- 1MoPlatte Sports Medicine, Ogallala, NE, United States
- 2LSU Health Sciences Center, New Orleans, LA, United States
A 78-year-old man with dementia experienced waxing and waning of symptoms with changes in altitude as he traveled from his home in the Rocky Mountains to lower elevations and back. To replicate the improvement in his symptoms with travel to lower elevations (higher pressure), the patient was treated with a near-identical repressurization in a hyperbaric chamber using compressed air. With four 1-h treatments at 1.3 Atmospheres Absolute (ATA) and concurrent administration of low-dose oral glutathione amino acid precursors, he recovered speech and showed improvement in activities of daily living. Regional broadcast media had documented his novel recovery. Nosocomial COVID-19 and withdrawal of hyperbaric air therapy led to patient demise 7 months after initiation of treatment. It is theorized that hyperbaric air therapy stimulated mitochondrial biochemical and physical changes, which led to clinical improvement.
Introduction
The effects of barometric pressure on human physiology and cognition date back to Evangelista Torricelli's invention of the barometer in 1643. In 1644, Torricelli observed, “We live submerged at the bottom of an ocean of the element air.”(1) Altitude ascents and descents in air have an effect on human physiology due to changes in both oxygen pressure and barometric pressure. The positive effects of descent from altitude on human pulmonary, rheumatic, and cardiac diseases have been documented during relocations of people from the Rocky Mountains (5000–10,000 ft above sea level) to the lower Missouri River Basin of the United States (~1000 ft above sea level) (2, 3). Kramer et al. (4) quantified and showed the durability of this phenomenon in chronic obstructive pulmonary disease (COPD) patients who relocated for 3 weeks from Jerusalem (altitude 800 m, 2500 ft, 13.4 psi, 0.91 ATA) to the Dead Sea (altitude 402 m of air below sea level 15.4 psi, 1.05 ATA). At the end of the Dead Sea relocation, parameters such as walking distance and maximum oxygen consumption improved and persisted for 2 weeks after returning to Jerusalem at 0.91ATA (4). The findings by Kramer et al. were substantiated by 50 years of physiological evidence of the bioactivity induced by pressure changes in the same range as his COPD patients who relocated from Jerusalem to the Dead Sea, moving from 1.0015 ATA to 1.3 ATA (5).
The opposite effect, i.e., worsening of COPD on ascent in “the ocean of air,” was observed by Dr. Orval Cunningham, professor of anesthesiology (University of Kansas), the specialty of gas physiology. While on a vacation to the Colorado Rocky Mountains during the Spanish flu pandemic of 1918, he became aware of the increased mortality of Spanish flu victims at higher altitude (6). He reasoned that, since a descent to lower elevations has a therapeutic effect on patients (2, 3), a further increase in pressure beyond sea level, i.e., to below “the ocean of air,” would have further benefit. Using a converted boiler as a hyperbaric chamber in Kansas City, Missouri (altitude 909 ft), Cunningham replicated this improvement caused by altitude descent on human disease when he treated an agonal Spanish flu patient in 1918, achieving complete recovery with four daily 1-h 1.6 ATA hyperbaric air treatment (HBAT) sessions (6, 7) Countless Spanish flu patients followed, and Cunningham extended his clinical treatment benefits to a variety of conditions (7–9). After a hundred years, his reasoning and success were replicated in the application of hyperbaric oxygen therapy (HBOT) to COVID-19 (10–12). and post-COVID “Long Haulers Syndrome.” (13–15).
The results obtained by Kramer et al.. (4) and Cunningham with compressed air (2, 3, 6–9) have been replicated in cerebral palsy children (16, 17), a drowned child (18), and two persistent postconcussion syndrome studies (19, 20). The pressure changes observed in these studies were in the range of the pressure changes observed in the Rocky Mountain/Great Plains relocators (2, 3) and the pressure changes experienced by our dementia patient on weekend trips from his home in the Rocky Mountains to western Nebraska. During these trips, he experienced clinical deteriorations and improvements that tracked altitude changes. Based on the above literature, the authors' personal experience, and the patient's clinical travel experience, the primary author replicated the clinical benefits experienced on the weekend trips to lower altitude (higher pressure) by treating his patient in a portable hyperbaric chamber. In this study, we report the sustained improvement in dementia with repetitive hyperbaric air therapy, using pressure similar to that used by others who have used altitude descent to improve their medical conditions (2–4).
Case description
The patient was a 78-year-old man with dementia who lived for 16 years in Gypsum, Colorado at 6400 feet (1944 m, 0.79 ATA, 11.6 psi) and was a summertime vacation home neighbor of the primary author in Lake McConaughy, Nebraska (3330 feet altitude, 972 m, 0.89 ATA, 13.0 psi). He was cognitively intact until a cerebral injury from an urgent cholecystectomy in the spring of 2019. Post-operatively, the family noticed an immediate cognitive change (“he was no longer himself”). The patient was reduced to social withdrawal, infrequent smiles, and little intelligible speech. He was subsequently diagnosed with dementia. In May 2019, his son-in-law attended a baseball game with the patient in Denver, Colorado (21) (altitude of 5200 feet, 1544 m, 0.83ATA, 12.1 psi) and noticed that he had become incoherent (delirious) around 4:00 PM during the middle innings of the game. Attendance at this game included travel from his home at an elevation of 6400 feet (0.79 ATA) over the Continental Divide (Eisenhower Tunnel, 11,111 feet, 3401 m, 0.66 ATA, 9.6 psi) and a pressure change of−0.13 ATA (see Figure 1). This was the first episode where the patient's sundowning had occurred well before its typical evening time.
