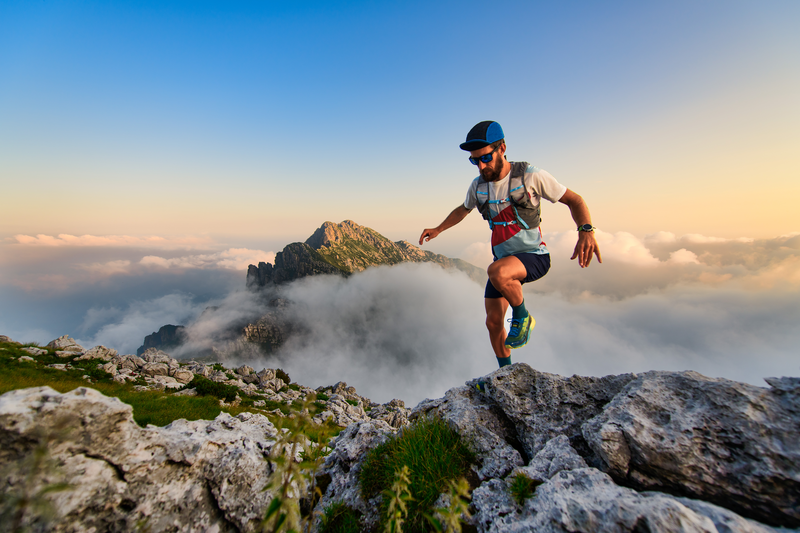
95% of researchers rate our articles as excellent or good
Learn more about the work of our research integrity team to safeguard the quality of each article we publish.
Find out more
ORIGINAL RESEARCH article
Front. Neurol. , 30 May 2024
Sec. Epilepsy
Volume 15 - 2024 | https://doi.org/10.3389/fneur.2024.1392977
This article is part of the Research Topic Epilepsy Mortality: Leading Causes of Death, Co-morbidities, Cardiovascular Risk and Prevention, Volume II View all 9 articles
Patients with epilepsy are prone to cognitive decline, depression, anxiety and other behavioral disorders. Cognitive comorbidities are particularly common and well-characterized in people with temporal lobe epilepsy, while inconsistently addressed in epileptic animals. Therefore, the aim of this study was to ascertain whether there is good evidence of cognitive comorbidities in animal models of epilepsy, in particular in the rat pilocarpine model of temporal lobe epilepsy. We searched the literature published between 1990 and 2023. The association of spontaneous recurrent seizures induced by pilocarpine with cognitive alterations has been evaluated by using various tests: contextual fear conditioning (CFC), novel object recognition (NOR), radial and T-maze, Morris water maze (MWM) and their variants. Combination of results was difficult because of differences in methodological standards, in number of animals employed, and in outcome measures. Taken together, however, the analysis confirmed that pilocarpine-induced epilepsy has an effect on cognition in rats, and supports the notion that this is a valid model for assessment of cognitive temporal lobe epilepsy comorbidities in preclinical research.
For many patients with epilepsy, a good quality of life and mental condition are as important as seizure control. In clinical practice, this interdisciplinary issue is often underappreciated and in epilepsy preclinical research the situation is not better. However, there is a notable rise of the awareness for neuropsychiatric comorbidities of epilepsy and the number of clinical (1, 2) and preclinical studies (3, 4) of comorbidities in epilepsy seems to grow over the years. Many clinical reports indicate that mental disorders are more common in patients with epilepsy than in the general population (5–8). In this regard, mood disorders are the most common, anxiety disorders the second most frequent and psychotic disorders the least frequent in people with temporal lobe epilepsy (5), psychoses being a little more widespread than personality disorders (6). Such high prevalence of psychiatric disorders is frequently demonstrated among patients with epilepsy when compared them with the general population or with individuals presenting non-epileptic neurological conditions (7), even if it may vary a lot due to differences in study methods and heterogeneity of epilepsy syndromes (8). More in detail, the estimated prevalence of depressive disorders in patients with epilepsy ranges from 13% to 35%, compared to less that 4% in the general population (9); the prevalence of anxiety disorders in people with epilepsy may reach 40%, compared to 3% in the general population (10); the prevalence of psychotic disorders is 5.6%–5.9% in people with epilepsy vs. 0.3% in people without it (6, 11). Besides mood and psychotic disorders, people with epilepsy suffer from cognitive decline and related attention deficit or learning and memory disabilities (12). The percentages are higher in older adults, in which the prevalence of cognitive disorders may reach 60% (12, 13). However, such studies typically include individuals with chronic epilepsy unresponsive to drug treatment (14, 15), which can make difficult the interpretation of the results. Attention deficit–hyperactivity disorders are observed in 28%–70% of people with epilepsy and are more common among children with epilepsy (16). The same problems, which worsen the quality of life of people with epilepsy, may be seen in the animals and appear in various preclinical reports.
Many scientific publications support the notion that an inadequate control of seizures may lead to changes in mood and cognition both in people with epilepsy and in laboratory animals (3, 17–19). In general, animal models allow the study of comorbidities without the bias of drug treatments (20–27). Between animal models useful for the study of epilepsy comorbidities, the pilocarpine model in rats is one of the most employed. This model mimics well the natural course of the temporal lobe epilepsy (28), which permits to monitor the development of psychiatric comorbidities in the different phases of the disease (i.e., the latency and chronic phase). Pilocarpine is a cholinergic agonist injected systemically or locally to induce status epilepticus (SE), which is followed by a latent period and the subsequently appearance of spontaneous recurrent seizures that mimics a focal epileptic condition similar to the human temporal lobe epilepsy (28). Besides the pilocarpine model, other animal models of acquired epilepsy like kainate, kindling, or traumatic brain injury are used to study comorbidities of epilepsy (3, 29). Absence models, like genetic generalized epilepsy with absence seizures like Genetic Absence Epilepsy Rats from Strasbourg (GAERS) or WAG/Rij rats have been employed for the same reason (29).
The term “cognition” can be defined as the mental action or process of acquiring knowledge and understanding through thought, experience, and the senses (30). To examine it in epilepsy, researchers use various behavioral tests assessing the starting point and evolving state of cognitive functions (e.g., learning, memory, and attention) in association with frequency of seizures or epileptic-like events. Different behavioral tests may provide different types of information. When employing contextual fear conditioning, for example, not only the memory of fear but also the context of experience is tested, so the results may be interpreted in terms of episodic memory on one hand and in terms of spatial memory on the other. Such multi-response testing can be useful in some cases but may also complicate the interpretation of the results. In addition, the outcome of learning/memory behavioral tests in animals will depend not only on the type of memory solicited, but also on the duration of the task, on the continuity or discontinuity of the procedures, on the duration of habituation and on many further factors (31). Behavioral tests of learning and memory are nearly always performed when studying epilepsy related cognitive dysfunction. Social behavior and social interaction tests are used less frequently (3, 29). Different learning and memory behavioral tasks may be applied separately or as a battery. When used in sequence, a logic order of tests (usually used from the least to the most stressful, in order to decrease the chance that behavioral responses are altered by prior test history) helps the researchers to better interpret their results in epileptic animals (32). A cognitive evaluation is typically run after testing locomotor activity and anxiety and before testing depression, which closes most of behavioral testing series. Concerning the cognitive ability tests only, their precise order in batteries may not be crucial, as the performance of animals in the different cognitive models seems to be independent of each other (33, 34). More important will be to compare the results obtained at the same disease stage.
Currently available tasks for studying learning and memory impairment in any neurobiological disease, epilepsy included, may be classified in different ways. One option is considering the prevailing type of memory engaged by the task (Figure 1; Table 1). In this respect (i) tests primarily measuring contextual memory include contextual and fear conditioning (CFC) and its variations, like contextual discrimination or delayed nonmatching to sample, conditioned taste aversion and social transmission of food preference test; (ii) tasks soliciting the recognition memory tests include the novel object recognition (NOR) test, the novel object preference (NOP) test and their variations; (iii) tests engaging mostly the working memory include the different mazes (T-maze, Y-maze, eight-arm radial maze); (iv) finally, tests analyzing principally the spatial memory include the Morris water maze (MWM) and the Barnes maze tests and, to some extent, the object location test (OLT) and the place preference test (PPT), in which the engagement of spatial memory is combined with contextual and working memory. It should be emphasized that this classification is not rigid. Most frequently, more than one type of memory is solicited in each cognitive task. Moreover, it is possible in many tasks to investigate only the recent, recall or remote memory. All in all, the complete spectrum of information provided by each test should be taken into account when choosing them and interpreting their results in epilepsy models. It should be also considered that, when working with epileptic animals, the situation is even more complex due to the natural progression of the disease and unpredictability of seizure occurrence.
The aim of the present study was to overview the currently available and most utilized behavioral tests employed to study cognitive impairment in temporal lobe epilepsy, and to evaluate if there is good enough evidence for cognitive/mental comorbidities in the rat pilocarpine model. Although some of these tests may have sex specific outcomes or require sex specific settings, the large majority of the papers identified by our search employed only male rats, preventing a proper evaluation of sex-dependency.
For this review, we searched PubMed and Web of Science for any pilocarpine or lithium-pilocarpine model in rats; we selected records or original articles (reviews were excluded) written in English and published in the years 1990–2023. We used the following keywords: pilocarpine, model, rats, epilepsy, memory, fear conditioning, cued conditioning, conditional fear, contextual fear, conditioned taste aversion, Morris water maze, radial arm maze, Y-maze, T-maze, double H-maze, Barnes maze, novel object test, novel object exploration, novel object discrimination, novel placement recognition, object recognition test, spontaneous object recognition test, object recognition task, object location test, olfactory discrimination test, social recognition, hole-board task, step-down passive avoidance test, passive shock avoidance test. Studies on pups were not included. Both animals with and without electrodes implanted were considered. We included all the articles on the pilocarpine or the lithium-pilocarpine model independent of sex, pre-treatments (i.e., methyl-scopolamine), duration of SE, and of drugs used to stop SE-induced seizures (i.e., diazepam, anesthetics, anesthetics and/or analgesics). The most important inclusion criteria were the development of spontaneous recurrent seizures and their characterization (at least in terms of seizure frequency) and the specification of the time-points of behavioral testing with reference to epilepsy development and progression. All reviewed studies included non-epileptic control animals, which were compared with epileptic ones employing appropriate statistical tests. No other specific requirement regarding the study design or duration of study was set as inclusion criterion. The goal was to collect data correlating the development of epilepsy in the pilocarpine model with the assessment of memory and/or learning alterations. This search identified 104 original articles matching the inclusion criteria.
Contextual and cued fear conditioning (CFC) are tests predominantly employed to assess associative learning in animals (35). The basics of CFC consist of an exposure to neutral surrounding (the context) and to a stimulus (the cue, that can be an auditory tone or a flash of light); these are then combined with an aversive unconditioned stimulus, typically a mild foot shock of a few seconds and intensity around 0.6–0.75 mA (max 1 mA in rodents). An aversive unconditioned stimulus leads to fear in animals when they are exposed to the context and cue. The outcome is a freezing behavior (a complete lack of movement except for that related to respiration); researchers mostly analyze the numbers of freezing in a defined period and/or their duration. The CFC test examines the ability of animals to anticipate unconditioned stimulus which will follow the conditioned stimulus (i.e., to learn from unpleasant experiences). The freezing behavior is generally analyzed automatically (35, 36). According to neurobehavioral findings, specific neocortical brain structures are primarily involved in fear memory, the prefrontal and the anterior cingulate cortex (36–38). Beside these, a well-established contribution of the hippocampal formation and entorhinal cortex (EC), together with inputs from basolateral amygdala (BLA), have been implicated in learning and recall of fear memory. Interestingly, the same brain structures take part in temporal lobe epilepsy circuits (39–41). Any epileptic interference with the EC-hippocampal complex, BLA or cortical memory circuits is therefore expected to influence contextual memory.
