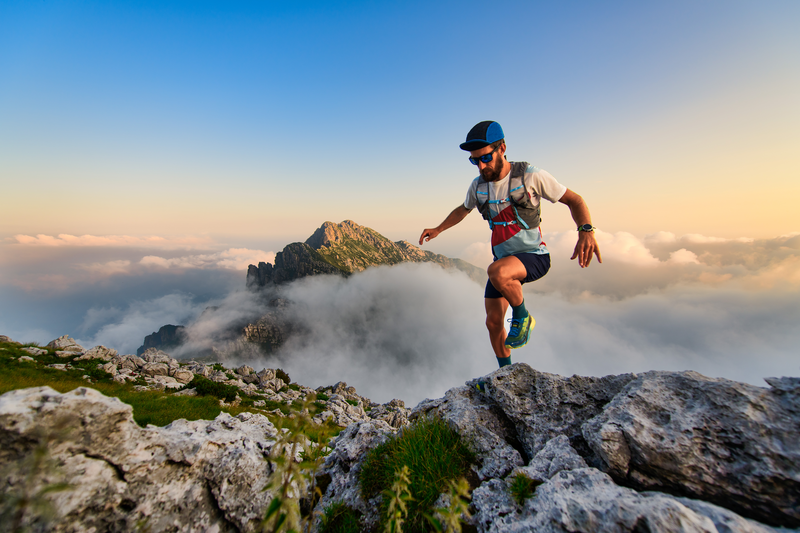
94% of researchers rate our articles as excellent or good
Learn more about the work of our research integrity team to safeguard the quality of each article we publish.
Find out more
SYSTEMATIC REVIEW article
Front. Neurol. , 10 April 2024
Sec. Cognitive and Behavioral Neurology
Volume 15 - 2024 | https://doi.org/10.3389/fneur.2024.1347991
Background: Nerve blocks are widely used in various surgeries to alleviate postoperative pain and promote recovery. However, the impact of nerve block on delirium remains contentious. This study aims to systematically evaluate the influence of Thoracic Paravertebral Nerve Block (TPVB) on the incidence of delirium in patients post Video-Assisted Thoracoscopic Surgery (VATS).
Methods: We conducted a systematic search of PubMed, Embase, Web of Science, Cochrane Library, and Scopus databases in June 2023. The search strategy combined free-text and Medical Subject Headings (MeSH) terms, including perioperative cognitive dysfunction, delirium, postoperative cognitive dysfunction, paravertebral nerve block, thoracic surgery, lung surgery, pulmonary surgery, and esophageal/esophagus surgery. We utilized a random effects model for the analysis and synthesis of effect sizes.
Results: We included a total of 9 RCTs involving 1,123 participants in our study. In VATS, TPVB significantly reduced the incidence of delirium on postoperative day three (log(OR): −0.62, 95% CI [−1.05, −0.18], p = 0.01, I2 = 0.00%) and postoperative day seven (log(OR): −0.94, 95% CI [−1.39, −0.49], p < 0.001, I2 = 0.00%). Additionally, our study indicates the effectiveness of TPVB in postoperative pain relief (g: −0.82, 95% CI [−1.15, −0.49], p < 0.001, I2 = 72.60%).
Conclusion: The comprehensive results suggest that in patients undergoing VATS, TPVB significantly reduces the incidence of delirium and notably diminishes pain scores.
Systematic review registration: CRD42023435528. https://www.crd.york.ac.uk/PROSPERO.
For Lung cancer serves as a prominent contributor to cancer-related fatalities across the globe, accounting for nearly a quarter of all cancer-related deaths (1). Despite recent advancements in targeted therapies, immunotherapy, and radiation treatment for lung cancer, early-stage surgical resection remains the predominant curative strategy for the vast majority of patients (2). Video-Assisted Thoracoscopic Surgery (VATS) remains the favored technique for surgical resection in early-stage lung cancer (3). Nonetheless, the rate of postoperative complications in elderly patients who undergo thoracic surgery varies from 12 to 47%, with delirium frequently observed (4). Postoperative delirium is defined as delirium occurring within one week after surgery or before the patient’s discharge from the hospital (whichever occurs earlier), meeting the diagnostic criteria for delirium as outlined in the fifth edition of the Diagnostic and Statistical Manual of Mental Disorders (5). Delirium is categorized into two types based on timing: emergence delirium and postoperative delirium (6, 7). Additionally, delirium is divided into three subtypes based on activity levels: hypoactive delirium, hyperactive delirium, and mixed-type delirium (8, 9).
