- 1Research Institute, Casa Colina Hospital and Centers for Healthcare, Pomona, CA, United States
- 2Institute of Advanced Consciousness Studies, Santa Monica, CA, United States
Background: Long COVID, also known as Post-COVID-19 syndrome, is characterized by multisystemic symptoms that persists for weeks to years beyond acute infection. It disproportionately affects women and those with pre-existing anxiety/depression, conditions more prevalent in females. The vagus nerve, with its extensive innervation and regulation of critical bodily functions, has become a focal point for therapeutic interventions. Transcutaneous vagus nerve stimulation (t-VNS) has emerged as a promising non-invasive treatment for COVID-19 conditions.
Methods: This pilot study assessed the efficacy of t-VNS in 24 female Long COVID patients (45.8 ± 11.7 years old; 20.2 ± 7.1 months since infection), who underwent a 10-day t-VNS intervention at home (30 min/session, twice a day). Cognition was considered the primary outcome, with anxiety, depression, sleep, fatigue, and smell as secondary outcomes. Outcomes were measured at baseline, post-intervention, and 1-month follow-up.
Results: Significant improvements were observed in various cognitive functions, anxiety, depression, and sleep at post-intervention, with benefits remaining or progressing at 1-month follow-up. Improvements in fatigue were delayed, reaching statistical significance at 1-month follow-up compared to baseline. No significant changes were noted in olfactory performance.
Conclusion: This pilot study provides preliminary evidence supporting the potential of t-VNS as a therapeutic intervention for female Long COVID patients. The encouraging results justify further rigorous investigation through larger, randomized controlled trials to confirm the efficacy of t-VNS, assess its generalizability to male cohorts, and explore biological markers to inform personalized treatment approaches. Our findings support the allocation of resources to conduct such trials and advance the understanding of t-VNS as a potential treatment for Long COVID.
1 Introduction
Post-COVID-19 syndrome, commonly known as Long COVID, is characterized by a constellation of symptoms that persist for weeks to years after initial COVID-19 infection. According to the World Health Organization, Long COVID is defined by the presence of new or continuing symptoms, usually 3 months from acute infection, lasting at least 2 months without an alternative diagnosis (1). Estimates of Long COVID prevalence vary widely, partly due to the variability in definition and samples studied. Prevalence estimates range from 7.5% to 41% in non-hospitalized adults and 37.6% in hospitalized adults (2). With its recent emergence, insights into Long COVID’s underlying mechanisms are limited, hindering the advancement of targeted treatments.
While the prevalence of COVID infection is similar for both sexes, accumulating evidence suggests that females are disproportionately affected by Long COVID (3) and are more likely than males to suffer from hyposmia/anosmia and neurological symptoms (4–7). Pre-existing conditions such as anxiety or depression, which are more prevalent in women, further heighten the risk of developing Long COVID (7). Although the exact mechanisms of Long COVID remain unclear, these observations have led to the hypothesis that Long COVID could be, in part, related to autoimmunity and persistent inflammation (8), which might explain the higher incidence of this syndrome in women, as immune response for both genetic and hormonal factors is stronger in women (9, 10).
Cognitive impairment (e.g., attention or memory issues), commonly reported as “brain fog,” is highly prevalent in Long COVID, affecting approximately 80% of long haulers (11). Neuroimaging studies have detected damages in various limbic and associative brain regions following COVID-19 infection, likely indicative of neuroinflammation and possible neurodegeneration (12, 13). Notably, chronic loss of smell correlates with cognitive decline and is an early predictor of Alzheimer’s disease (14). Female sex, as well as early presence of olfactory and neurological symptoms, are well-known risk factors for dementia and Alzheimer’s disease (15, 16). Thus, the intersection of female sex and post-COVID-19 could potentially exacerbate this vulnerability. Prompt and effective management of Long COVID symptoms, with an emphasis on cognitive deficits, is essential to potentially reduce the risk of long-term neurological sequelae.
The vagus nerve, a key component of the parasympathetic nervous system, plays a vital role in regulating various bodily functions, including heart rate, respiration, digestion, mood, immune response, and more (17). Vagus nerve stimulation (VNS), or vagal neuromodulation, has been utilized as a therapeutic intervention for a wide range of neurological and psychiatric disorders, expanding the scope of VNS beyond its initial indications for epilepsy and treatment-resistant depression (18–22). In the context of COVID-19, non-invasive VNS, such as transcutaneous auricular vagus nerve stimulation (t-VNS), has emerged as a potential adjunct therapy, with studies exploring its anti-inflammatory effects and its ability to reduce the burden of COVID-19 (23, 24). Considering the immense overlap between vagal functions and post-COVID-19 symptoms, the use of t-VNS could potentially mitigate the multi-organ dysfunction seen in long haulers. Indeed, a recent pilot study demonstrated that t-VNS could reverse many of the symptoms of Long COVID (25); however, the cognitive impacts were not thoroughly evaluated. Moreover, emerging research has illuminated sex-dependent autonomic responses to t-VNS (26), suggesting that females may experience different therapeutic effects compared to their male counterparts. This differential response is critical to consider, particularly in the context of Long COVID, where women are disproportionately affected. Such insights underscore the necessity of tailoring t-VNS protocols to optimize therapeutic outcomes across sexes. Due to limited resources and the pilot nature of this study, we focused on an exclusively female cohort to control for heterogeneity and maintain statistical power.
Therefore, the current prospective pilot study aims to investigate the efficacy of t-VNS in treating female patients with Long COVID, with an emphasis on cognitive impairments—a significant and debilitating aspect of post-COVID-19 symptomology. Secondary outcomes, including anxiety, depression, sleep, fatigue, and olfactory function, will also be assessed. By focusing on this particularly vulnerable group, our research seeks to not only alleviate the immediate burden of Long COVID but also to preemptively address the risk of subsequent neurodegenerative sequelae. Given the pressing need for effective interventions amidst Long COVID’s mounting public health burden, research focused on vulnerable patients who stand to benefit most is of critical importance.
