- 1Department of Paediatrics and Adolescent Health, Akershus University Hospital, Lørenskog, Norway
- 2Institute of Clinical Medicine, University of Oslo, Oslo, Norway
- 3Department of Clinical Molecular Biology (EpiGen), University of Oslo and Akershus University Hospital, Lørenskog, Norway
- 4Institute of Neuroscience and Physiology, Department of Psychiatry and Neurochemistry, Sahlgrenska Academy, University of Gothenburg, Mölndal, Sweden
- 5Clinical Neurochemistry Laboratory, Sahlgrenska University Hospital, Mölndal, Sweden
- 6UCL Institute of Neurology, Department of Neurodegenerative Disease, Queen Square, London, United Kingdom
- 7UK Dementia Research Institute, London, United Kingdom
- 8Hong Kong Center for Neurodegenerative Diseases, Kowloon, Hong Kong SAR, China
- 9Department of Neurology, Akershus University Hospital, Lørenskog, Norway
Introduction: The post-COVID-19 condition (PCC) is characterized by debilitating persistent symptoms, including symptoms suggesting neurological aberrations such as concentration difficulties, impaired memory, pain, and sleep disturbances. The underlying mechanisms remain elusive. This study aimed to investigate brain injury biomarkers, neurocognitive test performance, and self-reported neurological and neuropsychological symptoms in young people with PCC.
Methods: A total of 404 non-hospitalized adolescents and young adults aged 12–25 years who tested positive for SARS-CoV-2, along with 105 matched SARS-CoV-2 negative individuals, were prospectively enrolled and followed-up for 6 months (Clinical Trials ID: NCT04686734). All participants underwent comprehensive assessment encompassing clinical examinations, questionnaires, neurocognitive testing and blood sampling. Serum samples were immunoassayed for the brain injury biomarkers neurofilament light chain (Nfl) and glial fibrillary acidic protein (GFAp). At 6 months, cross-sectional analyses of serum Nfl/GFAp, neurocognitive test results and symptom scores were performed across groups based on adherence to PCC criteria as well as initial SARS-CoV-2 test results. Also, associations between Nfl/GFAp, neurocognitive test results, and symptom scores were explored.
Results: A total of 381 SARS-CoV-2 positive and 85 SARS-CoV-2 negative were included in the final analysis at 6 months, of whom 48% and 47%, respectively, adhered to the PCC criteria. Serum levels of Nfl and GFAp were almost equal across groups and did not differ from reference values in healthy populations. Also, neurocognitive test results were not different across groups, whereas symptom scores were significantly higher in patients fulfilling PCC criteria (independent of initial SARS-CoV-2 status). No significant associations between Nfl/GFAp, neurocognitive test results, and symptom scores were found.
Conclusion: Normal brain injury biomarkers and neurocognitive performance 6 months after mild COVID-19 implies that the persistent symptoms associated with PCC are not concurrent with ongoing central nervous system damage or permanent disruption of cognitive functions. This finding contradicts the notion of neuroinflammation as a likely explanation for the persistent symptoms.
Introduction
The majority of individuals infected with Severe Acute Respiratory Syndrome Coronavirus 2 (SARS-CoV-2), the virus that causes Corona Virus Disease of 2019 (COVID-19), typically recover to their baseline health status within a few weeks after the acute infection. However, a substantial portion of individuals experience persistent post-infective symptoms (1–3). Persisting symptoms have been reported in patients regardless of the severity of the acute COVID-19 infection (4), and even children and young adults who experienced predominantly mild cases of acute COVID-19 may endure prolonged symptoms (5). These enduring health issues commonly include various neurological complaints such as fatigue, post-exertional malaise, headache, memory difficulties, and sleep disturbances (6–8). The World Health organization (WHO) has defined these long-lasting symptoms following confirmed or suspected COVID-19 infection, with no alternative diagnosis to explain them, as Post-COVID-19 condition (PCC) (9). PCC exhibits significant clinical overlap with post-infective fatigue syndrome (PIFS) (10), and numerous queries concerning the underlying mechanisms of disease and its natural progression still lack definite answers. Further, it is still to be established whether the subjective experience of neurological and neuropsychological symptoms in PCC correspond with objectively measurable deficits (8, 11–13).