The presence of delirium has been definitively associated with higher patient mortality rates, reduced comfort during hospital stays, prolonged discharge times, increased hospital expenses, and additional strain on both patients’ families and the healthcare system (10, 11). Recent studies indicate that the implementation of suitable preventive measures can effectively decrease the occurrence of delirium (12), thereby improving patient comfort and the overall quality of healthcare.
Current studies suggest that delirium arises from a combination of various factors, where neuroinflammation plays a pivotal role in both its onset and progression (13, 14). Pain is universally recognized as a leading risk factor for post-surgical delirium (15). Nerve block is believed to have the potential to decrease postoperative pain (16, 17) and diminish the stress and inflammatory responses triggered by surgery (18–21), thus potentially reducing the occurrence of postoperative delirium. However, there is still an ongoing debate regarding whether nerve block can effectively lower the incidence of delirium (22, 23). This study aims to investigate the potential impact of Thoracic Paravertebral Nerve Block (TPVB) on postoperative delirium in patients undergoing VATS.
This study rigorously adhered to the principles outlined in the Preferred Reporting Items for Systematic Reviews and Meta-Analyses (PRISMA) (24) and Assessing the methodological quality of Systematic Reviews (AMSTAR) (25) guidelines. All data incorporated into our study were exclusively derived from published literature, obviating the necessity for ethical review. Furthermore, the study is registered in the International Prospective Register of Systematic Reviews (PROSPERO CRD42023435528).
In June 2023, a comprehensive search was carried out across various databases, including PubMed, Embase, Web of Science, Cochrane Library, and Scopus. The retrieval strategy combines a mixture of free-text and MeSH terms, including perioperative neurocognitive disorders, delirium, postoperative cognitive dysfunction, paravertebral nerve block, thoracic surgery, pulmonary surgery, lung surgery, esophageal/esophagus surgery, and others (Supplementary Appendix 1).
Two independent researchers conducted a comprehensive and independent assessment and review of the literature, covering titles, abstracts, and full-text articles, to determine the final inclusion of studies in this research. Any disagreements were resolved through discussion, and in cases where a consensus could not be reached, a third researcher intervened to make the final decision.
Inclusion Criteria.
The following criteria were employed for the inclusion of studies:
1. The literature must comprise a randomized controlled trial.
2. The study subjects must be patients undergoing thoracic or pulmonary surgery.
3. Paravertebral nerve block must be administered during the perioperative period.
4. The literature must evaluate the incidence of delirium.
5. Literature from the control group must also be incorporated into the study.
Exclusion Criteria.
The following criteria were applied for the exclusion of studies:
1. Literature classified as case reports.
2. Observational studies.
3. Retrospective cohort studies.
4. Review articles.
5. Trial protocols.
6. Insufficient or unclear data within the literature.
7. Inability to access the full text or contact the authors.
We have developed a data extraction table and conducted a preliminary test. Subsequently, two researchers independently conducted data extraction. Any discrepancies in data extraction were thoroughly discussed. In cases where the two independent researchers were unable to reach a consensus, a third researcher was consulted to make the final decision. The data extraction table comprises the following key elements: author names, publication year, study design, participant age, participant count, type of surgery, anesthesia agent types and dosages, Delirium incidence, and postoperative pain scores. These data were primarily sourced from numerical data presented in tables and figures. We employed the online tool WebPlotDigitizer (Version 4.6; WebPlotDigitizer, A. Rohatgi, Pacifica, CA, United States) to extract data presented in graphical form. We employed the equation proposed by Wan et al. to estimate the mean and standard deviation of data described with medians (interquartile range) (26).
We employed the Cochrane Collaboration’s Risk of Bias 2 tool (27) to assess potential bias in the included studies. This comprehensive tool evaluates various aspects, including random sequence generation, allocation concealment, blinding of patients, healthcare providers, data collectors, and outcome assessors, completeness of outcome data, selective outcome reporting, and other potential sources of bias. Each article was then categorized into one of three risk levels: “low,” “some concerns,” or “high.” To evaluate the quality of evidence for each outcome, we applied the Grading of Recommendations, Assessment, Development, and Evaluation (GRADE) methodology (28). This systematic approach allowed us to categorize the quality of evidence as either very low, low, moderate, or high.
We carried out data analysis leveraging Stata 17.0 and Review Manager 5.4 software platforms. To gauge the extent of variability among the selected studies, we employed τ2 (Tau squared) and I2 (I-squared) statistical metrics. These statistics were used to gauge and measure the degree of heterogeneity within the gathered data, thereby enabling a more rigorous interpretation of the findings (29). To optimally mitigate potential confounders and to more accurately mirror real-world scenarios, we opted for a random-effects model (30). Notably, when heterogeneity is notably low, outcomes from the random-effects model align with those of the fixed-effects model (30). Consequently, this study uniformly applied a random-effects model for calculating and amalgamating log-odds ratios (log(OR)) and their corresponding 95% confidence intervals (CI) in binary data, as well as in determining Hedges’s g (g) and their relevant 95% CI in continuous outcomes. This methodology bolsters the accuracy and dependability of our analyses.