2 Materials and methods
2.1 Participants
This study included 24 female patients (45.8 ± 11.7 years of age) with persistent Long COVID symptoms, with an average length of 20.2 ± 7.1 months since COVID-19 infection. The inclusion criteria for participants were: female sex, 18 years or older, and experiencing persistent (more than 3 months after infection) cognitive impairment (“brain fog”), such as attention or memory deficits. Exclusion criteria included contraindications for vagal neuromodulation or magnetic resonance imaging, pregnancy, Long COVID without cognitive impairment, and a history of neurological conditions. Among the participants, 16.7% had pre-existing asthma, and 37.5% had pre-existing anxiety or depression. These conditions have been identified as risk factors for developing Long COVID (3, 27). Medication information is included in Supplementary Figure S1 and Supplementary Table S1. Data were collected between March 2022 and January 2023. The patient enrollment process is delineated in the CONSORT flow chart provided as Figure 1. This study was approved by the Institutional Review Board at Casa Colina Hospital and Centers for Healthcare. All participants provided written informed consent prior to participation (Registered on clinicaltrials.gov as NCT05225220).
2.2 Study procedure
Participants underwent 10 consecutive days of auricular vagal neuromodulation therapy (AVNT) at the comfort of their home, twice a day, once in the morning and once in the evening, for 30 min per session (60 min/day). Treatment was delivered with the Parasym AVNT system (Parasym Health, London, United Kingdom), which was designed specifically for use in this patient population. The electrode was applied to the left tragus of the ear, stimulating the auricular branch of the vagus nerve, with micro-pulses of current a proprietary waveform with a pulse width of 250 μs and a pulse frequency of 25 Hz. The current intensity used was personalized by the participants based on their own sensitivity threshold, setting the correspondent level on an interval scale at each treatment session by slowly increasing the current intensity until a constant tingling sensation was felt. The mean output current intensity across treatments was 13.64 mA.
2.3 Outcome measures
Behavioral assessments were carried out at three timepoints: pre-intervention, post-intervention, and 1-month follow-up. Six participants were from out-of-state and had their 1-month follow-up assessments completed remotely.
2.3.1 Primary outcome measures
We used the NIH Cognitive toolbox to assess fluid cognition and included the following assessments: attention (Flanker Inhibitory Control and Attention Test [Flanker]), executive function (Dimensional Change Card Sort Test [DCCS]), episodic memory (Picture Sequencing Memory Test [PSM]), working memory (List Sorting Working Memory [LSWM]), and processing speed (Pattern Comparison Processing Speed [PCPS]). For the 1-month follow-up cognitive assessments, only LSWM and PSM were able to be administered remotely.
2.3.2 Secondary outcome measures
We additionally explored secondary outcomes related to anxiety (BURNS Anxiety Inventory [range 0–56]), depression (Becks Depression Inventory-II [range 0–63]), fatigue (Fatigue Severity Scale [range 9–63]), sleep (PROMIS Sleep Disturbance—Short Form [range 8–40]), and smell (Sniffin’ Sticks Test—12 items [range 0–12]).
2.4 Data analysis
The data were analyzed in JASP to first determine normality, followed by parametric or nonparametric tests as appropriate. Parametric repeated-measures ANOVA (3 timepoints) was carried out for anxiety, depression, and fatigue scores, whereas non-parametric Friedman’s test was used for cognition, sleep, and smell. Upon finding significance, we ran parametric (T-test) or nonparametric (Wilcoxon’s rank sum) pairwise comparisons with Bonferroni correction between the three timepoints on all the outcome measures. Cohen’s d was used to calculate the effect size for parametric tests and rank biserial correlation (r) for nonparametric tests. For analyzing changes in cognitive scores, the uncorrected standard score was used. Due to limitations in remote testing for six participants, only 18 participants completed all cognitive and smell assessments at follow-up.
In our dataset, a few participants submitted incomplete questionnaire responses. To address the resultant missing data, we employed data imputation using the k-nearest neighbors (kNN) function in RStudio (28). A k-value of 5 was used to conduct the imputations (29). This procedure led to the imputation of 9 values across various domains: anxiety (3), sleep (3), depression (2), and fatigue (1). To assess the k-value used for our kNN imputation, we evaluated the accuracy using the Normalized Root Mean Square Error (NRMSE) by comparing imputed values against a subset of the dataset where actual values were known but temporarily treated as missing. Additionally, we conducted an analysis excluding participants with missing data, and the results were consistent with the results obtained using imputed data.
3 Results
Participants were tested at three time points: pre-intervention (baseline), post-intervention, and 1-month follow-up after 10 days of vagal neuromodulation. The primary outcome was cognition (attention, executive function, working memory, episodic memory, and processing speed). Secondary outcomes included anxiety, depression, sleep disturbance, and fatigue. Bonferroni-adjusted p-values are reported for post hoc tests.
3.1 Baseline clinical symptoms
Prevalence of Long COVID symptoms at baseline are shown in Figure 2. The participants exhibited diverse symptoms across multiple systems. Following brain fog/cognitive impairment, which was an enrollment criterion, fatigue was the most common symptom, experienced by 79% of participants. Depression or anxiety and changes in smell or taste trailed behind (63%), then followed by headaches and sleep issues (58%). Please refer to Figure 2 for the extensive list of symptoms.