In CFC protocols, the conditioned and unconditioned stimuli are usually presented concomitantly or the unconditioned one is applied immediately after the conditioned stimulus. Such experimental flow is called “delayed conditioning.” In another type of testing, the two types of stimuli are separated by a defined time interval; such a procedure is called “trace conditioning.” Therefore, the trace conditioning differs from delay conditioning by the addition of a stimulus-free “trace” interval of seconds or minutes, separating the conditioned and unconditioned stimuli. It is believed that the longer the temporal gap in the trace protocol, the lesser the involvement of the hippocampus and the greater that of the prefrontal cortex (42). One of the most frequently employed protocols of CFC is the 2-days trial conditioning. It consists of day-1 training in a cage equipped with a sound source and with a metal grid floor. Animals are put into the cage and, after a few minutes of habituation, are exposed to an auditory stimulus of about 70–80 dB for a few tens of seconds (typically 15–30 s), before delivering a foot shock (generally 2 s of 0.6–0.75 mA) at the end of the auditory stimulus or immediately after its cessation. Such trial is then repeated for 3–5 times. After the training, the animals remain in the cage for another 1–2 min and then are put back to their home cage. In the following days, the animals are put into the same cage, and the contextual fear is evaluated by measuring the freezing response to the unpleasant context in which the animals were exposed during the training. The animals may be also put into a different context (e.g., cage with identical dimensions, but endowed with plastic floor and/or scented with an aroma) and the cue, the auditory stimulus, is then applied. Again, the freezing response is evaluated. As an alternative (similar to only context induced fear response measurement), only the cued fear may be evaluated by measuring the freezing response in the training context. Animals need more trials of training to learn the trace conditioning task. After day-1 of training, researchers may test the animals (i) the day after, in order to assess the recent memory, (ii) after 2–12 days, to test the memory consolidation process and (iii) after about 2 weeks or more, to investigate remote memory recall (memory retention). In any analyzed time point, the reduced freezing behavior indicates the impaired associative (episodic) memory, whereas an increased amount of freezing response may be associated with anxiety-like behavior (43, 44).
If used in epileptic rodents, the CFC protocol may be biased by acute seizure activity, which can resemble freezing. The spindles of spike-and-wave seizure activity may occur in parallel with freezing behavior in rodents (44, 45). Behavior arrest seizures are typical of absence epilepsy and are observed in rodent models (45, 46). In addition, the behavioral arrest associated with epileptiform spike–wave discharges has been described also in rats (47) and mice (48) exposed to pilocarpine. Considering that the CFC experiments with epileptic animals may be influenced by the occurrence of spontaneous seizures of any type (which may occur during the training, as well as at the phase of freezing response assessment), the trials should always include non-epileptic controls, and animals who experienced seizures 1–2 h prior or during the test should be excluded.
Another peculiarity of the contextual memory testing in epileptic animals relates to the learning phase. In the pilocarpine model of temporal lobe epilepsy in rats gives origin to an important hippocampal sclerosis at late phases of the disease development (28), which can lead to impairment of learning of cued fear in CFC. This does not necessarily imply that the CFC paradigm cannot be applied to epileptic animals. The different studies in adult rats treated with lithium-pilocarpine have shown similar results, i.e., an impaired contextual and cued fear memory. A small number of studies investigated fear learning or conditioning during the latency phase of the rat pilocarpine model (26, 49), without revealing any significant result. Most of the studies identified in our search, instead, evaluated fear conditioning and learning abilities in rats in the chronic phase of the disease; in particular, at about 2–3 months after SE. These studies reported a decreased contextual and/or cued fear associated with performance in pilocarpine rats, especially in advanced stages of the disease. The decrease was mainly reported in terms of lower spontaneous freezing duration in given context and/or diminished freezing time due to the tone fear conditioning cue (50, 51). Smolensky et al. (49) reported fear impairment in short term and long term memory cues only in the chronic phase (i.e., days 41–53 post SE), while such impairment was absent during latency (i.e., days 8–15 post SE). Focusing on the advanced stages of epilepsy, particular differences were consistently reported in epileptic rats that showed a significantly diminished freezing time in contextual and tone fear conditioning in comparison to controls at 2 months after pilocarpine (52) or shortly after 2 months (53). Similarly, Zubareva et al. (54) showed a contextual memory decline (decreased freezing time in the familiar cage compared to the controls) in pilocarpine rats 60–70 and 70–90 days after SE (55). Interestingly, Qiu et al. (56) found that pilocarpine rats had most severely impaired fear declarative memory at 2 months after SE, but then improved at 4 months while still performing worse than controls in CFC cues. However, one study reported an opposite result, i.e., an increased level of freezing response due to the auditory cue in delay protocol. Such a finding was observed for 4 consecutive days of training in comparison with controls at 1 month after SE (57). However, the CFC test was applied in this study to assess a single fear learning in epileptic rats at about 1 month post SE, thus differing from the others that refer to the late chronic epileptic animals at about 2–3 months post-SE. All in all, the outcome was similar: impairment of contextual memory in the chronic phase of temporal lobe epilepsy.
Conditioned taste aversion (CTA) is another form of associative learning and may be seen as a special type of classical conditioning; the animal typically learns to associate the novel taste of a new food with subsequent illness resulting from ingestion of some nausea-inducing agent. Most animals learn the task after a single pairing of a novel taste with a nausea-inducing agent (24). Even if interesting, this test is not frequently used in animals, especially in models of temporal lobe epilepsy. Nonetheless, a few studies in the pilocarpine or Li-pilocarpine models indicate that epileptic rats acquire a weak CTA when compared to their controls, which on the other hand learn to avoid conditioned intake very early. In particular, at about 1 month after SE, pilocarpine and Li-pilocarpine treated rats displayed learning deficits and poorer performance in CTA tasks with respect to controls (58). A deterioration of CTA memory has been also observed in pilocarpine treated rats tested during the latency period, i.e., 8–9 days post SE (59).
Another test capable of measuring context memory is social transmission of food preference, which is used to assess social communication ability. It is often performed to evaluate autism-like characteristics, more rarely to assess memory in rodents. However, studies of social transmission of food preferences in rats have shown that animals are more likely to eat novel food if they smell the food on another rat’s breath and after observing another rat eating that food (60). To our best knowledge, this test was not yet used to assess the associative memory in the pilocarpine model of epilepsy. Thus, this may be an option for epilepsy investigators.
The novel object recognition (or exploration) task and its variants permit recognition of the objects in relation to a defined context. The recognition is possible after adequate training, and it is often launched by a combination of spatial, temporal or emotional inputs (61). Like CFC, memory recognition processes primarily solicit hippocampal, neocortical and BLA circuits (37, 38). Today, the most frequently used test of recognition memory in rodents is the novel object recognition (NOR), sometimes denominated novel object exploration (NOE), object recognition task (ORT) or spontaneous object recognition test (SORT). Its less frequently employed variants are novel object placement, novel object preference and novel context preference tests. A distinct NOR variant is an olfactory discrimination test, which is based on the animals’ ability to detect differences between odors (2 or more in a multiple-choice test). An olfactory discrimination task is based on the association between a sensory stimulus and a food or water reward, and the frequency of correct choice for the stimulus associated with the reward is measured. Olfactory discrimination has an important impact also in NOR and its variants; investigators are used to thoroughly clean with ethanol the apparatus and the objects to remove odor cues before and between their use (62). All the above-mentioned tests have the recognition request in common; experimental animals must recognize the size or the shape of an object; they have to recognize the distance between the objects and walls; they do so in a given context. NOR and its variants overlap with object location test, which is more often used in spatial memory testing (OLT; see below). All object recognition tests are based on the spontaneous behavior of rodents.
The basic NOR task is conducted in an open field arena after habituation (regularly 20–30 min) of animals in the arena the day before. The day of trial, animals are allowed to explore in a first session two identical objects; in a subsequent session, one object is replaced by a different one. The new object should be similar to the original in height and volume, but different in shape and appearance. In NOR test, the objects are placed at an equal distance from the walls of the arena. The outcome measure is the time which the animals spend to explore the familiar and the novel object in the second session. As an alternative, researchers use a discrimination index, that is, the ratio of time spent exploring novel/familiar objects. The most employed time-lapse posed between the two sessions is between 5 min to 2 h. Occasionally, a 24 or 48 h delay can be used to explore the shift between recent and remote recognition memory. Measures are highly influenced by this time interval (63), and, on that base, NOR may be used to discriminate short-term, medium-term and long-term memory by evaluation of the retention interval (i.e., the time the animals maintain memory of sample objects presented during the familiarization phase before the test phase, when one of the familiar objects is replaced by a new one) (64).
The NOR test is relatively easy to perform and is therefore often employed when studying epilepsy comorbidities. An important procedural detail that has been employed in nearly all studies in epilepsy models is to exclude animals that experience a spontaneous recurrent seizure (SRS) during the testing or in the hour preceding it. What is emerging is that pilocarpine or Li-pilocarpine treated rats have a poor performance in NOR and its variants. Researchers report that they spend a similar amount of time exploring both the novel and the familiar object, or even more time with the familiar object, which clearly indicates that epileptic rats are unable to recall a recent recognition memory. Interestingly, similar results are found in the early (at about 2 weeks) post SE phase (65–71), and in the late chronic (1–2 months after SE) phase of the pilocarpine model (66, 67, 72–79). The results obtained during the latency phase are in their greater number parallel to chronic results (80–82), and report similarly less time of novel object exploration and a decreased discrimination index. The above mentioned result are validated in age matched controls. The majority of these studies use a 2 h delay between the training and the assessment phase of the test (65, 66, 68, 71). A few studies apply a 24 h gap between the sessions, but results are similar to those obtained with 2 h delay (67, 72, 81). Only the study of Detour and colleagues, which used the 24 h delay protocol, did not reveal any difference in NOR performance between controls and Li-pilocarpine treated rats tested 5 months after SE (20). In the same paper, however, the authors disclosed a memory decline in the same animals when employing the radial 8 arms maze (20). Another outlier study reported no changes in NOR test with respect to non-epileptic controls at 25 and 40 days after SE (83). Confirming the vast majority of NOR studies, impaired olfactory sensitivity and memory has been described 1 week and 2 months after pilocarpine-induced SE (84).
Whereas the NOR test and its variants strongly converge in identifying memory impairment in chronically epileptic animals after pilocarpine SE, result are more variable in early stages of the disease. In fact, many studies did not report deficits early after SE, i.e., at 3 days (74), at 4 days (83), at 7 days after SE (25, 27, 81, 83). In addition, Bernardi and Barros (25) reported negative results also 2 months after SE utilizing the SORT task. This atypical observation may be due to subtle differences between the NOR and the SORT test.
This is an extension of the classic NOR, able to establish if the novelty of objects was important to produce a conditioned increase in environmental preference (85). In the novel context preference test, the animals are given repeated access to familiar and novel objects in different environments and are supposed to display an increase in preference for the paired novelty object-novelty environment (86). The access time to the novel objects is usually at least 10 min. This test may be seen as a natural alternative to FCF, because it gives origin to conditioned association, but not to the fear associated with an environmental cue. However, even if relatively fast and simple, the use of this test is not frequent. Regarding the pilocarpine model, the authors of this review did not find any paper, in which the novel context preference test was used in epileptic animals.
The object location memory task (OLM or OLT) assesses spatial and reference memory. As NOR and its variants, it is based on the spontaneous tendency of rodents to spend more time exploring a novel object than a familiar object and ability to recognize it even if the object has been relocated (87) but, at the same time, it solicits the working memory employed at maze tasks.
Testing occurs in an open field arena, to which the animals are first habituated. The next day, objects of similar material but different shapes are introduced to the arena. They are spaced roughly equidistant from each other with space in the center, where the animal is put at the start of acquisition (training) phase. In the first trial, the animal is allowed to explore the arena with the objects. In the second trial (often 5 min or 2 h thereafter), the animal again encounters the same objects, except that one or two of them have switched positions. The researchers score the time spent sniffing the objects. The most frequently used version of OLT uses two objects, one of which is replaced in the second trial; a four object variant, with replacement of two, is also often used (86).
OLM task is used for assessing cognitive deficits in epileptic animals, although it has been rarely employed in pilocarpine treated rats. However, pilocarpine rats typically spend less time exploring novel location object with respect to control animals (67, 72, 83). This is observed already at 4 days after SE (83), and persists in late chronic phase (67, 83).
Working memory is a theoretical concept often used synonymously with short-term memory. It is believed to have a limited capacity that can hold information temporarily, to retain what can be important for reasoning and the guidance of decision-making (88). Working memory is sometimes perceived in close relationship with attention, as the attentional control has an additive impact on working memory resources (89). For this reason, some of the tests listed below may be also used for the assessment of attention deficit.
Tests employed to assess working memory include the T-maze and its variant Y-maze, and the 4 or 8-arm radial maze or double H-maze. Different tasks, such as left–right discrimination or forced alternation (to open arms), are used. Maze protocols require food deprivation and may introduce scent cue confounds. In these tests, the working memory is solicited along with spatial learning.
T- or Y-mazes are used to assess the decision cognitive ability of rodents. These are T- or Y-letter shaped apparatuses placed horizontally; they can be elevated or wall-enclosed. Animals performing the test are placed at the base of the T/Y and allowed to choose one of the goal arms at the other extremity. It is usually run in two trials of no more than 2 min duration. First, the animal enters one arm. On the second trial, the rodent tends to choose the arm not visited before, thus reflecting memory of the first choice. Such a tendency is called spontaneous alternation and can be reinforced by making the animal hungry and rewarding it with a preferred food in the previously unexplored arm. The number of trials that the animal needs to learn to successfully complete the task can be recorded in the data acquisition phase. Alternatively, the time needed to complete the task can be measured. Whereas two trials (one training and one test trial) may be sufficient for rewarded alternation, spontaneous alternation experiments generally require several training trials. Learning may be made even more difficult by including avoiding entrance into a closed/dark arm, that rodents would instinctively choose. Findings from different studies performed in T- or Y-mazes suggest that both the spontaneous and the rewarded alternation are very sensitive to dysfunction of the hippocampus, although other brain structures may be also involved (31, 90).
