- 1Spinal Surgery, Tongliao People's Hospital, Tongliao, Inner Mongolia, China
- 2Computer Network Information Center, Tongliao People's Hospital, Tongliao, Inner Mongolia, China
- 3Bone Trauma Surgery, The 960th Hospital of the PLA Joint Logistics Support Force, Jinan, Shandong, China
Background: Spinal cord injury (SCI) is a nervous system disease leading to motor and sensory dysfunction below the injury level, and can result in paralysis. MicroRNAs (miRNAs) play a key role in SCI treatment, and related research provides insights for SCI diagnosis and treatment. Bibliometrics is an important tool for literature statistics and evaluation, objectively summarizing multidimensional information. This study comprehensively overviews the field through bibliometric analysis of miRNA and SCI research, providing contemporary resources for future collaboration and clinical treatment.
Materials and methods: In this study, we searched the Web of Science Core Collection (WOSCC) database. After careful screening and data import, we extracted annual publications, citation counts, countries, institutions, authors, journals, highly cited articles, co-cited articles, keywords, and H-index. Bibliometrics and visualization analyses employed VOSviewer, CiteSpace, the R package “bibliometrix,” and online analytic platforms. Using Arrowsmith,1 we determined miRNA-SCI relationships and discussed potential miRNA mechanisms in SCI.
Results: From 2008 to 2024, the number of related papers increased annually, reaching 754. The number of yearly publications remained high and entered a period of rapid development. Researchers from 50 countries/regions, 802 institutions, 278 journals, and 3,867 authors participated in the field. Currently, China has advantages in the number of national papers, citations, institutions, and authors. However, it is necessary to strengthen cooperation among different authors, institutions, and countries to promote the production of important academic achievements. The research in the field currently focuses on nerve injury, apoptosis, and gene expression. Future research directions mainly involve molecular mechanisms, clinical trials, exosomes, and inflammatory reactions.
Conclusion: Overall, this study comprehensively analyzes the research status and frontier of miRNAs in SCI. A systematic summary provides a complete and intuitive understanding of the relationship between SCI and miRNAs. The presented findings establish a basis for future research and clinical application in this field.
Introduction
Spinal cord injury (SCI) is a disease of the nervous system that can lead to motor and sensory dysfunction below the injury level, and even paralysis (1). About 250,000–500,000 people suffer from this disease annually worldwide (2, 3). The lifetime treatment cost for each SCI patient in the United States is about $500,000 to $1 million, with annual costs exceeding $7 billion (4, 5). Patients experience significant physical and psychological pain, making SCI a major societal burden. The pathophysiological development of SCI is complex, involving cell death, axonal injury, glial scar formation, inflammation, and more (6). However, current clinical treatment effects are very limited, and there is no cure yet, making SCI a major focus of research.
MicroRNAs (miRNAs) are endogenous small single-stranded non-coding RNA molecules (18–25 nucleotides) that can bind to target messenger RNA (mRNA) molecules to interfere with translation (7, 8). As key regulatory factors of transcriptional gene expression changes in nervous system diseases, miRNAs have been widely concerned and studied by researchers (9). During SCI development, miRNA regulates neuronal plasticity, degeneration, axon regeneration, and myelin regeneration by changing gene expression (10, 11). Research shows miR-940 decreases after SCI, and miR-940 overexpression inhibits inflammation and promotes functional recovery (12). Another study showed miRNA-124 inhibits neuronal apoptosis and induces functional recovery in SCI rats (13). Clarifying miRNAs’ role in SCI pathophysiology is of great scientific significance for solving nerve regeneration and functional repair problems in patients.
Bibliometrics is an important tool for literature statistics and evaluation. By combining qualitative and quantitative analysis, this method can objectively and intuitively summarize multidimensional information (14). VOSviewer and CiteSpace are two popular bibliometric software (15, 16), which provide an overall view of basic data and dynamic trends. Research trends and hotspots in a field can be better explored and grasped by researchers through bibliometrics studies. Though there have been an increasing number of such studies with enhancement in bibliometrics visualization tools and research depth in recent years, no bibliometrics studies have focused on the role of miRNA in SCI.
Currently, a plethora of studies have explored the mechanistic relationship between miRNA and SCI, laying the foundation for clinical translation of SCI treatment. This study provides a comprehensive overview of the field through bibliometric analysis of miRNA and SCI research, enhancing overall understanding of researchers and providing contemporary resources for future collaboration and clinical treatment.
Materials and methods
Data sources and search strategies
In this study, we searched the Web of Science Core Collection (WOSCC) database, which contains a large number of documents in the biomedical field. The search used the following formats: TS = (microRNA*) AND TS = (Spinal cord injury). The time span was January 1, 2008 to January 31, 2024. After carefully searching the existing literature, we screened out 1,087 potential articles. We limited the types of articles to Article and limited the language to English, finally including 754 articles (Figure 1).
Data collection and statistics
Search results are downloaded in a “plain text file” format, with complete records and references in the WOSCC database. Data are extracted after careful screening and importing into Microsoft Excel 2016 for analysis: annual publications, citation numbers, countries, institutions, authors, journals, highly cited articles, co-cited articles, keywords, and H-index. The summarized data are imported into the online bibliometrics analysis platform,2 and a fitting curve is used to predict the number of published documents.
Bibliometric analysis
To analyze the bibliometrics of SCI and miRNA, we visually analyzed selected data. These data clarified research trends, influence, and distribution. This paper used VOSviewer software for visual analysis of authors, keywords, keyword co-occurrence timeline, density maps, journals, highly cited literature, and co-cited literature. CiteSpace software was used for detailed visualization of countries/regions cooperation network map, institutions cooperation network map, strongest citation burst, keyword clustering, journal dual graph overlay, etc. The node size represents the number of nodes; the lines between nodes represent cooperation between institutions, with thicker lines indicating closer relationships. From these results, inferences about relationships between research hotspots and frontiers were made.
Analysis of the potential mechanisms based on the Arrowsmith project
Arrowsmith (see text footnote 1) was utilized to identify relationships between miRNAs and SCI and evaluate potential miRNA mechanisms in SCI. Keywords filtered through Arrowsmith served as prediction groups, while those filtered through VOSviewer were confirmation groups. Venn diagrams were drawn for both to determine potential keywords for miRNA and SCI research. R software was used for gene ontology (GO) analysis and Kyoto Encyclopedia of Genes and Genomes (KEGG) pathway analysis of potential genes with correlation capacity ≥0.95. The STRING online database3 was used to create a potential gene protein–protein interaction (PPI) network, visualized with Cytoscape software. Cytoscape software also aided in screening genes within the PPI network.
Results
Annual publications and trends
Analyzing publication trends enables understanding of the developmental speed and changing attention to miRNAs in SCI treatment (Figure 2). From 2008 to 2024, 754 papers were published. Annual publication numbers rapidly increased from 2013 to 2019, peaking in 2019 (n = 102). Publications in 2019–2022 were relatively flat, but decreased significantly in 2023 (n = 55). With only January 2024 included, it is currently the year with the fewest publications (n = 6). The function formula of the fitting curve is “y = 1.2421x2.265, R2 = 0.9874,” indicating the research growth curve in this field fits well. These results show studying miRNAs is an important direction in SCI.
Country/region analysis and international cooperation
We found that a total of 50 countries/regions have published literature on the role of miRNAs in SCI, with China being the most prominent country, accounting for the largest number of publications (n = 527; 59.48%) and citations (n = 10,155; 40.32%) (Figures 3A,B; Table 1). With the globalization of knowledge and technology, international cooperation is increasingly close, and the United States plays an important role in the cooperation network. European countries have the closest cooperation (Figure 3C). Overall, although China has the largest number of publications, it still lacks international cooperation and needs to strengthen exchanges in the future.