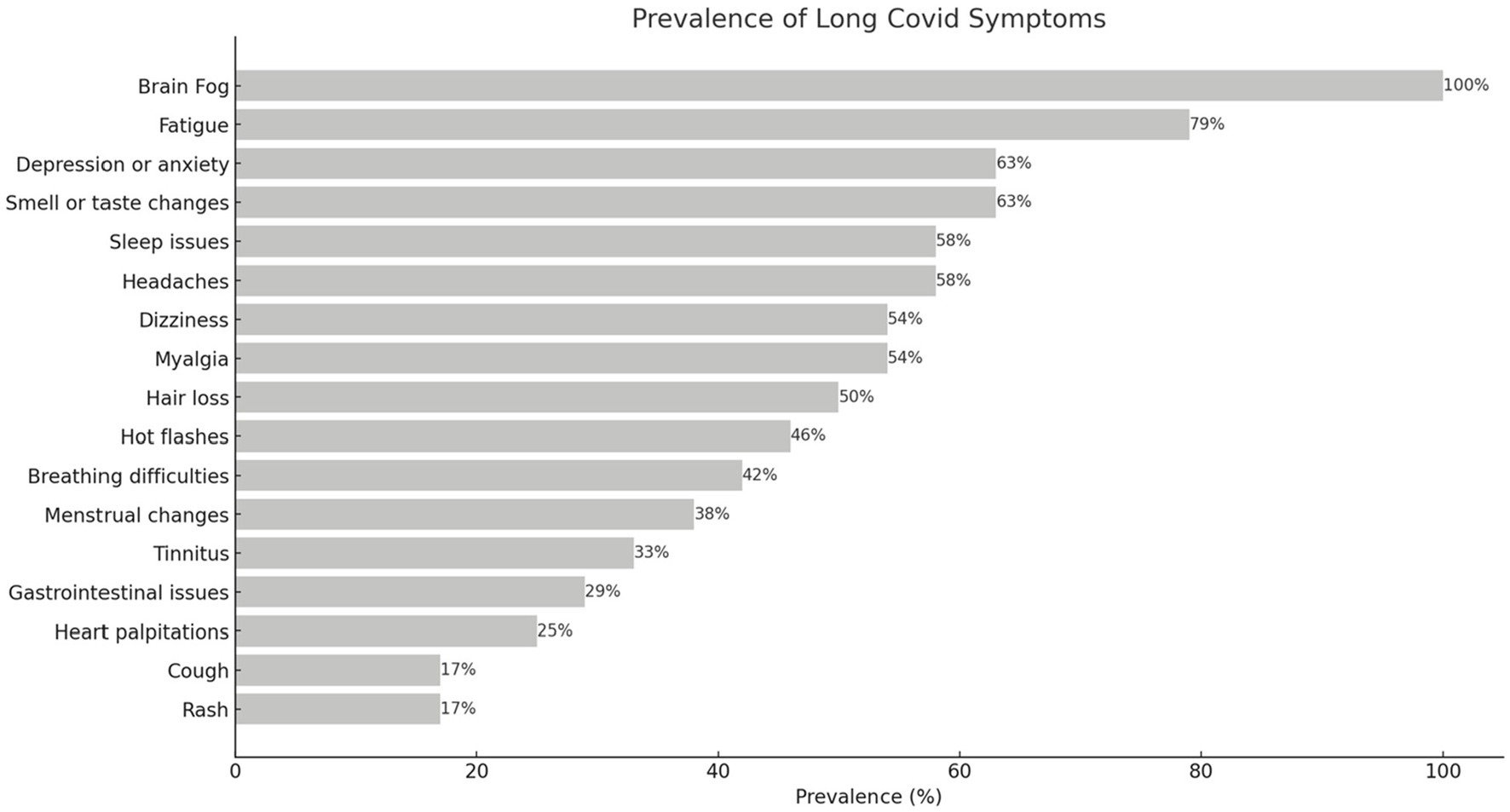
Figure 2. Prevalence of Long COVID symptoms at baseline. Brain fog (cognitive impairment) was an inclusion criterion; thus it is reported in all participants.
3.2 Primary outcome
Due to the absence of complete follow-up cognition data for six participants, Friedman’s test was run with n = 18 and revealed a main effect of time (p = 0.001) for fluid cognition (composite cognitive scores). Post hoc comparisons using Wilcoxon’s test with Bonferroni correction showed significant improvements from baseline to post-intervention (p = 0.003, r = 0.9) with further improvements at 1-month follow-up (pre vs. follow-up: p < 0.001, r = 0.98; post vs. follow-up: p = 0.003, r = 0.93) in composite fluid cognition. Given cognition was our primary outcome of interest, we further examined the cognitive subdomains. Significant gains were detected in Flanker Inhibitory Control and Attention from to pre to post (p = 0.009, r = 0.7) and from pre to follow-up (p < 0.001, r = 0.95). Moreover, there were significant increases from pre to post (p = 0.006, r = 0.86) and from pre to follow-up (p = 0.002, r = 0.94) in Pattern Comparison Processing Speed. Since Pattern Sequencing Memory (PSM) and List Sorting Working Memory (LSWM) contained the full dataset (n = 24) across all three timepoints and were normally distributed, they were analyzed with parametric tests. PSM scores significantly increased at post-intervention (p = 0.004, d = 0.55) and follow-up (p < 0.001, d = 0.82) when compared to baseline. However, LSWM only demonstrated improvements from baseline to follow-up (p = 0.029, d = 0.55). For Dimensional Change Card Sort test scores, a trending significance was found between pre-intervention and follow-up but did not survive multiple comparisons correction (p = 0.07). We additionally analyzed pre and post cognition scores available from all 24 patients and found similar results as the 18 patients but with slightly higher significance. For consistency and ease of visualization, the cognition graphs in Figure 3 reflect data from 18 patients, with the exception of PSM and LSWM (n = 24).