In this study, we employed log(OR) and g as statistical measures to assess the effect size differences between the experimental group and the control group. Essentially, log(OR) measures the same relationship as the odds ratio(OR), but it provides a more stable and normally distributed estimate, making it more suitable for studies with small sample sizes. On the other hand, g is a standardized measure of mean difference that accounts for the impact of sample size, allowing it to be compared with results from other studies. Compared to the simple standardized mean difference, g adjusts for estimation bias in studies with small samples, thereby offering a more accurate measure of effect size.
To assess and scrutinize publication bias for each assessed outcome, we utilized funnel plots and conducted Egger’s test as part of our analysis (29).
The investigators commenced their study with an initial database search, encompassing PubMed (n = 170), EMBASE (n = 217), Cochrane Library (n = 147), Web of Science (n = 149), and Scopus (n = 75), culminating in a total of 758 articles obtained. Subsequently, we removed 258 redundant articles. A pair of researchers screened out 436 papers based on their titles and abstracts. Subsequently, 64 articles underwent full-text assessment by the same duo, resulting in a final selection of 9 articles. For a comprehensive selection process, please consult Figure 1.
In this study, a total of eight studies conducted thoracoscopic lung lobe resection under general anesthesia combined with TPVB (31–38), while another study performed thoracoscopic surgery under general anesthesia combined with TPVB (39). In seven of the studies, the postoperative analgesia regimen employed Patient-Controlled Intravenous Analgesia (PCIA). In the remaining two studies, the pain management approach involved either continuous thoracic epidural analgesia or PCIA. Detailed information on each study can be found in Table 1.
A single study was categorized as posing a low risk of bias (35), whereas seven studies elicited some level of concern regarding bias risk (31–33, 36–39), and another study was flagged for high bias risk (34), as depicted in Figure 2. The quality assessment of the evidence is outlined in Figure 3.
Figure 2. Risk of bias summary: the author’s assessment of the bias risk factors in various studies.
Six RCTs studies (32–36, 39) were included in our postoperative three-day delirium analysis. We incorporated the delirium incidence rate (1–3 days) from each study that was closest to the third day. The TPVB group exhibited a lower delirium incidence rate three days post-surgery compared to the control cohort (log(OR): −0.62, 95% CI [−1.05, −0.18], p = 0.01, I2 = 0.00%) (Figure 4). No substantial heterogeneity was identified via Galbraith Plot evaluation (Figure 5). We conducted a publication bias funnel plot (Figure 6) and Egger’s test (p = 0.767; Supplementary Appendix 2), both of which did not indicate significant evidence of publication bias.
Five RCTs studies (31, 32, 34, 37, 38) were included in our postoperative seven-day delirium analysis. We included the delirium incidence rate closest to the seventh postoperative day. The delirium incidence rate at seven days postoperatively was lower in the TPVB group compared to the control group (log(OR): –0.94, 95% CI [−1.39, −0.49], p < 0.001, I2 = 0.00%) (Figure 7). Heterogeneity assessment using the Galbraith Plot indicated low heterogeneity (Figure 8). We conducted a publication bias funnel plot (Figure 9) and Egger’s test (p = 0.247; Supplementary Appendix 3), both of which did not reveal significant evidence of publication bias.
An analysis of post-surgical first-day pain scores was conducted, omitting a study with a divergent pain assessment approach (39). Six RCTs were integrated into our evaluation (31–36). When performing a meta-analysis on the remaining seven studies that employed VAS pain scores, we observed that on the first day postoperatively, the TPVB group had lower pain scores compared to the control group (g: −1.52, 95% CI [−2.87, −0.17], p = 0.03, I2 = 98.50%) (Supplementary Appendix 4). The documented I2 value signifies a substantial level of heterogeneity. Heterogeneity analysis was conducted using the Galbraith plot (Figure 10), and the influence of individual studies on the results can be observed in Figure 11.
Figure 10. A Galbraith plot related to postoperative pain within a one-day timeframe is presented in the figure.