Travel to the baseball game entailed 90 min of increasing hypobaric hypoxia from his home at 6400 ft (0.79 ATA) to the Eisenhower Tunnel (11,111 feet, 0.66 ATA) before descent to the baseball stadium at 5,280 ft (0.83 ATA). This included a peak stress of 15–20 min at the Eisenhower Tunnel. The early sundowning was confirmed by additional family members and the primary author's observations on multiple occasions during the summer of 2019 while traveling on the same route to his vacation home in Nebraska for weekend trips (3,330 ft., 0.89 ATA). After staying for a number of days at the decreased altitude (higher pressure), typically on the third day (Sunday afternoons), the family observed behavioral and symptomatic improvement. The primary author also witnessed the patient's cognitive deterioration post cholecystectomy, his further deterioration during visits of summer 2019, and rebounding by Sunday afternoons during vacation home visits.
The observations during the baseball game travel suggested that the hypobaric/hypoxic stress induced by road travel through Eisenhower Tunnel (0.66 ATA) on the patient's dementia (22, 23), a 0.13 ATA decrease in pressure and oxygen pressure, was similar to Cunningham's observations of Spanish flu patients in the Rocky Mountains (6). The lower-altitude higher-pressure rebound improvement observed in the mental status of the patients during Sunday afternoons, a 0.10 ATA increase from his hometown and a 0.23 ATA (3.4 psi) increase from the hypobaric/hypoxic stress zone of Eisenhower Tunnel (0.66 ATA), was in the range of bioactive pressure changes described by Kramer and Godfrey (4) (0.14 ATA increase in pressure), effects of altitude descent from the Rocky Mountains to the Great Plains (2, 3), recompression effects on patients with altitude sickness (24, 25), and the MacDonald–Fraser review of bioactivity in the micropressure range of 1.015–1.3 ATA (5). Based on the authors' previous low-pressure hyperbaric therapy experience with an Alzheimer's patient (26), a drowned child (18), and the medical literature (vide supra), the primary author proposed that the beneficial pressure changes experienced by the patient could be replicated in a portable chamber. Given the effects of HBOT on Nrf2 upregulation (27–29) and of NRF2 effects on intracellular glutathione (GSH) (30), supplemental GSH precursors were recommended adjunctively during hyperbaric treatment.
Treatment
The patient was initiated on daily 1.6 g sublingual glutathione amino acid precursors 3 weeks prior to hyperbaric treatment. The primary author and his wife trained the patient's spouse, children, and adult grandchildren on chamber operations, and hyperbaric treatment was commenced on 20 October 2019, approximately 6 months after cholecystectomy-induced cerebral injury. The treatments were carried out once/daily, 5 days/week, at 1.09 ATA/45–60 min of total treatment time for 20 treatments, 1-week break, repeat 20 treatments. The 0.3 ATA increase in pressure in each hyperbaric treatment was the same increase in pressure used in hyperbaric air treatment of a sub-acutely drowned 2-year-old girl in which global regrowth of brain tissue was demonstrated (18).
Outcome and follow-up
The patient received 14 hyperbaric treatments in 20 days. After the first four treatments, the improvements in speech, cognition, and activities of daily living were so significant that two television news stations in two different US states reported the patient's progress in separate broadcasts. See broadcast news links shown in Figures 2, 3 and Supplementary material.
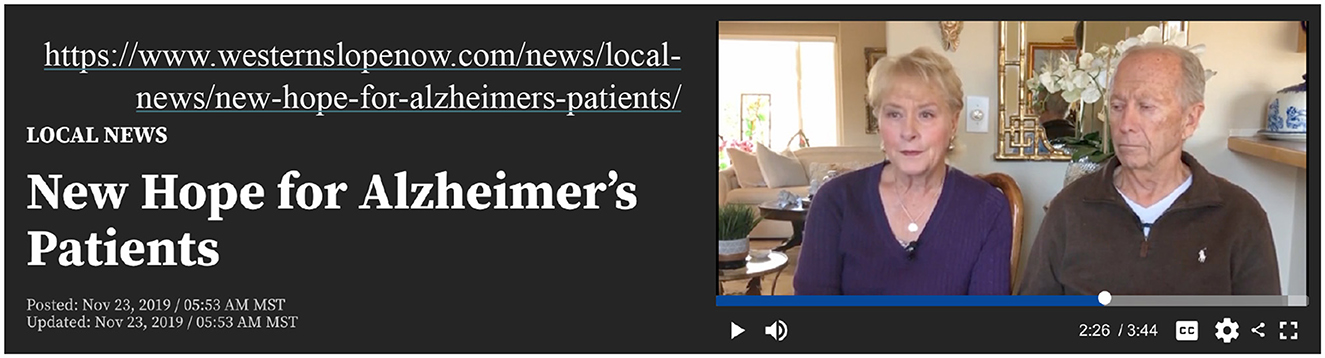
Figure 3. 11/23/2019 Local media news report of the patient and his wife after 14 hyperbaric treatments. The patient recounts his improvements in activities of daily living. At the 2:26 timestamp of the feature, the patient's spouse relates her husband telling her on day 4 of the therapy, “that machine is working, it's helping me - I know it is.” The patient had little to no functional speech before the treatment.
The broadcasts demonstrate the return of the patient's quick wit that had disappeared after the cholecystectomy and was still absent on the Sunday before the establishment of hyperbaric/glutathione therapy. The couple humorously referenced the matter of their upcoming 50th anniversary in March of 2020, with the patient stating that it should be remarkably different than what was expected before the establishment of the hyperbaric treatment.