T- and Y-mazes have been used to assess the cognitive abilities of epileptic animals using the pilocarpine model. In most studies, a deterioration of spatial working memory was observed. A T-maze test run during the latency phase evidenced a working memory deficit (74, 82, 91, 92). Likewise, a Y-maze tests run in the late chronic phase (40 days post-SE) highlighted a working memory deficit in pilocarpine animals in comparison to controls (93). In contrast with these studies, no difference between pilocarpine and non-epileptic control rats was observed about 1.5 months post SE by Smolensky et al. (49). In these latter study, however, there was a trend to perform worse in pilocarpine rats, and it may be reasonable to expect that this difference could become significant by increasing the number of tested animals.
These tests assess the natural ability of rodents to optimize their exploratory strategies. As in the T- or Y-maze tests, both spontaneous and rewarded alternations can be measured. Radial maze can also be used for spatial learning and memory assessment. Besides the classic dry version, an eight-arm radial water maze exists that is designed to evaluate reference and working memory performance while requiring the use extra-maze cues to locate escape platforms (94). Similarly, the double H water-maze is sometimes employed for spatial learning and memory assessment (95). The most employed of the above-mentioned is the 8-arm radial maze. An ordinary 8-arm radial maze consists in 8 equidistantly spaced arms that cross a central open area, which is separated from the arms by trapdoors. The animals are habituated to the apparatus and put off diet before the test to make them starving for food reward (they may lose about 10–15% of body weight). However, standardization of measures that increase motivation to search for the reward is very difficult, and this may increase variability of the results. They are positioned in the center and allowed to freely explore the apparatus, which is initially sprinkled with food all along the arms. In few following days, food is gradually restricted to the end of the arms, so that the animals become familiar with all arms in their whole length. At the time of testing, animals are placed again into the center of the apparatus with all arms filled with food and the doors open; once the animal enters the first arm, all other arms are closed. When the animal finishes all the food in the first arm, it returns to the center and the door of the empty arm behind is closed. The animal is allowed to stay for a few minutes in the center, then all arms open again, and the animal should enter another food filled arm, avoiding the one where it already ate all food. The test finishes when all eight arms are emptied. The test is repeated daily, and the researchers count predominately the number of trials/days needed for animals to successfully complete the task; many evaluate also the errors made by animals during the test execution (e.g., re-entries in empty arms). This test can also differentiate between the working and reference memory in a variant in which only four of the eight arms offer food during the training and the same arms are filled with food during the test. In this variant, two types of errors can be evaluated. A dysfunction of working memory may be identified if an animal re-enters an emptied arm, in which it ate everything before. In analogy, an impairment of the reference memory may be identified if an animal makes an error entering an empty arm, which it should know is empty because it was empty during the training phase.
Radial-arms mazes were used in epileptic rats, predominately the 8-arm radial maze in chronic phase of the disease modeled by pilocarpine (1–5 months’ post SE), documenting a deficit in the working memory. This was usually reported in terms of a significant increase in the number of trials needed to achieve the trial criteria, in terms of the time needed to obtain all pellets or in terms of elevated number of errors (number of wrong entries and re-entries) in epileptic animals as compared with non-epileptic controls (20, 77, 96–100). Similar results were observed with the 4-arm radial maze in pilocarpine and Li-pilocarpine rats 1 month after SE (58). In one of these studies, a positive correlation was observed between the number of SRS and the working memory impairment (98). Working memory deficits were also observed at earlier time-points (15 days’ post SE) in the 8-arm radial maze (93, 101).
As for CFC and recognition memory tests, the results obtained in radial-arms mazes are highly susceptible to interferences associated with epileptogenic events (i.e., behavioral and electroencephalographic seizures) or postictal behavior. Thus, seizure events should represent exclusion criteria. Another potential challenge for epilepsy researchers is the fact that the task requires starving animals, which increase the level of ketone bodies in the brain. This may represent a bias because the ketogenic diet, which can be mimicked by starvation, can be used to treat epilepsy. Indeed, acute or chronic starving of epileptic animals has been reported to reduce the frequency of seizures (102–104). However, none of the above-mentioned studies refers to starvation as an impediment of the experiment.
Spatial learning and memory is a hippocampal-dependent task (105, 106). The most widely used tests to analyze it in rodents are the Morris water maze, the Barnes maze and the radial eight-arms maze, which was described above; the object location test (OLT), also described above, also offers information on spatial memory.
The Morris water maze (MWM) test is run in a large circular tank filled with water, which is divided by imaginary lines into four quadrants. In one of these is located a submerged platform, which the animal is expected to find during the trial by using various spatial cues, like objects positioned in fixed places of the tank wall and/or on the walls surrounding the tank. To solve the task, the animal engages in spatial learning and memory, recognizing the place where it is at the start of the task using the cues on the walls.
The most frequently used protocol of MWM foresees an initial (about 2 min) habituation period inside the water tank without platform. In the training phase, the animal is placed into the water at different points of the tank for 4–6 trials of 5–15 min each in a single day. Animals generally find the platform in 1–2 min after the start of the assay. Once the animal finds the platform, it is allowed to stay on it for 10–20 s, then is withdrawn. With advancement of the days of training, it takes progressively less to reach the platform. It may take about 1 week for animals to learn to complete the task under a pre-defined cut-off time, depending on the number of trials per day. Non-epileptic animals usually achieve an optimal performance within 2 consecutive days, when 5–8 trials are applied per day. Five days of MWM cued learning is more than sufficient for intact rats. Latency to escape on platform, distance traveled, swimming speed and number of trials needed to learn to reach the platform within the cut-off conditions, are the parameters used to measure spatial memory (107, 108). It should, however, be considered that there are important sex-differences in many parameters that are routinely assessed through MWM (e.g., male rats are used to travel a greater distance in comparison with females, who in turn search faster than males during habituation and manifest more thigmotactic behavior when exploring the pool) (109).
After the acquisition phase, one may also evaluate the spatial memory recall in a probe test, in which the platform is removed from the pool and the rat is allowed to search for it in a defined time interval, usually 2 min. If the animal recalls correctly the position of the platform, it will spend most of the time swimming in the correct quadrant. Less time spent in the correct quadrant reflects an impairment in spatial memory retention or recall. Probe tests may be also repeated after extra periods of training, thus assessing additional spatial memory characteristics (remote memory recall); the animals after repeated-training are supposed to spend less time and/or to need less trials to learn the task in a supplementary probe test. There is also a variation of the test, in which the quadrant of the platform is changed and the animal’s flexibility and speed to learn the new positions is evaluated (cognitive flexibility testing). The most frequently reported outcome measure of MWM probe test is the time spent in the target quadrant. Other measures are proximity and chance ratio. A specific outcome measure is represented by thigmotactic swimming (i.e., the act of swimming close to the walls of the water maze tank lacking the focus), which may be observed in the acquisition phase but also in probe testing, and which gives a measure of inability to learn the task and engage memory functions. Thigmotactic swimming may be considered an anxiety-like behavior (110).
Given the training in the water and a relatively long time needed for animals to learn the task, this test may not be completely suitable for epileptic animals because, if it experiences a seizure while swimming, it may lose its balance and sink. Spontaneous seizures may be facilitated by the efforts required by the task, especially in chronic epilepsy models. These aspects of the MWM test should be taken into account when preparing the experiment and while interpreting the data. Investigators usually set as exclusion criteria the occurrence of SRS during and within 1 h after the MWM task.
Li-pilocarpine or pilocarpine treated rats show a spatial memory deficit in the MWM test in both cued learning and memory retain. Such findings are shared by many groups, independent of the phase of the disease in the pilocarpine model. The vast majority of the studies that we reviewed report a failure of cued learning (predominately in terms of longer escape latency; 86% of reviewed studies) and spatial memory retrieval (in particular in terms of lower time in or fewer crossings of target quadrant in a probe test; 66% of studies) with respect to intact controls (Table 2). Concerning thigmotactic swimming, the data suggest that animals unable to solve the task have generally a stronger thigmotactic behavior (21, 148). In most of the studies, MWM was run after recognition and maze tests, at the end of the behavioral trials and eventually prior to forced swimming test, because MWM is considered to be more stressful for animals as compared with recognition or mazes but milder than forced swimming (164, 165).
Table 2. Use of the Morris water maze in pilocarpine treated rats, at different phases of temporal lobe epilepsy model.
The Barnes maze (BM) assay is one of the three mostly employed assays to test spatial learning and memory (with the MWM and radial 8-arms maze). In the Barnes (dry land) maze, no strong aversive stimulus (like swimming in MWM) or food deprivation for reinforcement (like in the radial 8-arms maze) are used (166–168). Instead, a natural preference of rodents for the dark and closed places is exploited.
The apparatus of BM consists of a circular plane with 18–20 circular holes arranged along its entire circumference; the plane is bright light illuminated; under one of the holes is placed a cage, the only shelter available; animals should find the way to this hole during the task using spatial cues (visual reference points placed around the circular plane). Usually, it takes about 4 to 7 training repetitions to teach the animal to proceed quickly in a straight line toward the hole that hides the drop box (169). BM performance is not associated with significant stress, that may instead compromise other spatial memory tests (166). However, the absence of a strong aversive stimulus may reduce the motivation to complete the operation. If it is the case, a food reinforcement may be considered (170).
The test is run very much like MWM. Experimenters let the animal explore the maze during the habituation; then allow it to learn the position of an escape hole during the training trials; finally, spatial memory retention is tested by putting the animal again into the maze with the escape hole blocked. One recent variant of BM was used to test social interaction and may be classifies as a test assessing contextual memory; in such extension of BM rats (in the role of observers) improve their learning by observing the behavior of other rats (models) that had already acquired the task (171).
The parameters used to assess the classic BM include the number of trials needed to learn the position of the escape hole during the acquisition phase (i.e., the training) and the time spent in the vicinity of the escape hole in the probe test. The BM test has been used less frequently than MWM in epileptic rats, but seems to become progressively more popular (increasing number of studies published in the last decade). One of the reasons why BM is gaining popularity over MWM is that it is less stressful for rodents, as indicated by the fact that, contrary to MWM, it does not increase corticosterone levels (166, 172–174).
The BM test was successfully applied in the pilocarpine model. In all studies, the epileptic rats had significantly increased latency to find the hidden escape box when compared to matched controls. The results are consistent along the course of disease; an impairment in spatial navigation learning and memory was observed both in early chronic phase (69, 71, 80, 175) and late chronic phase (156, 176, 177) of the temporal lobe epilepsy.
The hole-board may be seen as a variant of the BM test. It consists of a small square arena with an extractable platform as floor, which has a set of equally spaced circular holes on its surface. Typically, it has an even number of holes (very frequent is 4-hole-board). Similarly, to BM, it allows the assessment of long-term spatial memory in rodents without the employment of water or food restriction, painful stimuli (electrical shocks) or any other aversive condition. In the 4-hole-board variant, holes are equidistant (one for each of the resulting quadrants). Animals put in the arena spontaneously approach the holes and explore them. If they are re-exposed to the hole-board, they lose interest in the novelty of the holes, unless researchers use a food reinforcement. Animals with an intact long-term memory show a reduction of the frequency of exploring the holes by nose-poking, or do not make errors when searching for the food. Usually, the total number of nose-pokes or the number of correct nose-pokes in food reward variants are counted and used as an index of long-term spatial memory. These numbers are stable across the days of testing in spatial memory of compromised animals.
We identified only one work in which hole board task was employed for assessment of spatial memory in pilocarpine rats. Patra et al. (178) report a significant spatial memory deficit at about 4 months after SE.
The lateralized reaction time task (LRTT) is mentioned because it is sometimes used to evaluate attention in pilocarpine treated animals. LRTT measures the basic cognitive processes of perception and response execution. It requires that animals make one specific response (e.g., a spacebar press) whenever a stimulus (e.g., a shape) appears. A laterally driven stimulus is delivered unexpectedly which permits the investigator to differentiate between stimulus-driven and controlled attention (179). LRTT can be digitized, such that the stimuli appear on the screen. Typically, there is only one type of stimulus, and it repeats throughout the experiment. The main utilized LRTT outcome measure is the speed of responding. This test was employed in epileptic rats by Pineda et al. (180) at about 2.5 months after SE, documenting a reduced attention in pilocarpine treated animals.