Multiple mechanisms have been suggested as potential underlying mechanisms of neurobiological aberrations in COVID-19 and PCC. In the acute and subacute stages, CNS involvement may be due to immune activation triggered by systemic inflammation, microvascular damage, thromboembolic events, or non-specific hypoxic effects resulting from severe illness (14, 15). One mechanism proposed to account for the manifestations of PCC revolves around activation of the neuroimmune system (16, 17). Alternatively, the symptoms of PCC may be explained from functional CNS alterations (18), analogous to common mechanisms of chronic pain conditions (19). This latter explanation acknowledges that symptoms may arise independently of neuronal damage and/or interoceptive afferent signals, and resonates with previous published evidence of psychosocial factors as important predictors of persistent symptoms (20, 21).
The intra-axonal protein neurofilament light chain (Nfl) is a validated biomarker for neuroaxonal injury and neuroinflammation regardless of cause (22–24). Numerous studies have demonstrated a strong correlation between levels of Nfl in cerebrospinal fluid (CSF) and blood serum samples (25, 26) rendering it widely applicable as a biomarker for neuroinflammation and neurodegeneration. Glial fibrillary acidic protein (GFAp) is an astrocytic cytoskeletal protein upregulated in activated astrocytes, recognized for its swift elevation in both CSF and serum in response to acute brain injuries. Studies have evidenced a robust correlation between the levels of GFAp detected in CSF and blood serum samples (27–30). Elevated serum levels of these biomarkers in the acute phase of COVID-19 infection provides evidence of astrocytic and neuroaxonal damage in patients undergoing a severe course of SARS-CoV-2 infection (13, 31–34). We have previously reported these biomarkers to be slightly elevated in the subacute phase of SARS-CoV-2 infection in adolescents and young adults with mildly symptomatic disease (11). Studies examining neuroinflammatory biomarkers during follow-up after COVID-19 infection yield varied outcomes, even when they are limited to mild initial cases (35, 36).
In the current study we report serum levels of NfL and GFAp at 6-This study aimed to investigate brain injury biomarkers, neurocognitive test performance, and self-reported neurological and neuropsychological symptoms in young people with PCC. We examined cross-sectional data from 6-month follow-up of a large prospective cohort of adolescents and young adults with and without COVID-19.
Methods
Study design
The long-term effects of COVID-19 in Adolescents (LoTECA) project is a longitudinal observational cohort study of non-hospitalized adolescents and young adults. Participants testing positive and negative for SARS-CoV-2 were included, with follow-up at 6 and 12 months (Clinical Trials ID: NCT04686734). Details of the study design have been described previously (20). This study reports results from the 6-month follow-up visit.
Ethical approval for this project was granted by The Regional Committee for Ethics in Medical Research. Written informed consent was obtained from each participant at study inclusion.
Participants
Between 24 December 2020 and 18 May 2021, a consecutive cohort of adolescents and young adults undergoing SARS-CoV-2 testing with reverse transcription-polymerase chain reaction (RT-PCR) were enrolled. All participants were recruited from one of two microbiological laboratories, Fürst Medical Laboratory or Department of Microbiology and Infection Control at Akershus University Hospital, both located in Southeast Norway. The prevailing strain of SARS-CoV-2 in this geographical area during most of the recruitment period was B.1.1.7 (Alpha). Any SARS-CoV-2 positive individuals were considered eligible for enrolment after fulfilling a 10-day quarantine. Concurrently, a SARS-CoV-2 negative control group was recruited among individuals exhibiting a similar distribution of sex and age as the SARS-CoV-2 infected cases. Within the SARS-CoV-2 negative group, some individuals had undergone testing due to acute infectious symptoms, while others were asymptomatic close contacts of confirmed cases.
Exclusion criteria at baseline encompassed the following: (1) A duration of more than 28 days since onset of symptoms; (2) Hospitalization due to COVID-19; (3) Pregnancy; and (4) Serological evidence of SARS-CoV-2 infection (in the SARS-CoV-2-negative group).