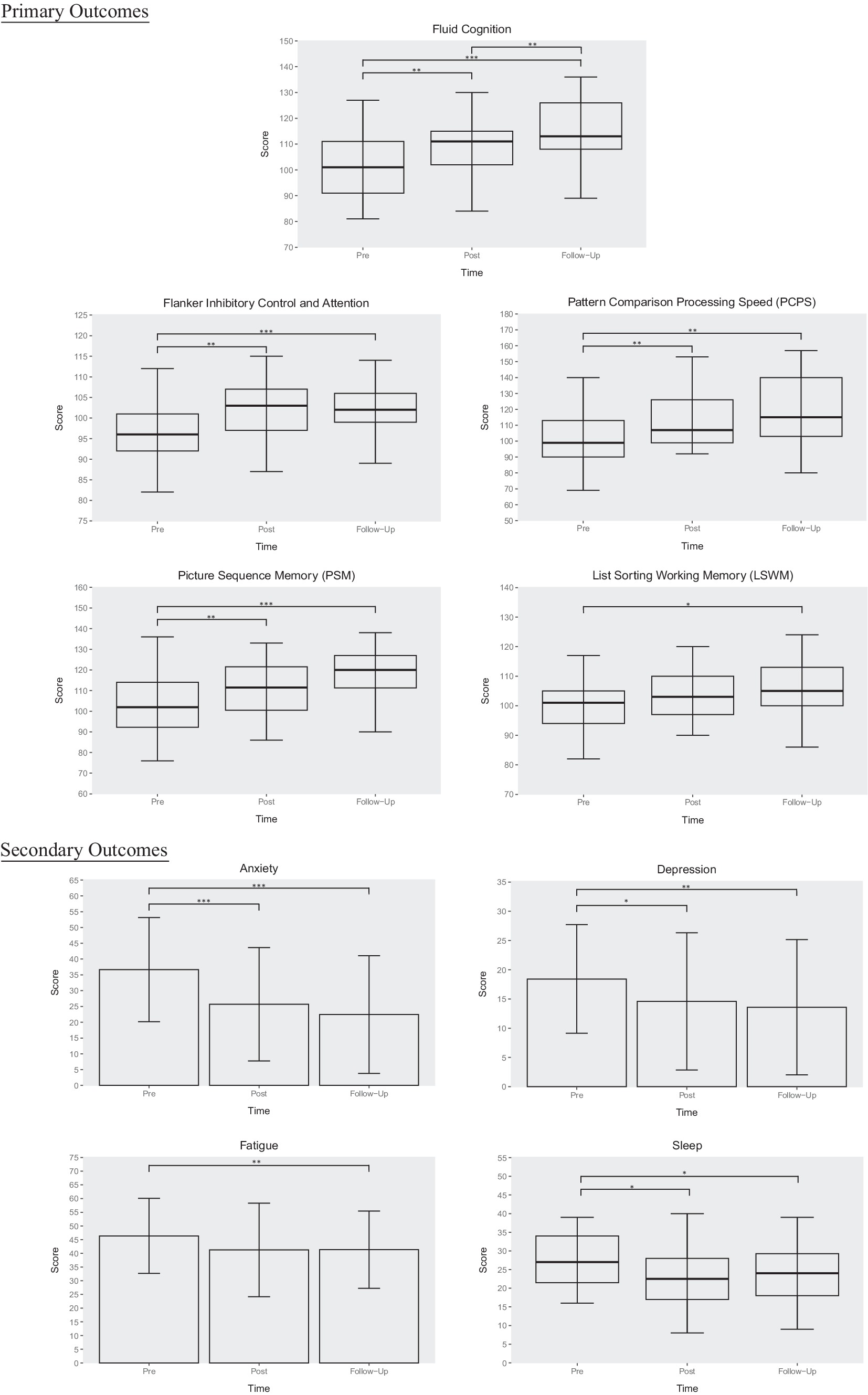
Figure 3. T-VNS intervention outcomes. This figure illustrates changes in cognition, anxiety, depression, fatigue, and sleep across three timepoints: pre-intervention, post-intervention, and 1-month follow-up. Boxplots are used for primarily nonparametric data, whereas column graphs depict parametric data. Error bars reflect standard error. *p < 0.05, **p < 0.01, ***p < 0.001.
3.3 Secondary outcomes
Results from 24 participants revealed a main effect of time for anxiety [F(2, 46) = 14.46, p < 0.001], depression [F(2, 46) = 7.05, p = 0.002], sleep (p = 0.02), and fatigue [F(1.43, 32.86) = 4.13, p = 0.037]. Post hoc testing using Bonferroni correction revealed significant improvements from baseline to post-intervention and 1-month follow-up for anxiety (pre vs. post: p = 0.001, d = 0.619; pre vs. follow-up: p < 0.001, d = 0.803), depression (pre vs. post: p = 0.025, d = 0.351; pre vs. follow-up: p = 0.009, d = 0.443), and sleep (pre vs. post: p = 0.036, r = 0.6; pre vs. follow-up: p = 0.021, r = 0.66). Fatigue improved from baseline to 1 month follow-up only (p = 0.005, d = 0.335). No significant changes in smell were detected.
4 Discussion
This pilot study provides preliminary evidence that transcutaneous vagus nerve stimulation may offer a promising non-invasive approach to managing multiple symptoms in female patients with post-COVID-19 condition (Long COVID). We observed significant improvements across cognitive performance, mood (anxiety and depression), sleep, and fatigue following 10 days of t-VNS. Although pre-post changes in fatigue did not meet statistical significance, fatigue continued to decrease at 1-month follow-up, eventually reaching significance. Similarly, all outcomes except smell exhibited sustained gradual improvements even after cessation of treatment. Limited research has examined the retention of benefits from non-invasive VNS; thus, our findings provide initial evidence of potential long-term benefits warranting further controlled investigation. However, t-VNS did not lead to significant changes in olfactory performance.