We performed a sensitivity analysis of the pain scores on the first day after surgery. In this process, we excluded the study published by Wei et al. (32) to enhance the reliability and real-world relevance of our study findings. On the first day postoperatively, the VAS pain scores in the TPVB group were significantly lower than those in the control group, and this result was statistically significant (g: −0.87, 95% CI [−1.25, −0.48], p < 0.001, I2 = 77.51%) (Figure 12). This suggests that the application of TPVB effectively reduces postoperative pain in patients, leading to improved comfort, enhanced surgical experience, and a smoother recovery process. Nonetheless, it is important to note that there is a relatively high level of heterogeneity among the included studies. Consequently, we should interpret these results with caution. We conducted a publication bias funnel plot and Egger’s test (p = 0.662), both of which did not reveal significant evidence of publication bias.
Our systematic review and meta-analysis clearly demonstrate that TPVB can effectively reduce the incidence of delirium in patients after VATS. According to the GRADE methodology, the quality of evidence for this conclusion is rated as high. Additionally, patients receiving TPVB also exhibit reduced postoperative pain scores. The importance of this study lies in indicating the effectiveness of TPVB in reducing the rates of delirium and pain intensity in patients undergoing VATS.
Current perioperative neurocognitive disorders (PND) include delirium (occurring within one week post-surgery or until discharge, whichever happens first), delayed neurocognitive recovery (a decline in cognitive abilities occurring within 30 days post-surgery), and postoperative neurocognitive disorder (lasting up to 12 months) (5). In our investigation, we discerned that TPVB markedly attenuates the incidence of postoperative delirium on the third and seventh days postoperatively, with low heterogeneity. This result suggests that the implementation of TPVB has a short-term impact on the occurrence of PND. However, research on the long-term effects of TPVB on neurocognitive function after the 7th day following VATS is relatively scarce. Therefore, determining the long-term impact of TPVB on neurocognitive function presents a challenge. Further investigation into the effects of TPVB on postoperative neurocognitive function is necessary. These studies should involve larger sample sizes in clinical trials, the use of standardized diagnostic criteria, long-term tracking of neurocognitive function presents after surgery, and comparisons with patients who have not undergone TPVB. Conducting such studies will be instrumental in gaining a more comprehensive understanding of the effects of TPVB on delirium and neurocognitive function. Ultimately, these investigations will furnish more precise recommendations for clinical practice. Through additional research, we can determine whether TPVB can be considered an effective strategy for reducing the occurrence of delirium and improving patients’ neurocognitive function. Furthermore, we can investigate other potential influencing factors to gain a deeper understanding of the mechanisms behind PND and explore preventive measures.
A recent meta-analysis examining the influence of perioperative peripheral nerve blocks on delirium in elderly individuals undergoing hip joint surgery, encompassing 19 randomized controlled trials with a total of 1,977 patients, indicated a decrease in the occurrence of delirium on the third postoperative day (OR: 0.59, 95% CI [0.40–0.87], p = 0.007, I2 = 35%). (40). However, it’s worth noting that another meta-analysis yielded contradictory results, indicating no statistically significant difference in the incidence of postoperative delirium between regional anesthesia and general anesthesia (41). But, this study is subject to considerable heterogeneity influenced by multiple factors. Additionally, there are substantial inconsistencies in both the statistical outcomes and the definitions used. Consequently, drawing a definitive conclusion from this study proves to be challenging.
In our study, we found that TPVB can significantly reduce postoperative pain scores. Postoperative pain is considered one of the significant risk factors for delirium, and this may be associated with the observed decrease in delirium rates on the third postoperative day. At the same time, current research suggests that postoperative opioid use is also one of the risk factors for the occurrence of delirium (42). Considering that TPVB efficiently diminishes postoperative pain levels, it may contribute to a lower delirium rates by minimizing the need for opioid analgesics (43, 44). Nonetheless, it’s worth mentioning that in this study, at the seven-day postoperative time point, patients in the TPVB group also demonstrated a significant decrease in delirium rates. This could be attributed to the ability of TPVB to mitigate perioperative and postoperative stress responses and inflammatory reactions induced by surgery, consequently reducing the incidence of delirium.
In our meta-analysis of pain scores, it is essential to take note that Wei et al. (39) utilized the FLACC scale for pain evaluation, while other studies employed the Visual Analog Scale (VAS) for pain assessment. As a result, we decided to exclude the study conducted by Wei et al. (39) from our analysis. Nevertheless, it is noteworthy that the study conducted by Wei et al. (39) also suggested a tendency towards lower pain scores (OR: 0.55, 95% CI [−0.04, 1.14], p = 0.065). Simultaneously, the heterogeneity analysis of pain scores on postoperative day one, as depicted in the Galbraith plot (Figure 10), and the influence of individual studies on the outcomes (Figure 11), clearly demonstrates the substantial heterogeneity evident in the study by Wei et al. published in 2022 (32). This heterogeneity has had a notable impact on the experimental results, potentially introducing inherent bias into the study findings. Consequently, during sensitivity analysis, the aforementioned study was excluded.
