- 1Tongji University School of Medicine, Shanghai, China
- 2Department of Urinary Surgery, Changhai Hospital, Naval Medical University, Shanghai, China
- 3Department of Military Health Statistics, Navy Medical University, Shanghai, China
- 4Department of Epidemiology and Statistics, School of Public Health, Medical College, Zhejiang University, Hangzhou, Zhejiang, China
- 5Department of Neurology, Huashan Hospital, Fudan University, Shanghai, China
Background: The relationship between epilepsy and risk of acute myocardial infarction (AMI) is not fully understood. Evidence from the Stockholm Heart Study indicates that the risk of AMI is increased in people with epilepsy. This study aims to analyze the temporal trends in prevalence, adverse clinical outcomes, and risk factors of AMI in patients with epilepsy (PWE).
Methods: Patients aged 18 years or older, diagnosed with epilepsy with or without AMI and hospitalized from January 1, 2008, to December 31, 2017, were identified from the National Inpatient Sample (NIS) database. The Cochran–Armitage trend test and logistic regressions were conducted using SAS 9.4. Odds ratios (ORs) were generated for multiple variables.
Results: A total of 8,456,098 inpatients were eligible for our analysis, including 181,826 comorbid with AMI (2.15%). The prevalence of AMI diagnosis in PWE significantly increased from 1,911.7 per 100,000 hospitalizations in 2008 to 2,529.5 per 100,000 hospitalizations in 2017 (Ptrend < 0.001). Inpatient mortality was significantly higher in epilepsy patients with AMI compared to those without AMI (OR = 4.61, 95% CI: 4.54 to 4.69). Factors significantly associated with AMI in PWE included age (≥75 years old vs. 18 ~ 44 years old, OR = 3.54, 95% CI: 3.45 to 3.62), atherosclerosis (OR = 4.44, 95% CI: 4.40 to 4.49), conduction disorders (OR = 2.21, 95% CI: 2.17 to 2.26), cardiomyopathy (OR = 2.11, 95% CI: 2.08 to 2.15), coagulopathy (OR = 1.52, 95% CI: 1.49 to 1.54), dyslipidemia (OR = 1.26, 95% CI: 1.24 to 1.27), peptic ulcer disease (OR = 1.23, 95% CI: 1.13 to 1.33), chronic kidney disease (OR = 1.23, 95% CI: 1.22 to 1.25), smoking (OR = 1.20, 95% CI: 1.18 to 1.21), and weight loss (OR = 1.20, 95% CI: 1.18 to 1.22).
Conclusion: The prevalence of AMI in PWE increased during the decade. Mortality rates were high among this population, highlighting the need for comprehensive attention to prophylaxis for risk factors and early diagnosis of AMI in PWE by physicians.
1 Introduction
Epilepsy, a complex disease causing a substantial burden on individuals and society, affects approximately 50 million people worldwide (1). Additionally, 2.4 million new cases are diagnosed each year (2). Despite its prevalence and severity, effective treatments remain limited. Throughout the course of “living with epilepsy,” patients may face various comorbidities, including acute myocardial infarction (AMI).
AMI is a leading cardiovascular disease, associated with high morbidity and mortality, resulting in approximately 9 million deaths annually (3). Recent researches suggest potential links between epilepsy and AMI, involving shared underlying pathophysiological mechanisms (4–6). Certain types of seizures are accompanied by cardiac complications, for instance, chronic epilepsy followed by seizure-induced cardiomyopathy causes abnormal remodeling of the heart, which may be leveraged by frequent hypoxia from spasms of the trachea and bronchus as well as their branches during seizures (7, 8). The anti-seizure medications, especially the enzyme-inducing anti-seizure medications (EIASMs) including carbamazepine, might substantially raise cholesterol levels, resulting in the increment of atherosclerosis and ensuing AMI (9). Furthermore, carbamazepine may increase the occurrence of AMI by inhibiting the electronic activity of myocardial cells when treating patients with epilepsy (10). Given these factors, patients with epilepsy (PWE) are at an increased risk for AMI. And, the co-occurrence of AMI in PWE might exacerbate the overall disease burden, extending beyond the challenges posed by seizures alone.
However, there are relatively few studies addressing this issue. Moreover, in real-world clinical settings, the adverse outcomes associated with the coexistence of epilepsy and AMI are often severely underestimated. Thus, in this study, we aimed to leverage the National (Nationwide) Inpatient Sample (NIS) database, the largest all-payer database in the United States, to explore the up-to-date situation and temporal trends of concomitant AMI in PWE, to compare the clinical outcome profiles between PWE with and without AMI, and to identify the independent risk factors of AMI, wishing to provide more precise and unearthed evidence for better management of the PWE.
2 Materials and methods
2.1 Data source and study population
Data from 2008 to 2017 in the NIS database were retrieved. The NIS is the largest all-payer database of hospital inpatient stays in the United States, containing discharge data from a 20% stratified sample of community hospitals. Using the sampling weights provided by the NIS, national estimates can be achieved (11). Since the NIS is a publicly available de-identified inpatient healthcare database, Institutional Review Board approval was not required according to the Healthcare Cost and Utilization Project (HCUP) data guidelines.
Using the NIS data from January 1, 2008, to December 31, 2017, a retrospective cohort study of inpatient PWE aged >18 years with or without subsequent AMI was conducted. The inclusion criteria were: (1) admitted from January 1, 2008, to December 31, 2017; and (2) had a diagnosis of epilepsy. Epilepsy in the diagnosis field was identified using International Classification of Diseases-9th Revision-Clinical Modification (ICD-9-CM) diagnostic or International Classification of Diseases-10th Revision-Clinical Modification (ICD-10-CM) diagnostic codes (12). AMI was defined in cases where the diagnosis included AMI, identified by ICD-9-CM or ICD-10-CM codes (Supplementary Table A1) (13). Records that met the following criteria were excluded: (1) age < 18 years old; (2) length of hospital stay ≤1 day; (3) total cost = 0; (4) missing in-hospital death status; and (5) transferred to another hospital or institution on the day of admission.
2.2 Primary and secondary outcomes
The primary outcome of this study was temporal trends in the prevalence of AMI in PWE. Secondary outcomes included rates and trends of the inpatient mortality among PWE with or without AMI; associations of AMI with inpatient adverse clinical outcomes, cost and length of stay (LOS); independent risk factors associated with AMI in PWE; and trends of these factors.