Over the next 4 months, the patient received 67 HBATs at 0–5/week with 11.2 g of GSH precursors. He maintained his cognitive gains and re-engaged in exercising, carried out household chores, and performed activities of daily living until a hospitalization for a urinary tract infection led to him contracting COVID-19 (March, 2020). During the 2-month hospitalization, HBAT and GSH precursors were unavailable, and the patient experienced significant cognitive decline. He was discharged home on multidrug therapy due to worsened dementia/agitation in early May of 2020. He required significant in-home nursing support, was no longer speaking, was frequently combative, and was occasionally delirious, i.e., much worse clinically than when undergoing prehyperbaric therapy and GSH precursors 7 months before. The patient no longer remembered or understood the improvement he had experienced with hyperbaric treatment and refused all attempts at treatment, except for a 1-h session in early June 2020. That single hour of therapy caused an immediate calming of the patient, allowing a trip to the local grocery store later in the day (31). This was his first outing in over 3 months of institutional and home confinement.
Encouraged by the trip to the grocery store, the family traveled to their vacation home (0.89 ATA) the following weekend, incurring another hypobaric/hypoxic stress in the Eisenhower Tunnel (0.66 ATA). Once at the vacation home, the patient went missing, prompting a neighborhood manhunt that found him huddled in the crawl space under his home (31). The family returned to the Colorado home the following morning by the same route, incurring another hypobaric/hypoxic stress event through the Eisenhower Tunnel. Upon arrival, agitation worsened over the next several days, necessitating sedation. An extreme nocturnal delirious event occurred requiring physical restraint (88), and the patient expired during sleep within a few days.
Discussion
This case report demonstrates the sensitivity of a patient's dementia to altitude ascents and descents and the replication of beneficial altitude descent conditions with hyperbaric chamber air treatments of the same magnitude. An increase in altitude is a hypobaric/hypoxic stress to living organisms (1, 25). Altitude hypobaric/hypoxic stress is a routine event with nearly every commercial airline flight. Because of in-flight cardiac arrests in patients with coronary artery disease, automatic external defibrillators have been placed in commercial airlines (32). Commercial airline-induced hypobaric/hypoxic stress has also been demonstrated to induce acute delirium in elderly cognitively impaired patients with neurodegenerative disease (33). In younger individuals, Ewing et al. (34) reported cognitive deficits induced by hypobaric/hypoxic stress on asymptomatic college students with a 1–3-year-old mild traumatic brain injury. A traumatic brain injury (TBI) group and a matched control group underwent cognitive testing at 12,467 feet (0.63 ATA) in a hypobaric chamber. Testing at 0.63 ATA demonstrated a statistically significant reduction in cognition in the TBI group compared to the matched controls, and this value was equivalent to acutely concussed patients.
The opposite change in pressure, a decrease in altitude/increase in pressure, is a hyperbaric hyperoxic recompression, similar to the treatment of mountain sickness (25) and divers with decompression illness (35). Duplicating the experience of demented patients in Sadlon et al.'s review (33), our patient repetitively experienced a deterioration in his dementia symptoms with intermittent trips to over 4,700 feet higher than his home elevation of 6,400 feet (Figure 1). These exacerbations of dementia symptoms were ameliorated by sustained descent to a lower elevation than his home, as reported in the literature (2–4). To improve his dementia symptoms, the same degree of pressure increase in his altitude descents was repetitively duplicated in a home-portable hyperbaric chamber. Each hyperbaric treatment was nearly equivalent to a virtual 1-h transport from his home altitude (0.79 ATA) in the Colorado Rockies to a theoretical landing in the Dead Sea (1.05 ATA), as described for the patients in the study by Krramer et al. (4). Fourteen repetitive hyperbaric air recompressions (“Dead Sea excursions), in combination with glutathione precursors to combat oxidative stress and inflammation, achieved marked cognitive improvement. The improvement was sustained for 4 months by additional hyperbaric and precursor therapy until the patient's demise from COVID-19 brain injury and repetitive hypobaric/hypoxic stress during altitude changes.
This case suggests the bioactivity of the unappreciated component of hyperbaric therapy, barometric pressure, due to the known similar effects in treatment of mountain sickness (25). HBOT has been traditionally defined as the use of 100% oxygen at > 1.4 ATA (35), and more recently > 1.0 ATA (36). These definitions ignored the effect of barometric pressure until the United States FDA pointed this out in 2011 (37). Recent gene expression experiments indicate that barometric pressure may be the dominant component of hyperbaric and hyperbaric oxygen therapy (38). In our patient, the recompression during motor vehicle travel to lower elevations caused equal increases in oxygen and barometric pressure, but the overall pressure of oxygen and amount of oxygen are a fraction of the typical pressures and amounts used in clinical hyperbaric oxygen therapy (36). Such small increases in oxygen and pressure have shown biological (5) and significant clinical effects for altitude sickness (25) but are not appreciated as a treatment for chronic neurological disease. In our case, the initial hyperbaric chamber treatments could not be construed as treating acute injury from the hypobaric/hypoxic trips to over 11,000 feet since our patient had been at his Gypsum home altitude (0.79 ATA) for 6 weeks prior to initiating hyperbaric treatment. The repetitive treatments appeared to duplicate the well-known trophic healing effects of repetitive hyperbaric therapy in other chronic conditions (36). The clinical improvements were durable until acute neurological injury from COVID-19 infection and repetitive hypobaric/hypoxic insults caused his death. His death mirrored the observation of altitude intolerance of chronic disease patients (2, 3, 6) and Dr. Cunnmingham's observation of increased mortality of Rocky Mountain dwelling patients with Spanish Flu (6), a disease very similar to COVID-19.