Investigational program
At enrolment and each follow-up assessment, all participants attended a comprehensive assessment program at the study center at Akershus University Hospital, Norway. This program encompassed clinical interview, physical examination, blood sample collection, vital sign recording, functional and neurocognitive testing, and completion of questionnaires. The complete investigational program of the LoTECA project has been published previously (20).
Laboratory assays
Blood samples were collected via antecubital venepuncture as the first part of the 6-month follow-up visit. Samples were subjected to analysis for routine clinical markers. To identify previous infection with COVID-19, serum samples were analyzed for SARS-CoV-2 nucleocapsid and receptor binding antibodies.
Serum samples for measurement of GFAp and Nfl was collected in 3.5 mL Vacuette R (Greiner bio-one GmbH) with gel. Samples underwent clotting for a minimum of 30 min. Within 2 h, they were processed by centrifugation at 2,200 g for 10 min. The aliquots were stored immediately at −80°C until analysis. Serum GFAp and Nfl measurement was conducted at the Clinical Neurochemistry Laboratory, Sahlgrenska University Hospital, Sweden, by certified laboratory technicians blinded to clinical data. The analysis was performed utilizing commercially available Single Molecule Array (Simoa) assays on an HD-X analyzer (Human Neuro 2-plex B assay), as instructed by the manufacturer (Quanterix, Billerica, MA). Calibrators were run in duplicates, while the samples were diluted four-fold and run as singlicates. To monitor assay performance, two quality control (QC) samples, with different concentration levels, were run in duplicates at the beginning and end of each analytical run. For the QC sample with a Nfl concentration of 14.1 pg./mL, repeatability and intermediate precision were both 6.2%, and for QC samples with a concentration of 77.3 pg./mL, both repeatability and intermediate precision was 5.9%. For GFAp CQ samples with concentration 99.4 pg./mL, repeatability was 4.4% and intermediate precision was 8.3%. For GFAp QC samples with concentration 281 pg./mL, repeatability was 5.6% and intermediate precision was 6.3%.
Neurocognitive testing
During the 6-month follow-up visit, all study participants underwent neurocognitive assessment with two standardized tests: the Digit-Span Test from the Wechsler Intelligence Scale for Children, 4th edition (WISC) (37), and the Hopkins Verbal Learning Test-Revised (HVLT-R) (38).
The Digit-Span Test is a tool for evaluating verbal and auditory working memory. An examiner presents a series of random digits verbally. The initial digit sequence comprises two random numbers, and with each subsequent sequence, an additional digit is included. During the digit span forward mode, the participant is tasked with repeating the digits in the same order as they were presented, while in the digit span backward mode, the digits are to be repeated in reverse order. A score of one point is assigned for each correctly recalled digit sequence. The test is discontinued when the participant provides incorrect responses for two sequences of equal length. Results are reported in the form of sum scores for digit span forward and backward, as well as a total sum score.
The HVLT-R test is designed to assess verbal learning, delayed recall, and recognition. A standardized procedure is followed, where the examiner orally presents a list of 12 words, and the participant is tasked with repeating as many of these words as possible in three consecutive trials. The cumulative score for verbal learning memory is determined by summing the total number of words remembered across the three trials, with a possible range of scores ranging from 0 to 36. To evaluate delayed verbal memory, the number of words successfully recalled after a 20-min interval is recorded; score ranges from 0 to 12. Subsequently, a list of 24 words is presented, of which 12 words are identical to those from the initial list. The number of correctly recognized words and falsely recognized words are recorded separately; scores range from 0 to 12.
Questionnaires
A questionnaire was employed to gather information regarding comorbidities, family medical history, current medication, smoking habits, substance abuse, physical activity and parental occupation. Parental occupation was used to gauge socioeconomic status.