Social recognition memory tests and their variants resident intruder tests reflect the ability of social animals to recognize and remember individuals of the same species, preferring social interaction over no social interaction and social novelty (i.e., they prefer interaction with a novel animal over a familiar animal) (181). The most popular tests of this kind are the two-trial social recognition test, the habituation-dishabituation paradigm test and discrimination paradigm test, all evaluating the time of interaction (i.e., olfactory investigation) of familiar and non-familiar subject. Rats with unimpaired social recognition memory spend less time investigating familiar individuals, compared to novel individual (182). For example, in the two-trial social recognition test the tested animal at first encounters another animal, then, in the following trial, encounters either the same animal (familiar) or another unknown animal (non-familiar); the measured parameter is reduction in time of investigation of the familiar animal, which reflects the establishment of social recognition memory (183). The habituation-dishabituation paradigm instead consists of five trials, in which the familiar animal is presented to the tested one for the first four trials and the non-familiar animal is introduced in the last trial. Similar to the two-trial social recognition test, a reduction in investigation time with the familiar animal may be observed over the trials, while investigation time increases with the non-familiar rat (184). With the discrimination paradigm the interaction occurs between the tested animal and the familiar and non-familiar ones simultaneously; again, the interaction time with each reflects social memory (185).
A few studies have revealed compromised social recognition memory in epileptic animals. A prolonged interaction time with the familiar animal was observed in epileptic rats at 8 and 56 days post SE (84). Another study reported impaired social memory in epileptic rats based on reduced time spent sniffing and grooming the non-familiar animal in comparison with non-epileptic animal (49). Likewise, a reduced motivation for social contact in terms of reduced interaction with non-familiar rats was reported in epileptic animals at 9 weeks post SE (22).
The Step-Down Avoidance Task (also called light/dark box test) is used for measuring aversive learning and memory, whose presence is observed by behavioral responses following an experience. The test is usually run as a one-trial task combining fear conditioning with an instrumental response, e.g., the active choice of an animal to avoid entering the dark compartment associated with an aversive event (186). The aversive stimulus is most frequently a foot-shock. Like in social interaction tasks, the step-down avoidance has been rarely employed in epileptic animals. However, shorter latency to step into the chamber with electric shock delivery has been reported in pilocarpine rats in the chronic period, 1 or 2 months after SE (75, 79, 187, 188), which indicates learning and memory impairment.
The behavioral tests conducted to access cognitive comorbidities in the rat pilocarpine model often face limitations inherent to the epileptic phenotype (3). The most important of them is the fact that, in chronic phase, spontaneous and recurrent seizures occur unpredictably, which could be a confounding factor for behavioral testing. Seizures occurring shortly before or during the test would affect the animal performance and the results. Video monitoring before and during testing could allow the exclusion of such cases (189). In addition, olfactory deficits (84) and hyperactivity (180) have been reported in pilocarpine rats. This could be a bias in tests requiring motor or odor intact functions, such as the maze-based tests and social interaction tests, respectively. In addition, the animals sex should always be reported and took in consideration when designing and analyzing results, because some behavioral tests, such as CFC (190) or MWM (109), have sex specific outcomes. The fear response measured in CFC tests is considerably lower during proestrus, when levels of the sex hormones estradiol and progesterone are high (190). Similarly, several variables measured routinely in MWM have been shown to vary between sexes (e.g., females search slightly faster than males during habituation, travel lower distances during learning or spend less time than males in the pool’s center over the test days) (109). Not only males may be different from females, but also distinct effects in the above mentioned behavioral tests may be found in cycling females during different hormonal phases. This is the main reason why the vast majority of studies reviewed in this work used exclusively males.
Some of these limitations may be overcome by following published recommendations or guidelines. General recommendations which should be followed in any research work involving animal research are described in the ARRIVE guidelines (Animal Research: Reporting of In Vivo Experiments) (191). Moreover, the Task Force of the International League Against Epilepsy (ILAE) and the American Epilepsy Society (AES) recently developed a guidance that includes Common Data Elements (CDEs) (192), which describes the different types of assessments and highlights the importance of rigorous data collection and transparent reporting in epilepsy. Tests are divided into 7 categories to examine syndrome-level behavioral dysfunctions, including learning and memory deficits. Guidelines discuss the complexities, limitations, and biases associated with behavioral testing, especially when performed on animals with epilepsy (189).
In sum, published data provide clear evidence of memory impairment in the pilocarpine model. The combination of results from different studies is often difficult because of variability in methodological standards, number of animals employed, and outcome measures. The use of a more rigorous and reproducible methodology following the ILAE-AES recommendations (192) would certainly favor a further advancement of the field. Nonetheless, a strong evidence emerges that cognitive impairment begins to appear in the pilocarpine model during the latency phase (i.e., before occurrence of SRSs), becoming intense and affecting different types of memory in the chronic phase, thus mimicking the cognitive co-morbidity profile observed in human temporal lobe epilepsy (193, 194). Altogether, this review supports the value of the rat pilocarpine model not only for modeling spontaneous seizures but also temporal lobe epilepsy-associated cognitive comorbidities.
The original contributions presented in the study are included in the article/supplementary material, further inquiries can be directed to the corresponding author.
AG: Writing – original draft, Writing – review & editing. PP: Writing – original draft, Writing – review & editing. FL: Writing – original draft, Writing – review & editing. LA: Writing – original draft, Writing – review & editing. MSi: Funding acquisition, Writing – original draft, Writing – review & editing. MSo: Conceptualization, Investigation, Methodology, Supervision, Writing – original draft, Writing – review & editing.
The author(s) declare that financial support was received for the research, authorship, and/or publication of this article. This study was supported by a grant from the European Union’s Horizon 2020 Work Programme (call H2020-FETOPEN-2018-2020) under grant agreement 964712 (PRIME; to MSi).
The authors declare that the research was conducted in the absence of any commercial or financial relationships that could be construed as a potential conflict of interest.
All claims expressed in this article are solely those of the authors and do not necessarily represent those of their affiliated organizations, or those of the publisher, the editors and the reviewers. Any product that may be evaluated in this article, or claim that may be made by its manufacturer, is not guaranteed or endorsed by the publisher.
1. Keezer, MR, Sisodiya, SM, and Sander, JW (2016). Comorbidities of epilepsy: current concepts and future perspectives. Lancet Neurol 15:106–15. doi: 10.1016/S1474-4422(15)00225-2
2. Michaelis, R, Tang, V, Wagner, JL, Modi, AC, LaFrance, WCJ, Goldstein, LH, et al. (2017). Psychological treatments for people with epilepsy. Cochrane Database Syst Rev 10:CD012081. doi: 10.1002/14651858.CD012081.pub2
3. Mazarati, A (2019). Can we and should we use animal models to study neurobehavioral comorbidities of epilepsy? Epilepsy Behav 101:106566. doi: 10.1016/j.yebeh.2019.106566
4. Löscher, W, and Stafstrom, CE (2023). Epilepsy and its neurobehavioral comorbidities: insights gained from animal models. Epilepsia 64:54–91. doi: 10.1111/epi.17433
5. Bragatti, JA, Torres, CM, Londero, RG, Assmann, JB, Fontana, V, Martin, KC, et al. (2010). Prevalence of psychiatric comorbidities in temporal lobe epilepsy: the value of structured psychiatric interviews. Epileptic Disord Int Epilepsy J Videotape 12:283–91. doi: 10.1684/epd.2010.0345
6. Gaitatzis, A, Trimble, MR, and Sander, JW (2004). The psychiatric comorbidity of epilepsy. Acta Neurol Scand 110:207–20. doi: 10.1111/j.1600-0404.2004.00324.x
7. Jones, R, Rickards, H, and Cavanna, AE (2010). The prevalence of psychiatric disorders in epilepsy: a critical review of the evidence. Funct Neurol 25:191–4.
8. Tellez-Zenteno, JF, Patten, SB, Jetté, N, Williams, J, and Wiebe, S (2007). Psychiatric comorbidity in epilepsy: a population-based analysis. Epilepsia 48:2336–44. doi: 10.1111/j.1528-1167.2007.01222.x
9. Fiest, KM, Dykeman, J, Patten, SB, Wiebe, S, Kaplan, GG, Maxwell, CJ, et al. (2013). Depression in epilepsy: a systematic review and meta-analysis. Neurology 80:590–9. doi: 10.1212/WNL.0b013e31827b1ae0
10. Pham, T, Sauro, KM, Patten, SB, Wiebe, S, Fiest, KM, Bulloch, AGM, et al. (2017). The prevalence of anxiety and associated factors in persons with epilepsy. Epilepsia 58:e107–10. doi: 10.1111/epi.13817
11. Clancy, MJ, Clarke, MC, Connor, DJ, Cannon, M, and Cotter, DR (2014). The prevalence of psychosis in epilepsy; a systematic review and meta-analysis. BMC Psychiatry 14:75. doi: 10.1186/1471-244X-14-75
12. Dodrill, CB . Progressive cognitive decline in adolescents and adults with epilepsy In: Progress in brain research Eds. Thomas Sutula and Asla Pitkanen. Amsterdam, Netherlands: Elsevier (2002). 399–407.