The precedence for our patient's improvement dates back to the 300-year initial history of hyperbaric therapy, which consisted solely of compressed air usage (6). This experience, long-forgotten by the modern hyperbaric oxygen therapy community, is undergoing a re-validation with accumulating animal and clinical studies documenting the bioactivity of lower pressures of compressed air, oxygen-enriched compressed air, and 100% oxygen in a variety of conditions (4, 16, 17, 19, 20, 39–43). Our patient's experience and this body of literature strongly indicate HBAT and low-pressure HBOT as therapeutic options for human disease.
The contribution of glutathione (GSH) amino acid precursors to our patient's recovery is impossible to prove but is suggested by the neuroprotective properties of GSH (44). GSH is an essential antioxidant against reactive oxygen and nitrogen species and is critical in regulating mitochondrial function, maintaining cell redox homeostasis, cell cycle regulation, apoptosis, immunological defense, and modulating pathological abnormalities (45, 46). Its deficiency is centrally involved in the pathophysiology of inflammation and many diseases, particularly Parkinson's disease, Alzheimer's disease, and amyotrophic lateral sclerosis (46–48). The glutathione amino acid formulation administered to our patient has been shown to increase intracellular glutathione (GSH) levels in cell cultures infected with RNA viruses (49).
Intracellular GSH levels are controlled by Nrf2 expression (30). Nrf2 regulates the cell's adaptive response to oxidants and electrophiles (30) and is increased (28, 29) and decreased (50) in diseases and hypobaric/hypoxic stress (51). Nrf2 upregulation has achieved disease amelioration in multiple conditions with multiple therapeutic agents (47, 52). This “systems medicine mechanism-based approach” to disease treatment (53) has described Nrf2 upregulation therapy as a “several diseases, one medicine” therapy; in summary, it is pleiotropic (47). Nrf2 is also upregulated by hyperbaric oxygen therapy in normal human endothelial cells (27) and in diseases (28, 29). We speculate that combining GSH precursors with HBAT caused a synergistic elevation of GSH, improvement of mitochondrial function through a mitohormesis effect, and clinical improvement in our patient. Upregulation of Nrf2 by hyperbaric therapy may explain the potentially unjustified criticism of widespread historical application to 132 diseases (54).
This case report did not include functional imaging documentation. In previous reports, we have documented HBOT-induced changes in chronic neurological disease with functional imaging changes in both SPECT (55–58) and PET (26). For example, the videographic fused images in Supplemental material and bookend still images of Figure 4 show improvement of function greater than 30% across the regions of interest obtained. The fused SPECT data set was obtained as the “prognosticator” data set of the whole brain after the first hour of HBOT at 1.5ATA. These images indirectly suggest the initial overexpression of Nrf2-generated GSH as stimulated by reactive oxygen and reactive nitrogen species (60). In the presented HBAT case, it is surmised that supplemental GSH precursors and hyperbaric air therapy contributed a greater percentage of reactive nitrogen species to the overall effect due to the 4:1 ratio of nitrogen to oxygen in air. SPECT in these cases may have been a proxy for Nrf2 upregulation. The intracellular trapping of the HMPAO SPECT radiopharmaceutical is mediated by glutathione levels in astrocytes (61). The HBOT SPECT brain blood flow and associated clinical improvements may have resulted, in part, from Nrf2-induced increases in astrocyte GSH. In the absence of HBAT and GSH precursors, we further speculate that the patient's cognitive decline post COVID-19 was due to a decline in intracellular glutathione. Glutathione depletion has been suggested as a major cause of the morbidity and mortality of COVID-19 (62).
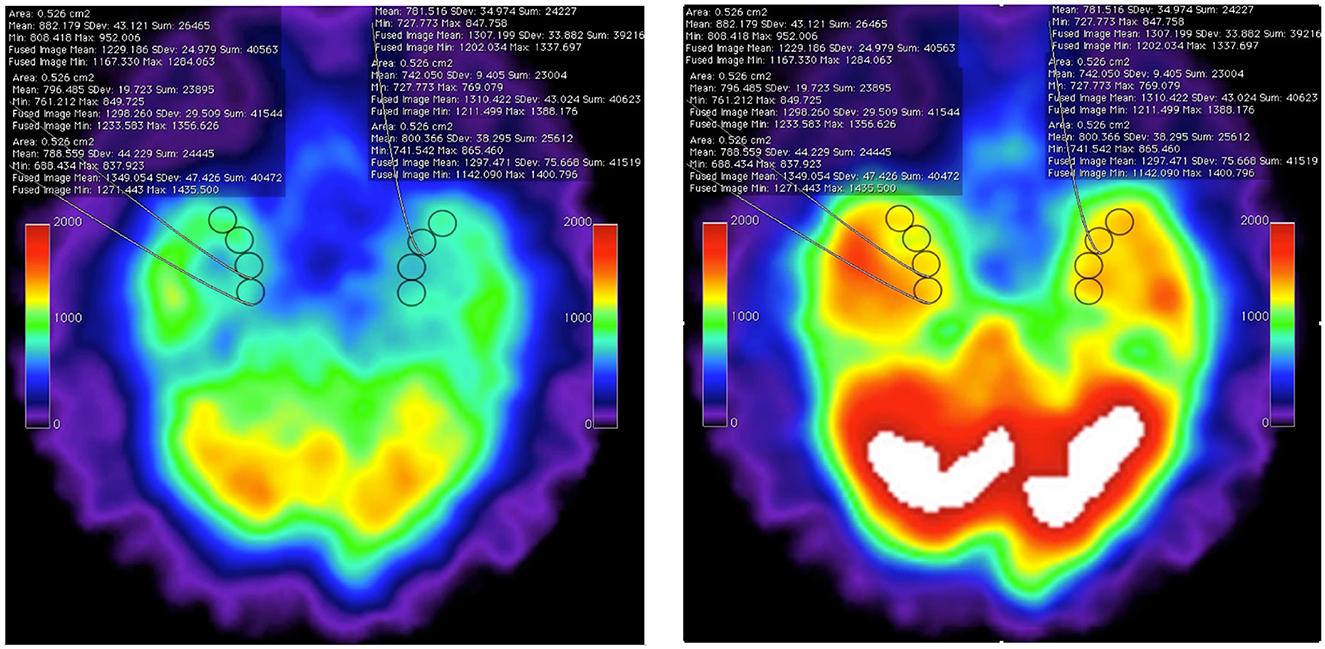
Figure 4. Axial SPECT imaging data from pre-HBOT baseline (left) and post 1-h HBOT data set (right) showing the inferior temporal lobes with eight regions of interest (ROI) in the medial temporal lobes. The statistical overlay documents increased intracellular GSH levels by Tc-99 HMPAO sequestration. These still images are captured from frame 1 and frame 20 of post-processed fusion of the two individual datasets performed on open-source Osirix clinical workstation software (see the source fusion video in Supplementary material). The “fused image” statistical data are obtained from the post-1-h HBOT HMPAO SPECT exam. Note that, at all ROIs, the uptake is increased over baseline by <30% [research data, Harch et al. (59)].