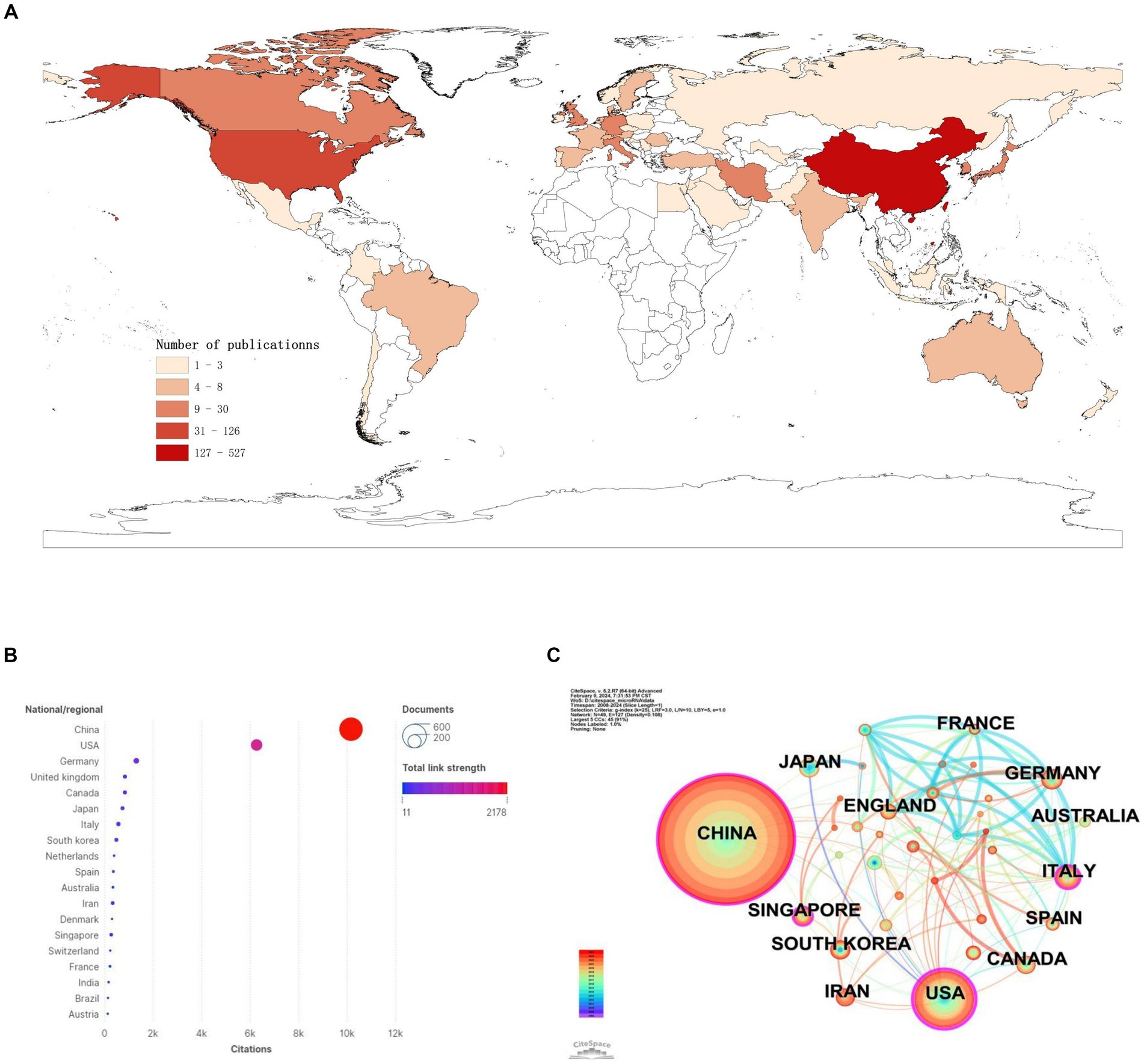
Figure 3. National/regional contributions of miRNAs and SCI-related research. (A) Comparative map of the cumulative number of papers published in each Countries/regions. (B) Countries/regions cited frequency bubble map. (C) Cooperation networks in countries/regions around the world.
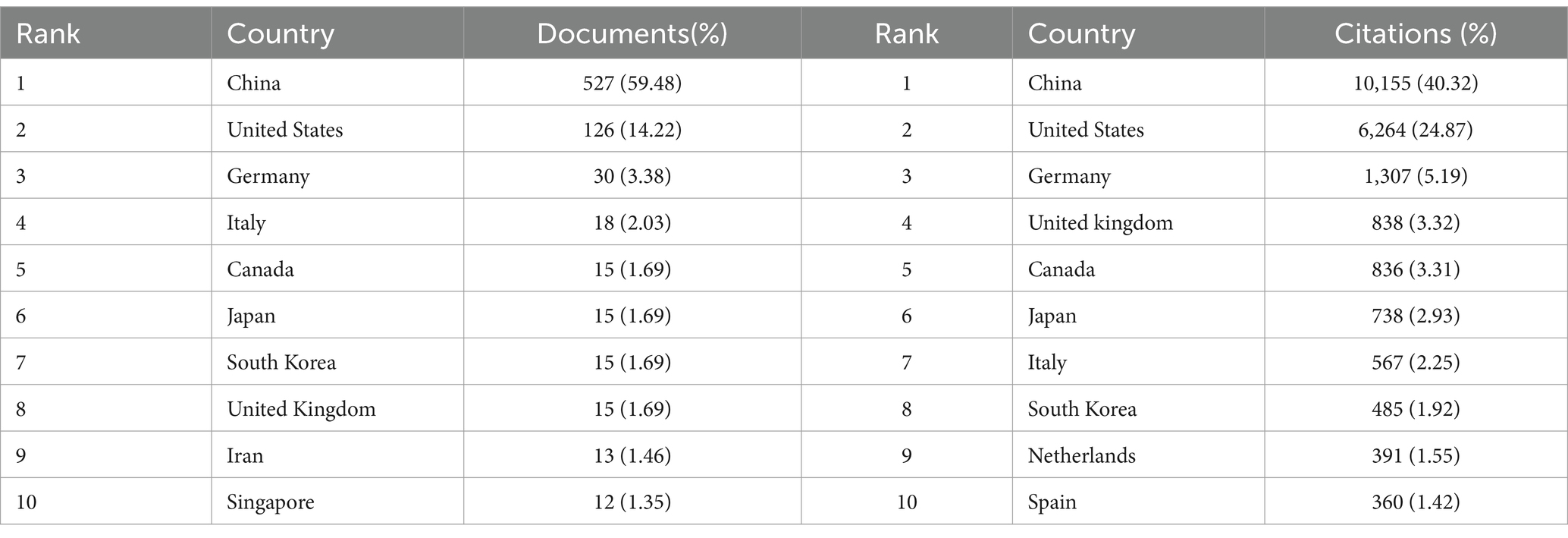
Table 1. Top 10 countries/regions in terms of the number of documents and citations on miRNAs in SCI.
Institutional contribution
Papers in this field have been published by 802 institutions. Most of the top 10 universities in publication count and citation count are from China. China Medical University published the most articles (n = 40; 2.67%) and received the most citations (n = 891; 2.26%), ranking first in both categories (Figures 4A,B; Table 2). Johns Hopkins University ranks first in centrality (coefficient = 0.05), while Chinese research institutions rank relatively high, showing that Chinese universities have made remarkable achievements and great influence in the field of miRNAs related to SCI, but there is still room for improvement (Figure 4C). Nevertheless, analyzing the scale and degree of inter-institutional collaboration reveals that institutions with more publications did not extensively collaborate with each other (Figure 4D).
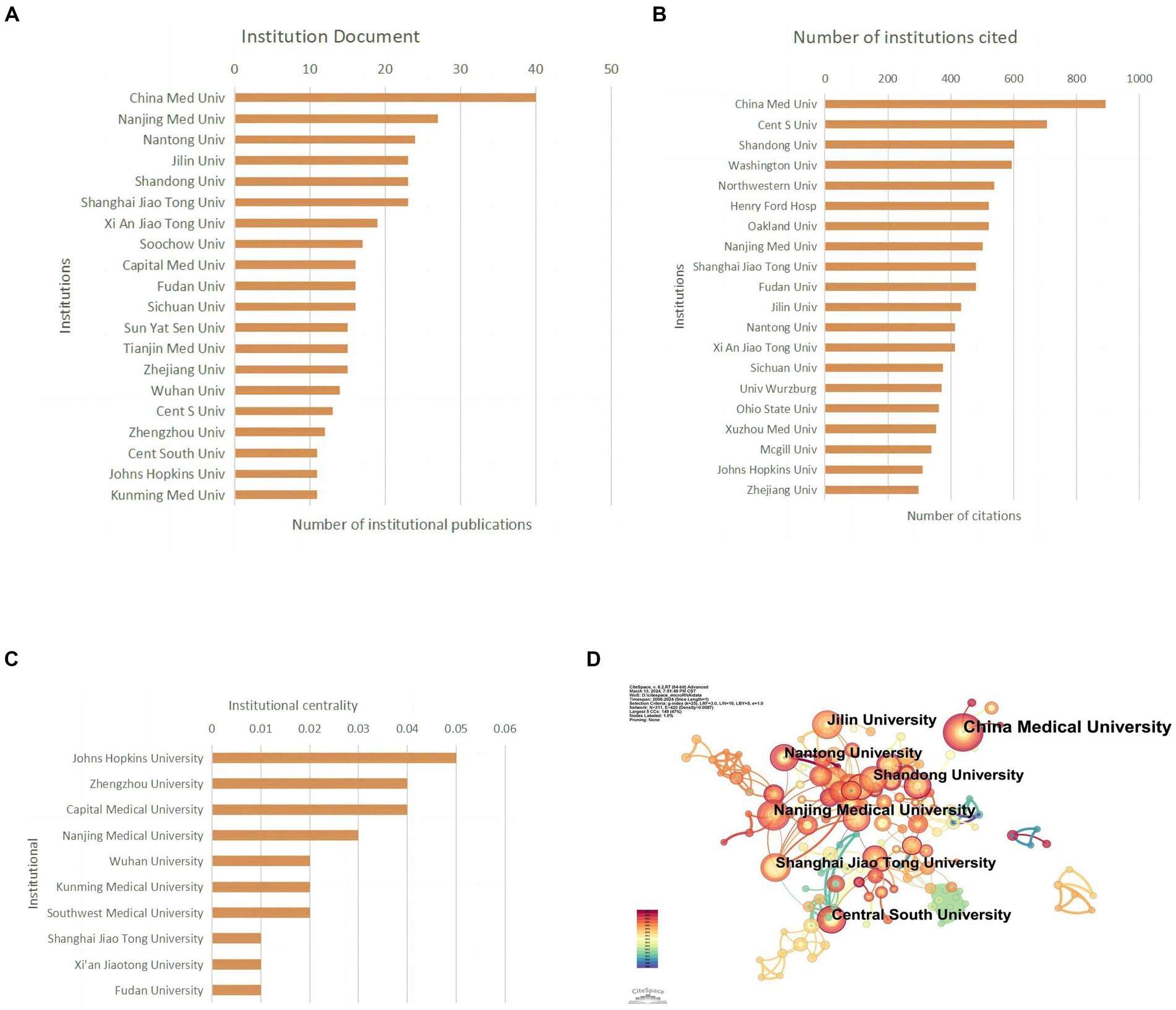
Figure 4. Institutional contribution on miRNAs in SCI research. (A) Top 10 institutions by publication volume. (B) Top 10 most cited institutions. (C) Top 10 intermediary centrality value of research institutions. (D) A visual network map of research institution contributions and collaborations.