2.3 Statistical analysis
Descriptive statistics were used to summarize the characteristics and demographics of all hospitalizations. Univariate analysis was performed for baseline characteristics and the outcomes between two groups: the PWE with AMI group and the PWE without AMI group. For continuous variables, the Kolmogorov–Smirnov test was first employed to evaluate the normality of the distribution (14). The independent t-test was then used for normally distributed continuous variables, and the Wilcoxon rank-sum test was used for non-normally distributed continuous variables. For categorical variables, the Chi-square test was employed. The Cochran–Armitage trend test was used to evaluate the significance of trends over time (15, 16). To assess the association between AMI and adverse inpatient clinical outcomes, cost, and LOS, univariate logistic regression models (unadjusted model 1) and multivariate-adjusted logistic regression models (17) (model 2 and 3) were implemented. Both model 2 and model 3 were adjusted for patient-level and hospital-level characteristics, and comorbidities (Supplementary Table A2). Missing variables were imputed in model 2 as the main analysis, while the original unimputed data were used in model 3 as the sensitivity analysis. Missing values in a categorical variable were imputed by the corresponding dominant category, and missing values in a continuous variable were imputed by the corresponding median or mean value, depending on the results of the normality test (18). Using the same covariates and missing data imputation method, univariate and multivariate-adjusted logistic regression models were also applied to explore the potential risk factors associated with AMI and epilepsy. Subgroup analyses were also performed based on the subtypes of AMI, including non-ST-segment elevation myocardial infarction (NSTEMI) and ST-segment elevation myocardial infarction (STEMI). According to the HCUP guidance, sampling weights were applied to generate the national estimates (19, 20). Every possible comparison between the study groups was considered. All tests were two-tailed with a significant level set at p ≤ 0.05. All analyses were conducted utilizing SAS 9.4 (SAS Institute Inc. Cary, NC).
3 Results
3.1 Study population
Among the 8,456,098 patients aged ≥18 years who were diagnosed with epilepsy from 2008 to 2017 and included in the study, 181,826 (2.15%) had concomitant AMI. Baseline characteristics are summarized in Supplementary Table A3. In the dataset analyzed, the majority of variables exhibited a missing rate of less than 2% among all hospitalizations. Race and median household income displayed higher missing rates of 6.89 and 2.74%, respectively.
3.2 Prevalence and temporal trends of AMI in PWE
The prevalence of AMI increased over 10 years, from 1,911.7 cases per 100,000 epilepsy hospitalizations in 2008 to 2,529.5 in 2017 (Ptrend < 0.001) (Figure 1). Figure 2 and Supplementary Table A4 show the temporal trends of AMI prevalence in subgroups categorized by sex, age, race, household income, insurance type, and hospital region. From 2008 to 2017, AMI prevalence in PWE increased across all subgroups. It increased with age, being 6–9 times higher in the ≥75 years old group than in the 18–44 years old group, and it showed higher prevalence in men than in women.
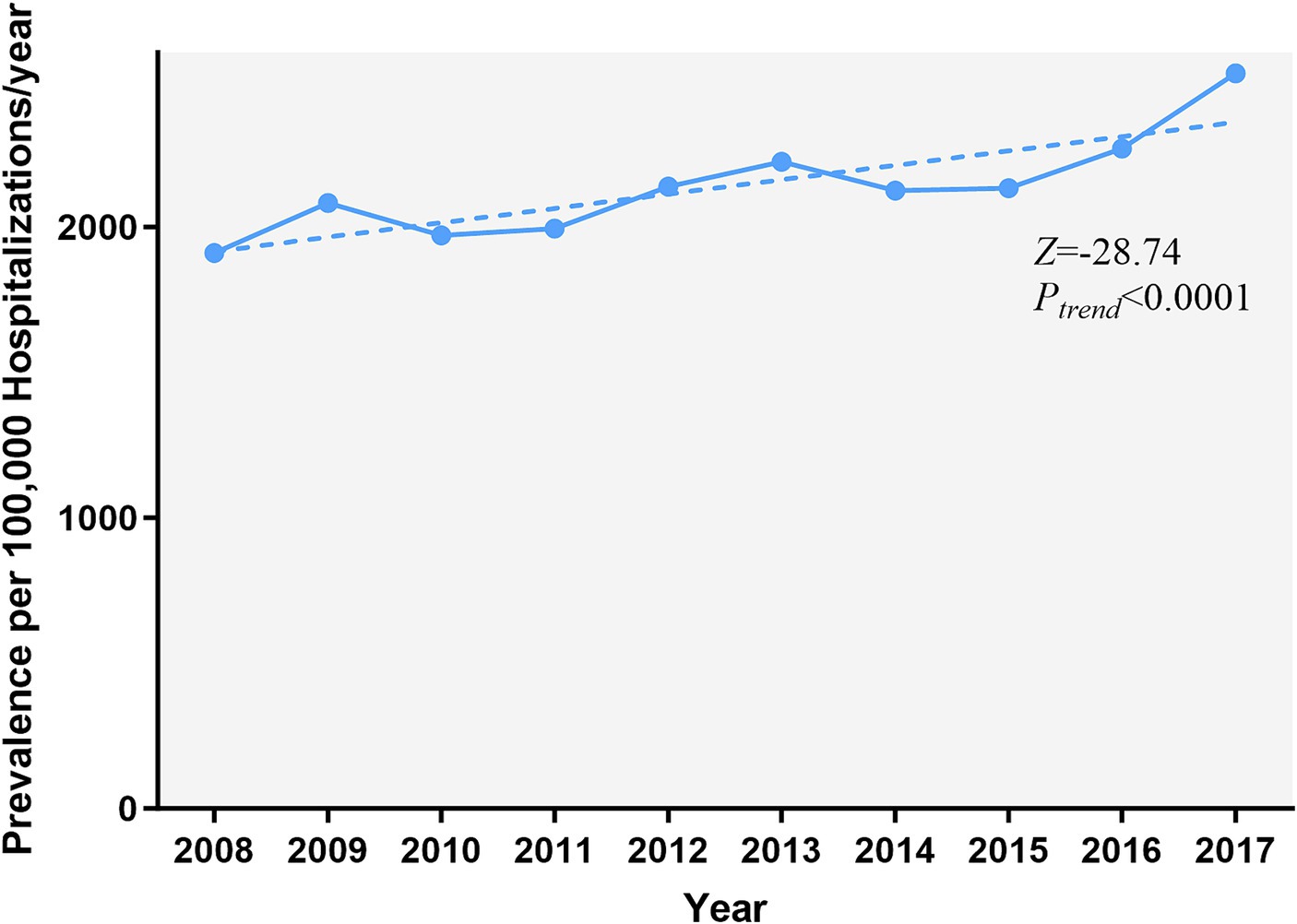
Figure 1. Temporal trends of AMI in PWE. The prevalence of AMI in PWE increased significantly from 2008 to 2017 (Ptrend < 0.001). The significance of trends over time was assessed using the Cochran-Armitage trend test. AMI, acute myocardial infarction; PWE, patients with epilepsy.