Conclusion
Cognitive deteriorations and improvements occurred in an elderly dementia patient with hypobaric/hypoxic motor vehicle ascents and descents from altitude. Temporary beneficial motor vehicle descents from altitude were replicated in a portable hyperbaric chamber. Fourteen hyperbaric treatments and oral glutathione precursor supplements resulted in cognitive improvements that were documented in television news mini-documentaries. The improvements were sustained for 4 months with additional hyperbaric and supplement treatment until the patient's hospital admission for UTIs, leading to nosocomial COVID-19 infection. Patient death was due to long COVID-19 dementia exacerbated by repetitive altitude insults. Hyperbaric-induced upregulation of NRF2-mediated glutathione synthesis is suggested as a contributory mechanism of action.
Data availability statement
The datasets presented in this article are not readily available because of ethical and privacy restrictions. Requests to access the datasets should be directed to the corresponding author.
Ethics statement
Ethical review and approval was not required for the study on human participants in accordance with the local legislation and institutional requirements. Written informed consent from the patients/participants or patients/participants' legal guardian/next of kin was not required to participate in this study in accordance with the national legislation and the institutional requirements. Written informed consent was obtained from the individual(s) for the publication of any potentially identifiable images or data included in this article. Written informed consent was obtained from the participant/patient(s) for the publication of this case report.
Author contributions
EF: Conceptualization, Data curation, Formal analysis, Funding acquisition, Investigation, Methodology, Project administration, Resources, Software, Supervision, Validation, Visualization, Writing – original draft, Writing – review & editing. PH: Data curation, Methodology, Conceptualization, Formal analysis, Project administration, Investigation, Writing – review & editing.
Funding
The author(s) declare that financial support was received for the research, authorship, and/or publication of this article. The publication of this article was partially funded by the North Dakota CARES ACT Grant EF received in October 2020 for the development and communications of Medical Countermeasures to COVID19 based on the protocols EF employed in this case report.
Acknowledgments
The authors thank the family of Robert S. Kostka (1941-2020), originally from Fargo, North Dakota for the privilege of working together on this medical home based “altitude wellness” project as their experience and advocacy has helped many families in the Great Plains and Rocky Mountain jurisdictions of the United States of America.
Conflict of interest
EF owns multiple hyperbaric chambers and medical imaging devices used in his practice of clinical medicine. From 2016 to 2020, MoPlatte Sports Medicine was the entity under which EF was a distributor for The ProImmune Company, LLC. EF remains a consultant to the inventor of ProImmune Immune Formulation 200, Albert B. Crum, MD (ABC). ABC's Canadian Patent (https://www.ic.gc.ca/opic-cipo/cpd/eng/patent/2963131/summary.html?) was a primary reference for the North Dakota CARES ACT Grant Committee approval of EFF's natropathic/medical countermeasures for COVID19 covered under the United States Federal PREP ACT of 2020. EF is a consultant for Joseph V. Cassarino, the current distributor of ProImmune Immune Formulation 200 at www.theBestImmuneSupport.com which has provided partial funding support in conjunction with the North Dakota CARES ACT grant support for the broadcasting of the hyperbaric and GSH pre-cursor medical countermeasures to COVID19 developed through EFF's clinical experience in this case on the following FCC regulated communications platforms: KFYR, KXEL, KCRO, WXJB, and KXNET. PH is the owner of an S Corporation that is the vehicle for his practice of hyperbaric medicine. He also performs consulting and gives expert witness testimony under this S Corp.
Publisher's note
All claims expressed in this article are solely those of the authors and do not necessarily represent those of their affiliated organizations, or those of the publisher, the editors and the reviewers. Any product that may be evaluated in this article, or claim that may be made by its manufacturer, is not guaranteed or endorsed by the publisher.