Our investigation does face certain limitations. Primarily, due to variations in the timing of delirium diagnosis across the included studies, we extracted delirium incidence rates closest to the third and seventh post-surgical days, incorporating them into the analysis. This approach may inevitably introduce some potential bias. Secondly, multiple diagnostic tools are currently at our disposal for diagnosing delirium, including the Confusion Assessment Method (CAM), the Richmond Agitation-Sedation Scale (RASS), the Memorial Delirium Assessment Scale (MDAS), the Nursing Delirium Screening Scale (Nu-DESC), the Mini-Mental State Examination (MMSE), and the Montreal Cognitive Assessment (MOCA), among others (45, 46). Nonetheless, there’s currently no uniform standard for diagnosing delirium. The research we incorporated utilized different methods for delirium diagnosis, including Nu-DESC, 3D-CAM, MoCA, MMSE, and one study did not explicitly specify its diagnostic approach. These variations in diagnostic methods may potentially influence the study results. Moreover, concerning the implementation of TPVB, most research favored ropivacaine as the primary agent, with a few opted for a combination of lidocaine. Notably, inconsistencies in dosage, volume, concentration, and injection site among these studies. These disparities could potentially affect the effectiveness of TPVB and create variances in the study outcomes.
In summary, this study indicates that TPVB has a positive short-term impact on reducing the incidence of delirium among patients undergoing VATS, with high-quality GRADE evidence supporting this conclusion. However, contradictions remain, and further research is necessary to fully understand TPVB’s effects on neurocognitive function and to refine postoperative management strategies. Such studies are essential for enhancing recovery support for surgical patients and ensuring a more comprehensive approach to their care.
The original contributions presented in the study are included in the article/Supplementary material, further inquiries can be directed to the corresponding author.
XZ: Writing – review & editing, Writing – original draft. WM: Writing – review & editing, Writing – original draft. LZ: Writing – review & editing, Writing – original draft. HZ: Writing – review & editing, Writing – original draft. LC: Writing – review & editing, Writing – original draft. YX: Writing – review & editing, Writing – original draft. LL: Writing – review & editing, Writing – original draft.
The author(s) declare that no financial support was received for the research, authorship, and/or publication of this article.
The authors would like to thank all the authors who participated in this study for their contributions to this study.
The authors declare that the research was conducted in the absence of any commercial or financial relationships that could be construed as a potential conflict of interest.
All claims expressed in this article are solely those of the authors and do not necessarily represent those of their affiliated organizations, or those of the publisher, the editors and the reviewers. Any product that may be evaluated in this article, or claim that may be made by its manufacturer, is not guaranteed or endorsed by the publisher.
The Supplementary material for this article can be found online at: https://www.frontiersin.org/articles/10.3389/fneur.2024.1347991/full#supplementary-material
1. Siegel, RL, Miller, KD, Fuchs, HE, and Jemal, A. Cancer statistics, 2021. CA Cancer J Clin. (2021) 71:7–33. doi: 10.3322/caac.21654
2. Ettinger, DS, Wood, DE, Aisner, DL, Akerley, W, Bauman, JR, Bharat, A, et al. Non-small cell lung Cancer, version 3.2022, NCCN clinical practice guidelines in oncology. J Natl Compr Cancer Netw. (2022) 20:497–30. doi: 10.6004/jnccn.2022.0025
3. Sihoe, ADL. Video-assisted thoracoscopic surgery as the gold standard for lung cancer surgery. Respirology. (2020) 25:49–60. doi: 10.1111/resp.13920