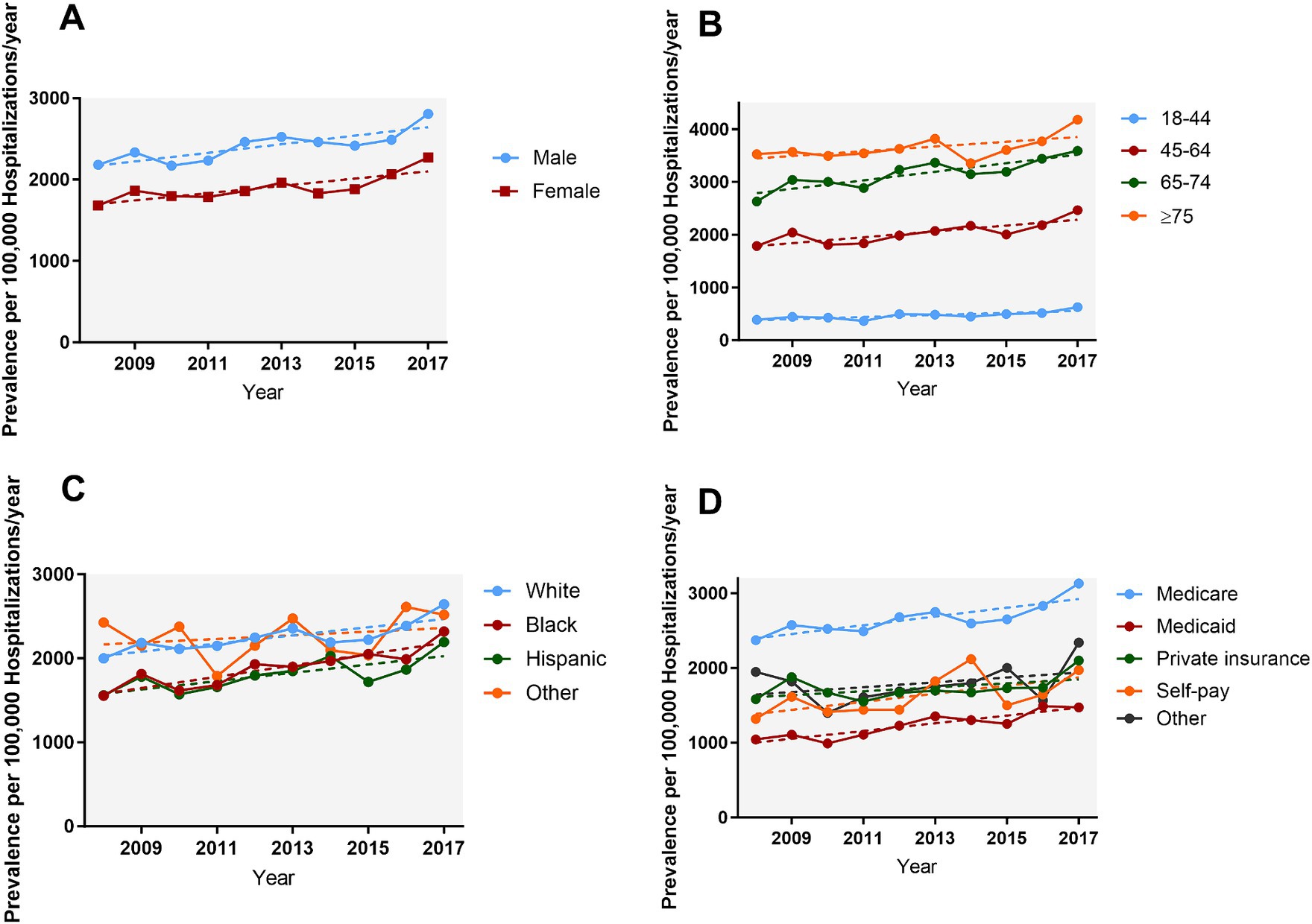
Figure 2. Temporal trends of AMI in PWE subgroups categorized by sex (A), age (B), race (C), and insurance type (D). From 2008 to 2017 the prevalence of AMI in PWE increased across all subgroups (all Ptrend < 0.05). AMI, acute myocardial infarction; PWE, patients with epilepsy.
Supplementary Figure A1 illustrates the increasing trend in both STEMI and NSTEMI cases over the past decade (Ptrend < 0.001). The trend of NSTEMI surges more conspicuously from visual inspection.
3.3 Association between AMI and adverse in-patient clinical outcomes
The in-patient mortality rate was higher in PWE with AMI (13.35%) compared to those without AMI (2.5%). Similarly, AMI was associated with a significantly higher risk of acute heart failure, acute respiratory failure, acute renal failure, cardiogenic shock, venous thromboembolism, fluid and electrolyte disorders, and neurological failure, as well as higher hospital costs and longer LOS (Supplementary Table A5).
The trends of in-hospital mortality remained relatively stable in both groups, with a modest decrease observed among patients with AMI and a subtle increase among those without AMI (Figure 3).
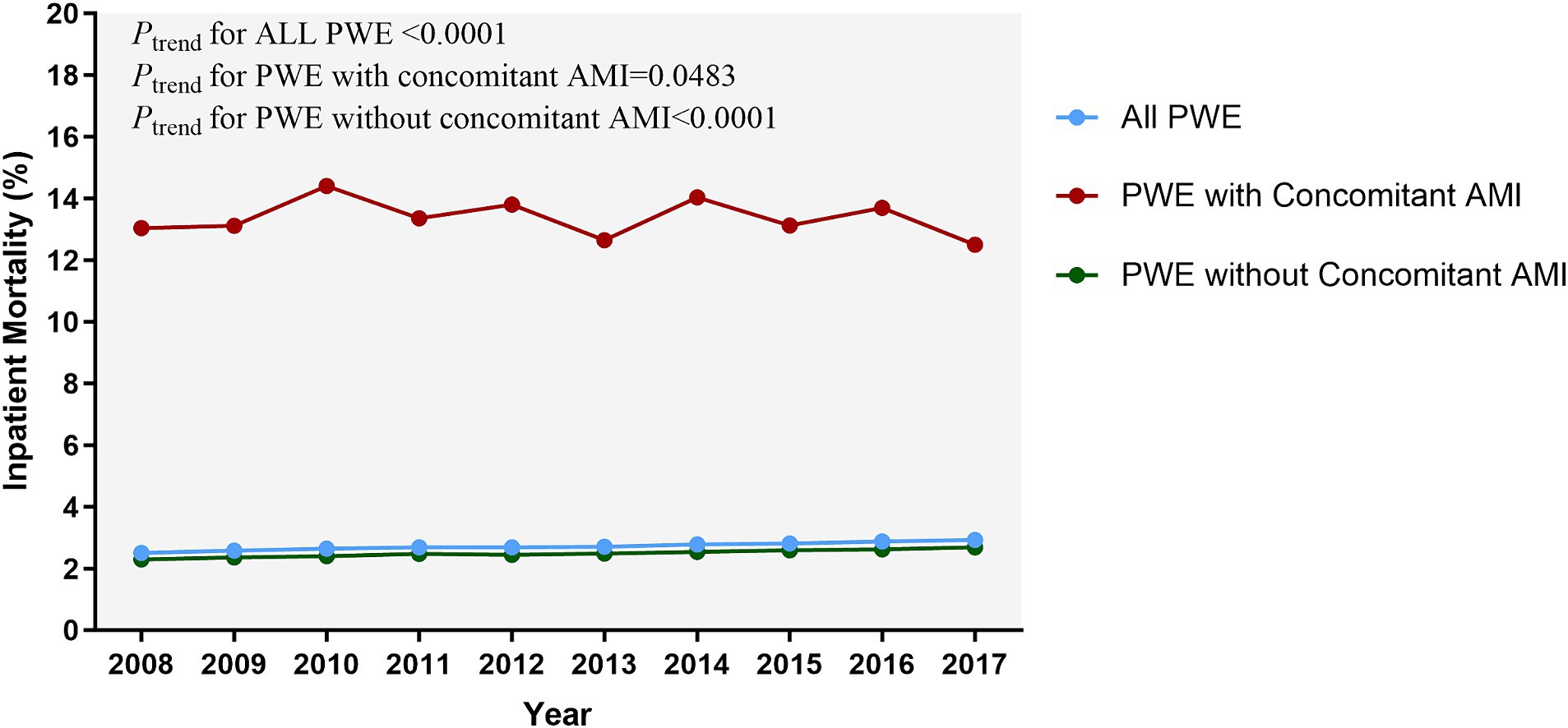
Figure 3. Temporal trends of inpatient mortality related to epilepsy. The inpatient mortality in PWE with AMI mildly decreased but remained high during the study period (13.04% in 2008 vs. 12.50% in 2017); (Ptrend = 0.0483). The significance of trends over time was assessed using the Cochran-Armitage trend test. AMI, acute myocardial infarction; PWE, patients with epilepsy.