Supplementary material
The Supplementary Material for this article can be found online at: https://www.frontiersin.org/articles/10.3389/fneur.2024.1356662/full#supplementary-material
References
1. West JB. Early history of high-altitude physiology. Ann N Y Acad Sci. (2016) 1365:33–42. doi: 10.1111/nyas.12719
2. Cunningham OJ. Oxygen therapy by means of compressed air. Anesth Analg. (1927) 6:64–6. doi: 10.1213/000005 39-192704000-00004
3. Midtownkcposter. Why Was There a Huge Steel Tank at 33rd and Harrison in the 1920s? Midtown KC Post. Available online at: https://midtownkcpost.com/why-was-there-a-huge-steel-tank-at-33rd-and-harrison-in-the-1920s/ (accessed April 25, 2024).
4. Kramer MR, Godfrey S. Dead Sea: natural oxygen enrichment at low altitude. Isr J Med Sci. (1996) 32:S20–3.
5. MacDonald AG, Fraser PJ. The transduction of very small hydrostatic pressures. Comp Biochem Physiol Mol Integr Physiol. (1999) 122:13–36. doi: 10.1016/S1095-6433(98)10173-3
7. Sellers LM. The Fallibility of the Forrestian Principle: Semper Primus Pervenio Maxima Cum Vi. Anesth Analg. (1965) 44:39–41. doi: 10.1213/00000539-196501000-00009
8. Alexander SC. Holding court with the ghost of gilman terrace: selected writings of ralph Milton waters. Anesth Anal. (2003) 96:1847. doi: 10.1097/00000539-200306000-00076
9. Waters RM. Cunningham theory revisited. Anesth Analg. (1965) 44:469–73. doi: 10.1213/00000539-196507000-00029
10. Harch PG. Hyperbaric oxygen treatment of novel coronavirus (COVID-19) respiratory failure. Med Gas Res. (2020) 10:61–2. doi: 10.4103/2045-9912.282177
11. Gorenstein SA, Castellano ML, Slone ES, Gillette B, Liu H, Alsamarraie C, et al. Hyperbaric oxygen therapy for COVID-19 patients with respiratory distress: treated cases versus propensity-matched controls. Undersea Hyperb Med. (2020) 405–13. doi: 10.22462/03.07.2020.1
12. Keller GA, Colaianni I, Coria J, Di Girolamo G, Miranda S. Clinical and biochemical short-term effects of hyperbaric oxygen therapy on SARS-Cov-2+ hospitalized patients with hypoxemic respiratory failure. Respir Med. (2023) 209:107155. doi: 10.1016/j.rmed.2023.107155
13. Huang P, Zhang LY, Tan YY, Chen SD. Links between COVID-19 and Parkinson's disease/Alzheimer's disease: reciprocal impacts, medical care strategies and underlying mechanisms. Transl Neurodegener. (2023) 12:5. doi: 10.1186/s40035-023-00337-1
14. Taquet M, Sillett R, Zhu L, Mendel J, Camplisson I, Dercon Q, et al. Neurological and psychiatric risk trajectories after SARS-CoV-2 infection: an analysis of 2-year retrospective cohort studies including 1 284 437 patients. The Lancet Psychiatry. (2022) 9:815–27. doi: 10.1016/S2215-0366(22)00260-7
15. Hadanny A, Zilberman-Itskovich S, Catalogna M, Elman-Shina K, Lang E, Finci S, et al. Long term outcomes of hyperbaric oxygen therapy in post covid condition: longitudinal follow-up of a randomized controlled trial. Sci Rep. (2024) 14:3604. doi: 10.1038/s41598-024-53091-3
16. Collet JP, Vanasse M, Marois P, Amar M, Goldberg J, Lambert J, et al. Hyperbaric oxygen for children with cerebral palsy: a randomised multicentre trial. Lancet. (2001) 357:582–6. doi: 10.1016/S0140-6736(00)04054-X
17. Mukherjee A, Raison M, Sahni T, Arya A, Lambert J, Marois P, et al. Intensive rehabilitation combined with HBO2 therapy in children with cerebral palsy: a controlled longitudinal study. Undersea Hyperb Med. (2014) 41:77–85.
18. Harch PG, Fogarty EF. Subacute normobaric oxygen and hyperbaric oxygen therapy in drowning, reversal of brain volume loss: a case report. Med Gas Res. (2017) 7:144–9. doi: 10.4103/2045-9912.208521
19. Wolf G, Cifu DX, Baugh L, Carne W, Profenna L. The effect of hyperbaric oxygen on symptoms following mild traumatic brain injury. J Neurotrauma (2012). (2549) 29:2606–12. doi: 10.1089/neu.2012.2549
20. Miller RS, Weaver LK, Bahraini N, Churchill S, Price RC, Skiba V, et al. Effects of hyperbaric oxygen on symptoms and quality of life among service members with persistent postconcussion symptoms: a randomized clinical trial. JAMA Intern Med. (2015) 175:43–52. doi: 10.1001/jamainternmed.2014.5479
21. Coors Field (Denver) – Society for American Baseball Research. Available online at: https://sabr.org/bioproj/park/coors-field-denver/ (accessed March 15, 2024).
22. Lieberman P, Morey A, Hochstadt J, Larson M, Mather S. Mount Everest: a space analogue for speech monitoring of cognitive deficits and stress. Aviat Space Environ Med. (2005) 76:B198–207.
23. About the Eisenhower-Johnson Memorial Tunnel. Colorado Department of Transportation. Available online at: https://www.codot.gov/travel/ejmt (accessed March 15, 2024).