4. Revenig, LM, Canter, DJ, Kim, S, Liu, Y, Sweeney, JF, Sarmiento, JM, et al. Report of a simplified frailty score predictive of short-term postoperative morbidity and mortality. J Am Coll Surg. (2015) 220:904–911e1. doi: 10.1016/j.jamcollsurg.2015.01.053
5. Evered, L, Silbert, B, Knopman, DS, Scott, DA, DeKosky, ST, Rasmussen, LS, et al. Recommendations for the nomenclature of cognitive change associated with anaesthesia and Surgery-2018. Anesthesiology. (2018) 129:872–9. doi: 10.1097/aln.0000000000002334
6. Silverstein Jeffrey, H, Timberger, M, Reich David, L, Uysal, S, and Warltier, DC. Central nervous system dysfunction after noncardiac surgery and Anesthesia in the elderly. Anesthesiology. (2007) 106:622–8. doi: 10.1097/00000542-200703000-00026
7. Zhang, Y, He, ST, Nie, B, Li, XY, and Wang, DX. Emergence delirium is associated with increased postoperative delirium in elderly: a prospective observational study. J Anesth. (2020) 34:675–87. doi: 10.1007/s00540-020-02805-8
8. Lipowski, ZJ. Delirium in the elderly patient. N Engl J Med. (1989) 320:578–82. doi: 10.1056/nejm198903023200907
9. Liptzin, B, and Levkoff, SE. An empirical study of delirium subtypes. Br J Psychiatry. (1992) 161:843–5. doi: 10.1192/bjp.161.6.843
10. Fiest, KM, Soo, A, Hee Lee, C, Niven, DJ, Ely, EW, Doig, CJ, et al. Long-term outcomes in ICU patients with delirium: a population-based cohort study. Am J Respir Crit Care Med. (2021) 204:412–20. doi: 10.1164/rccm.202002-0320OC
11. Hshieh, TT, Yang, T, Gartaganis, SL, Yue, J, and Inouye, SK. Hospital elder life program: systematic review and Meta-analysis of effectiveness. Am J Geriatr Psychiatry. (2018) 26:1015–33. doi: 10.1016/j.jagp.2018.06.007
12. Swarbrick, CJ, and Partridge, JSL. Evidence-based strategies to reduce the incidence of postoperative delirium: a narrative review. Anaesthesia. (2022) 77 Suppl 1:92–01. doi: 10.1111/anae.15607
13. Khachaturian, AS, Hayden, KM, Devlin, JW, Fleisher, LA, Lock, SL, Cunningham, C, et al. International drive to illuminate delirium: a developing public health blueprint for action. Alzheimers Dement. (2020) 16:711–25. doi: 10.1002/alz.12075
14. Davis, DHJ, Skelly, DT, Murray, C, Hennessy, E, Bowen, J, Norton, S, et al. Worsening cognitive impairment and neurodegenerative pathology progressively increase risk for delirium. Am J Geriatr Psychiatry. (2015) 23:403–15. doi: 10.1016/j.jagp.2014.08.005
15. Hayhurst, CJ, Hughes, CG, and Pandharipande, PP. The conundrum of pain, opiate use, and delirium: Analgosedation or analgesia-first approach? Am J Respir Crit Care Med. (2021) 204:502–3. doi: 10.1164/rccm.202104-0968ED
16. Morrison, RS, Dickman, E, Hwang, U, Akhtar, S, Ferguson, T, Huang, J, et al. Regional nerve blocks improve pain and functional outcomes in hip fracture: a randomized controlled trial. J Am Geriatr Soc. (2016) 64:2433–9. doi: 10.1111/jgs.14386
17. Abou-Setta, AM, Beaupre, LA, Rashiq, S, Dryden, DM, Hamm, MP, Sadowski, CA, et al. Comparative effectiveness of pain management interventions for hip fracture: a systematic review. Ann Intern Med. (2011) 155:234–45. doi: 10.7326/0003-4819-155-4-201108160-00346
18. Xu, YJ, Sun, X, Jiang, H, Yin, YH, Weng, ML, Sun, ZR, et al. Randomized clinical trial of continuous transversus abdominis plane block, epidural or patient-controlled analgesia for patients undergoing laparoscopic colorectal cancer surgery. Br J Surg. (2020) 107:e133–41. doi: 10.1002/bjs.11403
19. Liu, R, Qin, H, Wang, M, Li, K, and Zhao, G. Transversus abdominis plane block with general anesthesia blunts the perioperative stress response in patients undergoing radical gastrectomy. BMC Anesthesiol. (2019) 19:205. doi: 10.1186/s12871-019-0861-0
20. Zhang, W, Cong, X, Zhang, L, Sun, M, Li, B, Geng, H, et al. Effects of thoracic nerve block on perioperative lung injury, immune function, and recovery after thoracic surgery. Clin Transl Med. (2020) 10:e38. doi: 10.1002/ctm2.38
21. Bagry, H, de la Cuadra Fontaine, JC, Asenjo, JF, Bracco, D, and Carli, F. Effect of a continuous peripheral nerve block on the inflammatory response in knee arthroplasty. Reg Anesth Pain Med. (2008) 33:17–23. doi: 10.1016/j.rapm.2007.06.398