Sleep problems and pain were recorded through the Karolinska Sleep Inventory and the Brief Pain Inventory, respectively (39, 40). In the Karolinska Sleep Inventory, a total of 12 items addressed frequency of sleep disturbances on 6-point Likert scales, where 1 is “never” and 6 is “all the time”; then, the scoring was reversed, and total sum score was computed across all items ranging from 12 to 72, where lower scores indicate more sleep disturbances. Accordingly, indexes for insomnia, awakening problems, and sleepiness were computed as sum scores across relevant items. In the Brief Pain Inventory, a total of four items addressed different aspects of pain on 10-point Likert scales, where 1 is “no pain” and 10 is “worst pain imaginable”; total sum score was computed across all items ranging from 4 to 40, where higher scores indicate more pain.
In addition, five neurological/neurocognitive symptoms were assessed: Concentration difficulty, difficulty making decisions, memory difficulty, feeling confused or disoriented, and headache. The frequency of these symptoms were assessed using five-point Likert scales, ranging from 1 to 5, with options spanning from “never” to “each day/always.”
SARS-CoV-2 immunization
Data pertaining to vaccination status was acquired through linkage with the Norwegian Immunization Register (41).
Case definitions
The WHO definition of Post COVID-19 Condition (PCC) (9) and the modified Fukuda-case definition of Post-Infective Fatigue Syndrome (PIFS) (42) were applied and operationalized at 6-month follow-up, as thoroughly described previously (20). In brief, all participants were categorized as either case or non-case in accordance with both definitions. To enhance accuracy, a distinction was drawn between definite and uncertain classifications, considering concurrent medical and psychiatric comorbidities that could potentially account for the reported symptoms. Both clinical findings, laboratory reports and questionnaire data from baseline and at 6-month follow-up were considered in the identification of PCC and PIFS cases. Two medical doctors blinded to the participants’ initial SARS-CoV-2 status conducted the assessment independently.
Participants were stratified into four groups based on COVID status and adherence to PCC criteria as follows: (1) COVID-19 positive individuals who adhered to PCC criteria (COVID+PCC+); (2) COVID-19 positive individuals who did not adhere to PCC criteria (COVID+PCC-); (3) COVID-19 negative individuals who adhered to PCC criteria (COVID-PCC+); and (4) COVID-19 negative individuals who did not adhere to PCC criteria (COVID-PCC-). A similar categorization was undertaken based on adherence to PIFS criteria (PIFS+ or PIFS-).
Statistical analysis
For cross-sectional comparisons between COVID-19 positive and COVID-19 negative cases, chi-square test and Wilcoxon rank-sum test were applied as appropriate based on distribution of the data. For comparison across the four groups according to COVID status and PCC/PIFS adherence, one-way ANOVA or Kruskal-Wallis tests were used as appropriate. Post-hoc analyses were conducted to investigate differences between groups that exhibited statistically significant results.
Associations between symptoms, neurocognitive test results, neurological findings and the two brain injury biomarkers Nfl and GFAp were investigated using the non-parametrical Spearman’s rho test.
Statistical analyses were executed using Stata Statistical Software: Release 16 (Statacorp LLC, College Station, TX). A significance threshold of p < 0.05 was adopted (two-sided test). Bonferroni correction was incorporated in the spearman’s rho test to account for test multiplicity.
Results
At baseline 509 (404 SARS-CoV-2 positive, 105 SARS-CoV-2 negative) children and young adults were included in the study. A total of 26 participants were lost to follow-up (22 COVID-19 cases and 4 COVID-19 negative controls). Of the COVID-19 negative controls, 16 were excluded from analyses at 6 months due to SARS-CoV-2 infection during the follow-up period, either self-reported or diagnosed from the appearance of plasma SARS-CoV-2 nucleocapsid antibodies. In addition, one COVID-19 case suffering from multiple sclerosis was excluded from the current analysis of neurological involvement. A total of 466 participants (381 SARS-CoV-2 positive, 85 SARS-CoV-2 negative) were included in the final analysis of the present paper.
The median time from baseline visit to follow-up was 193 days for both the SARS-CoV-2 positive and SARS-CoV-2 negative group. An overview of demographics and background characteristics are reported in Table 1.
As previously reported (20), there was no difference in adherence to the WHO post-COVID-19 condition nor in adherence to the criteria of post infectious fatigue syndrome based on previous COVID-19 exposure.