Journal analysis
Related literature was published in 278 journals, with the most published article in European Review for Medical and Pharmacological Sciences (n = 25; 6.4%), the most cited journal in Experimental Neurology (n = 715; 6.9%) (Figures 5A,B; Table 3), and the highest H-index in European Review for Medical and Pharmacological Sciences (Figure 5C). Considering journal classification and influence factors, research quality needs further improvement. Analysis of superimposed journal graphs shows yellow bars indicate articles in molecular/biology/immunology journals often cite articles in molecular/biology/genetics journals (Figure 5D). Per cooperative network analysis, journals fell into four clusters, with the large node/density of European Review for Medical and Pharmacological Sciences, Frontiers in Molecular Neuroscience, and Neural Regeneration Research indicating these journals’ important roles in the field (Figures 5E,F).
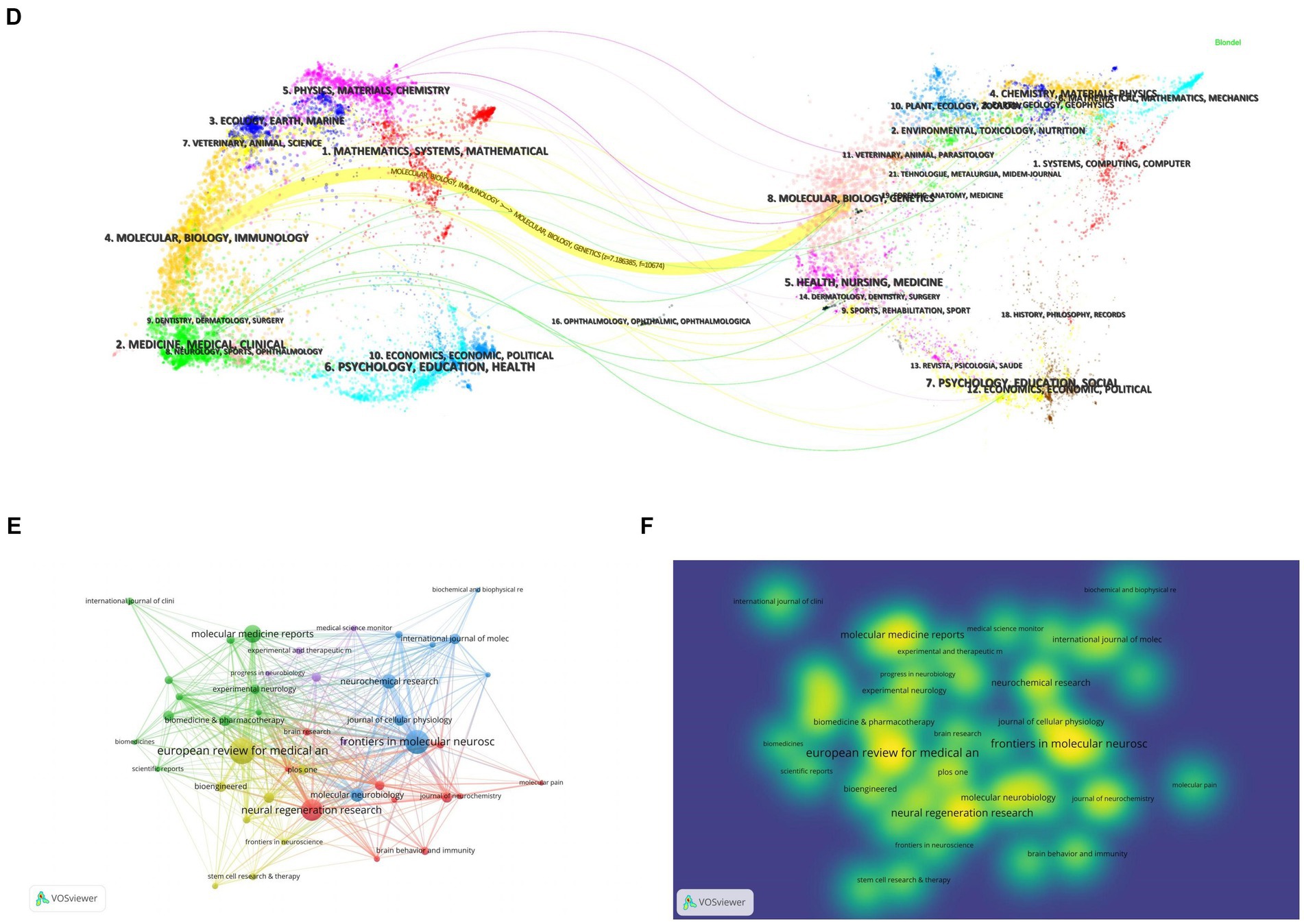
Figure 5. Journal characteristics of miRNAs and SCI-related research. (A) Top 10 journals by publication volume. (B) Top 10 most cited journals. (C) Top 10 journal H-index. (D) The dual-map overlay of journals in WOSCC. (E) Visual network map of core journals. (F) Visual density view of the core journals.
Author contribution and collaboration analysis
A total of 3,867 authors have published relevant papers, with Ma, H publishing the most papers (n = 11, 3.63%) and also being the most cited author (n = 357, 4.68%), indicating extensive research in this field (Figures 6A,B; Table 4). Wang, Y, with the highest H-index, is the most influential author (Figure 6C). Many academic groups are active in this field, such as Ma, H, Feng, SQ, He, XJ, Lu, HB, etc. Authors within groups collaborate closely, but inter-group collaboration is insufficient (Figure 6D).