In the multivariable logistic regression analysis, which adjusted patient-level, hospital-level and comorbidity related factors, AMI was independently associated with increased inpatient death (OR = 4.61, 95% CI: 4.54 to 4.68), acute heart failure (OR = 3.51, 95% CI: 3.46 to 4.56), acute respiratory failure (OR = 3.44, 95% CI: 3.40 to 3.47), acute renal failure (OR = 2.19, 95% CI: 2.16 to 2.21), neurological failure (OR = 1.91, 95% CI: 1.89 to 1.93), and heart attack (OR = 17.74, 95% CI: 17.24 to 18.25) (Figure 4; Supplementary Table A5). AMI was also related to an increased cost of $44,567 and an increased LOS of 1.685 days after comprehensively adjusting for confounders (Table 1). The robustness of these results was confirmed by the sensitivity analysis (Supplementary Table A6).
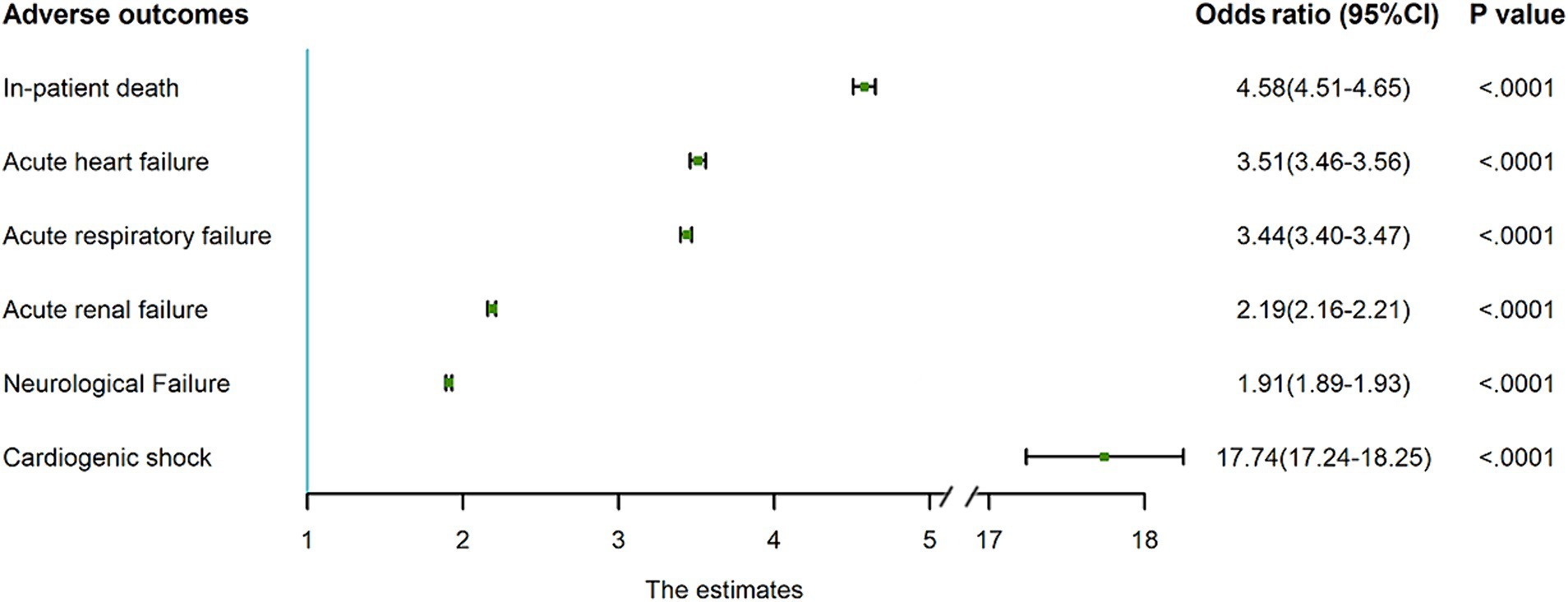
Figure 4. Association of concomitant AMI with adverse clinical outcomes in PWE. Concomitant AMI was independently associated with higher risk of in-patient death, acute heart failure, acute respiratory failure, acute renal failure, neurological failure, and cardiogenic shock. Obtained from multivariate logistic regression models with each adverse outcome as the dependent variable and concomitant AMI as the independent variable, adjusted for patient-level factors (sex, age, race, insurance type, income level, discharge year and season), hospital-level factors (hospital ownership, hospital region, hospital size, hospital location, teaching status), and comorbidity related factors. The analysis accounted for the survey design and was based on the imputed dataset (missing values in category variables were imputed by the dominant category, and missing values in continuous variables were imputed by the median value). AMI, acute myocardial infarction; PWE, patients with epilepsy; CI, confidence interval.
Compared with PWE alone, the presence of either STEMI or NSTEMI in PWE significantly elevated the risk of adverse inpatient outcomes. Numerically, the risk of adverse clinical outcome seems higher in PWE with concomitant STEMI than in PWE with NSTEMI, especially in in-patient death and cardiogenic shock (Supplementary Table A8).