Comparison according to COVID-19 status and PCC adherence
Results from comparison across the four groups according to COVID status and PCC adherence are presented in Table 2. Significant differences between groups were found for all symptoms of neurocognitive dysfunction, as well as pain and sleep difficulties. Post-hoc test results are reported in Supplementary Table 1. Generally, the post-hoc tests of reported symptoms showed significant differences between groups that differed in PCC adherence, but not between groups with differences in COVID status and similar PCC adherence.
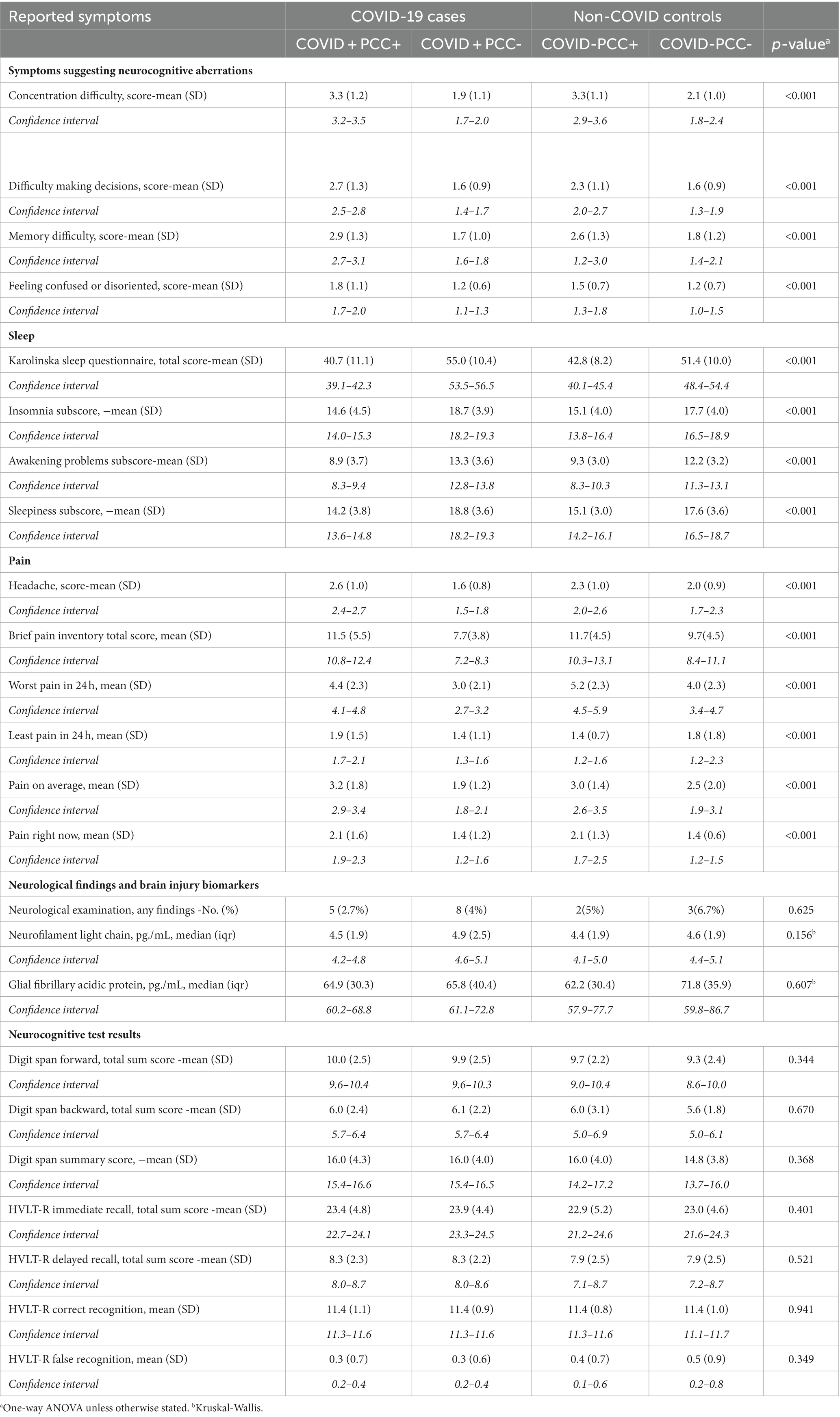
Table 2. Cross-sectional comparison of symptoms, clinical and laboratory findings, and neurocognitive test results among COVID-19 cases and non-COVID controls for participants with and without post-COVID-19 Condition (PCC) at 6 months follow-up.