24. Jay GD, Tetz DJ, Hartigan CF, Lane LL, Aghababian RV. Portable hyperbaric oxygen therapy in the emergency department with the modified Gamow bag. Ann Emerg Med. (1995) 26:707–11. doi: 10.1016/S0196-0644(95)70042-0
25. Kasic JF, Yaron M, Nicholas RA, Lickteig JA, Roach R. Treatment of acute mountain sickness: hyperbaric versus oxygen therapy. Ann Emerg Med. (1991) 20:1109–12. doi: 10.1016/S0196-0644(05)81385-X
26. Harch PG, Fogarty EF. Hyperbaric oxygen therapy for Alzheimer's dementia with positron emission tomography imaging: a case report. Med Gas Res. (2018) 8:181–4. doi: 10.4103/2045-9912.248271
27. Godman CA, Chheda KP, Hightower LE, Perdrizet G, Shin D-G, Giardina C. Hyperbaric oxygen induces a cytoprotective and angiogenic response in human microvascular endothelial cells. Cell Stress Chaperones. (2010) 15:431–42. doi: 10.1007/s12192-009-0159-0
28. Meng XE, Zhang Y, Li N, Fan DF, Yang C, Li H, et al. Effects of hyperbaric oxygen on the Nrf2 signaling pathway in secondary injury following traumatic brain injury. Genet Mol Res. (2016) 15:gmr.15016933. doi: 10.4238/gmr.15016933
29. Dhamodharan U, Karan A, Sireesh D, Vaishnavi A, Somasundar A, Rajesh K, et al. Tissue-specific role of Nrf2 in the treatment of diabetic foot ulcers during hyperbaric oxygen therapy. Free Radic Biol Med. (2019) 138:53–62. doi: 10.1016/j.freeradbiomed.2019.04.031
30. Ma Q. Role of Nrf2 in oxidative stress and toxicity. Annu Rev Pharmacol Toxicol. (2013) 53:401–26. doi: 10.1146/annurev-pharmtox-011112-140320
31. Cheng ST. Dementia caregiver burden: a research update and critical analysis. Curr Psychiatry Rep. (2017) 19:1–8. doi: 10.1007/s11920-017-0818-2
32. Defibrillators on Commercial Airlines. Available online at: https://simpleflying.com/do-commercial-aircraft-carry-defibrillators-onboard/#:~:text=In%202004%2C%20the%20Federal~%20Aviation,be%20trained%20to%20use%20it (accessed May 22, 2024).
33. Sadlon A, Ensslin A, Freystätter G, Gagesch M, Bischoff-Ferrari HA. Are patients with cognitive impairment fit to fly? Current evidence and practical recommendations. J Travel Med. (2021) 28:taaa123. doi: 10.1093/jtm/taaa123
34. Ewing R, McCarthy D, Gronwall D, Wrightson P. Persisting effects of minor head injury observable during hypoxic stress. J Clin Exp Neuropsychol. (1980) 2:147–55. doi: 10.1080/01688638008403789
35. Hampson NB. Hyperbaric Oxygen Therapy: 1999 Committee Report. Kensington, MD: Undersea and Hyperbaric Medical Society (1999).
36. Moon RE. Hyperbaric Oxygen Therapy Indications, 14th Edn. North Palm Beach, FL: Best Publishing Company. (2019).
37. Harch PG. Oxygen and pressure epigenetics: understanding hyperbaric oxygen therapy after 355 years as the oldest gene therapy known to man. Townsend Lett. (2018) 417:30–4. Available online at: https://www.townsendletter.com/article/oxygen-and-pressure-epigenetics-understanding-hyperbaric-oxygen-therapy-after-355-years-as-the-oldest-gene-therapy-known-to-man/ (accessed May 22, 2024).
38. Chen Y, Nadi NS, Chavko M, Auker CR, McCarron RM. Microarray analysis of gene expression in rat cortical neurons exposed to hyperbaric air and oxygen. Neurochem Res. (2009) 34:1047–56. doi: 10.1007/s11064-008-9873-8
39. Ishihara A. Mild hyperbaric oxygen: mechanisms and effects. The J Physiol Sci. (2019) 69:573–80. doi: 10.1007/s12576-019-00678-5
40. Rusyniak DE, Kirk MA, May JD, Kao LW, Brizendine EJ, Welch JL, et al. Hyperbaric oxygen Therapy in acute ischemic stroke: results of the hyperbaric oxygen in acute ischemic stroke trial pilot study. Stroke. (2003) 34:571–4. doi: 10.1161/01.STR.0000050644.48393.D0
41. Rossignol DA, Rossignol LW, Smith S, Schneider C, Logerquist S, Usman A, et al. Hyperbaric treatment for children with autism: a multicenter, randomized, double-blind, controlled trial. BMC Pediatr. (2009) 9:21. doi: 10.1186/1471-2431-9-21
42. Malek M, Duszczyk M, Zyszkowski M, Ziembowicz A, Salinska E. Hyperbaric oxygen and hyperbaric air treatment result in comparable neuronal death reduction and improved behavioral outcome after transient forebrain ischemia in the gerbil. Exp Brain Res. (2013) 224:1–14. doi: 10.1007/s00221-012-3283-5
43. MacLaughlin KJ, Barton GP, Braun RK, MacLaughlin JE, Lamers JJ, Marcou MD. Hyperbaric air mobilizes stem cells in humans; a new perspective on the hormetic dose curve. Front Neurol. (2023) 14:1192793. doi: 10.3389/fneur.2023.1192793
44. Pérez-Sala D, Pajares MA. Appraising the role of astrocytes as suppliers of neuronal glutathione precursors. Int J Mol Sci. (2023) 24:8059. doi: 10.3390/ijms24098059
45. Alam MM, Kishino A, Sung E, Sekine H, Abe T, Murakami S, et al. Contribution of NRF2 to sulfur metabolism and mitochondrial activity. Redox Biol. (2023) 60:102624. doi: 10.1016/j.redox.2023.102624