3.4 Risk factors of AMI in PWE
The following factors were found to be associated with concomitant AMI in PWE after adjusting for confounding variables: age ≥ 75 years (OR = 3.54, 95% CI: 3.45 to 3.62), no insurance (OR = 1.41, 95% CI: 1.37 to 1.45), admission on the weekend (OR = 1.21, 95% CI: 1.20 to 1.23), atherosclerosis (OR = 4.44, 95% CI: 4.40 to 4.49), conduction disorders (OR = 2.21, 95% CI: 2.17 to 2.26), cardiomyopathy (OR = 2.11, 95% CI: 2.08 to 2.15), coagulopathy (OR = 1.52, 95% CI: 1.49 to 1.54), dyslipidemia (OR = 1.26, 95% CI: 1.24 to 1.27), peptic ulcer disease excluding bleeding (OR = 1.23, 95% CI: 1.13 to 1.33), chronic kidney disease (CKD) (OR = 1.23, 95% CI: 1.22 to 1.25), smoking history (OR = 1.20, 95% CI: 1.18 to 1.21), and weight loss (OR = 1.20, 95% CI: 1.18 to 1.22) (Supplementary Table A7).
Trends in risk factor profiles are shown in Table 2. From 2008 to 2017, the prevalence of most risk factors increased, except for conduction disorders, chronic blood loss anemia, and uncomplicated diabetes.
4 Discussion
In this study, by exploring a nationwide inpatient sample database, we found that AMI occurred in approximately 2.15% of hospitalizations involving PWE. Notably, the prevalence of AMI diagnosis in PWE significantly increased over time. Furthermore, AMI was strongly associated with increased inpatient mortality in PWE in both the adjusted and unadjusted models. Atherosclerosis, older age, conduction disorders, cardiomyopathy, coagulopathy, dyslipidemia, peptic ulcer disease, smoking, and weight loss were identified as independent risk factors for AMI in PWE. Nearly all of these risk factors showed an increasing trend from 2008 to 2017.
In this study, we found the average prevalence of AMI in PWE was approximately 2,150 per 100,000 hospitalizations per year, aligning with a recent retrospective cohort study conducted in South Carolina (6). However, our study’s AMI prevalence appeared to be ten times higher than that observed in general hospitalizations, which ranged from 2.4–3.1 per 1,000 hospitalizations per year (21). Nevertheless, recent studies have consistently shown a higher risk of AMI in PWE compared to individuals without epilepsy (5, 9), and our findings support this observation.
Given the widespread awareness of traditional cardiovascular disease risks and subsequent innovations in medication and risk factor management, the prevalence of heart attacks in the United States has appreciably decreased over the past two decades (22, 23). However, in contrast to the decreasing trends of AMI prevalence in the general public, this nationwide real-world study observed a significantly rising trend of AMI in PWE by almost 32% from 2008 to 2017. Notably, this trend was consistent across all age groups including young populations, although the proportion remained highest in patients over 75 years of age.
Several factors may explain the higher prevalence and rising trends of AMI in PWE. First, the increasing trends in almost all AMI risk factors in PWE likely contributed to the elevated prevalence of this condition. Second, physicians may overlook somatic comorbidities in PWE, contributing to higher AMI prevalence. Indeed, previous reports indicate that somatic comorbidities in PWE are significant but frequently neglected burdens (24, 25). While some of these somatic comorbidities are traditional risk factors for AMI (26), in clinical practice, doctors are often focused on controlling seizures, potentially neglecting the comprehensive management of epilepsy and its comorbidities, including AMI (27). Third, compliance issues might also lead to rising AMI prevalence, as evidence shows that PWE often struggle with understanding of their illness, complex drug regimens, costs, and adverse events caused by medications (28).
Given the rising trend of AMI in PWE, as well as the 4.61-fold higher mortality risk and 17.74-fold higher heart attack risk in this cohort, recognizing AMI risk factors in PWE and implementing effective prophylaxis is crucial.
Among all the AMI-related risk factors with an elevated trend, dyslipidemia was the most prominent, increasing significantly from 19.29% in 2008 to 32.01% in 2017. High levels of low-density lipoprotein (LDL) and low levels of high-density lipoprotein (HDL) are strongly associated with the onset of AMI (29, 30). Additionally, other risk factors appear to be directly and indirectly related to dyslipidemia. Atherosclerosis, for example, acts as a link between hyperlipidemia and AMI. Hyperlipidemia leads to lipid deposition beneath the arterial endothelium, forming macroscopic plaques. The rupture of these plaques is a common trigger of acute coronary artery thrombosis, leading to AMI (31).
Furthermore, peripheral arterial disease and AMI share common mechanisms. Dyslipidemia aggravates atherosclerosis, leading to plaque enlargement and subsequent peripheral vascular disease and coronary artery stenosis (32). Consequently, patients with peripheral vascular disease have high rates of simultaneous coronary atherosclerosis, resulting in a high risk of myocardial infarction in this population (33).
In addition to the fact that dyslipidemia is prevalent in patients with CKD (34), recent evidence illustrates its contribution to the pathogenesis of renal disease caused by impaired cholesterol efflux, which is known as the “lipid nephrotoxicity hypothesis” (35, 36). When CKD occurs, the kidney secretes hormones, enzymes, and cytokines in response, causing characteristic vascular changes. CKD-related mediators and hemodynamic alterations can also cause cardiac damage, accelerating cardiovascular disease progression, including AMI (37). Furthermore, abnormal glucose metabolism can lead to dyslipidemia, and lipid changes can result in type 2 diabetes (38). Elevated triglycerides lead to increased levels of free fatty acids, inducing insulin resistance and β-cell dysfunction (39, 40). A recent study also highlighted that higher HDL-C concentrations are negatively associated with hyperglycemia (41), and it is well established that patients with diabetes are an increased risk of AMI (42).
There is also a strong association between dyslipidemia and obesity. Dyslipidemias are common in obese patients (43), and insufficient fat oxidation can increase weight gain risk (44). Moreover, obese patients are at high risk for AMI, making lipid-lowering interventions essential.
Given the clear relevance of dyslipidemia and other AMI risk factors we identified, controlling serum lipid levels is crucial in preventing AMI in PWE (42). The propagation of lipid-modifying therapy and increased awareness of dyslipidemia among the public and physicians has led to a decline in serum lipid levels in the United States (22, 45–47). As mentioned earlier, this decline coincides with the decreased prevalence of AMI in the general population. However, PWE in our study had a high prevalence of dyslipidemia, consistent with previous literature (48, 49).
Three possible reasons might explain the high prevalence of dyslipidemia in PWE. First, the lifestyles of PWE could be a contributing factor (50). Second, the administration of EIASMs, such as carbamazepine, phenytoin and phenobarbital, could play a role. EIASMs are metabolized by hepatic cytochrome P450 enzymes, which competitively interfere with cholesterol catabolism to bile acids, catalyzed by the same enzyme system (51–54). Additionally, these medications are associated with mild hypothyroidism, potentially triggering hypercholesterolemia (55). Third, a lack of awareness of lipid management criteria in PWE may also play a role.