13. Reyes, A, Kaestner, E, Edmonds, EC, Christina Macari, A, Wang, ZI, Drane, DL, et al. (2021). Diagnosing cognitive disorders in older adults with epilepsy. Epilepsia 62:460–71. doi: 10.1111/epi.16780
14. Seidenberg, M, Pulsipher, DT, and Hermann, B (2007). Cognitive progression in epilepsy. Neuropsychol Rev 17:445–54. doi: 10.1007/s11065-007-9042-x
15. Thompson, PJ, and Duncan, JS (2005). Cognitive decline in severe intractable epilepsy. Epilepsia 46:1780–7. doi: 10.1111/j.1528-1167.2005.00279.x
16. Nickels, KC, Zaccariello, MJ, Hamiwka, LD, and Wirrell, EC (2016). Cognitive and neurodevelopmental comorbidities in paediatric epilepsy. Nat Rev Neurol 12:465–76. doi: 10.1038/nrneurol.2016.98
17. Stafstrom, CE (2014). Epilepsy comorbidities: how can animal models help? Adv Exp Med Biol 813:273–81. doi: 10.1007/978-94-017-8914-1_22
18. Santana-Gomez, CE, Medel-Matus, JS, and Rundle, BK (2021). Animal models of post-traumatic epilepsy and their neurobehavioral comorbidities. Seizure 90:9–16. doi: 10.1016/j.seizure.2021.05.008
19. Medel-Matus, J-S, Shin, D, Sankar, R, and Mazarati, A (2017). Kindling epileptogenesis and panic-like behavior: their bidirectional connection and contribution to epilepsy-associated depression. Epilepsy Behav 77:33–8. doi: 10.1016/j.yebeh.2017.10.001
20. Detour, J, Schroeder, H, Desor, D, and Nehlig, A (2005). A 5-month period of epilepsy impairs spatial memory, decreases anxiety, but spares object recognition in the Lithium-pilocarpine model in adult rats. Epilepsia 46:499–508. doi: 10.1111/j.0013-9580.2005.38704.x
21. Inostroza, M, Cid, E, Brotons-Mas, J, Gal, B, Aivar, P, Uzcategui, YG, et al. (2011). Hippocampal-dependent spatial memory in the water maze is preserved in an experimental model of temporal lobe epilepsy in rats. PLoS One 6:e22372. doi: 10.1371/journal.pone.0022372
22. Inostroza, M, Cid, E, Menendez de la Prida, L, and Sandi, C (2012). Different emotional disturbances in two experimental models of temporal lobe epilepsy in rats. PLoS One 7:e38959. doi: 10.1371/journal.pone.0038959
23. Wang, C-A, Lai, M-C, Lui, C-C, Yang, S-N, Tiao, M-M, Hsieh, C-S, et al. (2007). An enriched environment improves cognitive performance after early-life status epilepticus accompanied by an increase in phosphorylation of extracellular signal-regulated kinase 2. Epilepsy Behav 11:303–9. doi: 10.1016/j.yebeh.2007.06.016
24. Chambers, KC (2018). Conditioned taste aversions. World J Otorhinolaryngol Head Neck Surg 4:92–100. doi: 10.1016/j.wjorl.2018.02.003
25. Bernardi, RB, and Barros, HMT (2004). Carbamazepine enhances discriminative memory in a rat model of epilepsy. Epilepsia 45:1443–7. doi: 10.1111/j.0013-9580.2004.52403.x
26. Fournier, NM, and Persinger, MA (2004). The neuromatrix and the epileptic brain: behavioral and learning preservation in limbic epileptic rats treated with ketamine but not acepromazine. Epilepsy Behav 5:119–27. doi: 10.1016/j.yebeh.2003.11.017
27. Kalemenev, SV, Zubareva, OE, Frolova, EV, Sizov, VV, Lavrentyeva, VV, NYa, L, et al. (2015). Impairment of exploratory behavior and spatial memory in adolescent rats in lithium-pilocarpine model of temporal lobe epilepsy. Dokl Biol Sci 463:175–7. doi: 10.1134/S0012496615040055
28. Curia, G, Longo, D, Biagini, G, Jones, RSG, and Avoli, M (2008). The pilocarpine model of temporal lobe epilepsy. J Neurosci Methods 172:143–57. doi: 10.1016/j.jneumeth.2008.04.019
29. Brooks-Kayal, AR, Bath, KG, Berg, AT, Galanopoulou, AS, Holmes, GL, Jensen, FE, et al. (2013). Issues related to symptomatic and disease-modifying treatments affecting cognitive and neuropsychiatric comorbidities of epilepsy. Epilepsia 54:44–60. doi: 10.1111/epi.12298
30. Dhakal, A, and Bobrin, BD. Cognitive deficits In: StatPearls. Treasure Island (FL): StatPearls Publishing (2024)
31. Deacon, RMJ, and Rawlins, JNP (2006). T-maze alternation in the rodent. Nat Protoc 1:7–12. doi: 10.1038/nprot.2006.2
32. McIlwain, KL, Merriweather, MY, Yuva-Paylor, LA, and Paylor, R (2001). The use of behavioral test batteries: effects of training history. Physiol Behav 73:705–17. doi: 10.1016/s0031-9384(01)00528-5
33. Kassai, F, Ernyey, AJ, Kozma, K, Plangár, I, and Gyertyán, I (2022). Lack of general learning ability factor in a rat test battery measuring a wide spectrum of cognitive domains. J Integr Neurosci 21:012. doi: 10.31083/j.jin2101012
34. Urbach, YK, Bode, FJ, Nguyen, HP, Riess, O, and von Hörsten, S (2010). Neurobehavioral tests in rat models of degenerative brain diseases. Methods Mol Biol Clifton NJ 597:333–56. doi: 10.1007/978-1-60327-389-3_24
35. Curzon, P, Rustay, NR, and Browman, KE. Cued and contextual fear conditioning for rodents In: JJ Buccafusco , editor. Methods of behavior analysis in neuroscience. Boca Raton (FL): CRC Press/Taylor & Francis (2009)
36. Abate, G, Colazingari, S, Accoto, A, Conversi, D, and Bevilacqua, A (2018). Dendritic spine density and EphrinB2 levels of hippocampal and anterior cingulate cortex neurons increase sequentially during formation of recent and remote fear memory in the mouse. Behav Brain Res 344:120–31. doi: 10.1016/j.bbr.2018.02.011
37. Kitamura, T, Ogawa, SK, Roy, DS, Okuyama, T, Morrissey, MD, Smith, LM, et al. (2017). Engrams and circuits crucial for systems consolidation of a memory. Science 356:73–8. doi: 10.1126/science.aam6808
38. Liu, X, Ramirez, S, Pang, PT, Puryear, CB, Govindarajan, A, Deisseroth, K, et al. (2012). Optogenetic stimulation of a hippocampal engram activates fear memory recall. Nature 484:381–5. doi: 10.1038/nature11028
39. Friedman, A, Behrens, CJ, and Heinemann, U (2007). Cholinergic dysfunction in temporal lobe epilepsy. Epilepsia 48:126–30. doi: 10.1111/j.1528-1167.2007.01300.x
40. Huberfeld, G, Blauwblomme, T, and Miles, R (2015). Hippocampus and epilepsy: findings from human tissues. Rev Neurol 171:236–51. doi: 10.1016/j.neurol.2015.01.563
41. Behr, C, D’Antuono, M, Hamidi, S, Herrington, R, Lévesque, M, Salami, P, et al. (2014). Limbic networks and epileptiform synchronization: the view from the experimental side. Int Rev Neurobiol 114:63–87. doi: 10.1016/B978-0-12-418693-4.00004-2
42. Gilmartin, MR, and Helmstetter, FJ (2010). Trace and contextual fear conditioning require neural activity and NMDA receptor-dependent transmission in the medial prefrontal cortex. Learn Mem 17:289–96. doi: 10.1101/lm.1597410
43. Brandão, ML, Zanoveli, JM, Ruiz-Martinez, RC, Oliveira, LC, and Landeira-Fernandez, J (2008). Different patterns of freezing behavior organized in the periaqueductal gray of rats: association with different types of anxiety. Behav Brain Res 188:1–13. doi: 10.1016/j.bbr.2007.10.018
44. Johnson, PL, and Shekhar, A (2012). An animal model of panic vulnerability with chronic disinhibition of the dorsomedial/perifornical hypothalamus. Physiol Behav 107:686–98. doi: 10.1016/j.physbeh.2012.03.016
45. Sarkisova, K, and van Luijtelaar, G (2011). The WAG/Rij strain: a genetic animal model of absence epilepsy with comorbidity of depression [corrected]. Prog Neuro-Psychopharmacol Biol Psychiatry 35:854–76. doi: 10.1016/j.pnpbp.2010.11.010
46. Russo, E, Citraro, R, Davoli, A, Gallelli, L, Di Paola, ED, and De Sarro, G (2013). Ameliorating effects of aripiprazole on cognitive functions and depressive-like behavior in a genetic rat model of absence epilepsy and mild-depression comorbidity. Neuropharmacology 64:371–9. doi: 10.1016/j.neuropharm.2012.06.039
47. Pearce, PS, Friedman, D, Lafrancois, JJ, Iyengar, SS, Fenton, AA, Maclusky, NJ, et al. (2014). Spike-wave discharges in adult Sprague-Dawley rats and their implications for animal models of temporal lobe epilepsy. Epilepsy Behav EB 32:121–31. doi: 10.1016/j.yebeh.2014.01.004
48. Wulsin, AC, Franco-Villanueva, A, Romancheck, C, Morano, RL, Smith, BL, Packard, BA, et al. (2018). Functional disruption of stress modulatory circuits in a model of temporal lobe epilepsy. PLoS One 13:e0197955. doi: 10.1371/journal.pone.0197955
49. Smolensky, IV, Zubareva, OE, Kalemenev, SV, Lavrentyeva, VV, Dyomina, AV, Karepanov, AA, et al. (2019). Impairments in cognitive functions and emotional and social behaviors in a rat lithium-pilocarpine model of temporal lobe epilepsy. Behav Brain Res 372:112044. doi: 10.1016/j.bbr.2019.112044
50. McKay, BE, and Persinger, MA (2004). Normal spatial and contextual learning for ketamine-treated rats in the pilocarpine epilepsy model. Pharmacol Biochem Behav 78:111–9. doi: 10.1016/j.pbb.2004.02.019
51. Szyndler, J, Wierzbabobrowicz, T, Skorzewska, A, Maciejak, P, Walkowiak, J, Lechowicz, W, et al. (2005). Behavioral, biochemical and histological studies in a model of pilocarpine-induced spontaneous recurrent seizures. Pharmacol Biochem Behav 81:15–23. doi: 10.1016/j.pbb.2005.01.020
52. Dos Santos, JG, Longo, BM, Blanco, MM, Menezes De Oliveira, MG, and Mello, LE (2005). Behavioral changes resulting from the administration of cycloheximide in the pilocarpine model of epilepsy. Brain Res 1066:37–48. doi: 10.1016/j.brainres.2005.09.037
53. Bogovyk, R, Lunko, O, Fedoriuk, M, Isaev, D, Krishtal, O, Holmes, GL, et al. (2017). Effects of protease-activated receptor 1 inhibition on anxiety and fear following status epilepticus. Epilepsy Behav 67:66–9. doi: 10.1016/j.yebeh.2016.11.003
54. Zubareva, OE, Dyomina, AV, Kovalenko, AA, Roginskaya, AI, Melik-Kasumov, TB, Korneeva, MA, et al. (2023). Beneficial effects of probiotic Bifidobacterium longum in a Lithium–pilocarpine model of temporal lobe epilepsy in rats. Int J Mol Sci 24:8451. doi: 10.3390/ijms24098451
55. Zubareva, OE, Sinyak, DS, Kalita, AD, Griflyuk, AV, Diespirov, GP, Postnikova, TY, et al. (2023). Antiepileptogenic effects of Anakinra, lamotrigine and their combination in a Lithium–pilocarpine model of temporal lobe epilepsy in rats. Int J Mol Sci 24:15400. doi: 10.3390/ijms242015400
56. Qiu, X, Kinoshita, M, Peng, A, Li, W, Lai, W, Wang, J, et al. (2020). Time-dependent impairment of fear conditioning and associated brain regions after pilocarpine-induced status epilepticus. Front Behav Neurosci 14:149. doi: 10.3389/fnbeh.2020.00149
57. Zhang, L, Feng, D, Tao, H, De, X, Chang, Q, and Hu, Q (2015). Increased stathmin expression strengthens fear conditioning in epileptic rats. Biomed Rep 3:28–32. doi: 10.3892/br.2014.386
58. Persinger, MA, Bureau, YRJ, and Peredery, O (1994). Dissociation between conditioned taste aversion and radial maze learning following seizure-induced multifocal brain damage: quantitative tests of serial vs. parallel circuit models of memory. Physiol Behav 56:225–35. doi: 10.1016/0031-9384(94)90188-0
59. Sroubek, J, Hort, J, Komárek, V, Langmeier, M, and Brozek, G (2001). Acquisition and retrieval of conditioned taste aversion is impaired by brain damage caused by two hours of pilocarpine-induced status epilepticus. Physiol Res 50:609–17.
60. Galef, BGJ (2003). Social learning of food preferences in rodents: rapid appetitive learning. Curr Protoc Neurosci 8:21. doi: 10.1002/0471142301.ns0805ds21
61. Mecklinger, A (2000). Interfacing mind and brain: A neurocognitive model of recognition memory. Psychophysiology 37:565–82.