The vagus nerve is a mixed nerve with approximately 80% afferent (sensory) and 20% efferent axonal projections. Stimulation of the auricular branch is thought to activate these afferent pathways, which then signal to the nucleus of the solitary tract in the brainstem to project to other central structures, leading to a cascade of neurophysiological changes that may potentially produce therapeutic effects (21). While the mechanism of action for the therapeutic benefits of VNS is still under investigation, transcutaneous VNS has been shown to affect the same neural pathway as invasive VNS (30). Potential mechanisms underlying VNS efficacy could include attenuation of systemic inflammation (17, 18, 24), an increase in cerebral blood flow (31, 32), normalization of autonomic function (33), and modulation of neurotransmitters (34, 35). Additionally, vagal neuromodulation also influences the hypothalamic–pituitary–adrenal (HPA) axis, which controls the body’s response to stress and regulates many processes, including mood and energy levels (17). Notably, conditions such as depression and anxiety, which can exacerbate inflammatory states leading to more fatigue, may be ameliorated through these pathways (36).
Cognitive enhancements with moderate to large effect sizes spanned multiple domains including attention, processing speed, episodic memory, working memory, and overall fluid cognition. Previous research has shown improvements in various aspects of cognition following non-invasive VNS in healthy adults, including cognitive flexibility (37), action control (38), post-error slowing (39), and different types of memory (40, 41). Furthermore, t-VNS mediated cognitive enhancements have also been reported in patients with neurological and neuropsychiatric disorders (42–45). Additionally, we observed clinically meaningful reductions in symptoms of anxiety and depression. Prior studies corroborate the antidepressant and anxiolytic effects of t-VNS (22, 46–48). Furthermore, t-VNS also alleviated sleep disturbances and fatigue severity, consistent with previous reports in insomnia patients (35, 49).
The current findings extend the potential multifaceted benefits of VNS to individuals with Long COVID. The improvements in our study are also partially supported by existing COVID-19 studies. Non-invasive vagus nerve stimulation has demonstrated success in attenuating symptoms in acute and chronic COVID-19 conditions. Using a similar t-VNS protocol with the Parasym device, Verbanck et al. (25) also reported improvements in depression, fatigue, cognition, and sleep from a pilot study of 20 Long COVID patients. However, cognition and sleep scores were not evaluated using validated scales and were grouped together as part of a composite personal score along with other symptoms, precluding the delineation of individual symptom attenuations. Our research builds upon these initial findings, offering a granular analysis with validated scales that distinctly evaluates cognitive and sleep scores. Moreover, Badran et al. (50) investigated the efficacy of t-VNS in 13 long-haulers using a double-blind randomized controlled trial (RCT). Though they did not find significant quantitative differences (underpowered), they reported a qualitative trend of t-VNS improving more than sham in mental fatigue. Qualitative differences in memory and attention have also been reported in acute, hospitalized COVID-19 patients (n = 21), when comparing t-VNS with sham (24). The lack of significant quantitative differences observed in these RCT studies may be attributable to several factors, including the heterogeneous nature of the patient population, the limited statistical power arising from small sample sizes, and the potential sex differences in treatment response. By focusing on an exclusively female cohort, our study aims to control for heterogeneity and provide detailed insights into the therapeutic responses of female long haulers to t-VNS. While our research cannot directly compare sex-dependent responses due to the absence of a male cohort, the therapeutic effects of t-VNS observed in women—who are disproportionately affected by Long COVID—may also extend to male patients. This targeted approach lays the groundwork for future comparative studies to explore potential sex differences in treatment responses.
As an initial pilot investigation, the lack of a control group limits definitive conclusions regarding the efficacy of t-VNS for Long COVID symptom management. However, the moderate to large effect sizes observed make significant placebo effects less likely. Additionally, robust randomized controlled trials of VNS have demonstrated significant benefits in various neurological and psychiatric conditions (51–53), which implies that the observed benefits in our study are less likely to be solely attributed to placebo effects. Though spontaneous recovery cannot be excluded given the lack of a control group, the chronicity of symptoms at enrollment (average 20.2 months post-infection) renders spontaneous recovery in a short 3-week period less likely. Moving forward, large, double blind, randomized, sham-controlled trials with extended follow-up should further evaluate t-VNS efficacy. Our promising pilot results provide preliminary evidence to justify additional rigorous evaluation and larger investments for such trials.
In conclusion, this pilot study suggests that transcutaneous vagal nerve stimulation may be a potential therapeutic approach for ameliorating a spectrum of persistent symptoms in females with Long COVID. Notably, we observed significant changes in cognition, mood, sleep, and fatigue, with potential long-term retention of benefits. However, due to the lack of a control group, we cannot exclude the possibility of other factors contributing to the observed improvements. To substantiate the efficacy of t-VNS and to address the limitations of our pilot study, subsequent research should include larger, double-blind, randomized, sham-controlled trials with extended follow-up periods. While our study did not encompass male participants, the robust responses in our female cohort hint at the potential universality of t-VNS benefits, laying the groundwork for future research to explore and validate these effects across sexes. Future investigations delving into the biological underpinnings of t-VNS’s therapeutic action can help pave the way for precision medicine approaches in Long COVID management.
Data availability statement
The raw data supporting the conclusions of this article will be made available by the authors, without undue reservation.
Ethics statement
The studies involving humans were approved by Institutional Review Board at Casa Colina Hospital and Centers for Healthcare. The studies were conducted in accordance with the local legislation and institutional requirements. The participants provided their written informed consent to participate in this study.
Author contributions
ZZ: Conceptualization, Data curation, Formal analysis, Funding acquisition, Investigation, Methodology, Project administration, Resources, Supervision, Validation, Visualization, Writing – original draft, Writing – review & editing. NS: Data curation, Formal analysis, Visualization, Writing – review & editing. JW: Data curation, Investigation, Methodology, Writing – review & editing. ER: Project administration, Resources, Writing – review & editing.