Our study highlights the significant disease burden of PWE with AMI: increasing prevalence, worse clinical outcomes, and high in-hospital mortality. Nevertheless, this study only included inpatient patients, and many PWE might experience “silent” myocardial infarctions without hospitalization. Therefore, attention is needed concerning the relationship between epilepsy and AMI. As such, when PWE experience chest pain, AMI should be immediately considered. Additionally, the threat of hyperlipidemia in PWE should be emphasized among physicians, with sufficient evaluation of ASM treatment considering of both seizure control and lipid management. Early diagnosis and prevention for PWE at higher risk of AMI are crucial. Moreover, accessible patient education, regular lipid monitoring and electrocardiograph are urgently needed. Finally, as widely acknowledged in the society, the vital risk factors for seizures in PWE are self-discontinue treatment, significant alcohol or illicit drug use, etc. (56). Thereby, controlling those risk factors may decrease the frequency of seizures, protecting the myocardial cells from hypoxia caused by spasm of respiratory muscles during seizures.
4.1 Limitations
The current analysis has some limitations. First, due to the population characteristics of NIS, we only investigated the condition in adult PWE. Thus, pediatric patients, who are more vulnerable to heart attack owing to the ketogenic diet followed by them, remain to be further studied. Second, the NIS database does not document some vital patient information, such as medications, etiologies, course, and genetic profiles. This limitation caused potential bias in evaluating outcomes and may omit possible risk factors. Third, since the NIS database is primarily retrospective, exploring the causal relationship between any two factors is challenging. Fourth, previous studies have elucidated a higher prevalence of AMI in patients with refectory epilepsy. However, the ICD code for refractory epilepsy is seldomly implemented, resulting in less classification of epilepsy in this database. This limitation advances the paucity of investigating the correlation between the two in this study.
5 Conclusion
The trend of concomitant AMI in PWE has been increasing over the past decade. Concomitant AMI could worsen outcomes and increase the risk of mortality in this population. More attention with regard to cardiovascular risks, especially AMI, should be addressed in PWE besides seizure control.
Data availability statement
Publicly available datasets were analyzed in this study. This data can be found at: https://hcup-us.ahrq.gov/.
Ethics statement
Ethical approval was not required for the study involving humans in accordance with the local legislation and institutional requirements. Written informed consent to participate in this study was not required from the participants or the participants' legal guardians/next of kin in accordance with the national legislation and the institutional requirements.
Author contributions
ZP: Writing – original draft, Writing – review & editing. XX: Conceptualization, Investigation, Writing – original draft. SW: Formal analysis, Methodology, Writing – original draft. XC: Conceptualization, Investigation, Methodology, Validation, Writing – original draft. XL: Data curation, Validation, Visualization, Writing – original draft. CC: Data curation, Resources, Writing – original draft. PY: Supervision, Validation, Visualization, Writing – review & editing. YQ: Supervision, Writing – review & editing. JH: Supervision, Writing – review & editing.
Funding
The author(s) declare financial support was received for the research, authorship, and/or publication of this article. This work was supported by the National Nature Science Foundation of China [grant number 82073671] and Shanghai Science and Technology Development Funds [22QA1411400].
Conflict of interest
The authors declare that the research was conducted in the absence of any commercial or financial relationships that could be construed as a potential conflict of interest.
Publisher’s note
All claims expressed in this article are solely those of the authors and do not necessarily represent those of their affiliated organizations, or those of the publisher, the editors and the reviewers. Any product that may be evaluated in this article, or claim that may be made by its manufacturer, is not guaranteed or endorsed by the publisher.
Supplementary material
The Supplementary material for this article can be found online at: https://www.frontiersin.org/articles/10.3389/fneur.2024.1378682/full#supplementary-material
Abbreviations
AMI, acute myocardial infarction; PWE, patients with epilepsy; NIS, National Inpatient Sample; OR, odds ratio; EIASM, enzyme-inducing anti-seizure medication; HCUP, Healthcare Cost and Utilization Project; ICD-9-CM, International Classification of Diseases-9th Revision-Clinical Modification; ICD-10-CM, International Classification of Diseases-10th Revision-Clinical Modification; LOS, length of stay; NSTEMI, non-ST-segment elevation myocardial infarction; STEMI, ST-segment elevation myocardial infarction; CKD, chronic kidney disease; LDL, low-density lipoprotein; HDL, high-density lipoprotein.
References
1. EPILEPSY A public health imperative international league against epilepsy. (2019). Available at: https://www.who.int/about/licensing.
2. WHO . Epilepsy - Fact Sheets. (2024). Available at: https://www.who.int/news-room/fact-sheets/detail/epilepsy (Accessed July 30, 2024).
3. WHO . The top 10 causes of death. (2020). Available at: https://www.who.int/news-room/fact-sheets/detail/the-top-10-causes-of-death (Accessed July 30, 2024).
4. Janszky, I, Hallqvist, J, Tomson, T, Ahlbom, A, Mukamal, KJ, and Ahnve, S. Increased risk and worse prognosis of myocardial infarction in patients with prior hospitalization for epilepsy-the Stockholm heart epidemiology program. Brain. (2009) 132:2798–804. doi: 10.1093/brain/awp216
5. Cheng, CY, Hsu, CY, Wang, TC, Liu, CY, Yang, YH, and Yang, WH. Risk of cardiac morbidities and sudden death in patients with epilepsy and no history of cardiac disease: a population-based Nationwide study. Mayo Clin Proc. (2021) 96:964–74. doi: 10.1016/j.mayocp.2020.04.050
6. Wilson, DA, Wannamaker, BB, Malek, AM, and Selassie, AW. Myocardial infarction after epilepsy onset: a population-based retrospective cohort study. Epilepsy Behav. (2018) 88:181–8. doi: 10.1016/j.yebeh.2018.09.009
7. Biet, M, Morin, N, Lessard-Beaudoin, M, Graham, RK, Duss, S, Gagné, J, et al. Prolongation of action potential duration and QT interval during epilepsy linked to increased contribution of neuronal sodium channels to cardiac late Na + current. Circ Arrhythm Electrophysiol. (2015) 8:912–20. doi: 10.1161/CIRCEP.114.002693
8. Read, MI, McCann, DM, Millen, RN, Harrison, JC, Kerr, DS, and Sammut, IA. Progressive development of cardiomyopathy following altered autonomic activity in status epilepticus. Am J Physiol Heart Circ Physiol. (2015) 309:H1554–64. doi: 10.1152/ajpheart.00256.2015
9. Lee-Lane, E, Torabi, F, Lacey, A, Fonferko-Shadrach, B, Harris, D, Akbari, A, et al. Epilepsy, antiepileptic drugs, and the risk of major cardiovascular events. Epilepsia. (2021) 62:1604–16. doi: 10.1111/epi.16930
10. Zaccara, G, and Lattanzi, S. Comorbidity between epilepsy and cardiac arrhythmias: implication for treatment. Epilepsy Behav. (2019) 97:304–12. doi: 10.1016/j.yebeh.2019.05.038
11. Healthcare COST and UTILIZATION PROJECT -HCUP a federal-STATE-INDUSTRY PARTNERSHIP in health data. Available at: http://www.hcup-us.ahrq.gov.