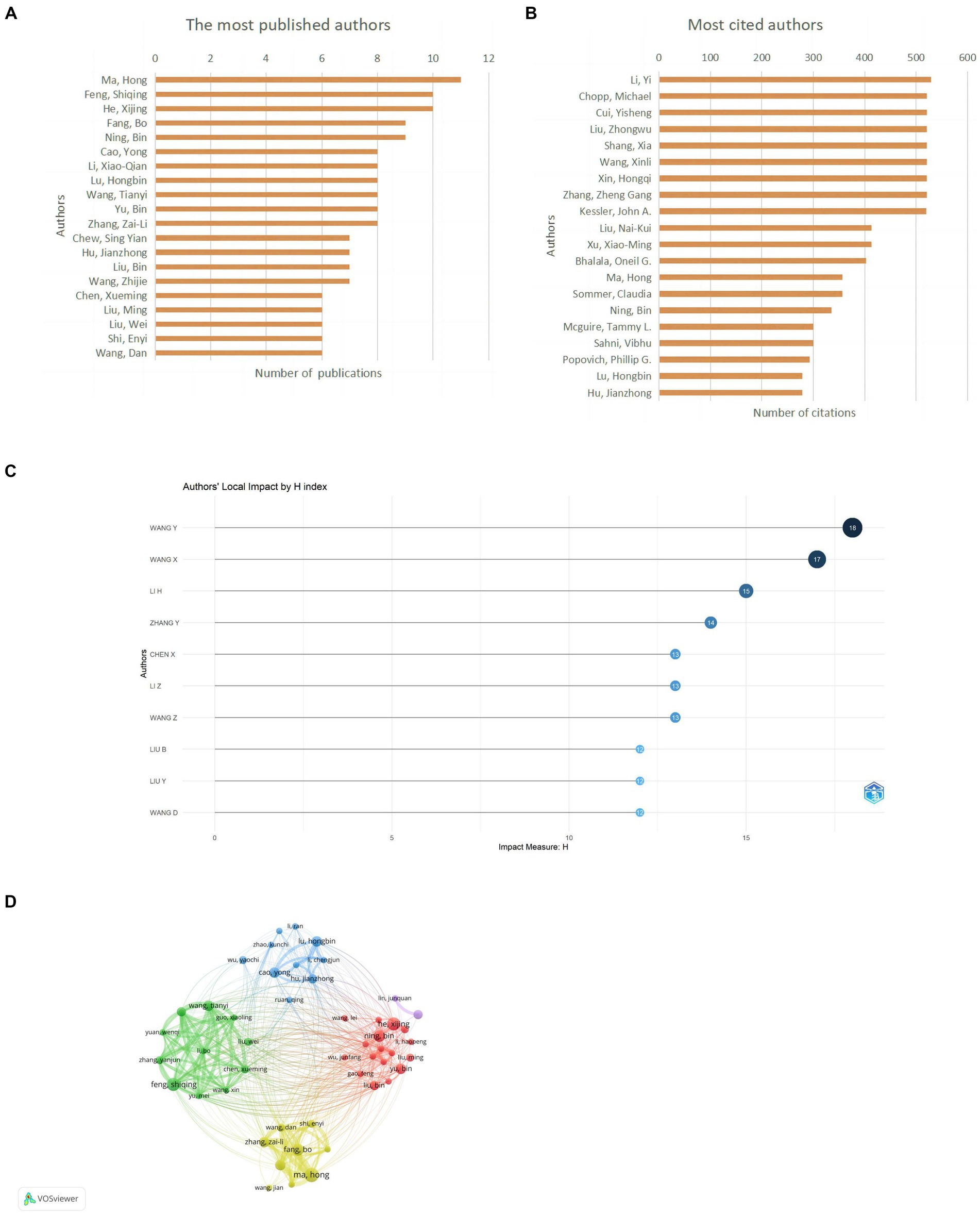
Figure 6. Author contribution on miRNAs in SCI research. (A) Top 10 authors by publication volume. (B) Top 10 most cited authors. (C) Top 10 author H-index. (D) A visual network map of author contributions and collaborations.
Analysis of highly cited articles and co-citation articles
Table 5 shows the top 10 cited publications, led by Xin HQ’s article in Stem Cells, with 521 citations. It reported Mir-133b promotes neural plasticity and recovery post-stroke treatment via transfer of exosome-enriched particles. Liu NK’s article in Experimental Neurology, “Altered microRNA expression post-traumatic spinal cord injury,” was cited 153 times (Table 6). VOSviewer software is used to draw the visual map and visual density view of the cited documents and co-cited documents in order to obtain better visual effect. Only references with citation frequency ≥ 40 are shown in the cited chart. Xin (2013) was cited by more literatures (Figures 7A,B). Only references with citation frequency ≥ 30 are displayed in the co-cited reference map. Liu NK (2009) and Bartel Dp (2004) have attracted wide attention (Figures 7C,D).
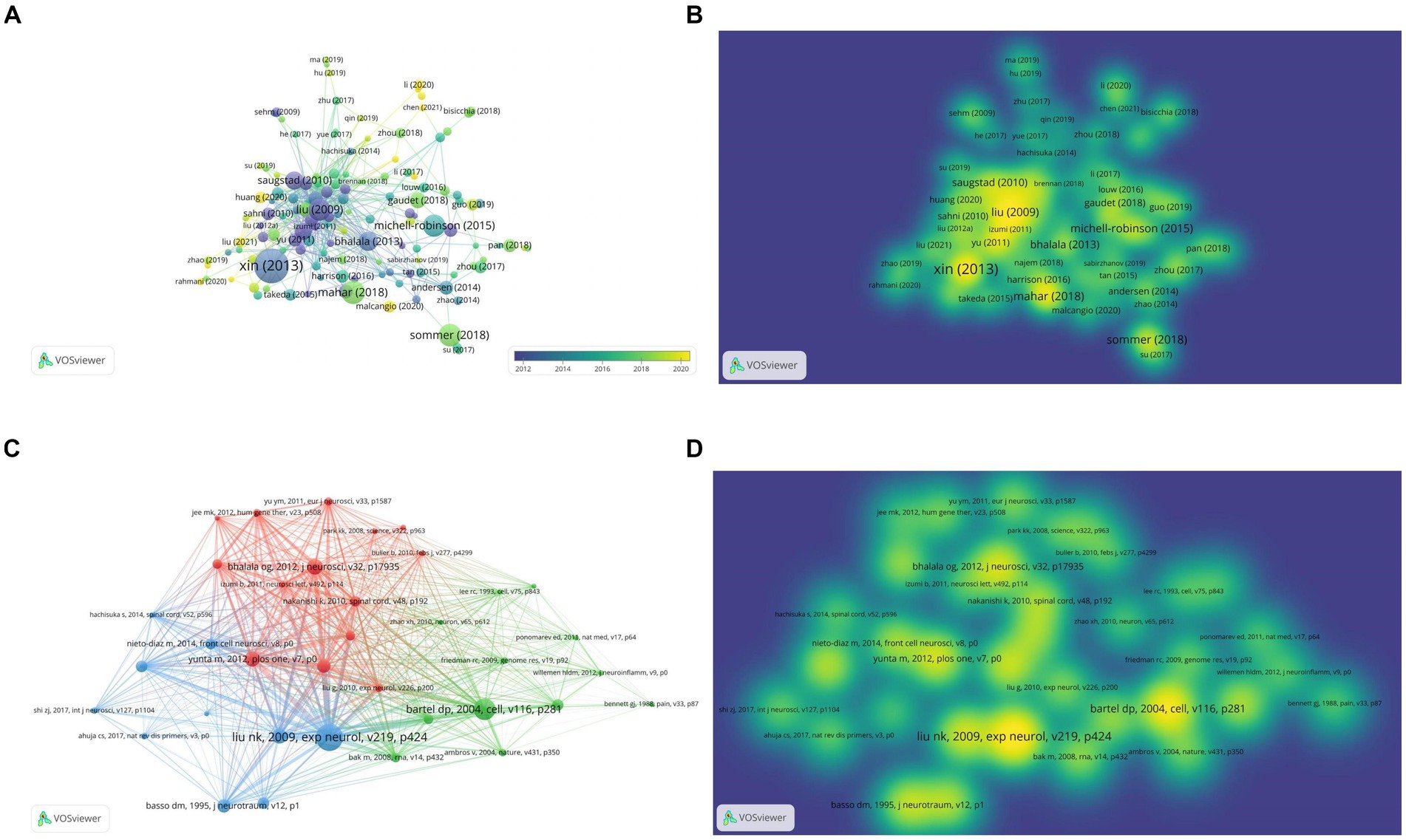
Figure 7. Characteristics of highly cited and co-citation articles on miRNAs and SCI. (A) Visual network diagram of the cited article. (B) Visual density view of the cited articles. (C) Visual network diagram of co-cited articles. (D) Visual density view of co-cited articles.
Keyword analysis
Keywords are words that have a high degree of generalization of the overall research content of an article. Through co-occurrence analysis of 3,151 keywords in all literatures, it was found that research mainly focuses on SCI, expression, apoptosis, microRNAs, etc. (Figure 8A). The LLR algorithm was used to perform cluster analysis on all keywords, drawing a cluster map with a total of nine clusters. The smaller the classification number, the more keywords that classification contains. #0 neuropathic pain, #1 central nervous system; each tag is interrelated and developed, rather than existing independently (Figure 8B). According to keyword co-occurrence timeline chart of the references, this development and change can also be confirmed from 2018 to 2019, SCI related research content is the richest (Figure 8C).