Brain injury biomarkers, neurocognitive test performance, and clinical neurological findings did not differ across the four groups (Table 2; Figure 1). Similarly, there were no differences across four groups stratified according to COVID-19 status and adherence to PIFS criteria (Figure 2; Supplementary Tables 2, 3).
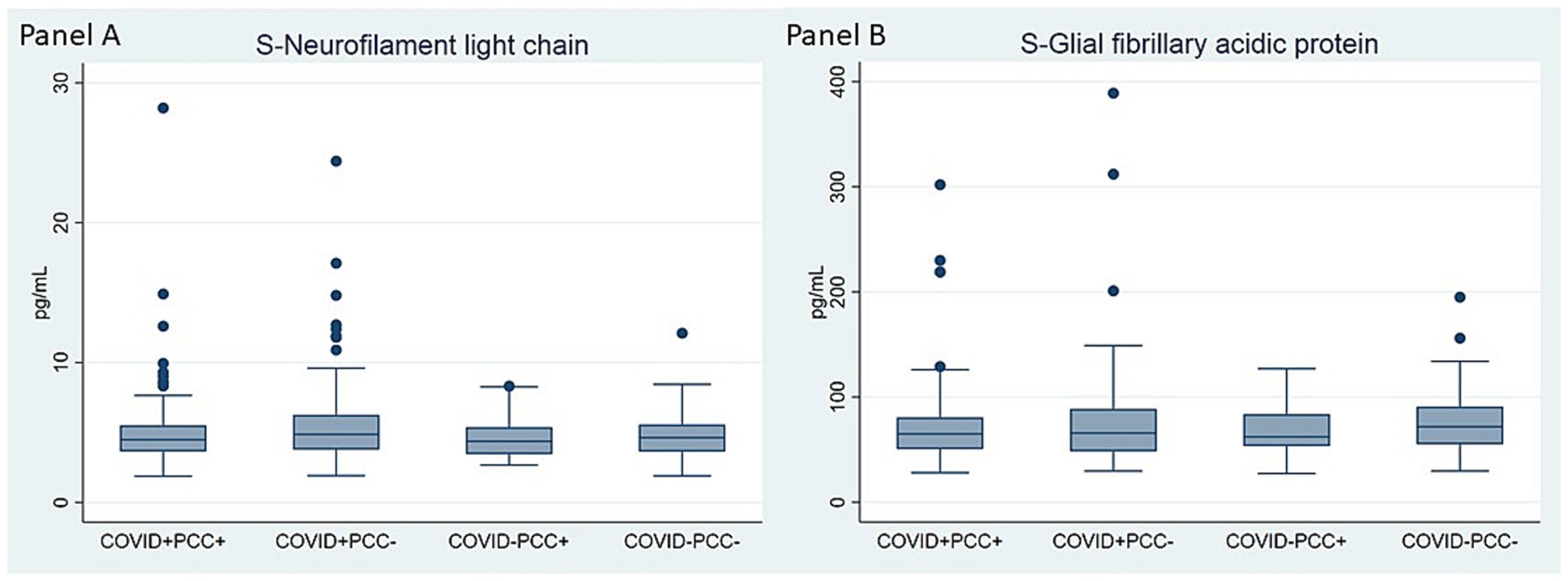
Figure 1. Comparison of serum levels of neurofilament light chain (A) and glial fibrillary acidic protein (B) at 6-months follow-up within groups of COVID-19 status and PCC adherence. Kruskal-Wallis test was conducted to examine the differences between groups. Panel (A): Chi square = 5.22, p = 0.156, df = 3; Panel (B): Chi square = 1.84, p = 0.607, df = 3.
