores compared to autoantibody-negative patients. Further studies are needed to identify potential relationships between certain subgroups of autoantibodies with clinical symptoms including cognitive impairment.
The here observed frequencies are in a similar range to previous studies. For example, in a recent cohort of 349 patients with various neurodegenerative diseases, established autoantibodies overlapping with our diagnostic panel were detected in 11.8% (26). Likewise, 13.8% of 93 patients with neurodegenerative disorders in another study had surface-reactive autoantibodies, although less common in AD (27). Focusing on NMDAR autoantibodies, an early study from our center reported 16.1% seropositivity in 286 patients with neurodegenerative dementia, with the highest prevalence in the subgroup of unclassified dementia (15).
Detection of autoantibodies in patients with cognitive impairment is clearly not sufficient for the classification of dementia as being “autoimmune,” as even well-characterized autoantibodies also regularly occur in control populations, at least in serum (26). The concept of autoimmune dementia so far embraces conditions with predominant memory impairment typically characterized by subacute onset, a rapid, fluctuating progression and inflammatory CSF parameters, often with detected anti-neuronal autoantibodies and responsiveness to immunotherapy (3, 28, 29). In some patients with autoimmune dementia, neurodegeneration biomarker profiles can mimic protein patterns indicative of neuronal destruction seen in neurodegenerative dementias. On the other hands, autoantibodies may not always cause damage, but could be mere bystanders or even convey positive effects, ranging from limiting damage to reducing neurodegeneration-associated proteins such as β-amyloid, or facilitating remyelination (30).
It is subject of intensive research, which autoantibodies may contribute to cognitive impairment and how they exert effects. The current study did not assess pathogenic functions of the identified autoantibodies. For some of them, however, previous studies demonstrated pathogenicity that can be plausibly linked to cognitive impairment, even though numbers of study participants were generally low. For example, cognitive impairment was the main common feature of five patients with GlyR autoantibodies in one study, associated with elevated tTau/pTau in CSF (31). Similarly, in our analysis, the two patients with serum GlyR autoantibodies had the diagnosis of early-stage AD with increased pTau/tTau levels. Autoantibodies against KCNA2 have also been reported in progressive dementia, in one case with an AD CSF profile (32). In patients with FTD, autoantibodies against GluR3 and IgLON5 were found (33, 34), which are known to induce receptor internalization and impair long-term synaptic plasticity (35, 36). We could further identify several dementia patients with autoantibodies against GFAP and/or astrocytes, which seems an interesting new marker not only for a subacute autoimmune meningoencephalomyelitis (37–40), but also for patients with slowly progressing cognitive decline and dementia (41–44).
Autoantibodies in our study were not equally distributed between subgroups of patients. In AD patients, 30% had IgG binding to certain brain antigens, which is in line with previous findings of a wide range of autoantibodies observed in AD, such as against NMDAR, dopamine receptor, acetylcholine receptor, and many more using targeted and unbiased detection approaches (12, 14, 15, 45–50). The highest prevalence was observed in vascular dementia with several patients harboring not previously described autoantibodies against the choroid plexus. Whether such autoantibodies can impair blood–brain barrier function and potentially dysregulate permeability for neurotoxic molecules is currently unclear. However, studies on autoantibodies targeting endothelial barrier function in the brain suggest that this can be a pathogenic mechanism (51–54).
The frequent finding of ANAs adds to previous studies which, for example, found significantly increased serum frequencies in patients with FTD (60% versus 13% in healthy controls) (55). Data on ANAs in CSF are almost not available. We thus focused on CSF and found 20.5% of available CSF samples from the dementia cohort to be positive for ANAs. Although ANAs have long been considered non-pathogenic due to their intracellular targets, increasing evidence suggests that autoantibodies can reach intracellular epitopes and can have disease-related effects (56, 57). Development of pathology may take much longer compared to binding of autoantibodies to neuronal surface receptors. It is tempting to speculate, however, whether such protracted subtle effects may build up over time and contribute to cognitive impairment when patients at risk have high-level autoantibodies, a concept that was recently coined “smoldering humoral autoimmunity” (1).