62. Lueptow, LM (2017). Novel object recognition test for the investigation of learning and memory in mice. J Vis Exp 30:55718. doi: 10.3791/55718
63. Ennaceur, A, and Delacour, J (1988). A new one-trial test for neurobiological studies of memory in rats. 1: behavioral data. Behav Brain Res 31:47–59. doi: 10.1016/0166-4328(88)90157-x
64. Taglialatela, G, Hogan, D, Zhang, W-R, and Dineley, KT (2009). Intermediate- and long-term recognition memory deficits in Tg2576 mice are reversed with acute calcineurin inhibition. Behav Brain Res 200:95–9. doi: 10.1016/j.bbr.2008.12.034
65. Brewster, AL, Lugo, JN, Patil, VV, Lee, WL, Qian, Y, Vanegas, F, et al. (2013). Rapamycin reverses status epilepticus-induced memory deficits and dendritic damage. PLoS One 8:e57808. doi: 10.1371/journal.pone.0057808
66. Falcicchia, C, Paolone, G, Emerich, DF, Lovisari, F, Bell, WJ, Fradet, T, et al. (2018). Seizure-suppressant and neuroprotective effects of encapsulated BDNF-producing cells in a rat model of temporal lobe epilepsy. Mol Ther Methods Clin Dev 9:211–24. doi: 10.1016/j.omtm.2018.03.001
67. Jiang, Y, Han, C, Liu, H, Wang, X, Zhang, X, Meng, F, et al. (2018). Abnormal hippocampal functional network and related memory impairment in pilocarpine-treated rats. Epilepsia 59:1785–95. doi: 10.1111/epi.14523
68. Wang, L, Shi, H, Kang, Y, and Guofeng, W (2020). Hippocampal low-frequency stimulation improves cognitive function in pharmacoresistant epileptic rats. Epilepsy Res 168:106194. doi: 10.1016/j.eplepsyres.2019.106194
69. Wyatt-Johnson, SK, Sommer, AL, Shim, KY, and Brewster, AL (2021). Suppression of microgliosis with the Colony-stimulating factor 1 receptor inhibitor PLX3397 does not attenuate memory defects during Epileptogenesis in the rat. Front Neurol 12:651096. doi: 10.3389/fneur.2021.651096
70. Xu, Z, Mo, F, Yang, G, Fan, P, Lu, B, Liang, W, et al. (2023). Impaired spatial firing representations of neurons in the medial entorhinal cortex of the epileptic rat using microelectrode arrays. Research 6:0229. doi: 10.34133/research.0229
71. Schartz, ND, Sommer, AL, Colin, SA, Mendez, LB, and Brewster, AL (2019). Early treatment with C1 esterase inhibitor improves weight but not memory deficits in a rat model of status epilepticus. Physiol Behav 212:112705. doi: 10.1016/j.physbeh.2019.112705
72. Jiang, Y, Liu, D-F, Zhang, X, Liu, H-G, and Zhang, J-G (2020). Microstructure and functional connectivity-based evidence for memory-related regional impairments in the brains of pilocarpine-treated rats. Brain Res Bull 154:127–34. doi: 10.1016/j.brainresbull.2019.11.010
73. Mazumder, AG, Patial, V, and Singh, D (2019). Mycophenolate mofetil contributes to downregulation of the hippocampal interleukin type 2 and 1β mediated PI3K/AKT/mTOR pathway hyperactivation and attenuates neurobehavioral comorbidities in a rat model of temporal lobe epilepsy. Brain Behav Immun 75:84–93. doi: 10.1016/j.bbi.2018.09.020
74. Mohammad, H, Sekar, S, Wei, Z, Moien-Afshari, F, and Taghibiglou, C (2019). Perampanel but not amantadine prevents behavioral alterations and Epileptogenesis in pilocarpine rat model of status epilepticus. Mol Neurobiol 56:2508–23. doi: 10.1007/s12035-018-1230-6
75. Osuntokun, OS, Olayiwola, G, Adekomi, DA, Oyeyipo, IP, and Ayoka, AO (2021). Proanthocyanidin from Vitis vinifera attenuates memory impairment due to convulsive status epilepticus. Epilepsy Behav 124:108333. doi: 10.1016/j.yebeh.2021.108333
76. Shishmanova-Doseva, M, Atanasova, D, Uzunova, Y, Yoanidu, L, Peychev, L, Marinov, P, et al. (2021). Effects of Lacosamide treatment on Epileptogenesis, neuronal damage and behavioral comorbidities in a rat model of temporal lobe epilepsy. Int J Mol Sci 22:4667. doi: 10.3390/ijms22094667
77. Shishmanova-Doseva, M, Atanasova, D, Ioanidu, L, Uzunova, Y, Atanasova, M, Peychev, L, et al. (2022). The anticonvulsant effect of chronic treatment with topiramate after pilocarpine-induced status epilepticus is accompanied by a suppression of comorbid behavioral impairments and robust neuroprotection in limbic regions in rats. Epilepsy Behav 134:108802. doi: 10.1016/j.yebeh.2022.108802
78. Sun, C, Fu, J, Qu, Z, Jia, L, Li, D, Zhen, J, et al. (2021). Chronic intermittent hypobaric hypoxia restores Hippocampus function and rescues cognitive impairments in chronic epileptic rats via Wnt/β-catenin signaling. Front Mol Neurosci 13:617143. doi: 10.3389/fnmol.2020.617143
79. Costa-Ferro, ZSM, De Oliveira, GN, Da Silva, DV, Marinowic, DR, Machado, DC, Longo, BM, et al. (2020). Intravenous infusion of bone marrow mononuclear cells promotes functional recovery and improves impaired cognitive function via inhibition of rho guanine nucleotide triphosphatases and inflammatory signals in a model of chronic epilepsy. Brain Struct Funct 225:2799–813. doi: 10.1007/s00429-020-02159-7
80. Lee, SH, Choi, BY, Kim, JH, Kho, AR, Sohn, M, Song, HK, et al. (2017). Late treatment with choline alfoscerate (l-alpha glycerylphosphorylcholine, α-GPC) increases hippocampal neurogenesis and provides protection against seizure-induced neuronal death and cognitive impairment. Brain Res 1654:66–76. doi: 10.1016/j.brainres.2016.10.011
81. Pearson, JN, Schulz, KM, and Patel, M (2014). Specific alterations in the performance of learning and memory tasks in models of chemoconvulsant-induced status epilepticus. Epilepsy Res 108:1032–40. doi: 10.1016/j.eplepsyres.2014.04.003
82. Pearson, JN, Rowley, S, Liang, L-P, White, AM, Day, BJ, and Patel, M (2015). Reactive oxygen species mediate cognitive deficits in experimental temporal lobe epilepsy. Neurobiol Dis 82:289–97. doi: 10.1016/j.nbd.2015.07.005
83. Chauvière, L, Rafrafi, N, Thinus-Blanc, C, Bartolomei, F, Esclapez, M, and Bernard, C (2009). Early deficits in spatial memory and Theta rhythm in experimental temporal lobe epilepsy. J Neurosci 29:5402–10. doi: 10.1523/JNEUROSCI.4699-08.2009
84. Lopes, MW, Lopes, SC, Santos, DB, Costa, AP, Gonçalves, FM, De Mello, N, et al. (2016). Time course evaluation of behavioral impairments in the pilocarpine model of epilepsy. Epilepsy Behav 55:92–100. doi: 10.1016/j.yebeh.2015.12.001
85. Bevins, RA, Besheer, J, Palmatier, MI, Jensen, HC, Pickett, KS, and Eurek, S (2002). Novel-object place conditioning: behavioral and dopaminergic processes in expression of novelty reward. Behav Brain Res 129:41–50. doi: 10.1016/s0166-4328(01)00326-6
86. Dix, SL, and Aggleton, JP (1999). Extending the spontaneous preference test of recognition: evidence of object-location and object-context recognition. Behav Brain Res 99:191–200. doi: 10.1016/s0166-4328(98)00079-5
87. Vogel-Ciernia, A, and Wood, MA (2014). Examining object location and object recognition memory in mice. Curr Protoc Neurosci 69:8.31.1–17. doi: 10.1002/0471142301.ns0831s69
88. Nee, DE, and D’Esposito, M (2018). The representational basis of working memory. Curr Top Behav Neurosci 37:213–30. doi: 10.1007/7854_2016_456
89. Archibald, LMD, Levee, T, and Olino, T (2015). Attention allocation: relationships to general working memory or specific language processing. J Exp Child Psychol 139:83–98. doi: 10.1016/j.jecp.2015.06.002
90. Pioli, EY, Gaskill, BN, Gilmour, G, Tricklebank, MD, Dix, SL, Bannerman, D, et al. (2014). An automated maze task for assessing hippocampus-sensitive memory in mice. Behav Brain Res 261:249–57. doi: 10.1016/j.bbr.2013.12.009
91. Dos Santos, JG, Tabosa, A, Do Monte, FHM, Blanco, MM, De Oliveira, FA, and Mello, LE (2005). Electroacupuncture prevents cognitive deficits in pilocarpine-epileptic rats. Neurosci Lett 384:234–8. doi: 10.1016/j.neulet.2005.04.079
92. Pearson, JN, Warren, E, Liang, L-P, Roberts, LJ, and Patel, M (2017). Scavenging of highly reactive gamma-ketoaldehydes attenuates cognitive dysfunction associated with epileptogenesis. Neurobiol Dis 98:88–99. doi: 10.1016/j.nbd.2016.11.011
93. Mathew, J, Balakrishnan, S, Antony, S, Abraham, PM, and Paulose, C (2012). Decreased GABA receptor in the cerebral cortex of epileptic rats: effect of Bacopa monnieri and Bacoside-a. J Biomed Sci 19:25. doi: 10.1186/1423-0127-19-25
94. Penley, SC, Gaudet, CM, and Threlkeld, SW (2013). Use of an eight-arm radial water maze to assess working and reference memory following neonatal brain injury. J Vis Exp 4:50940. doi: 10.3791/50940
95. Faure, J, Akimana, G, Carneiro, JEM, Cosquer, B, Ferrandon, A, Geiger, K, et al. (2013). A comprehensive behavioral evaluation in the lithium–pilocarpine model in rats: effects of carisbamate administration during status epilepticus. Epilepsia 54:1203–13. doi: 10.1111/epi.12219
96. Leite, JP, Nakamura, EM, Lemos, T, and Masur, J (1990). Learning impairment in chronic epileptic rats following pilocaprpine-induced status epilepticus. Braz J Med Biol Res 23:681–3.
97. Do Val-da Silva, RA, Peixoto-Santos, JE, Scandiuzzi, RC, Balista, PA, Bassi, M, Glass, ML, et al. (2016). Decreased neuron loss and memory dysfunction in pilocarpine-treated rats pre-exposed to hypoxia. Neuroscience 332:88–100. doi: 10.1016/j.neuroscience.2016.06.047
98. Wolf, DC, Bueno-Júnior, LS, Lopes-Aguiar, C, Do Val Da Silva, RA, Kandratavicius, L, and Leite, JP (2016). The frequency of spontaneous seizures in rats correlates with alterations in sensorimotor gating, spatial working memory, and parvalbumin expression throughout limbic regions. Neuroscience 312:86–98. doi: 10.1016/j.neuroscience.2015.11.008
99. Richard, GR, Titiz, A, Tyler, A, Holmes, GL, Scott, RC, and Lenck-Santini, P-P (2013). Speed modulation of hippocampal theta frequency correlates with spatial memory performance: speed-Theta modulation and cognition. Hippocampus 23:1269–79. doi: 10.1002/hipo.22164
100. Serpa, A, Bento, M, Caulino-Rocha, A, Pawlak, S, and Cunha-Reis, D (2022). Opposing reduced VPAC1 and enhanced VPAC2 VIP receptors in the hippocampus of the Li2+−pilocarpine rat model of temporal lobe epilepsy. Neurochem Int 158:105383. doi: 10.1016/j.neuint.2022.105383
101. Mathew, J, Peeyush Kumar, T, Khan, RS, and Paulose, CS (2010). Behavioral deficit and decreased GABA receptor functional regulation in the cerebellum of epileptic rats: effect of Bacopa monnieri and bacoside a. Epilepsy Behav 17:441–7. doi: 10.1016/j.yebeh.2010.01.012
102. Bough, KJ, Gudi, K, Han, FT, Rathod, AH, and Eagles, DA (2002). An anticonvulsant profile of the ketogenic diet in the rat. Epilepsy Res 50:313–25. doi: 10.1016/s0920-1211(02)00086-4
103. DeVivo, DC, Malas, KL, and Leckie, MP (1975). Starvation and seizures. Observation on the electroconvulsive threshold and cerebral metabolism of the starved adult rat. Arch Neurol 32:755–60. doi: 10.1001/archneur.1975.00490530077008
104. Harney, JP, Madara, J, Madara, J, and I’Anson, H (2002). Effects of acute inhibition of fatty acid oxidation on latency to seizure and concentrations of beta hydroxybutyrate in plasma of rats maintained on calorie restriction and/or the ketogenic diet. Epilepsy Res 49:239–46. doi: 10.1016/s0920-1211(02)00046-3
105. Best, PJ, White, AM, and Minai, A (2001). Spatial processing in the brain: the activity of hippocampal place cells. Annu Rev Neurosci 24:459–86. doi: 10.1146/annurev.neuro.24.1.459
106. Muller, RU, Stead, M, and Pach, J (1996). The hippocampus as a cognitive graph. J Gen Physiol 107:663–94. doi: 10.1085/jgp.107.6.663
108. Morris, R (1984). Developments of a water-maze procedure for studying spatial learning in the rat. J Neurosci Methods 11:47–60. doi: 10.1016/0165-0270(84)90007-4
109. Zorzo, C, Arias, JL, and Méndez, M (2023). Are there sex differences in spatial reference memory in the Morris water maze? A large-sample experimental study. Learn Behav. doi: 10.3758/s13420-023-00598-w