22. Memtsoudis, SG, Cozowicz, C, Bekeris, J, Bekere, D, Liu, J, Soffin, EM, et al. Peripheral nerve block anesthesia/analgesia for patients undergoing primary hip and knee arthroplasty: recommendations from the international consensus on Anesthesia-related outcomes after surgery (ICAROS) group based on a systematic review and meta-analysis of current literature. Reg Anesth Pain Med. (2021) 46:971–85. doi: 10.1136/rapm-2021-102750
23. Li, T, Jiang, C, and Smith, FG. Regional vs general Anesthesia and incidence of postoperative delirium in older patients undergoing hip fracture surgery-reply. JAMA. (2022) 327:1708–9. doi: 10.1001/jama.2022.3544
24. Page, MJ, McKenzie, JE, Bossuyt, PM, Boutron, I, Hoffmann, TC, Mulrow, CD, et al. The PRISMA 2020 statement: an updated guideline for reporting systematic reviews. BMJ. (2021) 372:n71. doi: 10.1136/bmj.n71
25. Shea, BJ, Reeves, BC, Wells, G, Thuku, M, Hamel, C, Moran, J, et al. AMSTAR 2: a critical appraisal tool for systematic reviews that include randomised or non-randomised studies of healthcare interventions, or both. BMJ. (2017) 358:j4008. doi: 10.1136/bmj.j4008
26. Wan, X, Wang, W, Liu, J, and Tong, T. Estimating the sample mean and standard deviation from the sample size, median, range and/or interquartile range. BMC Med Res Methodol. (2014) 14:135. doi: 10.1186/1471-2288-14-135
27. Sterne, JAC, Savović, J, Page, MJ, Elbers, RG, Blencowe, NS, Boutron, I, et al. RoB 2: a revised tool for assessing risk of bias in randomised trials. BMJ. (2019) 28:l4898. doi: 10.1136/bmj.l4898
28. Schünemann, HJ, Oxman, AD, Brozek, J, Glasziou, P, Jaeschke, R, Vist, GE, et al. Grading quality of evidence and strength of recommendations for diagnostic tests and strategies. BMJ. (2008) 336:1106–10. doi: 10.1136/bmj.39500.677199.AE
29. Cumpston, M, Li, T, Page, MJ, Chandler, J, Welch, VA, Higgins, JP, et al. Updated guidance for trusted systematic reviews: a new edition of the Cochrane handbook for systematic reviews of interventions. Cochrane Database Syst Rev. (2019) 10:Ed000142. doi: 10.1002/14651858.Ed000142
30. Borenstein, M, Hedges, LV, Higgins, JP, and Rothstein, HR. A basic introduction to fixed-effect and random-effects models for meta-analysis. Res Synth Methods. (2010) 1:97–11. doi: 10.1002/jrsm.12
31. Dongjie, Q, Longbiao, Z, Peng, L, Li, J, Hongmeng, X, Zhiyan, C, et al. Effects of thoracic paravertebral nerve block on postoperative pain and postoperative delirium in elderly patients undergoing thoracoscopic lobectomy [journal article]. Medicine. (2023) 102:e32907. doi: 10.1097/MD.0000000000032907
32. Wei, W, Zheng, X, Gu, Y, Fu, W, Tang, C, and Yao, Y. Effect of general anesthesia with thoracic paravertebral block on postoperative delirium in elderly patients undergoing thoracoscopic lobectomy: a randomized-controlled trial [journal article]. BMC Anesthesiol. (2022) 22:1. doi: 10.1186/s12871-021-01532-1
33. Zhang, JW, Feng, XY, Yang, J, Wang, ZH, Wang, Z, and Bai, LP. Ultrasound-guided single thoracic paravertebral nerve block and erector spinae plane block for perioperative analgesia in thoracoscopic pulmonary lobectomy: a randomized controlled trial [article]. Insights Imaging. (2022) 13:16. doi: 10.1186/s13244-021-01151-x
34. Heng, L, Wang, M, Wang, M, Li, L, and Zhu, S. Thoracic paravertebral block ameliorates postoperative delirium in geriatric patients [journal article]. Thorac Cardiovasc Surg. (2022) 70:439–44. doi: 10.1055/s-0041-1731788
35. Chen, XD, Liu, QS, and Fan, L. Effects of thoracic paravertebral block combined with s-ketamine on postoperative pain and cognitive function after thoracoscopic surgery [article]. Heliyon. (2022) 8:e12231. doi: 10.1016/j.heliyon.2022.e12231
36. Liu, W, Luo, T, Wang, F, Zhang, D, Liu, T, Huang, J, et al. Effect of preoperative thoracic paravertebral blocks on emergence agitation during tracheal extubation: a randomized controlled trial [article]. Front Med. (2022) 9. doi: 10.3389/fmed.2022.902908