The study has several limitations. Related to the retrospective study design, CSF and serum samples, clinical information, neuropsychological assessments and imaging were not available for the entire cohort. Despite the number of 195 study participants, some disease subgroups were too small for a robust statistical analysis, in particular as the patients showed variability in disease course and comorbidities. The age difference between dementia patients and controls is likely related to the preferential age of the different diseases, however, it may have affected our findings as (humoral) autoimmunity and inflammation is age-dependent. Although our diagnostic assay using unfixed murine brain sections has been consistently used in different neuropsychiatric clinical conditions to identify novel autoantibodies [e.g. (21, 22, 58, 59)], the handling of unfixed brain has technical challenges, which so far prevented broader application in routine laboratories, thus validation across different centers is pending.
Taken together, the present study reports increased frequencies of established and novel autoantibodies in patients with cognitive impairment, suggesting that many more autoantibodies can be seen in the CSF of patients with cognitive impairment, than currently investigated using routine assays. Evolving data on immunotherapy-responsive cases suggest that some antibodies are not mere bystanders of dysregulated autoimmunity following neurodegeneration. Better understanding of the autoantibodies’ function will help to identify future patients who might benefit from treatment. The generation of human disease-derived monoclonal autoantibodies will likely change our approach to autoimmune dementia in the near future, as we will learn about the pathogenicity of these antibodies, their antigenic targets on neurons and glial cells, their contribution to disease, and how they can facilitate the development of novel, antibody-selective therapies.
Data availability statement
The original contributions presented in the study are included in the article/supplementary material, further inquiries can be directed to the corresponding author.
Ethics statement
The studies involving humans were approved by Charité Universitätsmedizin Berlin Institutional Review Board, #EA1/258/18. The studies were conducted in accordance with the local legislation and institutional requirements. The participants provided their written informed consent to participate in this study.
Author contributions
FS: Conceptualization, Data curation, Formal analysis, Investigation, Methodology, Writing – original draft, Writing – review & editing. HF: Data curation, Writing – original draft, Writing – review & editing. MB: Data curation, Supervision, Writing – review & editing. MH: Supervision, Writing – review & editing. LL: Data curation, Writing – review & editing. WS: Data curation, Investigation, Writing – review & editing. BT: Data curation, Investigation, Writing – review & editing. HP: Conceptualization, Data curation, Funding acquisition, Investigation, Methodology, Project administration, Resources, Supervision, Writing – original draft, Writing – review & editing.
Funding
The author(s) declare that financial support was received for the research, authorship, and/or publication of this article. This work was supported by grants to HP from the German Research Foundation (DFG) [grants FOR3004, PR1274/4-1, PR1274/5-1, and PR1274/9-1, clinical research unit 5023/1 “BECAUSE-Y” (project number 504745852)], the Helmholtz Association (HIL-A03), and the German Federal Ministry of Education and Research (Connect-Generate 16GW0279K).
Acknowledgments
We thank Stefanie Bandura, Doreen Brandl, and Matthias Sillmann for excellent technical assistance.
Conflict of interest