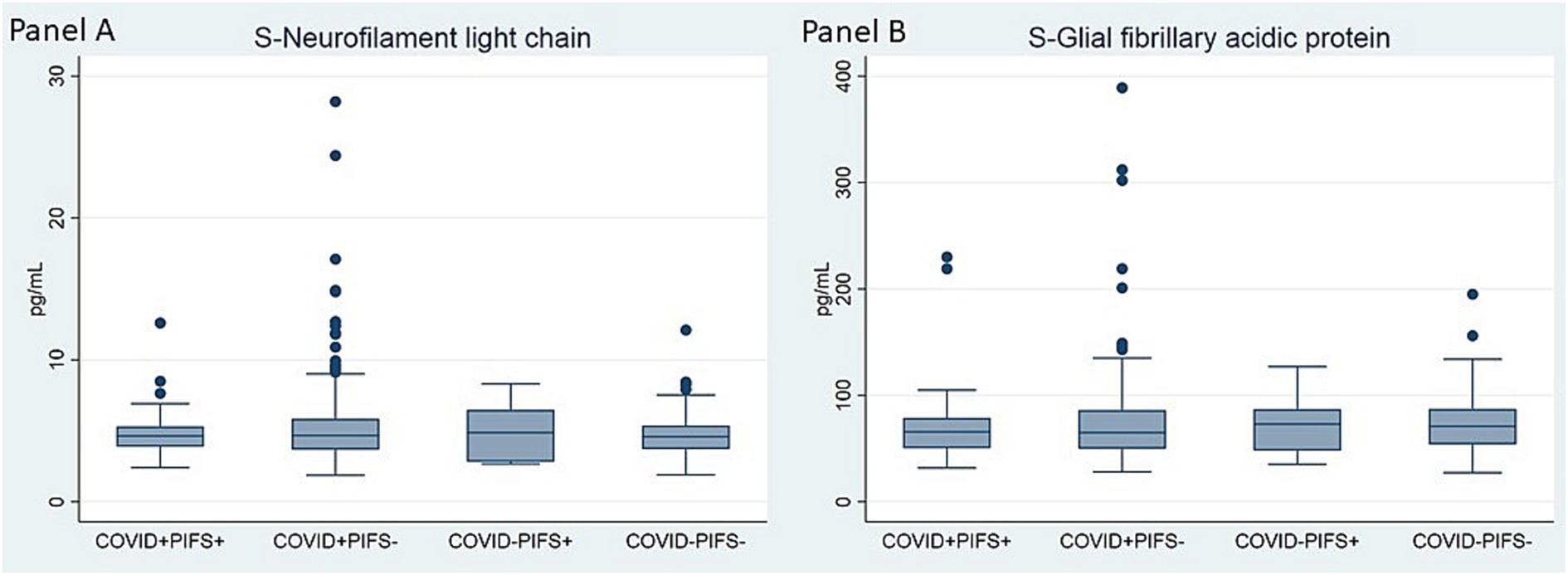
Figure 2. Comparison of serum levels of neurofilament light chain (A) and glial fibrillary acidic protein (B) at 6-months follow-up within groups of COVID-19 status and PIFS adherence. Kruskal-Wallis test was conducted to examine the differences between groups Panel (A): Chi square = 0.89, p = 0.827, df = 3; Panel (B): Chi square = 0.87, p = 0.834, df = 3.
Associations to PCC within the SARS-CoV-2 positive cohort
Associations between PCC, PIFS, as well as subjectively reported symptoms, brain injury biomarkers, and neurocognitive test results are presented in Figure 3. Neither of the biomarkers, Nfl or GFAp, demonstrated any association with PCC nor PIFS, nor were they associated with the reported symptoms or neurocognitive test results. In contrast, both PCC and PIFS exhibited significant association with any subjective symptoms of pain, sleep disturbances, memory issues, difficulty concentrating, decision-making challenges and feeling confused or disorientated. However, none of the subjective symptoms was associated with neurocognitive test results. Complete overview of correlation coefficients and significance levels from the spearman’s rho test are provided in Supplementary Table 4.