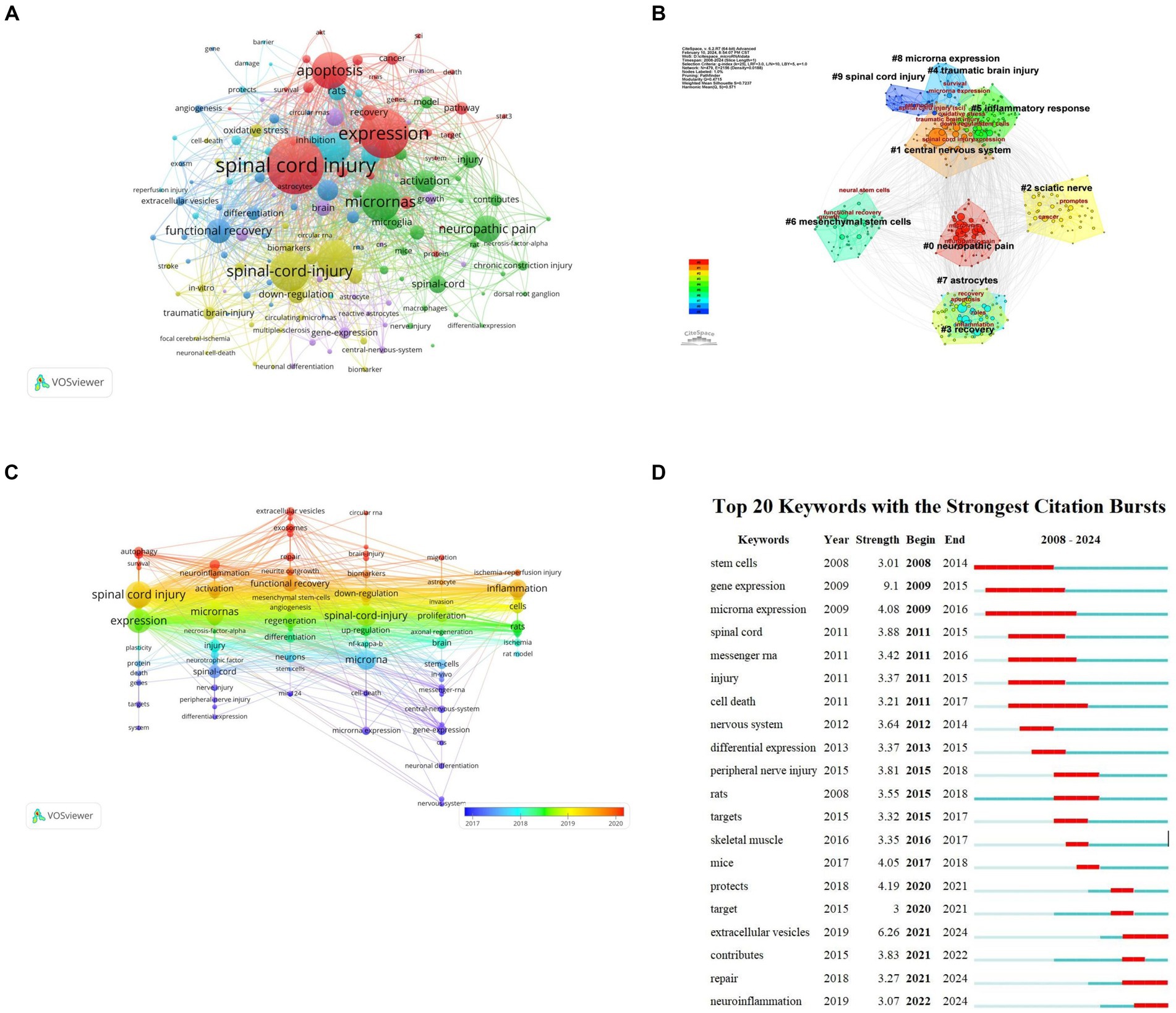
Figure 8. Keyword analysis of miRNAs and SCI research. (A) Keyword clustering network map. (B) Keyword clustering analysis. (C) Keyword co-occurrence timeline by keywords on miRNAs in SCI. (D) Top 20 Keyword with the strongest citation bursts on miRNAs in SCI.
Emergent words refer to keywords that appear within a short time or are used frequently reflecting a change in research direction within a field. Based on keyword intensity and duration research direction can be roughly divided into three stages: (1) 2008–2015 focusing on changes in RNA and related gene expression post spinal cord injury and stem cell therapy; (2) 2015–2018 focusing on peripheral nerve injury treatment and exploring in vivo SCI treatment in mice; and (3) 2018–2024 primarily studying extracellular vesicles for SCI repair and neuroinflammation alleviation. This development and change is also confirmed by the co-citation time chart of references. Keyword co-occurrence timeline reflects the richest SCI research content from 2018 to 2019 (Figure 8D).
Analysis of potential mechanisms based on the Arrowsmith project
We selected the keywords from the Arrowsmith project as the prediction group (n = 2,779) and the keywords extracted by VOSviewer as the confirmation group (n = 728). A Venn diagram showed 204 common keywords, which can serve as potential research directions for studying miRNAs in SCI (Figure 9A). We extracted genes corresponding to these potential keywords for Gene Ontology (GO) analysis and Kyoto Encyclopedia of Genes and Genomes (KEGG) pathway enrichment analysis. The GO analysis showed biological processes (BP) focused on cell responses to hormones, oxidative stress, phosphorus metabolism, growth factors, and oxidative stress. Cellular components (CC) were mainly concentrated in receptor complexes, transcriptional regulatory complexes, and vesicle cavities. Molecular functions (MF) were remarkably rich in transcription factor binding and kinase binding (Figure 9B). KEGG pathway enrichment analysis showed the PD-1/PD-L1 signaling pathway played a certain role (Figure 9C). A Protein–Protein Interaction (PPI) network was constructed and key genes were screened. It was found that AKT, MAPK, FOXO, and SMAD were potential targets related to miRNA treatment of SCI, indicating these genes played important roles in regulating cell pathophysiological processes. They are key players in SCI treatment (Figures 9D,E).
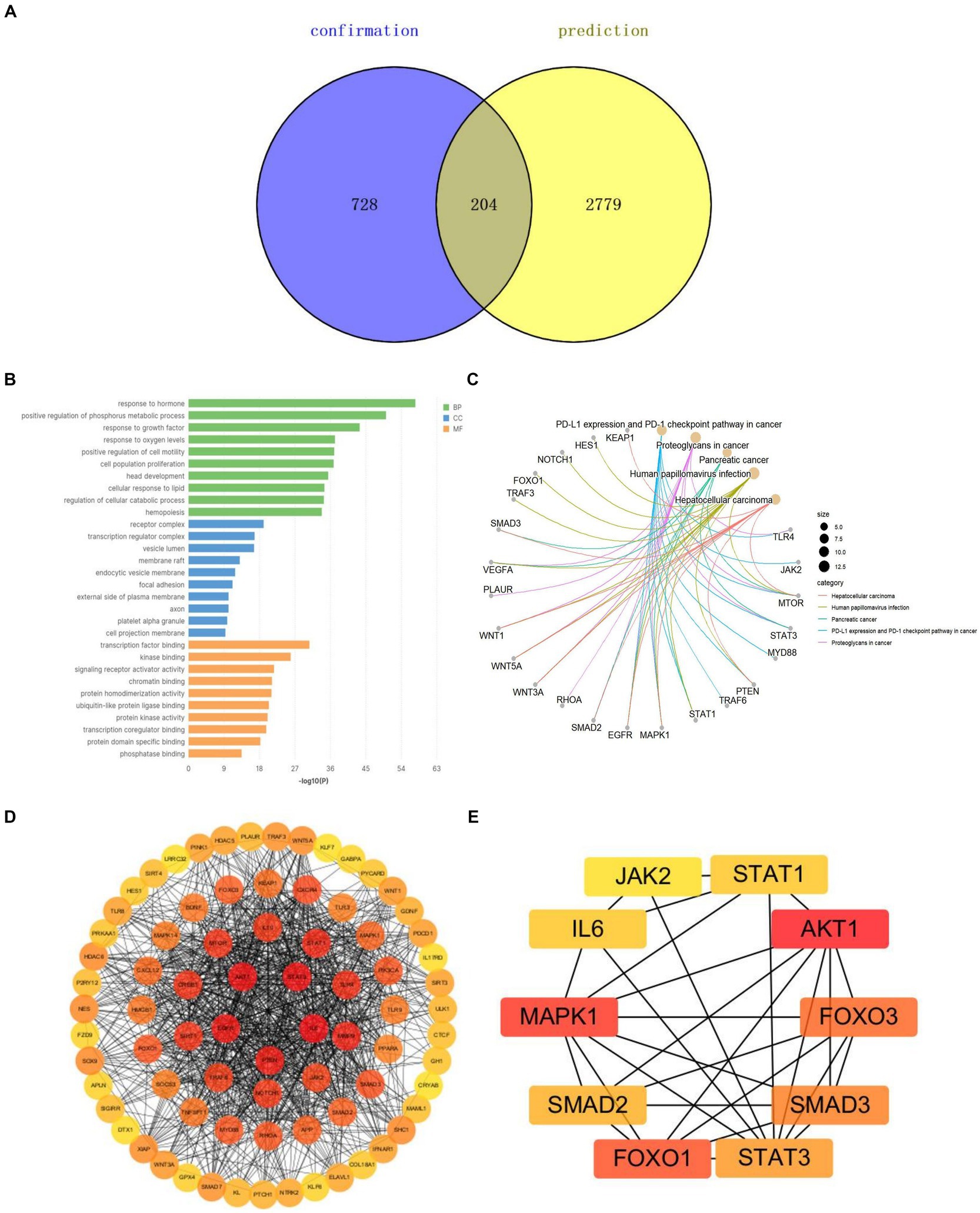
Figure 9. Potential keyword analysis of miRNAs and SCI research. (A) Venn diagrams for keywords of prediction and confirmation groups. (B) GO analysis of the potential key genes. (C) KEGG pathway enrichment analysis of the potential key genes. (D) PPI network of miRNA-related targets in SCI. (E) Hub genes of miRNA-related targets in SCI.