The authors declare that the research was conducted in the absence of any commercial or financial relationships that could be construed as a potential conflict of interest.
Publisher’s note
All claims expressed in this article are solely those of the authors and do not necessarily represent those of their affiliated organizations, or those of the publisher, the editors and the reviewers. Any product that may be evaluated in this article, or claim that may be made by its manufacturer, is not guaranteed or endorsed by the publisher.
References
1. Prüss, H . Autoantibodies in neurological disease. Nat Rev Immunol. (2021) 21:798–813. doi: 10.1038/s41577-021-00543-w
2. Duong, SL, and Pruss, H. Molecular disease mechanisms of human antineuronal monoclonal autoantibodies. Trends Mol Med. (2022) 29:20–34. doi: 10.1016/j.molmed.2022.09.011
3. Flanagan, EP, McKeon, A, Lennon, VA, Boeve, BF, Trenerry, MR, Tan, KM, et al. Autoimmune dementia: clinical course and predictors of immunotherapy response. Mayo Clin Proc. (2010) 85:881–97. doi: 10.4065/mcp.2010.0326
4. Hansen, N . Current nosology of neural autoantibody-associated dementia. Front Aging Neurosci. (2021) 13:711195. doi: 10.3389/fnagi.2021.711195
5. Marquetand, J, van Lessen, M, Bender, B, Reimold, M, Elsen, G, Stoecker, W, et al. Slowly progressive LGI1 encephalitis with isolated late-onset cognitive dysfunction: a treatable mimic of Alzheimer's disease. Eur J Neurol. (2016) 23:e28–9. doi: 10.1111/ene.12939
6. McKeon, A, Marnane, M, O'Connell, M, Stack, JP, Kelly, PJ, and Lynch, T. Potassium channel antibody associated encephalopathy presenting with a frontotemporal dementia like syndrome. Arch Neurol. (2007) 64:1528–30. doi: 10.1001/archneur.64.10.1528
7. Finke, C, Prüss, H, Heine, J, Reuter, S, Kopp, UA, Wegner, F, et al. Evaluation of cognitive deficits and structural hippocampal damage in encephalitis with leucine-rich, glioma-inactivated 1 antibodies. JAMA Neurol. (2017) 74:50–9. doi: 10.1001/jamaneurol.2016.4226
8. Gaig, C, Graus, F, Compta, Y, Högl, B, Bataller, L, Brüggemann, N, et al. Clinical manifestations of the anti-IgLON5 disease. Neurology. (2017) 88:1736–43. doi: 10.1212/WNL.0000000000003887
9. Gruter, T, Mollers, FE, Tietz, A, Dargvainiene, J, Melzer, N, Heidbreder, A, et al. Clinical, serological and genetic predictors of response to immunotherapy in anti-IgLON5 disease. Brain. (2022) 146:600–11. doi: 10.1093/brain/awac090
10. Ryding, M, Gamre, M, Nissen, MS, Nilsson, AC, Okarmus, J, Poulsen, AAE, et al. Neurodegeneration induced by anti-IgLON5 antibodies studied in induced pluripotent stem cell-derived human neurons. Cells. (2021) 10:837. doi: 10.3390/cells10040837
11. Barthel, PC, Staabs, F, Li, LY, Buthut, M, Otto, C, Ruprecht, K, et al. Immunoreactivity to astrocytes in different forms of dementia: high prevalence of autoantibodies to GFAP. Brain Behav Immun Health. (2023) 29:100609. doi: 10.1016/j.bbih.2023.100609
12. Wu, J, and Li, L. Autoantibodies in Alzheimer's disease: potential biomarkers, pathogenic roles, and therapeutic implications. J Biomed Res. (2016) 30:361–72. doi: 10.7555/JBR.30.20150131
13. Thyrian, JR, Hertel, J, Schulze, LN, Dorr, M, Pruss, H, Hempel, P, et al. Prevalence and determinants of agonistic autoantibodies against alpha1-adrenergic receptors in patients screened positive for dementia: results from the population-based DelpHi-study. J Alzheimers Dis. (2018) 64:1091–7. doi: 10.3233/JAD-171096
14. Busse, S, Brix, B, Kunschmann, R, Bogerts, B, Stoecker, W, and Busse, M. N-methyl-d-aspartate glutamate receptor (NMDA-R) antibodies in mild cognitive impairment and dementias. Neurosci Res. (2014) 85:58–64. doi: 10.1016/j.neures.2014.06.002
15. Doss, S, Wandinger, KP, Hyman, BT, Panzer, JA, Synofzik, M, Dickerson, B, et al. High prevalence of NMDA receptor IgA/IgM antibodies in different dementia types. Ann Clin Transl Neurol. (2014) 1:822–32. doi: 10.1002/acn3.120