Funding
The author(s) declare that financial support was received for the research, authorship, and/or publication of this article. This work was supported by the Norris Foundation.
Conflict of interest
The authors declare that the research was conducted in the absence of any commercial or financial relationships that could be construed as a potential conflict of interest.
Publisher’s note
All claims expressed in this article are solely those of the authors and do not necessarily represent those of their affiliated organizations, or those of the publisher, the editors and the reviewers. Any product that may be evaluated in this article, or claim that may be made by its manufacturer, is not guaranteed or endorsed by the publisher.
Supplementary material
The Supplementary material for this article can be found online at: https://www.frontiersin.org/articles/10.3389/fneur.2024.1393371/full#supplementary-material
References
1. Soriano, JB, Murthy, S, Marshall, JC, Relan, P, and Diaz, JVWHO Clinical Case Definition Working Group on Post-COVID-19 Condition. A clinical case definition of post-COVID-19 condition by a Delphi consensus. Lancet Infect Dis. (2022) 22:e102–7. doi: 10.1016/S1473-3099(21)00703-9
2. Nittas, V, Gao, M, West, EA, Ballouz, T, Menges, D, Wulf Hanson, S, et al. Long COVID through a public health Lens: an umbrella review. Public Health Rev. (2022) 43:1604501. doi: 10.3389/phrs.2022.1604501
3. Bai, F, Tomasoni, D, Falcinella, C, Barbanotti, D, Castoldi, R, Mulè, G, et al. Female gender is associated with long COVID syndrome: a prospective cohort study. Clin Microbiol Infect. (2021) 28:611.e9–611.e16. doi: 10.1016/j.cmi.2021.11.002
4. Lechien, JR, Chiesa-Estomba, CM, De Siati, DR, Horoi, M, Le Bon, SD, Rodriguez, A, et al. Olfactory and gustatory dysfunctions as a clinical presentation of mild-to-moderate forms of the coronavirus disease (COVID-19): a multicenter European study. Eur Arch Otorrinolaringol. (2020) 277:2251–61. doi: 10.1007/s00405-020-05965-1
5. Meng, X, Deng, Y, Dai, Z, and Meng, Z. COVID-19 and anosmia: a review based on up-to-date knowledge. Am J Otolaryngol. (2020) 41:102581. doi: 10.1016/j.amjoto.2020.102581
6. Sudre, CH, Murray, B, Varsavsky, T, Graham, MS, Penfold, RS, Bowyer, RC, et al. Attributes and predictors of long COVID. Nat Med. (2021) 27:626–31. doi: 10.1038/s41591-021-01292-y
7. Yong, SJ. Long COVID or post-COVID-19 syndrome: putative pathophysiology, risk factors, and treatments. Infect Dis. (2021) 53:737–54. doi: 10.1080/23744235.2021.1924397
8. Peluso, MJ, Lu, S, Tang, AF, Durstenfeld, MS, Ho, H-E, Goldberg, SA, et al. Markers of immune activation and inflammation in individuals with Postacute sequelae of severe acute respiratory syndrome coronavirus 2 infection. J Infect Dis. (2021) 224:1839–48. doi: 10.1093/infdis/jiab490
9. Rubtsov, AV, Rubtsova, K, Kappler, JW, and Marrack, P. Genetic and hormonal factors in female-biased autoimmunity. Autoimmun Rev. (2010) 9:494–8. doi: 10.1016/j.autrev.2010.02.008
10. Taneja, V. Sex Hormones Determine Immune Response. Front Immunol. (2018) 9:1931. doi: 10.3389/fimmu.2018.01931
11. Graham, EL, Clark, JR, Orban, ZS, Lim, PH, Szymanski, AL, Taylor, C, et al. Persistent neurologic symptoms and cognitive dysfunction in non-hospitalized Covid-19 long haulers. Ann Clin Transl Neurol. (2021) 8:5:1073–85. doi: 10.1002/acn3.51350
12. Douaud, G, Lee, S, Alfaro-Almagro, F, Arthofer, C, Wang, C, McCarthy, P, et al. SARS-CoV-2 is associated with changes in brain structure in UK biobank. Nature. (2022) 604:697–707. doi: 10.1038/s41586-022-04569-5
13. Thomasson, M, Voruz, P, Cionca, A, Jacot de Alcântara, I, Nuber-Champier, A, Allali, G, et al. Markers of limbic system damage following SARS-CoV-2 infection. Brain Commun. (2023) 5:fcad177. doi: 10.1093/braincomms/fcad177
14. Vilarello, BJ, Jacobson, PT, Tervo, JP, Waring, NA, Gudis, DA, Goldberg, TE, et al. Olfaction and neurocognition after COVID-19: a scoping review. Front Neurosci. (2023) 17:1198267. doi: 10.3389/fnins.2023.1198267
15. Rahman, A, Jackson, H, Hristov, H, Isaacson, RS, Saif, N, Shetty, T, et al. Sex and gender driven modifiers of Alzheimer’s: the role for estrogenic control across age, race, medical, and lifestyle risks. Front Aging Neurosci. (2019) 11:315. doi: 10.3389/fnagi.2019.00315
16. Zou, Y, Lu, D, Liu, L, Zhang, H, and Zhou, Y. Olfactory dysfunction in Alzheimer’s disease. Neuropsychiatr Dis Treat. (2016) 12:869–75. doi: 10.2147/NDT.S104886
17. Breit, S, Kupferberg, A, Rogler, G, and Hasler, G. Vagus nerve as modulator of the brain-gut Axis in psychiatric and inflammatory disorders. Front Psych. (2018) 9:44. doi: 10.3389/fpsyt.2018.00044