Discussion
Research progress on the effects of miRNAs on SCI
Spinal cord injury is a nervous system disease causing physical and psychological harm to patients and a heavy economic burden on society (17). It can be divided into primary and secondary injury. Primary injury is typically mechanical, while secondary injury can lead to insufficient perfusion of gray matter post-injury and spread to surrounding white matter (18, 19). Injury-induced oxidative stress, inflammation, glial or fibrotic scar formation, demyelination, and nerve injury can result in partial paralysis (20). Current research focuses on neuroprotection, neurorepair, and scar inhibition therapy. Neuroprotective therapies focus on reducing/preventing secondary damage (6). The goal of nerve repair therapy is to reshape neurovascular recovery of the nervous system (21). Scar inhibition is a potential strategy to control scar growth and promote axonal regeneration by inhibiting astrocyte proliferation and hypertrophy (22, 23). Current clinical treatments mainly involve surgical decompression and drug therapy. Surgical decompression can improve spinal cord compression, increase blood flow at the injury site, and reduce expansion. However, surgical treatment can lead to greater trauma and can only provide symptomatic relief rather than a fundamental cure (24, 25). Injectable drug therapy can also cause immune rejection, as well as excessive drug release and rapid degradation (26). In the context of medical treatment, the central objective is to mitigate hindrances to axon growth, ensuring a microenvironment that is conducive to nerve development. As part of this, there is an ongoing exploration of strategies that could potentially rebuild damaged neural circuits. Simultaneously, there is a concerted effort to promote functional recovery, enhancing the overall quality of life for patients. Lastly, there is a continuous search for suitable grafts that could support and guide the process of axon regeneration, ensuring optimal outcomes, miRNA-based therapy, blood vessel intervention and combination of multiple treatments will play a key role in the treatment of SCI in the future (27).
MicroRNAs are considered important biomarkers and therapeutic targets in SCI development (28). They exhibit tissue specificity and stability; miRNA content changes post-SCI are prerequisites for biological effects (29). MiRNAs serve as pathological biomarkers for Alzheimer’s disease, epilepsy, brain injury, and other conditions (30, 31). Bioinformatics analyses show abnormal miRNAs’ potential target genes post-SCI involve SCI pathogenesis, including inflammation, oxidative stress, apoptosis, and neuronal death (32). They also impact astrocytes and scar formation (33). Research on miRNAs and SCI can inform SCI diagnosis and treatment, but miRNAs’ pathophysiological significance remains incompletely determined. We use various bibliometrics tools for quantitative analysis, citation analysis, and visualization to reveal research hotspots and prospects. Compared to traditional review and meta-analysis, our approach explores research trends across multiple dimensions, providing richer, deeper, and more diversified information. It offers new reference and direction for SCI treatment and development.
General research trends on the role of miRNAs in SCI
Bibliometrics can systematically classify and analyze literatures on different topics, enabling better understanding of correlations between hot spots, trends, and topics within a research field (34). This study applies bibliometrics methods to examine literature on miRNAs’ role in SCI restoration. From 2008 to 2022, related literature rose yearly, totaling 754 articles. Publications remained high and entered rapid growth. Involving 50 countries/regions, 802 institutions, 278 journals, and 3,867 authors, indicating sustained researcher interest and a hot topic in SCI restoration.
China currently has clear advantages in terms of national paper count, citation count, institutional ranking, and author ranking. However, cooperative network analysis shows the United States occupies an important position in the cooperative network, with high H-index for research institutions, indicating the United States also plays an important role in promoting related work. China’s overall link strength in the cooperation network remains insufficient; international exchanges and cooperation should be strengthened. Ma, H and Feng, SQ from China are field leaders and may continue to hold leadership positions, but their research teams are primarily domestically based. Journal research shows European Review for Medical and Pharmacsciences is the most published journal, also with the highest H-index, while Experimental Neurology is the most cited. Considering journal division and impact factors, research quality in this field needs further improvement. Chinese research institutions and scholars can advance miRNA research in SCI recovery by strengthening international cooperation, introducing advanced methods and technologies, critically evaluating and improving research papers, and actively participating in academic conferences. Such efforts can enhance academic level and reputation, and contribute important scientific achievements to the field.
Hotspots and research trends on the role of miRNAs in SCI
Cited and co-cited documents refer to research in which one document cites another, and multiple documents cite the same one, respectively (35). Research references help scholars understand hotspots and frontiers in a field, identify key documents with important value, reveal academic networks, and strengthen knowledge dissemination. Combining literature contents in Tables 5, 6, we found inflammation, neuron protection, axon regeneration, scar formation, and specific mechanisms post-SCI remain research foci.
Keyword analysis extracts keywords or key phrases through processing and statistically analyzing large document, article, or data sets, enabling understanding of research topics, hotspots, and basic content. This study’s keywords are spinal cord injury, expression, apoptosis, and miRNAs. Changes in miRNA gene expression lead to increased apoptosis and improved SCI. This strategy remains a hot research topic. miR-7a treats SCI by inhibiting neuronal apoptosis and oxidative stress (36), while miR-487b inhibits inflammation and neuronal apoptosis in SCI by targeting Ifitm3 (37). Based on keyword cluster analysis, nerve injury closely relates to inflammation. Currently, combined with keyword co-occurrence timeline and Reference citation bursts, keywords gradually transition to extracellular vesicles and neuroinflammation. Long et al. reported astrocyte-derived exosomes rich in miR-873a-5p inhibit neuroinflammation post-traumatic brain injury through microglial phenotypic regulation (38). Future directions include exosomes carrying miRNAs to inhibit inflammation for SCI treatment.
According to GO analysis and KEGG pathway, miRNAs actively participate in pathophysiological activities post-SCI. The PD-1/PD-L1 signaling pathway is involved in inhibiting neuroinflammation and improving traumatic brain injury (39). PPI network predicts AKT, MAPK, and FOXO as important pivotal genes, potentially key in apoptosis, autophagy, and inflammation. Currently, these genes are widely studied in SCI research. miR-21-5p, miR-92b-3p, and miR-34a all inhibit neuronal apoptosis and promote nerve regeneration via AKT (40–42). Excessive miR-340-5p reduces spinal cord injury-induced neuroinflammation and apoptosis by modulating P38-MAPK signaling (43). FOXO is widely reported in anti-inflammatory and anti-cancer studies (44, 45). SMAD plays an important role in SCI remyelination (46). Elucidating miRNA-target gene regulatory mechanisms may achieve reliable therapeutic effects and more comprehensive, effective rehabilitation programs for SCI patients.
Prospects of therapeutic of miRNAs in SCI
At present, effective clinical treatment methods for SCI remain lacking, and emerging treatment methods such as cell transplantation, epigenetic regulation, artificial scaffold transplantation, and gene therapy are the main research directions for researchers (47, 48). Secondary injury usually leads to changes in the spinal cord microenvironment, resulting in changes in miRNA expression (49). This discovery provides potential opportunities for gene intervention (50). MiR-33-5p alleviates rat SCI and protects PC12 cells from lipopolysaccharide-induced apoptosis (51). MiR-433-5p overexpression protects against acute spinal cord injury by activating MAPK1 (52). MiR-7a improves spinal cord injury by inhibiting neuronal apoptosis and oxidative stress (36). Although the use of miRNAs as a therapeutic agent has attracted considerable attention due to its therapeutic effect on SCI, The limitations of using miRNAs as a therapeutic tool lie in their delivery and activation in vivo (29).
Exosomes, as intercellular communication carriers, play an irreplaceable role in maintaining multicellular organism functional integrity. Protected by a phospholipid layer, miRNAs can be accurately transported to target sites and avoid hydrolysis by extracellular enzymes, enhancing blood–brain barrier penetration and preventing misdelivery. Jiang et al. (53) confirmed neuron-derived exosome-delivered miR-124-3p protects spinal cord from traumatic injury by inhibiting neurotoxic microglia and astrocyte activation. Liu et al. (54) demonstrated hypoxic-preconditioned mesenchymal stem cell-derived exosome shuttle miR-216a-5p repaired traumatic spinal cord injury by altering microglia M1/M2 polarization. Recent miRNA delivery technology advances raise high expectations for miRNA therapeutics. While no clinical trials yet use exosome-attached miRNAs to treat human SCI, experimental data strongly support this approach’s feasibility. We must further analyze its beneficial effects and potential risks before applying it clinically.
Limitations
This study, for the first time, conducted systematic bibliometric and visual analysis on miRNA and SCI-related documents extracted from the WOSCC database. Based on the Arrowsmith project, we also revealed potential mechanisms between miRNA and SCI. The study’s results laid a foundation for further research in this field. However, the study also has limitations. First, the included database data is relatively simple, and some other data may be ignored. Additionally, excluding articles not in English may impact conclusions. The database used for this study is updated in real-time and does not include papers published after the search date, which may result in differences between results at publication time and this result.