110. Higaki, A, Mogi, M, Iwanami, J, Min, L-J, Bai, H-Y, Shan, B-S, et al. (2018). Recognition of early stage thigmotaxis in Morris water maze test with convolutional neural network. PLoS One 13:e0197003. doi: 10.1371/journal.pone.0197003
111. Feng, Y, Lin, Y, Han, Y, Pang, Y, Xu, J, Xue, Y, et al. (2018). 2R,4R-APDC, a metabotropic glutamate receptor agonist, reduced neuronal apoptosis by upregulating MicroRNA-128 in a rat model after seizures. Neurochem Res 43:591–9. doi: 10.1007/s11064-017-2453-z
112. Huang, C-W, Cheng, J-T, Tsai, J-J, Wu, S-N, and Huang, C-C (2009). Diabetic hyperglycemia aggravates seizures and status epilepticus-induced hippocampal damage. Neurotox Res 15:71–81. doi: 10.1007/s12640-009-9008-2
113. Shao, Y, Feng, Y, Xie, Y, Luo, Q, Chen, L, Li, B, et al. (2016). Protective effects of Thymoquinone against Convulsant activity induced by Lithium-pilocarpine in a model of status epilepticus. Neurochem Res 41:3399–406. doi: 10.1007/s11064-016-2074-y
114. Mahfoz, AM, Abdel-Wahab, AF, Afify, MA, Shahzad, N, Ibrahim, IAA, ElSawy, NA, et al. (2017). Neuroprotective effects of vitamin D alone or in combination with lamotrigine against lithium-pilocarpine model of status epilepticus in rats. Naunyn Schmiedeberg's Arch Pharmacol 390:977–85. doi: 10.1007/s00210-017-1400-5
115. Kim, J-E, Choi, H-C, Song, H-K, and Kang, T-C (2019). Perampanel affects up-stream regulatory signaling pathways of GluA1 phosphorylation in Normal and epileptic rats. Front Cell Neurosci 13:80. doi: 10.3389/fncel.2019.00080
116. Liu, J, Si, Z, Li, S, Huang, Z, He, Y, Zhang, T, et al. (2018). The Calcineurin inhibitor FK506 prevents cognitive impairment by inhibiting reactive Astrogliosis in pilocarpine-induced status epilepticus rats. Front Cell Neurosci 11:428. doi: 10.3389/fncel.2017.00428
117. Li, M, Cui, L, Feng, X, Wang, C, Zhang, Y, Wang, L, et al. (2019). Losmapimod protected epileptic rats from hippocampal neuron damage through inhibition of the MAPK pathway. Front Pharmacol 10:625. doi: 10.3389/fphar.2019.00625
118. Tariq, M, Ahmad, M, Moutaery, KA, and Deeb, SA (2008). Pentoxifylline ameliorates lithium-pilocarpine induced status epilepticus in young rats. Epilepsy Behav 12:354–65. doi: 10.1016/j.yebeh.2007.12.004
119. Ali, AE, Mahdy, HM, Elsherbiny, DM, and Azab, SS (2018). Rifampicin ameliorates lithium-pilocarpine-induced seizures, consequent hippocampal damage and memory deficit in rats: impact on oxidative, inflammatory and apoptotic machineries. Biochem Pharmacol 156:431–43. doi: 10.1016/j.bcp.2018.09.004
120. Zhao, T, Ding, Y, Li, M, Zhou, C, and Lin, W (2019). Silencing lncRNA PVT1 inhibits activation of astrocytes and increases BDNF expression in hippocampus tissues of rats with epilepsy by downregulating the Wnt signaling pathway. J Cell Physiol 234:16054–67. doi: 10.1002/jcp.28264
121. Kalemenev, SV, Zubareva, OE, Sizov, VV, Lavrent’eva, VV, NYa, L, KKh, K, et al. (2016). Memantine attenuates cognitive impairments after status epilepticus induced in a lithium–pilocarpine model. Dokl Biol Sci 470:224–7. doi: 10.1134/S0012496616050148
122. Jun, Y, JiangTao, X, YuanGui, H, YongBin, S, Jun, Z, XiaoJun, M, et al. (2009). Erythropoietin pre-treatment prevents cognitive impairments following status epilepticus in rats. Brain Res 1282:57–66. doi: 10.1016/j.brainres.2009.05.062
123. Xing, Y, Qin, Y, Jing, W, Zhang, Y, Wang, Y, Guo, D, et al. (2016). Exposure to Mozart music reduces cognitive impairment in pilocarpine-induced status epilepticus rats. Cogn Neurodyn 10:23–30. doi: 10.1007/s11571-015-9361-1
124. Deng, X, Wang, M, Hu, S, Feng, Y, Shao, Y, Xie, Y, et al. (2019). The neuroprotective effect of Astaxanthin on pilocarpine-induced status epilepticus in rats. Front Cell Neurosci 13:123. doi: 10.3389/fncel.2019.00123
125. Gelfuso, EA, Reis, SL, Pereira, AMS, Aguiar, DSR, and Beleboni, RO (2020). Neuroprotective effects and improvement of learning and memory elicited by erythravine and 11α-hydroxy-erythravine against the pilocarpine model of epilepsy. Life Sci 240:117072. doi: 10.1016/j.lfs.2019.117072
126. Gao, J, An, L, Xu, Y, and Huang, Y (2018). Catalpol exerts an anti-epilepticus effect, possibly by regulating the Nrf2-Keap1-ARE signaling pathway. Med Sci Monit 24:9436–41. doi: 10.12659/MSM.911902
127. Gao, F, Gao, Y, Liu, Y, Wang, L, and Li, Y (2014). Berberine exerts an anticonvulsant effect and ameliorates memory impairment and oxidative stress in a pilocarpine-induced epilepsy model in the rat. Neuropsychiatr Dis Treat 10:2139–45. doi: 10.2147/NDT.S73210
128. Ge, Y-X, Tian, X-Z, Lin, Y-Y, and Liu, X-Y (2016). Chronic treatment with levetiracetam reverses deficits in hippocampal LTP in vivo in experimental temporal lobe epilepsy rats. Neurosci Lett 628:194–200. doi: 10.1016/j.neulet.2016.06.043
129. Jia, R, Jia, N, Yang, F, Liu, Z, Li, R, Jiang, Y, et al. (2019). Hydrogen alleviates necroptosis and cognitive deficits in Lithium–pilocarpine model of status epilepticus. Cell Mol Neurobiol 39:857–69. doi: 10.1007/s10571-019-00685-5
130. Song, X, He, R, Han, W, Li, T, Xie, L, Cheng, L, et al. (2019). Protective effects of the ROCK inhibitor fasudil against cognitive dysfunction following status epilepticus in male rats. J Neurosci Res 97:506–19. doi: 10.1002/jnr.24355
131. Titiz, AS, Mahoney, JM, Testorf, ME, Holmes, GL, and Scott, RC (2014). Cognitive impairment in temporal lobe epilepsy: role of online and offline processing of single cell information. Hippocampus 24:1129–45. doi: 10.1002/hipo.22297
132. White, HS, Alex, AB, Pollock, A, Hen, N, Shekh-Ahmad, T, Wilcox, KS, et al. (2012). A new derivative of valproic acid amide possesses a broad-spectrum antiseizure profile and unique activity against status epilepticus and organophosphate neuronal damage. Epilepsia 53:134–46. doi: 10.1111/j.1528-1167.2011.03338.x
133. Zhao, Q, Marolewski, A, Rusche, JR, and Holmes, GL (2006). Effects of uridine in models of epileptogenesis and seizures. Epilepsy Res 70:73–82. doi: 10.1016/j.eplepsyres.2006.03.003
134. Zhou, J-L, Zhao, Q, and Holmes, GL (2007). Effect of levetiracetam on visual-spatial memory following status epilepticus. Epilepsy Res 73:65–74. doi: 10.1016/j.eplepsyres.2006.08.007
135. Pascente, R, Frigerio, F, Rizzi, M, Porcu, L, Boido, M, Davids, J, et al. (2016). Cognitive deficits and brain myo-inositol are early biomarkers of epileptogenesis in a rat model of epilepsy. Neurobiol Dis 93:146–55. doi: 10.1016/j.nbd.2016.05.001
136. Mortari, MR, Cunha, AOS, Dos Anjos, LC, Amaral, HO, Quintanilha, MVT, Gelfuso, EA, et al. (2022). A new class of peptides from wasp venom: a pathway to antiepileptic/neuroprotective drugs. Brain Commun 5:fcad016. doi: 10.1093/braincomms/fcad016
137. El-Sayed, SS, El-Yamany, MF, Salem, HA, and El-Sahar, AE (2021). New insights into the effects of vinpocetine against neurobehavioral comorbidities in a rat model of temporal lobe epilepsy via the downregulation of the hippocampal PI3K/mTOR signalling pathway. J Pharm Pharmacol 73:626–40. doi: 10.1093/jpp/rgab011
138. Semenikhina, M, Bogovyk, R, Fedoriuk, M, Nikolaienko, O, Al Kury, LT, Savotchenko, A, et al. (2019). Inhibition of protease-activated receptor 1 ameliorates behavioral deficits and restores hippocampal synaptic plasticity in a rat model of status epilepticus. Neurosci Lett 692:64–8. doi: 10.1016/j.neulet.2018.10.058
139. Zheng, J, Zhang, T, Liu, H, Huang, Z, Teng, J, Deng, J, et al. (2021). Cytisine exerts an anti-epileptic effect via α7nAChRs in a rat model of temporal lobe epilepsy. Front Pharmacol 12:706225. doi: 10.3389/fphar.2021.706225
140. Kumar, H, Katyal, J, and Kumar, GY (2023). Effect of U50488, a selective kappa opioid receptor agonist and levetiracetam against lithium-pilocarpine-induced status epilepticus, spontaneous convulsive seizures and related cognitive impairment. Neurosci Lett 815:137477. doi: 10.1016/j.neulet.2023.137477
141. Nicoletti, JN, Lenzer, J, Salerni, EA, Shah, SK, Elkady, A, Khalid, S, et al. (2010). Vascular endothelial growth factor attenuates status epilepticus-induced behavioral impairments in rats. Epilepsy Behav 19:272–7. doi: 10.1016/j.yebeh.2010.07.011
142. Chen, Y, Feng, Z, Shen, M, Lin, W, Wang, Y, Wang, S, et al. (2020). Insight into Ginkgo biloba L. extract on the improved spatial learning and memory by Chemogenomics knowledgebase, molecular docking, molecular dynamics simulation, and bioassay validations. ACS Omega 5:2428–39. doi: 10.1021/acsomega.9b03960
143. Frisch, C, Kudin, AP, Elger, CE, Kunz, WS, and Helmstaedter, C (2007). Amelioration of water maze performance deficits by topiramate applied during pilocarpine-induced status epilepticus is negatively dose-dependent. Epilepsy Res 73:173–80. doi: 10.1016/j.eplepsyres.2006.10.001
144. Sun, J, Gao, X, Meng, D, Xu, Y, Wang, X, Gu, X, et al. (2017). Antagomirs targeting MiroRNA-134 attenuates epilepsy in rats through regulation of oxidative stress, mitochondrial functions and autophagy. Front Pharmacol 8:524. doi: 10.3389/fphar.2017.00524
145. Wang, P, Nan, S, Zhang, Y, and Fan, J (2022). Effects of GABA(B) receptor positive allosteric modulator BHF177 and IRS-1 on apoptosis of hippocampal neurons in rats with refractory epilepsy via the PI3K/Akt pathway. Cell Biol Int 46:1775–86. doi: 10.1002/cbin.11839
146. Shakeel, W, Javaid, S, Anjum, SMM, Rasool, MF, Samad, N, Alasmari, F, et al. (2020). Time course evaluation of lacosamide alone and in polypharmacy on behavioral manifestations and oxidative stress in lithium-pilocarpine-induced model. J Physiol Pharmacol 71:10. doi: 10.26402/jpp.2020.4.10
147. Gulec Suyen, G, Isbil-Buyukcoskun, N, Kahveci, N, Sengun, E, and Ozluk, K (2016). Immediate and delayed treatment with gabapentin, carbamazepine and CNQX have almost similar impact on cognitive functions and behavior in the lithium-pilocarpine model in rats. Pharmacol Biochem Behav 148:128–35. doi: 10.1016/j.pbb.2016.07.003
148. Vrinda, M, Sasidharan, A, Aparna, S, Srikumar, BN, Kutty, BM, and Shankaranarayana Rao, BS (2017). Enriched environment attenuates behavioral seizures and depression in chronic temporal lobe epilepsy. Epilepsia 58:1148–58. doi: 10.1111/epi.13767
149. Venturin, GT, Greggio, S, Marinowic, DR, Zanirati, G, Cammarota, M, Machado, DC, et al. (2011). Bone marrow mononuclear cells reduce seizure frequency and improve cognitive outcome in chronic epileptic rats. Life Sci 89:229–34. doi: 10.1016/j.lfs.2011.06.006
150. Mao, K, Lei, D, Zhang, H, and You, C (2017). Anticonvulsant effect of piperine ameliorates memory impairment, inflammation and oxidative stress in a rat model of pilocarpine-induced epilepsy. Exp Ther Med 13:695–700. doi: 10.3892/etm.2016.4001
151. Qu, Z, Jia, L, Xie, T, Zhen, J, Si, P, Cui, Z, et al. (2019). (−)-Epigallocatechin-3-Gallate protects against Lithium-pilocarpine-induced epilepsy by inhibiting the toll-like receptor 4 (TLR4)/nuclear factor-κB (NF-κB) signaling pathway. Med Sci Monit 25:1749–58. doi: 10.12659/MSM.915025
152. Khan, R, Krishnakumar, A, and Paulose, CS (2008). Decreased glutamate receptor binding and NMDA R1 gene expression in hippocampus of pilocarpine-induced epileptic rats: neuroprotective role of Bacopa monnieri extract. Epilepsy Behav 12:54–60. doi: 10.1016/j.yebeh.2007.09.021
153. Qiao, Q, Tian, S, Zhang, Y, Che, L, Li, Q, Qu, Z, et al. (2023). A ketogenic diet may improve cognitive function in rats with temporal lobe epilepsy by regulating endoplasmic reticulum stress and synaptic plasticity. Mol Neurobiol 61:2249–64. doi: 10.1007/s12035-023-03659-3