46. Vašková J, Kočan L, Vaško L, Perjési P. Glutathione-related enzymes and proteins: a review. Molecules. (2023) 28:1447. doi: 10.3390/molecules28031447
47. Morris G, Walker AJ, Walder K, Berk M, Marx W, Carvalho AF, et al. Increasing Nrf2 activity as a treatment approach in neuropsychiatry. Mol Neurobiol. (2021) 58:2158–82. doi: 10.1007/s12035-020-02212-w
48. Aoyama K, Nakaki T. Impaired glutathione synthesis in neurodegeneration. Int J Mol Sci. (2013) 14:21021–44. doi: 10.3390/ijms141021021
49. Vasireddi M, Crum A, May H, Katz D, Hilliard J. A novel antiviral inhibits Zika virus infection while increasing intracellular glutathione biosynthesis in distinct cell culture models. Antiviral Res. (2019) 161:46–52. doi: 10.1016/j.antiviral.2018.09.004
50. Verma R, Chopra A, Giardina C, Sabbisetti V, Smyth JA, Hightower E. Hyperbaric oxygen therapy (HBOT) suppresses biomarkers of cell stress and kidney injury in diabetic mice. Cell Stress Chaperones. (2015) 20:495–505. doi: 10.1007/s12192-015-0574-3
51. Paul S, Gangwar A, Bhargava K, Ahmad Y. STAT3-RXR-Nrf2 activates systemic redox and energy homeostasis upon steep decline in pO2 gradient. Redox Biol. (2018) 11:423–38. doi: 10.1016/j.redox.2017.10.013
52. Zhou XD, Wang JL, Guo DD, Jiang WW Li ZK, Wang L, et al. Neuroprotective effect of targeted regulatory Nrf2 gene on rats with acute brain injury induced by carbon monoxide poisoning. Environ Toxicol. (2021) 36:1742–57. doi: 10.1002/tox.23295
53. Cuadrado A, Manda G, Hassan A, Alcaraz MJ, Barbas C, Daiber A, et al. Transcription fator NRF2 as a therapeutic target for chronic diseases: a systems medicine approach.
54. Gabb G, Robin ED. Hyperbaric oxygen, a thearpy in search of diseases. Chest. (1987) 92:1074–82. doi: 10.1378/chest.92.6.1074
55. Harch PG, Fogarty EF, Staab PK, Van Meter K. Low pressure hyperbaric oxygen therapy and SPECT brain imaging in the treatment of blast-induced chronic traumatic brain injury (post-concussion syndrome) and post-traumatic stress disorder: a case report. Cases J. (2009) 2:6538. doi: 10.4076/1757-1626-2-6538
56. Harch PG, Andrews SR, Fogarty EF, Lucarini J, Van Meter KW. Case control study: hyperbaric oxygen treatment of mild traumatic brain injury persistent post-concussion syndrome and post-traumatic stress disorder. Med Gas Res. (2017) 7:156–74. doi: 10.4103/2045-9912.215745
57. Harch PG. Late Treatment of Decompression Illness and Use of SPECT Brain Imaging. In RE Moon, PE Sheffield, editors Treatment of Decompression Illness. 45th Workshop of the Undersea and Hyperbaric Medical Society. Kensington, MD: UHMS, 203–242.
58. Van Meter KW, Harch PG, Andrews LC, Simanonok JP, Staab PK, Gottlieb SF. Should the pressure be off or on in the use of oxygen in the treatment of carbon monoxide-poisoned patients? Ann Emerg Med. (1994) 24:283–8. doi: 10.1016/S0196-0644(05)83748-5
59. Harch PG, Andrews SR, Fogarty EF, Amen D, Pezzullo JC, Lucarini J, et al. A phase I study of low-pressure hyperbaric oxygen therapy for blast-induced post-concussion syndrome and post-traumatic stress disorder. J Neurotrauma. (2012) 29:168–85. doi: 10.1089/neu.2011.1895
60. Poff AM, Kernagis D, D'Agostino DP. Hyperbaric environment: oxygen and cellular damage versus protection. Compr Physiol. (2011) 7:213–34. doi: 10.1002/cphy.c150032
61. Zerarka S, Pellerin L, Slosman D, Magistretti PJ. Astrocytes as a predominant cellular site of 99mTc-HMPAO retention. J Cereb Blood Flow Metab. (2001) 21:456–68. doi: 10.1097/00004647-200104000-00014
Keywords: hyperbaric, glutathione, pre-cursors, altitude, wellness, pandemic, dementia, COVID-19
Citation: Fogarty EF and Harch PG (2024) Case report: Dementia sensitivity to altitude changes and effective treatment with hyperbaric air and glutathione precursors. Front. Neurol. 15:1356662. doi: 10.3389/fneur.2024.1356662
Received: 15 December 2023; Accepted: 10 May 2024;
Published: 19 June 2024.
Edited by:
Dominic D'Agostino, University of South Florida, United StatesReviewed by:
Anandakumar Shunmugavel, Case Western Reserve University, United StatesXuehua Liu, Capital Medical University, China
Copyright © 2024 Fogarty and Harch. This is an open-access article distributed under the terms of the Creative Commons Attribution License (CC BY). The use, distribution or reproduction in other forums is permitted, provided the original author(s) and the copyright owner(s) are credited and that the original publication in this journal is cited, in accordance with accepted academic practice. No use, distribution or reproduction is permitted which does not comply with these terms.
*Correspondence: Edward F. Fogarty, ZWZvZ2FydHkmI3gwMDA0MDtyZWFscmFkcy5jb20=; bW9wbGF0dGUmI3gwMDA0MDtpY2xvdWQuY29t