Conclusion
The study highlights the global research interest, development trends and future clinical application potential of miRNAs in SCI treatment. We have confirmed the key role of miRNA in spinal cord injury and it has garnered continuous attention from researchers, becoming a hotspot of research in this field. Since 2008, annual publications in this field have increased. China leads in publication quantity, research institutions, and authors, but requires strengthened international collaboration. Current research focuses have shifted from single miRNA treatments to SCI treatment using exosome-attached miRNAs. In mechanism research, AKT, MAPK, FOXO, and SMAD are potential targets of miRNAs influencing SCI. There are relatively few clinical studies on miRNAs in SCI treatment. However, large-scale, multicenter clinical trials are needed to prove treatment effectiveness and safety.
Data availability statement
The original contributions presented in the study are included in the article/supplementary material, further inquiries can be directed to the corresponding authors.
Author contributions
BH: Methodology, Resources, Writing – original draft. YZ: Software, Visualization, Writing – original draft. CC: Conceptualization, Writing – original draft. BW: Investigation, Writing – original draft. HZ: Data curation, Writing – review & editing. BL: Supervision, Writing – review & editing. RZ: Validation, Writing – review & editing. FF: Validation, Writing – review & editing.
Funding
The authors declare that financial support was received for the research, authorship, and/or publication of this article. We thank the Science and Technology Program of the Joint Fund of Scientific Research for the Public Hospitals of Inner Mongolia Academy of Medical Sciences (No. 2023GLLH0379) for funding this research.
Acknowledgments
We thank to all clinicians and other hospital staff for their participation and contribution to this study.
Conflict of interest
The authors declare that the research was conducted in the absence of any commercial or financial relationships that could be construed as a potential conflict of interest.
Publisher’s note
All claims expressed in this article are solely those of the authors and do not necessarily represent those of their affiliated organizations, or those of the publisher, the editors and the reviewers. Any product that may be evaluated in this article, or claim that may be made by its manufacturer, is not guaranteed or endorsed by the publisher.
Footnotes
References
1. McDonald, JW, and Sadowsky, C. Spinal-cord injury. Lancet. (2002) 359:417–25. doi: 10.1016/S0140-6736(02)07603-1
2. DeVivo, MJ, Go, BK, and Jackson, AB. Overview of the national spinal cord injury statistical center database. J Spinal Cord Med. (2002) 25:335–8. doi: 10.1080/10790268.2002.11753637
3. Selassie, A, Cao, Y, and Saunders, LL. Epidemiology of traumatic spinal cord injury among persons older than 21 years: a population-based study in South Carolina, 1998-2012. Top Spinal Cord Inj Rehabil. (2015) 21:333–44. doi: 10.1310/sci2015-313
4. DeVivo, MJ. Causes and costs of spinal cord injury in the United States. Spinal Cord. (1997) 35:809–13. doi: 10.1038/sj.sc.3100501
5. Fiedler, IG, Laud, PW, Maiman, DJ, and Apple, DF. Economics of managed care in spinal cord injury. Arch Phys Med Rehabil. (1999) 80:1441–9. doi: 10.1016/S0003-9993(99)90256-3
6. Anjum, A, Yazid, MD’, Fauzi Daud, M, Idris, J, Ng, AMH, Selvi Naicker, A, et al. Spinal cord injury: pathophysiology, multimolecular interactions, and underlying recovery mechanisms. Int J Mol Sci. (2020) 21:7533. doi: 10.3390/ijms21207533
7. Diener, C, Keller, A, and Meese, E. Emerging concepts of miRNA therapeutics: from cells to clinic. Trends Genet. (2022) 38:613–26. doi: 10.1016/j.tig.2022.02.006
8. Lu, TX, and Rothenberg, ME. MicroRNA. J Allergy Clin Immunol. (2018) 141:1202–7. doi: 10.1016/j.jaci.2017.08.034
9. Ning, B, Gao, L, Liu, RH, Liu, Y, Zhang, NS, and Chen, ZY. microRNAs in spinal cord injury: potential roles and therapeutic implications. Int J Biol Sci. (2014) 10:997–1006. doi: 10.7150/ijbs.9058
10. Zhang, C, Talifu, Z, Xu, X, Liu, W, Ke, H, Pan, Y, et al. MicroRNAs in spinal cord injury: a narrative review. Front Mol Neurosci. (2023) 16:1099256. doi: 10.3389/fnmol.2023.1099256
11. Silvestro, S, and Mazzon, E. MiRNAs as promising translational strategies for neuronal repair and regeneration in spinal cord injury. Cells. (2022) 11:2177. doi: 10.3390/cells11142177
12. Wang, B, Shen, PF, Qu, YX, Zheng, C, Xu, JD, Xie, ZK, et al. miR-940 promotes spinal cord injury recovery by inhibiting TLR4/NF-κB pathway-mediated inflammation. Eur Rev Med Pharmacol Sci. (2019) 23:3190–7. doi: 10.26355/eurrev_201904_17677
13. Xu, Z, Zhang, K, Wang, Q, and Zheng, Y. MicroRNA-124 improves functional recovery and suppresses Bax-dependent apoptosis in rats following spinal cord injury. Mol Med Rep. (2019) 19:2551–60. doi: 10.3892/mmr.2019.9904
14. Zhiguo, F, Ji, W, Shenyuan, C, Guoyou, Z, Chen, K, Hui, Q, et al. A swift expanding trend of extracellular vesicles in spinal cord injury research: a bibliometric analysis. J Nanobiotechnol. (2023) 21:289. doi: 10.1186/s12951-023-02051-6
15. Wei, N, Xu, Y, Li, Y’, Shi, J, Zhang, X, You, Y, et al. A bibliometric analysis of T cell and atherosclerosis. Front Immunol. (2022) 13:948314. doi: 10.3389/fimmu.2022.948314
16. Xu, Y, Zhang, Z, He, J, and Chen, Z. Immune effects of macrophages in rheumatoid arthritis: a bibliometric analysis from 2000 to 2021. Front Immunol. (2022) 13:903771. doi: 10.3389/fimmu.2022.903771
17. Ahuja, CS, Nori, S, Tetreault, L, Wilson, J, Kwon, B, Harrop, J, et al. Traumatic spinal cord injury-repair and regeneration. Neurosurgery. (2017) 80:S9–s22. doi: 10.1093/neuros/nyw080
18. Yu, Q, Jiang, X, Liu, X, Shen, W, Mei, X, Tian, H, et al. Glutathione-modified macrophage-derived cell membranes encapsulated metformin nanogels for the treatment of spinal cord injury. Biomater Adv. (2022) 133:112668. doi: 10.1016/j.msec.2022.112668
19. Yang, B, Zhang, F, Cheng, F, Ying, L, Wang, C, Shi, K, et al. Strategies and prospects of effective neural circuits reconstruction after spinal cord injury. Cell Death Dis. (2020) 11:439. doi: 10.1038/s41419-020-2620-z
20. Hutson, TH, and Di Giovanni, S. The translational landscape in spinal cord injury: focus on neuroplasticity and regeneration. Nat Rev Neurol. (2019) 15:732–45. doi: 10.1038/s41582-019-0280-3
21. Kastner, A, and Matarazzo, V. Neuroprotective and Neurorestorative processes after spinal cord injury: the case of the Bulbospinal respiratory neurons. Neural Plast. (2016) 2016:7692602. doi: 10.1155/2016/7692602
22. Tran, AP, Warren, PM, and Silver, J. The biology of regeneration failure and success after spinal cord injury. Physiol Rev. (2018) 98:881–917. doi: 10.1152/physrev.00017.2017
23. Ahuja, CS, Wilson, JR, Nori, S, Kotter, MRN, Druschel, C, Curt, A, et al. Traumatic spinal cord injury. Nat Rev Dis Primers. (2017) 3:17018. doi: 10.1038/nrdp.2017.18
24. Fehlings, MG, and Perrin, RG. The role and timing of early decompression for cervical spinal cord injury: update with a review of recent clinical evidence. Injury. (2005) 36:S13–26. doi: 10.1016/j.injury.2005.06.011
25. Batchelor, PE, Wills, TE, Skeers, P, Battistuzzo, CR, Macleod, MR, Howells, DW, et al. Meta-analysis of pre-clinical studies of early decompression in acute spinal cord injury: a battle of time and pressure. PLoS One. (2013) 8:e72659. doi: 10.1371/journal.pone.0072659