12. American Academy of Neurology. ICD-9 to ICD-10 conversion of epilepsy. Available at: https://www.aan.com/siteassets/home-page/tools-and-resources/practicing-neurologist--administrators/billing-and-coding/icd-10-cm/16aanepilepsycrosswalk_tr.pdf (Accessed July 30, 2024).
13. Coloma, PM, Valkhoff, VE, Mazzaglia, G, Nielsson, MS, Pedersen, L, Molokhia, M, et al. Identification of acute myocardial infarction from electronic healthcare records using different disease coding systems: a validation study in three European countries. BMJ Open. (2013) 3:e002862. doi: 10.1136/bmjopen-2013-002862
14. Stephens, MA . EDF statistics for goodness of fit and some comparisons. J Am Stat Assoc. (1974) 69:730–7. doi: 10.2307/2286009
15. Cochran, WG . Some methods for strengthening the common χ2 tests. Biometrics. (1954) 10:417–51. doi: 10.2307/3001616
16. Armitage, P . Tests for linear trends in proportions and frequencies. Biometrics. (1955) 11:375–86. doi: 10.2307/3001775
17. Walker, SH, and Duncan, DB. Estimation of the probability of an event as a function of several independent variables. Biometrika. (1967) 54:167–79. doi: 10.2307/2333860
18. Acuña, E, and Rodriguez, C. The treatment of missing values and its effect in the classifier accuracy. Classification, clustering, and data mining application. Proceedings of the Meeting of the International Federation of Classification Societies (IFCS), Illinois Institute of Technology, Chicago. (2004).
19. Khera, R, Angraal, S, Couch, T, Welsh, JW, Nallamothu, BK, Girotra, S, et al. Adherence to methodological standards in research using the National Inpatient Sample. JAMA. (2017) 318:2011. doi: 10.1001/jama.2017.17653
20. Khera, R, and Krumholz, HM. With great power comes great responsibility: big data research from the National Inpatient Sample. Circ Cardiovasc Qual Outcomes. (2017) 10:e003846. doi: 10.1161/CIRCOUTCOMES.117.003846
21. Wang, OJ, Wang, Y, Chen, J, and Krumholz, HM. Recent trends in hospitalization for acute myocardial infarction. Am J Cardiol. (2012) 109:1589–93. doi: 10.1016/j.amjcard.2012.01.381
22. Virani, SS, Alonso, A, Benjamin, EJ, Bittencourt, MS, Callaway, CW, Carson, AP, et al. Heart disease and stroke statistics—2020 update: a report from the American Heart Association. Circulation. (2020) 141:e139–596. doi: 10.1161/CIR.0000000000000757
23. Salah, HM, Minhas, AMK, Khan, MS, Khan, SU, Ambrosy, AP, Blumer, V, et al. Trends in hospitalizations for heart failure, acute myocardial infarction, and stroke in the United States from 2004 to 2018. Am Heart J. (2022) 243:103–9. doi: 10.1016/j.ahj.2021.09.009
24. Novy, J, Bell, GS, Peacock, JL, Sisodiya, SM, and Sander, JW. Epilepsy as a systemic condition: link with somatic comorbidities. Acta Neurol Scand. (2017) 136:352–9. doi: 10.1111/ane.12779
25. Gaitatzis, A, Sisodiya, SM, and Sander, JW. The somatic comorbidity of epilepsy: a weighty but often unrecognized burden. Epilepsia. (2012) 53:1282–93. doi: 10.1111/j.1528-1167.2012.03528.x
27. Boro, A, and Haut, S. Medical comorbidities in the treatment of epilepsy. Epilepsy Behav. (2003) 4:2–12. doi: 10.1016/j.yebeh.2003.07.002
28. Shaaban, J, Ishak, H, and Ismail, AH. Factors associated with drug compliance among epilepsy patients. Int Med J. (2011) 18:78–79.
29. Gordon, T, Castelli, WP, Hjortland, MC, Kannel, WB, and Dawber, TR. High density lipoprotein as a protective factor against coronary heart disease. The Framingham study. Am J Med. (1977) 62:707–14. doi: 10.1016/0002-9343(77)90874-9
30. Ference, BA, Ginsberg, HN, Graham, I, Ray, KK, Packard, CJ, Bruckert, E, et al. Low-density lipoproteins cause atherosclerotic cardiovascular disease. 1. Evidence from genetic, epidemiologic, and clinical studies. A consensus statement fromthe European atherosclerosis society consensus panel. Eur Heart J. (2017) 38:2459–72. doi: 10.1093/eurheartj/ehx144
31. Bentzon, JF, Otsuka, F, Virmani, R, and Falk, E. Mechanisms of plaque formation and rupture. Circ Res. (2014) 114:1852–66. doi: 10.1161/CIRCRESAHA.114.302721
32. Dieter, RS, Dieter, RA, Jr., and Dieter, RI III (Eds.). Peripheral arterial disease. McGraw-Hill Education. (2009). Available at: https://accesscardiology.mhmedical.com/content.aspx?bookid=1789§ionid=119998534
33. Olivier, CB, Mulder, H, Hiatt, WR, Jones, WS, Fowkes, FGR, Rockhold, FW, et al. Incidence, characteristics, and outcomes of myocardial infarction in patients with peripheral artery disease: insights from the EUCLID trial. JAMA Cardiol. (2019) 4:7–15. doi: 10.1001/jamacardio.2018.4171
34. Chen, SC, Hung, CC, Kuo, MC, Lee, JJ, Chiu, YW, Chang, JM, et al. Association of Dyslipidemia with renal outcomes in chronic kidney disease. PLoS One. (2013) 8:e55643. doi: 10.1371/journal.pone.0055643
35. Samuel, VT, and Shulman, GI. Mechanisms for insulin resistance: common threads and missing links. Cell. (2012) 148:852–71. doi: 10.1016/j.cell.2012.02.017
36. Moorhead, JF, El-Nahas, M, Chan, MK, and Varghese, Z. Lipid nephrotoxicity in chronic PROGRESSIVE glomerular and TUBULO-interstitial disease. Lancet. (1982) 320:1309–11. doi: 10.1016/S0140-6736(82)91513-6
37. Jankowski, J, Floege, J, Fliser, D, Böhm, M, and Marx, N. Cardiovascular disease in chronic kidney disease: pathophysiological insights and therapeutic options. Circulation. (2021) 143:1157–72. doi: 10.1161/CIRCULATIONAHA.120.050686
38. Parhofer, KG . Interaction between glucose and lipid metabolism: more than diabetic dyslipidemia. Diabetes Metab J. (2015) 39:353–62. doi: 10.4093/dmj.2015.39.5.353