18. Bonaz, B, Sinniger, V, and Pellissier, S. Anti-inflammatory properties of the vagus nerve: potential therapeutic implications of vagus nerve stimulation. J Physiol. (2016) 594:5781–90. doi: 10.1113/JP271539
19. Boon, P, Moors, I, De Herdt, V, and Vonck, K. Vagus nerve stimulation and cognition. Seizure. (2006) 15:259–63. doi: 10.1016/j.seizure.2006.02.014
20. Engineer, ND, Kimberley, TJ, Prudente, CN, Dawson, J, Tarver, WB, and Hays, SA. Targeted Vagus nerve stimulation for rehabilitation after stroke. Front Neurosci. (2019) 13:280. doi: 10.3389/fnins.2019.00280
21. Howland, RH. Vagus nerve stimulation. Curr Behav Neurosci Rep. (2014) 1:64–73. doi: 10.1007/s40473-014-0010-5
22. Kong, J, Fang, J, Park, J, Li, S, and Rong, P. Treating depression with transcutaneous auricular Vagus nerve stimulation: state of the art and future perspectives. Front Psych. (2018) 9:20. doi: 10.3389/fpsyt.2018.00020
23. Tornero, C, Pastor, E, Garzando, M, Orduña, J, Forner, MJ, Bocigas, I, et al. Non-invasive Vagus Nerve Stimulation for COVID-19: Results From a Randomized Controlled Trial (SAVIOR I). Front Neurol. (2022) 13:864. doi: 10.3389/fneur.2022.820864
24. Uehara, L, Corrêa, JCF, Ritti, R, Leite, P, de Faria, DRG, Pacheco-Barrios, K, et al. Transcutaneous auricular vagus nerve stimulation effects on inflammatory markers and clinical evolution of patients with COVID-19: a pilot randomized clinical trial. Expert Rev Med Devices. (2022) 19:915–20. doi: 10.1080/17434440.2022.2154147
25. Verbanck, C. (2021). Transcutaneous Auricular Vagus Nerve Stimulation (tVNS) can Reverse the Manifestations of the Long-COVID Syndrome: A Pilot Study. Available at: https://www.semanticscholar.org/paper/Transcutaneous-Auricular-Vagus-Nerve-Stimulation-of-Verbanck-Corazza/8a39f3f621d92576369d988a59bf617e3935cf36
26. Yokota, H, Edama, M, Hirabayashi, R, Sekine, C, Otsuru, N, Saito, K, et al. Effects of stimulus frequency, intensity, and sex on the autonomic response to transcutaneous Vagus nerve stimulation. Brain Sci. (2022) 12:1038. doi: 10.3390/brainsci12081038
27. Tsampasian, V, Elghazaly, H, Chattopadhyay, R, Debski, M, Naing, TKP, Garg, P, et al. Risk factors associated with post−COVID-19 condition: a systematic review and Meta-analysis. JAMA Intern Med. (2023) 183:566–80. doi: 10.1001/jamainternmed.2023.0750
28. Troyanskaya, O, Cantor, M, Sherlock, G, Brown, P, Hastie, T, Tibshirani, R, et al. Missing value estimation methods for DNA microarrays. Bioinformatics. (2001) 17:520–5. doi: 10.1093/bioinformatics/17.6.520
29. Huang, J, Keung, JW, Sarro, F, Li, Y-F, Yu, YT, Chan, WK, et al. Cross-validation based K nearest neighbor imputation for software quality datasets: an empirical study. J Syst Softw. (2017) 132:226–52. doi: 10.1016/j.jss.2017.07.012
30. Yap, JYY, Keatch, C, Lambert, E, Woods, W, Stoddart, PR, and Kameneva, T. Critical review of transcutaneous Vagus nerve stimulation: challenges for translation to clinical practice. Front Neurosci. (2020) 14:284. doi: 10.3389/fnins.2020.00284
31. Fang, J, Egorova, N, Rong, P, Liu, J, Hong, Y, Fan, Y, et al. Early cortical biomarkers of longitudinal transcutaneous vagus nerve stimulation treatment success in depression. NeuroImage Clin. (2017) 14:105–11. doi: 10.1016/j.nicl.2016.12.016
32. Vonck, K, De Herdt, V, Bosman, T, Dedeurwaerdere, S, Van Laere, K, and Boon, P. Thalamic and limbic involvement in the mechanism of action of vagus nerve stimulation, a SPECT study. Seizure. (2008) 17:699–706. doi: 10.1016/j.seizure.2008.05.001
33. Clancy, JA, Mary, DA, Witte, KK, Greenwood, JP, Deuchars, SA, and Deuchars, J. Non-invasive Vagus nerve stimulation in healthy humans reduces sympathetic nerve activity. Brain Stimul. (2014) 7:871–7. doi: 10.1016/j.brs.2014.07.031
34. Carreno, FR, and Frazer, A. Vagal nerve stimulation for treatment-resistant depression. Neurotherapeutics. (2017) 14:716–27. doi: 10.1007/s13311-017-0537-8
35. Jiao, Y, Guo, X, Luo, M, Li, S, Liu, A, Zhao, Y, et al. Effect of transcutaneous Vagus nerve stimulation at auricular concha for insomnia: a randomized clinical trial. Evid Based Complement Alternat Med. (2020) 2020:e6049891:1–7. doi: 10.1155/2020/6049891
36. Lee, C-H, and Giuliani, F. The role of inflammation in depression and fatigue. Front Immunol. (2019) 10:1696. doi: 10.3389/fimmu.2019.01696
37. Borges, U, Knops, L, Laborde, S, Klatt, S, and Raab, M. Transcutaneous Vagus nerve stimulation May enhance only specific aspects of the Core executive functions. A randomized crossover trial. Front Neurosci. (2020) 14:523. doi: 10.3389/fnins.2020.00523
38. Jongkees, BJ, Immink, MA, Finisguerra, A, and Colzato, LS. Transcutaneous Vagus nerve stimulation (tVNS) enhances response selection during sequential action. Front Psychol. (2018) 9:1159. doi: 10.3389/fpsyg.2018.01159