16. Gardoni, F, Stanic, J, Scheggia, D, Benussi, A, Borroni, B, and Di Luca, M. NMDA and AMPA receptor autoantibodies in brain disorders: from molecular mechanisms to clinical features. Cells. (2021) 10:77. doi: 10.3390/cells10010077
17. Kayser, MS, and Dalmau, J. Anti-NMDA receptor encephalitis, autoimmunity, and psychosis. Schizophr Res. (2016) 176:36–40. doi: 10.1016/j.schres.2014.10.007
18. Bartels, F, Strönisch, T, Farmer, K, Rentzsch, K, Kiecker, F, and Finke, C. Neuronal autoantibodies associated with cognitive impairment in melanoma patients. Ann Oncol. (2019) 30:823–9. doi: 10.1093/annonc/mdz083
19. Elahi, FM, and Miller, BL. A clinicopathological approach to the diagnosis of dementia. Nat Rev Neurol. (2017) 13:457–76. doi: 10.1038/nrneurol.2017.96
20. Endres, D, Schlump, A, Nickel, K, Berger, B, Runge, K, Lange, T, et al. Frontotemporal dementia associated with intrathecal antibodies against axon initial segments. Alzheimers Dement. (2023) 19:736–9. doi: 10.1002/alz.12780
21. Endres, D, von Zedtwitz, K, Matteit, I, Bünger, I, Foverskov-Rasmussen, H, Runge, K, et al. Spectrum of novel anti–central nervous system autoantibodies in the cerebrospinal fluid of 119 patients with schizophreniform and affective disorders. Biol Psychiatry. (2022) 92:261–74. doi: 10.1016/j.biopsych.2022.02.010
22. Kreye, J, Reincke, SM, Kornau, H-C, Sánchez-Sendin, E, Corman, VM, Liu, H, et al. A therapeutic non-self-reactive SARS-CoV-2 antibody protects from lung pathology in a COVID-19 Hamster model. Cell. (2020) 183:1058–1069.e19. doi: 10.1016/j.cell.2020.09.049
23. Lütt, A, Michel, K, Kruger, D, Volz, MS, Nassir, M, Schulz, E, et al. High prevalence and functional effects of serum antineuronal antibodies in patients with gastrointestinal disorders. Neurogastroenterol Motil. (2018) 30:e13292. doi: 10.1111/nmo.13292
24. Schiavon, CR, Griffin, ME, Pirozzi, M, Parashuraman, R, Zhou, W, Jinnah, HA, et al. Compositional complexity of rods and rings. Mol Biol Cell. (2018) 29:2303–16. doi: 10.1091/mbc.E18-05-0274
25. Damoiseaux, J, Andrade, LEC, Carballo, OG, Conrad, K, Francescantonio, PLC, Fritzler, MJ, et al. Clinical relevance of HEp-2 indirect immunofluorescent patterns: the international consensus on ANA patterns (ICAP) perspective. Ann Rheum Dis. (2019) 78:879–89. doi: 10.1136/annrheumdis-2018-214436
26. Daguano Gastaldi, V, Bh Wilke, J, Weidinger, CA, Walter, C, Barnkothe, N, Teegen, B, et al. Factors predisposing to humoral autoimmunity against brain-antigens in health and disease: analysis of 49 autoantibodies in over 7000 subjects. Brain Behav Immun. (2023) 108:135–47. doi: 10.1016/j.bbi.2022.10.016
27. Giannoccaro, MP, Gastaldi, M, Rizzo, G, Jacobson, L, Vacchiano, V, Perini, G, et al. Antibodies to neuronal surface antigens in patients with a clinical diagnosis of neurodegenerative disorder. Brain Behav Immun. (2021) 96:106–12. doi: 10.1016/j.bbi.2021.05.017
28. Banks, SA, Sechi, E, and Flanagan, EP. Autoimmune encephalopathies presenting as dementia of subacute onset and rapid progression. Ther Adv Neurol Disord. (2021) 14:175628642199890. doi: 10.1177/1756286421998906
29. Hansen, N, Juhl, AL, Grenzer, IM, Hirschel, S, Teegen, B, Fitzner, D, et al. Cerebrospinal fluid Total tau protein correlates with longitudinal, progressing cognitive dysfunction in anti-neural autoantibody-associated dementia and Alzheimer's dementia: a case-control study. Front Immunol. (2022) 13:837376. doi: 10.3389/fimmu.2022.837376
30. Kocurova, G, Ricny, J, and Ovsepian, SV. Autoantibodies targeting neuronal proteins as biomarkers for neurodegenerative diseases. Theranostics. (2022) 12:3045–56. doi: 10.7150/thno.72126
31. Hansen, N, Bartels, C, Stocker, W, Wiltfang, J, and Fitzner, D. Impaired verbal memory recall in patients with axonal degeneration and serum Glycine-receptor autoantibodies-case series. Front Psychol. (2021) 12:778684. doi: 10.3389/fpsyt.2021.778684