26. Cai, M, Chen, L, Wang, T, Liang, Y, Zhao, J, Zhang, X, et al. Hydrogel scaffolds in the treatment of spinal cord injury: a review. Front Neurosci. (2023) 17:1211066. doi: 10.3389/fnins.2023.1211066
27. Zhou, R, Li, J, Wang, R, Chen, Z, and Zhou, F. The neurovascular unit in healthy and injured spinal cord. J Cereb Blood Flow Metab. (2023) 43:1437–55. doi: 10.1177/0271678X231172008
28. Hachisuka, S, Kamei, N, Ujigo, S, Miyaki, S, Yasunaga, Y, and Ochi, M. Circulating microRNAs as biomarkers for evaluating the severity of acute spinal cord injury. Spinal Cord. (2014) 52:596–600. doi: 10.1038/sc.2014.86
29. Rodrigues, LF, Moura-Neto, V, and TCLS, ES. Biomarkers in spinal cord injury: from prognosis to treatment. Mol Neurobiol. (2018) 55:6436–48. doi: 10.1007/s12035-017-0858-y
30. Shao, Y, and Chen, Y. Roles of circular RNAs in neurologic disease. Front Mol Neurosci. (2016) 9:25. doi: 10.3389/fnmol.2016.00025
31. Wang, K. The ubiquitous existence of MicroRNA in body fluids. Clin Chem. (2017) 63:784–5. doi: 10.1373/clinchem.2016.267625
32. Liu, NK, Wang, XF, Lu, QB, and Xu, XM. Altered microRNA expression following traumatic spinal cord injury. Exp Neurol. (2009) 219:424–9. doi: 10.1016/j.expneurol.2009.06.015
33. Wang, W, Liu, R, Su, Y, Li, H, Xie, W, and Ning, B. MicroRNA-21-5p mediates TGF-β-regulated fibrogenic activation of spinal fibroblasts and the formation of fibrotic scars after spinal cord injury. Int J Biol Sci. (2018) 14:178–88. doi: 10.7150/ijbs.24074
34. Cheng, K, Guo, Q, Yang, W, Wang, Y, Sun, Z, and Wu, H. Mapping knowledge landscapes and emerging trends of the links between bone metabolism and diabetes mellitus: a bibliometric analysis from 2000 to 2021. Front Public Health. (2022) 10:918483. doi: 10.3389/fpubh.2022.918483
35. Zhong, H, Zhou, Y, Mei, SY, Tang, R, Feng, JH, He, ZY, et al. Scars of COVID-19: a bibliometric analysis of post-COVID-19 fibrosis. Front Public Health. (2022) 10:967829. doi: 10.3389/fpubh.2022.967829
36. Ding, LZ, Xu, J, Yuan, C, Teng, X, and Wu, QM. MiR-7a ameliorates spinal cord injury by inhibiting neuronal apoptosis and oxidative stress. Eur Rev Med Pharmacol Sci. (2020) 24:11–7. doi: 10.26355/eurrev_202001_19890
37. Tong, D, Zhao, Y, Tang, Y, Ma, J, Wang, M, Li, B, et al. MiR-487b suppressed inflammation and neuronal apoptosis in spinal cord injury by targeted Ifitm3. Metab Brain Dis. (2022) 37:2405–15. doi: 10.1007/s11011-022-01015-3
38. Long, X, Yao, X, Jiang, Q, Yang, Y, He, X, Tian, W, et al. Astrocyte-derived exosomes enriched with miR-873a-5p inhibit neuroinflammation via microglia phenotype modulation after traumatic brain injury. J Neuroinflammation. (2020) 17:89. doi: 10.1186/s12974-020-01761-0
39. Gao, X, Li, W, Syed, F, Yuan, F, Li, P, and Yu, Q. PD-L1 signaling in reactive astrocytes counteracts neuroinflammation and ameliorates neuronal damage after traumatic brain injury. J Neuroinflammation. (2022) 19:43. doi: 10.1186/s12974-022-02398-x
40. Qi, L, Jiang-Hua, M, Ge-Liang, H, Qing, C, and Ya-Ming, L. MiR-34a inhibits spinal cord injury and blocks spinal cord neuron apoptosis by activating Phatidylinositol 3-kinase (PI3K)/AKT pathway through targeting CD47. Curr Neurovasc Res. (2019) 16:373–81. doi: 10.2174/1567202616666190906102343
41. Chen, Z, Li, Z, Jiang, C, Jiang, X, and Zhang, J. MiR-92b-3p promotes neurite growth and functional recovery via the PTEN/AKT pathway in acute spinal cord injury. J Cell Physiol. (2019) 234:23043–52. doi: 10.1002/jcp.28864
42. Lv, X, Liang, J, and Wang, Z. MiR-21-5p reduces apoptosis and inflammation in rats with spinal cord injury through PI3K/AKT pathway. Panminerva Med. (2020). 13:7–11. doi: 10.23736/S0031-0808.20.03974-9
43. Qian, Z, Chang, J, Jiang, F, Ge, D, Yang, L, Li, Y, et al. Excess administration of miR-340-5p ameliorates spinal cord injury-induced neuroinflammation and apoptosis by modulating the P38-MAPK signaling pathway. Brain Behav Immun. (2020) 87:531–42. doi: 10.1016/j.bbi.2020.01.025
44. Liu, Y, Ao, X, Ding, W, Ponnusamy, M, Wu, W, Hao, X, et al. Critical role of FOXO3a in carcinogenesis. Mol Cancer. (2018) 17:104. doi: 10.1186/s12943-018-0856-3
45. Wu, X, Niu, K, Wang, X, Zhao, J, Wang, H, Li, D, et al. microRNA-252 and FoxO repress inflammaging by a dual inhibitory mechanism on dawdle-mediated TGF-β pathway in Drosophila. Genetics. (2022) 220:iyab234. doi: 10.1093/genetics/iyab234
46. Han, T, Song, P, Wu, Z, Liu, Y, Ying, W, and Shen, C. Inflammation modifies miR-21 expression within neuronal extracellular vesicles to regulate Remyelination following spinal cord injury. Stem Cell Rev Rep. (2023) 19:2024–37. doi: 10.1007/s12015-023-10560-y
47. Ao, Q, Wang, AJ, Chen, GQ, Wang, SJ, Zuo, HC, and Zhang, XF. Combined transplantation of neural stem cells and olfactory ensheathing cells for the repair of spinal cord injuries. Med Hypotheses. (2007) 69:1234–7. doi: 10.1016/j.mehy.2007.04.011
48. Li, G, Che, MT, Zhang, K, Qin, LN, Zhang, YT, Chen, RQ, et al. Graft of the NT-3 persistent delivery gelatin sponge scaffold promotes axon regeneration, attenuates inflammation, and induces cell migration in rat and canine with spinal cord injury. Biomaterials. (2016) 83:233–48. doi: 10.1016/j.biomaterials.2015.11.059
49. Pinchi, E, Frati, A, Cantatore, S, D'Errico, S, Russa, R, Maiese, A, et al. Acute spinal cord injury: a systematic review investigating miRNA families involved. Int J Mol Sci. (2019) 20:1–26. doi: 10.3390/ijms20081841
50. Yan, H, Hong, P, Jiang, M, and Li, H. MicroRNAs as potential therapeutics for treating spinal cord injury. Neural Regen Res. (2012) 7:1352–9. doi: 10.3969/j.issn.1673-5374.2012.17.011
51. Li, Z, Rong, YL, and Zhang, YS. MiR-33-5p alleviates spinal cord injury in rats and protects PC12 cells from lipopolysaccharide-induced apoptosis. Kaohsiung J Med Sci. (2023) 39:52–60. doi: 10.1002/kjm2.12610
52. Zhou, CL, Li, F, Wu, XW, Cong, CL, Liu, XD, Tian, J, et al. Overexpression of miRNA-433-5p protects acute spinal cord injury through activating MAPK1. Eur Rev Med Pharmacol Sci. (2020) 24:2829–35. doi: 10.26355/eurrev_202003_20644
53. Jiang, D, Gong, F, Ge, X, Lv, C, Huang, C, Feng, S, et al. Neuron-derived exosomes-transmitted miR-124-3p protect traumatically injured spinal cord by suppressing the activation of neurotoxic microglia and astrocytes. J Nanobiotechnol. (2020) 18:105. doi: 10.1186/s12951-020-00665-8
Keywords: spinal cord injury, miRNAs, bibliometrics, inflammation, nerve
Citation: Hu B, Zhao Y, Chen C, Wu B, Zhang H, Liu B, Zheng R and Fang F (2024) Research hotspots and trends of microRNAs in spinal cord injury: a comprehensive bibliometric analysis. Front. Neurol. 15:1406977. doi: 10.3389/fneur.2024.1406977
Edited by:
Hideki Nakano, Kyoto Tachibana University, JapanReviewed by:
Zhou Zheng, Nanjing Medical University, ChinaSonglin Zhou, Nantong University, China
Junjie Dong, The First Affiliated Hospital of Kunming Medical University, China
Copyright © 2024 Hu, Zhao, Chen, Wu, Zhang, Liu, Zheng and Fang. This is an open-access article distributed under the terms of the Creative Commons Attribution License (CC BY). The use, distribution or reproduction in other forums is permitted, provided the original author(s) and the copyright owner(s) are credited and that the original publication in this journal is cited, in accordance with accepted academic practice. No use, distribution or reproduction is permitted which does not comply with these terms.
*Correspondence: Runquan Zheng, 337355033@qq.com; Fang Fang, FFspine2020@163.com
†These authors share first authorship