154. Fukumura, S, Sasaki, M, Kataoka-Sasaki, Y, Oka, S, Nakazaki, M, Nagahama, H, et al. (2018). Intravenous infusion of mesenchymal stem cells reduces epileptogenesis in a rat model of status epilepticus. Epilepsy Res 141:56–63. doi: 10.1016/j.eplepsyres.2018.02.008
155. Liu, C, Wen, Y, Huang, H, Lin, W, Huang, M, Lin, R, et al. (2019). Over-expression of 5-HT6 receptor and activated Jab-1/p-c-Jun play important roles in pilocarpine-induced seizures and learning-memory impairment. J Mol Neurosci 67:388–99. doi: 10.1007/s12031-018-1238-4
156. Dyomina, AV, Smolensky, IV, and Zaitsev, AV (2023). Refinement of the Barnes and Morris water maze protocols improves characterization of spatial cognitive deficits in the lithium-pilocarpine rat model of epilepsy. Epilepsy Behav 147:109391. doi: 10.1016/j.yebeh.2023.109391
157. Jia, C, Zhang, R, Wei, L, Xie, J, Zhou, S, Yin, W, et al. (2023). Investigation of the mechanism of tanshinone IIA to improve cognitive function via synaptic plasticity in epileptic rats. Pharm Biol 61:100–10. doi: 10.1080/13880209.2022.2157843
158. Nejm, MB, Victorino, DB, Guimarães-Marques, MJ, Scorza, CA, Finsterer, J, Scorza, FA, et al. (2021). Effects of fish oil supplementation on spatial memory in rats with pilocarpine-induced epilepsy assessed using the Morris water maze test. Epileptic Disord 23:476–84. doi: 10.1684/epd.2021.1289
159. Klatte, K, Kirschstein, T, Otte, D, Pothmann, L, Muller, L, Tokay, T, et al. (2013). Impaired D-serine-mediated Cotransmission mediates cognitive dysfunction in epilepsy. J Neurosci 33:13066–80. doi: 10.1523/JNEUROSCI.5423-12.2013
160. Wu, X, Zhao, Y, Ding, J, Si, Z, Cheng, D, Shi, H, et al. (2018). Decreased vesicular acetylcholine transporter related to memory deficits in epilepsy: a [18 F] VAT positron emission tomography brain imaging study. Epilepsia 59:1655–66. doi: 10.1111/epi.14533
161. Kubová, H, Mareš, P, Suchomelová, L, Brožek, G, Druga, R, and Pitkänen, A (2004). Status epilepticus in immature rats leads to behavioural and cognitive impairment and epileptogenesis. Eur J Neurosci 19:3255–65. doi: 10.1111/j.0953-816X.2004.03410.x
162. Suleymanova, EM, Borisova, MA, and Vinogradova, LV (2019). Early endocannabinoid system activation attenuates behavioral impairments induced by initial impact but does not prevent epileptogenesis in lithium–pilocarpine status epilepticus model. Epilepsy Behav 92:71–8. doi: 10.1016/j.yebeh.2018.12.001
163. Ferreira, ES, Vieira, LG, Moraes, DM, Amorim, BO, Malheiros, JM, Hamani, C, et al. (2018). Long-term effects of anterior thalamic nucleus deep brain stimulation on spatial learning in the pilocarpine model of temporal lobe epilepsy. Neuromodulation Technol Neural Interface 21:160–7. doi: 10.1111/ner.12688
164. Hånell, A, and Marklund, N (2014). Structured evaluation of rodent behavioral tests used in drug discovery research. Front Behav Neurosci 8:252. doi: 10.3389/fnbeh.2014.00252
165. Engelmann, M, Ebner, K, Landgraf, R, and Wotjak, CT (2006). Effects of Morris water maze testing on the neuroendocrine stress response and intrahypothalamic release of vasopressin and oxytocin in the rat. Horm Behav 50:496–501. doi: 10.1016/j.yhbeh.2006.04.009
166. Harrison, FE, Hosseini, AH, and McDonald, MP (2009). Endogenous anxiety and stress responses in water maze and Barnes maze spatial memory tasks. Behav Brain Res 198:247–51. doi: 10.1016/j.bbr.2008.10.015
167. Mei, J, Kohler, J, Winter, Y, Spies, C, Endres, M, Banneke, S, et al. (2020). Automated radial 8-arm maze: a voluntary and stress-free behavior test to assess spatial learning and memory in mice. Behav Brain Res 381:112352. doi: 10.1016/j.bbr.2019.112352
168. He, L-W, Zeng, L, Tian, N, Li, Y, He, T, Tan, D-M, et al. (2020). Optimization of food deprivation and sucrose preference test in SD rat model undergoing chronic unpredictable mild stress. Anim Models Exp Med 3:69–78. doi: 10.1002/ame2.12107
169. Rosenfeld, CS, and Ferguson, SA (2014). Barnes maze testing strategies with small and large rodent models. J Vis Exp 26:e51194. doi: 10.3791/51194
170. Youn, J, Ellenbroek, BA, van Eck, I, Roubos, S, Verhage, M, and Stiedl, O (2012). Finding the right motivation: genotype-dependent differences in effective reinforcements for spatial learning. Behav Brain Res 226:397–403. doi: 10.1016/j.bbr.2011.09.034
171. Yamada, M, and Sakurai, Y (2018). An observational learning task using Barnes maze in rats. Cogn Neurodyn 12:519–23. doi: 10.1007/s11571-018-9493-1
172. McLay, RN, Freeman, SM, and Zadina, JE (1998). Chronic corticosterone impairs memory performance in the Barnes maze. Physiol Behav 63:933–7. doi: 10.1016/s0031-9384(97)00529-5
173. Sternberg, EM, Glowa, JR, Smith, MA, Calogero, AE, Listwak, SJ, Aksentijevich, S, et al. (1992). Corticotropin releasing hormone related behavioral and neuroendocrine responses to stress in Lewis and Fischer rats. Brain Res 570:54–60. doi: 10.1016/0006-8993(92)90563-o
174. Haider, S, and Tabassum, S (2018). Impact of 1-day and 4-day MWM training techniques on oxidative and neurochemical profile in rat brain: a comparative study on learning and memory functions. Neurobiol Learn Mem 155:390–402. doi: 10.1016/j.nlm.2018.09.003
175. Schartz, ND, Wyatt-Johnson, SK, Price, LR, Colin, SA, and Brewster, AL (2018). Status epilepticus triggers long-lasting activation of complement C1q-C3 signaling in the hippocampus that correlates with seizure frequency in experimental epilepsy. Neurobiol Dis 109:163–73. doi: 10.1016/j.nbd.2017.10.012
176. Izadi, A, Pevzner, A, Lee, DJ, Ekstrom, AD, Shahlaie, K, and Gurkoff, GG (2019). Medial septal stimulation increases seizure threshold and improves cognition in epileptic rats. Brain Stimul 12:735–42. doi: 10.1016/j.brs.2019.01.005
177. Zamudio, SR, Pichardo-Macías, LA, Díaz-Villegas, V, Flores-Navarrete, IL, and Guzmán-Velázquez, S (2019). Subchronic cerebrolysin treatment alleviates cognitive impairments and dendritic arborization alterations of granular neurons in the hippocampal dentate gyrus of rats with temporal lobe epilepsy. Epilepsy Behav 97:96–104. doi: 10.1016/j.yebeh.2019.05.025
178. Patra, PH, Barker-Haliski, M, White, HS, Whalley, BJ, Glyn, S, Sandhu, H, et al. (2019). Cannabidiol reduces seizures and associated behavioral comorbidities in a range of animal seizure and epilepsy models. Epilepsia 60:303–14. doi: 10.1111/epi.14629
179. Jentsch, JD, Aarde, SM, and Seu, E (2009). Effects of atomoxetine and methylphenidate on performance of a lateralized reaction time task in rats. Psychopharmacology 202:497–504. doi: 10.1007/s00213-008-1181-0
180. Pineda, E, Jentsch, JD, Shin, D, Griesbach, G, Sankar, R, and Mazarati, A (2014). Behavioral impairments in rats with chronic epilepsy suggest comorbidity between epilepsy and attention deficit/hyperactivity disorder. Epilepsy Behav 31:267–75. doi: 10.1016/j.yebeh.2013.10.004
181. Gheusi, G, Bluthé, RM, Goodall, G, and Dantzer, R (1994). Social and individual recognition in rodents: methodological aspects and neurobiological bases. Behav Process 33:59–87. doi: 10.1016/0376-6357(94)90060-4
182. Wang, X, and Zhan, Y (2022). Regulation of social recognition memory in the hippocampal circuits. Front Neural Circuits 16:839931. doi: 10.3389/fncir.2022.839931
183. Thor, DH, Wainwright, KL, and Holloway, WR (1982). Persistence of attention to a novel conspecific: some developmental variables in laboratory rats. Dev Psychobiol 15:1–8. doi: 10.1002/dev.420150102
184. Sekiguchi, R, Wolterink, G, and van Ree, JM (1991). Short duration of retroactive facilitation of social recognition in rats. Physiol Behav 50:1253–6. doi: 10.1016/0031-9384(91)90591-b
185. Engelmann, M, Wotjak, CT, and Landgraf, R (1995). Social discrimination procedure: an alternative method to investigate juvenile recognition abilities in rats. Physiol Behav 58:315–21. doi: 10.1016/0031-9384(95)00053-l
186. Ogren, SO (1985). Evidence for a role of brain serotonergic neurotransmission in avoidance learning. Acta Physiol Scand Suppl 544:1–71.
187. Shao, Y, Li, B, Huang, Y, Luo, Q, Xie, Y, and Chen, Y (2017). Thymoquinone attenuates brain injury via an antioxidative pathway in a status epilepticus rat model. Transl Neurosci 8:9–14. doi: 10.1515/tnsci-2017-0003
188. Shishmanova-Doseva, M, Tchekalarova, J, Nenchovska, Z, Ivanova, N, Georgieva, K, and Peychev, L (2020). The effect of chronic treatment with Lacosamide and Topiramate on cognitive functions and impaired emotional responses in a pilocarpine-induced post-status epilepticus rat model. Folia Med 62:723–9. doi: 10.3897/folmed.62.e51473
189. Mazarati, A, Jones, NC, Galanopoulou, AS, Harte-Hargrove, LC, Kalynchuk, LE, Lenck-Santini, P-P, et al. (2018). A companion to the preclinical common data elements on neurobehavioral comorbidities of epilepsy: a report of the TASK3 behavior working group of the ILAE/AES joint translational TASK force. Epilepsia Open 3:24–52. doi: 10.1002/epi4.12236
190. Graham, BM, and Daher, M (2016). Estradiol and progesterone have opposing roles in the regulation of fear extinction in female rats. Neuropsychopharmacology 41:774–80. doi: 10.1038/npp.2015.202
191. Percie du Sert, N, Hurst, V, Ahluwalia, A, Alam, S, Avey, MT, Baker, M, et al. (2020). The ARRIVE guidelines 2.0: updated guidelines for reporting animal research. PLoS Biol 18:e3000410. doi: 10.1371/journal.pbio.3000410
192. Loring, DW, Lowenstein, DH, Barbaro, NM, Fureman, BE, Odenkirchen, J, Jacobs, MP, et al. (2011). Common data elements in epilepsy research: development and implementation of the NINDS epilepsy CDE project. Epilepsia 52:1186–91. doi: 10.1111/j.1528-1167.2011.03018.x
193. Moore, PM, and Baker, GA (2002). The neuropsychological and emotional consequences of living with intractable temporal lobe epilepsy: implications for clinical management. Seizure 11:224–30. doi: 10.1053/seiz.2001.0668
Keywords: epilepsy, pilocarpine model, cognitive abilities, comorbidity, rats
Citation: Guarino A, Pignata P, Lovisari F, Asth L, Simonato M and Soukupova M (2024) Cognitive comorbidities in the rat pilocarpine model of epilepsy. Front. Neurol. 15:1392977. doi: 10.3389/fneur.2024.1392977
Received: 28 February 2024; Accepted: 30 April 2024;
Published: 30 May 2024.
Edited by:
Christopher Michael DeGiorgio, University of California, Los Angeles, United StatesReviewed by:
Libor Velisek, New York Medical College, United StatesCopyright © 2024 Guarino, Pignata, Lovisari, Asth, Simonato and Soukupova. This is an open-access article distributed under the terms of the Creative Commons Attribution License (CC BY). The use, distribution or reproduction in other forums is permitted, provided the original author(s) and the copyright owner(s) are credited and that the original publication in this journal is cited, in accordance with accepted academic practice. No use, distribution or reproduction is permitted which does not comply with these terms.
*Correspondence: Marie Soukupova, bWFyaWUuc291a3Vwb3ZhQHVuaWZlLml0
Disclaimer: All claims expressed in this article are solely those of the authors and do not necessarily represent those of their affiliated organizations, or those of the publisher, the editors and the reviewers. Any product that may be evaluated in this article or claim that may be made by its manufacturer is not guaranteed or endorsed by the publisher.
Research integrity at Frontiers
Learn more about the work of our research integrity team to safeguard the quality of each article we publish.