32. Timaus, C, von Gottberg, P, Hirschel, S, Lange, C, Wiltfang, J, and Hansen, N. KCNA2 autoimmunity in progressive cognitive impairment: case series and literature review. Brain Sci. (2021) 11:89. doi: 10.3390/brainsci11010089
33. Borroni, B, Stanic, J, Verpelli, C, Mellone, M, Bonomi, E, Alberici, A, et al. Anti-AMPA GluA3 antibodies in frontotemporal dementia: a new molecular target. Sci Rep. (2017) 7:6723. doi: 10.1038/s41598-017-06117-y
34. Bright, F, Werry, EL, Dobson-Stone, C, Piguet, O, Ittner, LM, Halliday, GM, et al. Neuroinflammation in frontotemporal dementia. Nat Rev Neurol. (2019) 15:540–55. doi: 10.1038/s41582-019-0231-z
35. Haselmann, H, Mannara, F, Werner, C, Planaguma, J, Miguez-Cabello, F, Schmidl, L, et al. Human autoantibodies against the AMPA receptor subunit GluA2 induce receptor reorganization and memory dysfunction. Neuron. (2018) 100:e109. doi: 10.1016/j.neuron.2018.07.048
36. Palese, F, Bonomi, E, Nuzzo, T, Benussi, A, Mellone, M, Zianni, E, et al. Anti-GluA3 antibodies in frontotemporal dementia: effects on glutamatergic neurotransmission and synaptic failure. Neurobiol Aging. (2020) 86:143–55. doi: 10.1016/j.neurobiolaging.2019.10.015
37. Brandebura, AN, Paumier, A, Onur, TS, and Allen, NJ. Astrocyte contribution to dysfunction, risk and progression in neurodegenerative disorders. Nat Rev Neurosci. (2023) 24:23–39. doi: 10.1038/s41583-022-00641-1
38. Fang, B, McKeon, A, Hinson, SR, Kryzer, TJ, Pittock, SJ, Aksamit, AJ, et al. Autoimmune glial fibrillary acidic protein Astrocytopathy: a novel Meningoencephalomyelitis. JAMA Neurol. (2016) 73:1297–307. doi: 10.1001/jamaneurol.2016.2549
39. Gravier-Dumonceau, A, Ameli, R, Rogemond, V, Ruiz, A, Joubert, B, Muniz-Castrillo, S, et al. Glial fibrillary acidic protein autoimmunity: a French cohort study. Neurology. (2022) 98:e653–68. doi: 10.1212/WNL.0000000000013087
40. Lin, H, Huang, Y, Zeng, H, Wang, M, Guan, S, Chen, G, et al. Overlapping clinical syndromes in patients with glial fibrillary acidic protein IgG. Neuroimmunomodulation. (2020) 27:69–74. doi: 10.1159/000505730
41. Friedrich, M, Hartig, J, Prüss, H, Ip, CW, and Volkmann, J. Rapidly progressive dementia: extending the spectrum of GFAP-astrocytopathies? Ann Clin Transl Neurol. (2022) 9:410–5. doi: 10.1002/acn3.51513
42. Hansen, N, Stocker, W, Wiltfang, J, Bartels, C, Rentzsch, K, and Bouter, C. Case report: semantic variant of primary progressive aphasia associated with anti-glial fibrillary acid protein autoantibodies. Front Immunol. (2021) 12:760021. doi: 10.3389/fimmu.2021.760021
43. Lopez, OL, Rabin, BS, Huff, FJ, Rezek, D, and Reinmuth, OM. Serum autoantibodies in patients with Alzheimer's disease and vascular dementia and in nondemented control subjects. Stroke. (1992) 23:1078–83. doi: 10.1161/01.STR.23.8.1078
44. Schott, K, Wormstall, H, Dietrich, M, Klein, R, and Batra, A. Autoantibody reactivity in serum of patients with Alzheimer's disease and other age-related dementias. Psychiatry Res. (1996) 59:251–4. doi: 10.1016/0165-1781(95)02703-3
45. Busse, M, Kunschmann, R, Dobrowolny, H, Hoffmann, J, Bogerts, B, Steiner, J, et al. Dysfunction of the blood-cerebrospinal fluid-barrier and N-methyl-D-aspartate glutamate receptor antibodies in dementias. Eur Arch Psychiatry Clin Neurosci. (2018) 268:483–92. doi: 10.1007/s00406-017-0768-z
46. Colasanti, T, Barbati, C, Rosano, G, Malorni, W, and Ortona, E. Autoantibodies in patients with Alzheimer's disease: pathogenetic role and potential use as biomarkers of disease progression. Autoimmun Rev. (2010) 9:807–11. doi: 10.1016/j.autrev.2010.07.008
47. Giil, LM, Aarsland, D, Hellton, K, Lund, A, Heidecke, H, Schulze-Forster, K, et al. Antibodies to multiple receptors are associated with neuropsychiatric symptoms and mortality in Alzheimer's disease: a longitudinal study. J Alzheimers Dis. (2018) 64:761–74. doi: 10.3233/JAD-170882