39. Briaud, I, Harmon, JS, Kelpe, CL, Segu, VB, and Poitout, V. Lipotoxicity of the pancreatic beta-cell is associated with a glucose-dependent, substrate-driven increase in esterification of fatty acids into neutral lipids. Diabetes Res Clin Pract. (2000) 50:147. doi: 10.1016/s0168-8227(00)81954-3
40. Lee, Y, Hirose, H, Ohneda, M, Johnson, JH, McGarry, JD, and Unger, RH. β-Cell lipotoxicity in the pathogenesis of non-insulin-dependent diabetes mellitus of obese rats: impairment in adipocyte-β-cell relationships. Proc Natl Acad Sci USA. (1994) 91:10878–82. doi: 10.1073/pnas.91.23.10878
41. Barter, PJ, Rye, KA, Tardif, JC, Waters, DD, Matthijs, BS, Breazna, A, et al. Effect of torcetrapib on glucose, insulin, and hemoglobin a1c in subjects in the investigation of lipid level management to understand its impact in atherosclerotic events (illuminate) trial. Circulation. (2011) 124:555–62. doi: 10.1161/CIRCULATIONAHA.111.018259
42. Visseren, FLJ, MacH, F, Smulders, YM, Carballo, D, Koskinas, KC, Bäck, M, et al. ESC guidelines on cardiovascular disease prevention in clinical practice. Eur Heart J. (2021) 42:3227–337. doi: 10.1093/eurheartj/ehab484
43. Bays, HE, Toth, PP, Kris-Etherton, PM, Abate, N, Aronne, LJ, Brown, WV, et al. Obesity, adiposity, and dyslipidemia: a consensus statement from the National Lipid Association. J Clin Lipidol. (2013) 7:304–83. doi: 10.1016/j.jacl.2013.04.001
44. Ravussin, E, and Gautier, JF. Metabolic predictors of weight gain. Int J Obes. (1999) 23:S37–41. doi: 10.1038/sj.ijo.0800793
45. Carroll, MD, Fryar, CD, and Nguyen, DT. Total and high-density lipoprotein cholesterol in adults: United States, 2015–2016 NCHS Data Brief. Hyattsville, MD: CDC of United States (2017). Available at: https://www.cdc.gov/nchs/data/databriefs/db290.pdf
46. Shin, D, Kongpakpaisarn, K, and Bohra, C. Trends in the prevalence of metabolic syndrome and its components in the United States 2007–2014. Int J Cardiol. (2018) 259:216–9. doi: 10.1016/j.ijcard.2018.01.139
47. Patel, N, Bhargava, A, Kalra, R, Parcha, V, Arora, G, Muntner, P, et al. Trends in lipid, lipoproteins, and statin use among U.S. adults. J Am Coll Cardiol. (2019) 74:2525–8. doi: 10.1016/j.jacc.2019.09.026
48. Svalheim, S, Luef, G, Rauchenzauner, M, Mørkrid, L, Gjerstad, L, and Taubøll, E. Cardiovascular risk factors in epilepsy patients taking levetiracetam, carbamazepine or lamotrigine. Acta Neurol Scand. (2010) 122:30–3. doi: 10.1111/j.1600-0404.2010.01372.x
49. Vivanco-Hidalgo, RM, Gomez, A, Moreira, A, Díez, L, Elosua, R, and Roquer, J. Prevalence of cardiovascular risk factors in people with epilepsy. Brain Behav. (2017) 7:e00618. doi: 10.1002/brb3.618
50. Szałwińska, K, Cyuńczyk, M, Kochanowicz, J, and Witkowska, AM. Dietary and lifestyle behavior in adults with epilepsy needs improvement: a case-control study from northeastern Poland. Nutr J. (2021) 20:62. doi: 10.1186/s12937-021-00704-6
51. Brodie, MJ, Mintzer, S, Pack, AM, Gidal, BE, Vecht, CJ, and Schmidt, D. Enzyme induction with antiepileptic drugs: cause for concern? Epilepsia. (2013) 54:11–27. doi: 10.1111/j.1528-1167.2012.03671.x
52. Castro-Gago, M, Novo-Rodríguez, MI, Blanco-Barca, MO, Urisarri-Ruíz de Cortázar, A, Rodríguez-García, J, Rodríguez-Segade, S, et al. Evolution of serum lipids and lipoprotein (a) levels in epileptic children treated with carbamazepine, valproic acid, and phenobarbital. J Child Neurol. (2006) 21:48–53. doi: 10.1177/08830738060210011601
53. Demircio, S, Soylu, A, and Dirik, E. Carbamazepine and valproic acid: effects on the serum lipids and liver functions in children. Pediatr Neurol. (2000) 23:142–6. doi: 10.1016/S0887-8994(00)00175-2
54. Josephson, CB, Wiebe, S, Delgado-Garcia, G, Gonzalez-Izquierdo, A, Denaxas, S, Sajobi, TT, et al. Association of Enzyme-Inducing Antiseizure Drug use with long-term cardiovascular disease. JAMA Neurol. (2021) 78:1367. doi: 10.1001/jamaneurol.2021.3424
55. Hamed, SA, Hamed, EA, Kandil, MR, El-Shereef, HK, Abdellah, MM, and Omar, H. Serum thyroid hormone balance and lipid profile in patients with epilepsy. Epilepsy Res. (2005) 66:173–83. doi: 10.1016/j.eplepsyres.2005.08.004
Keywords: acute myocardial infarction, atherosclerotic cardiovascular disease, clinical outcomes, dyslipidemia, epilepsy, prevalence, risk factors
Citation: Pan Z, Xu X, Wu S, Chen X, Luo X, Chen C, Yu P, Qin Y and He J (2024) Temporal trends, in-hospital outcomes, and risk factors of acute myocardial infarction among patients with epilepsy in the United States: a retrospective national database analysis from 2008 to 2017. Front. Neurol. 15:1378682. doi: 10.3389/fneur.2024.1378682
Edited by:
Christopher Michael DeGiorgio, University of California, Los Angeles, United StatesReviewed by:
Jianxiang Liao, Shenzhen Children’s Hospital, ChinaSawsan A. Zaitone, University of Tabuk, Saudi Arabia
Copyright © 2024 Pan, Xu, Wu, Chen, Luo, Chen, Yu, Qin and He. This is an open-access article distributed under the terms of the Creative Commons Attribution License (CC BY). The use, distribution or reproduction in other forums is permitted, provided the original author(s) and the copyright owner(s) are credited and that the original publication in this journal is cited, in accordance with accepted academic practice. No use, distribution or reproduction is permitted which does not comply with these terms.
*Correspondence: Jia He, hejia63@yeah.net; Yingyi Qin, yingyi_qin@163.com
†These authors have contributed equally to this work and share first authorship