39. Sellaro, R, van Leusden, JWR, Tona, K-D, Verkuil, B, Nieuwenhuis, S, and Colzato, LS. Transcutaneous Vagus nerve stimulation enhances post-error slowing. J Cogn Neurosci. (2015) 27:2126–32. doi: 10.1162/jocn_a_00851
40. Jacobs, HIL, Riphagen, JM, Razat, CM, Wiese, S, and Sack, AT. Transcutaneous vagus nerve stimulation boosts associative memory in older individuals. Neurobiol Aging. (2015) 36:1860–7. doi: 10.1016/j.neurobiolaging.2015.02.023
41. Jandackova, V, Vasendova, V, Jackowska, M, and Koenig, J. The effect of long-term non-invasive vagus nerve stimulation on cognitive performance: results from a randomized placebo controlled trial. Brain Stimul. (2023) 16:6. doi: 10.1016/j.brs.2023.03.028
42. Aniwattanapong, D, List, JJ, Ramakrishnan, N, Bhatti, GS, and Jorge, R. Effect of Vagus nerve stimulation on attention and working memory in neuropsychiatric disorders: a systematic review. Neuromodulation. (2022) 25:343–55. doi: 10.1016/j.neurom.2021.11.009
43. Broncel, A, Bocian, R, Kłos-Wojtczak, P, Kulbat-Warycha, K, and Konopacki, J. Vagal nerve stimulation as a promising tool in the improvement of cognitive disorders. Brain Res Bull. (2020) 155:37–47. doi: 10.1016/j.brainresbull.2019.11.011
44. Giraudier, M, Ventura-Bort, C, and Weymar, M. Transcutaneous Vagus nerve stimulation (tVNS) improves high-confidence recognition memory but not emotional word processing. Front Psychol. (2020) 11:1276. doi: 10.3389/fpsyg.2020.01276
45. Vargas-Caballero, M, Warming, H, Walker, R, Holmes, C, Cruickshank, G, and Patel, B. Vagus nerve stimulation as a potential therapy in early Alzheimer’s disease: a review. Front Hum Neurosci. (2022) 16:866434. doi: 10.3389/fnhum.2022.866434
46. George, MS, Ward, HE, Ninan, PT, Pollack, M, Nahas, Z, Anderson, B, et al. A pilot study of vagus nerve stimulation (VNS) for treatment-resistant anxiety disorders. Brain Stimul. (2008) 1:112–21. doi: 10.1016/j.brs.2008.02.001
47. Grolaux, PJD. Transcutaneous Vagus nerve stimulation in private healthcare center: a small-scale investigation targeting anxiety, irritable bowel syndrome and chronic pain. J Neurol Neuromed. (2019) 4:7–22. doi: 10.29245/2572.942X/2019/5.1251
48. Hein, E, Nowak, M, Kiess, O, Biermann, T, Bayerlein, K, Kornhuber, J, et al. Auricular transcutaneous electrical nerve stimulation in depressed patients: a randomized controlled pilot study. J Neural Transm. (2013) 120:821–7. doi: 10.1007/s00702-012-0908-6
49. Wu, D, Wang, J, and Yuan, Y. Effects of transcranial direct current stimulation on naming and cortical excitability in stroke patients with aphasia. Neurosci Lett. (2015) 589:115–20. doi: 10.1016/j.neulet.2015.01.045
50. Badran, BW, Huffman, SM, Dancy, M, Austelle, CW, Bikson, M, Kautz, SA, et al. A pilot randomized controlled trial of supervised, at-home, self-administered transcutaneous auricular vagus nerve stimulation (taVNS) to manage long COVID symptoms. Bioelectron Med. (2022) 8:13. doi: 10.1186/s42234-022-00094-y
51. Cimpianu, C-L, Strube, W, Falkai, P, Palm, U, and Hasan, A. Vagus nerve stimulation in psychiatry: a systematic review of the available evidence. J Neural Transm. (2017) 124:145–58. doi: 10.1007/s00702-016-1642-2
52. Vonck, K, Raedt, R, Naulaerts, J, De Vogelaere, F, Thiery, E, Van Roost, D, et al. Vagus nerve stimulation…25 years later! What do we know about the effects on cognition? Neurosci Biobehav Rev. (2014) 45:63–71. doi: 10.1016/j.neubiorev.2014.05.005
Keywords: Long COVID, transcutaneous vagus nerve stimulation (t-VNS), female health, mood and cognition, sleep, fatigue, neuromodulation
Citation: Zheng ZS, Simonian N, Wang J and Rosario ER (2024) Transcutaneous vagus nerve stimulation improves Long COVID symptoms in a female cohort: a pilot study. Front. Neurol. 15:1393371. doi: 10.3389/fneur.2024.1393371
Edited by:
Ursula S. Hofstoetter, Medical University of Vienna, AustriaReviewed by:
Ivan Chakalov, University Medical Center Göttingen, GermanyJianghai Ruan, The Affiliated Hospital of Southwest Medical University, China
Copyright © 2024 Zheng, Simonian, Wang and Rosario. This is an open-access article distributed under the terms of the Creative Commons Attribution License (CC BY). The use, distribution or reproduction in other forums is permitted, provided the original author(s) and the copyright owner(s) are credited and that the original publication in this journal is cited, in accordance with accepted academic practice. No use, distribution or reproduction is permitted which does not comply with these terms.
*Correspondence: Zhong Sheng Zheng, YXpoZW5nQGNhc2Fjb2xpbmEub3Jn