48. San Segundo-Acosta, P, Montero-Calle, A, Fuentes, M, Rabano, A, Villalba, M, and Barderas, R. Identification of Alzheimer's disease autoantibodies and their target biomarkers by phage microarrays. J Proteome Res. (2019) 18:2940–53. doi: 10.1021/acs.jproteome.9b00258
49. Wallukat, G, Pruss, H, Muller, J, and Schimke, I. Functional autoantibodies in patients with different forms of dementia. PLoS One. (2018) 13:e0192778. doi: 10.1371/journal.pone.0192778
50. Wang, BZ, Zailan, FZ, Wong, BYX, Ng, KP, and Kandiah, N. Identification of novel candidate autoantibodies in Alzheimer's disease. Eur J Neurol. (2020) 27:2292–6. doi: 10.1111/ene.14290
51. Atzeni, F, Pipitone, N, Iaccarino, L, Masala, IF, Weiss, R, Alciati, A, et al. Rheumatic diseases and autoimmune vascular dementia. Autoimmun Rev. (2017) 16:1265–9. doi: 10.1016/j.autrev.2017.10.011
52. Fu, Y, and Yan, Y. Emerging role of immunity in cerebral small vessel disease. Front Immunol. (2018) 9:67. doi: 10.3389/fimmu.2018.00067
53. Hempel, P, Heinig, B, Jerosch, C, Decius, I, Karczewski, P, Kassner, U, et al. Immunoadsorption of agonistic autoantibodies against alpha1-adrenergic receptors in patients with mild to moderate dementia. Ther Apher Dial. (2016) 20:523–9. doi: 10.1111/1744-9987.12415
54. Li, LY, Kreye, J, Burek, M, Cordero-Gomez, C, Barthel, PC, Sánchez-Sendín, E, et al. Brain blood vessel autoantibodies in patients with NMDA and GABA(a) receptor encephalitis: identification of unconventional myosin-X as target antigen. Front Cell Neurosci. (2023) 17:1077204. doi: 10.3389/fncel.2023.1077204
55. Cavazzana, I, Alberici, A, Bonomi, E, Ottaviani, R, Kumar, R, Archetti, S, et al. Antinuclear antibodies in frontotemporal dementia: the tip's of autoimmunity iceberg? J Neuroimmunol. (2018) 325:61–3. doi: 10.1016/j.jneuroim.2018.10.006
56. Nasarallah, GK, Fakhroo, AD, Khan, T, Cyprian, FS, Al Ali, F, Ata, MMA, et al. Detection of antinuclear antibodies targeting intracellular signal transduction, metabolism, apoptotic processes and cell death in critical COVID-19 patients. Mediterr J Hematol Infect Dis. (2022) 14:e2022076. doi: 10.4084/MJHID.2022.076
57. Rocchi, A, Sacchetti, S, De Fusco, A, Giovedi, S, Parisi, B, Cesca, F, et al. Autoantibodies to synapsin I sequestrate synapsin I and alter synaptic function. Cell Death Dis. (2019) 10:864. doi: 10.1038/s41419-019-2106-z
58. Franke, C, Boesl, F, Goereci, Y, Gerhard, A, Schweitzer, F, Schroeder, M, et al. Association of cerebrospinal fluid brain-binding autoantibodies with cognitive impairment in post-COVID-19 syndrome. Brain Behav Immun. (2023) 109:139–43. doi: 10.1016/j.bbi.2023.01.006
Keywords: neurodegenerative dementia, autoantibodies, CSF, cognitive impairment, autoimmunity
Citation: Staabs F, Foverskov Rasmussen H, Buthut M, Höltje M, Li LY, Stöcker W, Teegen B and Prüss H (2024) Brain-targeting autoantibodies in patients with dementia. Front. Neurol. 15:1412813. doi: 10.3389/fneur.2024.1412813
Edited by:
Madepalli Krishnappa Lakshmana, Florida International University, United StatesReviewed by:
Jan Říčný, National Institute of Mental Health, CzechiaAnushruti Ashok, Cleveland Clinic, United States
Copyright © 2024 Staabs, Foverskov Rasmussen, Buthut, Höltje, Li, Stöcker, Teegen and Prüss. This is an open-access article distributed under the terms of the Creative Commons Attribution License (CC BY). The use, distribution or reproduction in other forums is permitted, provided the original author(s) and the copyright owner(s) are credited and that the original publication in this journal is cited, in accordance with accepted academic practice. No use, distribution or reproduction is permitted which does not comply with these terms.
*Correspondence: Harald Prüss, aGFyYWxkLnBydWVzc0BjaGFyaXRlLmRl