37. Zhao, W, Li, C, Wang, Z, Shen, J, and Jia, H. Effect of paravertebral nerve block combined with general anesthesia on intraoperative regional cerebral oxygen saturation in elderly patients undergoing thoracoscopic lobectomy [article]. Chin J Anesthesiol. (2021) 41:939–42. doi: 10.3760/cma.j.cn131073.20210326.00810
38. Xie, H, Zhou, J, Du, W, Zhang, S, Huang, R, Han, Q, et al. Impact of thoracic paravertebral block combined with general anesthesia on postoperative cognitive function and serum adiponectin levels in elderly patients undergoing lobectomy [journal article]. Wideochirurgia I inne techniki maloinwazyjne. (2019) 14:538–44. doi: 10.5114/wiitm.2019.84742
39. Wei, W, Fan, Y, Liu, W, Zhao, T, Tian, H, Xu, Y, et al. Combined non-intubated anaesthesia and paravertebral nerve block in comparison with intubated anaesthesia in children undergoing video-assisted thoracic surgery [journal article]. Acta Anaesthesiol Scand. (2020) 64:810–8. doi: 10.1111/aas.13572
40. Kim, SY, Jo, HY, Na, HS, Han, SH, Do, SH, and Shin, HJ. The effect of peripheral nerve block on postoperative delirium in older adults undergoing hip surgery: a systematic review and Meta-analysis of randomized controlled trials. J Clin Med. (2023) 12. doi: 10.3390/jcm12072459
41. Bhushan, S, Huang, X, Duan, Y, and Xiao, Z. The impact of regional versus general anesthesia on postoperative neurocognitive outcomes in elderly patients undergoing hip fracture surgery: a systematic review and meta-analysis. Int J Surg. (2022) 105:106854. doi: 10.1016/j.ijsu.2022.106854
42. Duprey, MS, Dijkstra-Kersten, SMA, Zaal, IJ, Briesacher, BA, Saczynski, JS, Griffith, JL, et al. Opioid use increases the risk of delirium in critically ill adults independently of pain. Am J Respir Crit Care Med. (2021) 204:566–72. doi: 10.1164/rccm.202010-3794OC
43. Clegg, A, and Young, JB. Which medications to avoid in people at risk of delirium: a systematic review. Age Ageing. (2011) 40:23–9. doi: 10.1093/ageing/afq140
44. O'Connell, KM, Quistberg, DA, Tessler, R, Robinson, BRH, Cuschieri, J, Maier, RV, et al. Decreased risk of delirium with use of regional analgesia in geriatric trauma patients with multiple rib fractures. Ann Surg. (2018) 268:534–40. doi: 10.1097/sla.0000000000002929
45. Wong, CL, Holroyd-Leduc, J, Simel, DL, and Straus, SE. Does this patient have delirium?: value of bedside instruments. JAMA. (2010) 304:779–86. doi: 10.1001/jama.2010.1182
Keywords: delirium, thoracic paravertebral nerve block, thoracic surgery thoracoscopy, thoracoscope, postoperative analgesia, meta-analysis
Citation: Zhou X, Mao W, Zhao L, Zhu H, Chen L, Xie Y and Li L (2024) Effect of thoracic paravertebral nerve block on delirium in patients after video-assisted thoracoscopic surgery: a systematic review and meta-analysis of randomized controlled trials. Front. Neurol. 15:1347991. doi: 10.3389/fneur.2024.1347991
Received: 15 January 2024; Accepted: 28 March 2024;
Published: 10 April 2024.
Edited by:
Mirella Russo, University of Studies G. d'Annunzio Chieti and Pescara, ItalyReviewed by:
Chang Xu, Sichuan University, ChinaCopyright © 2024 Zhou, Mao, Zhao, Zhu, Chen, Xie and Li. This is an open-access article distributed under the terms of the Creative Commons Attribution License (CC BY). The use, distribution or reproduction in other forums is permitted, provided the original author(s) and the copyright owner(s) are credited and that the original publication in this journal is cited, in accordance with accepted academic practice. No use, distribution or reproduction is permitted which does not comply with these terms.
*Correspondence: Linji Li, bGxqLXN0ZXBoZW5AMTYzLmNvbQ==
†These authors have contributed equally to this work
Disclaimer: All claims expressed in this article are solely those of the authors and do not necessarily represent those of their affiliated organizations, or those of the publisher, the editors and the reviewers. Any product that may be evaluated in this article or claim that may be made by its manufacturer is not guaranteed or endorsed by the publisher.
Research integrity at Frontiers
Learn more about the work of our research integrity team to safeguard the quality of each article we publish.