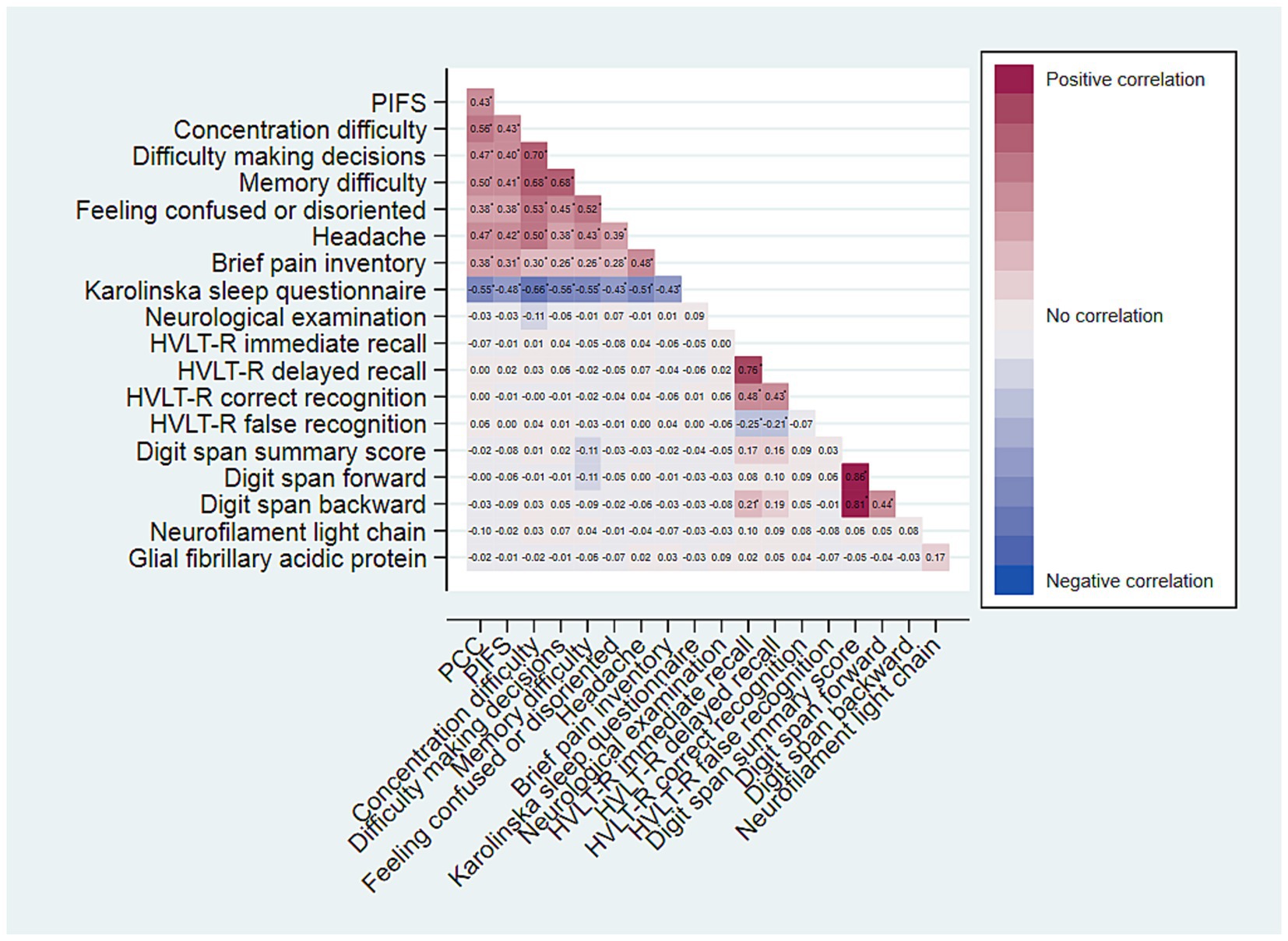
Figure 3. Heatplot of Spearman’s Rho correlation coefficients for variables of neurocognitive symptoms, neurocognitive test results and adherence to PCC and PIFS, respectively. Coefficients marked with *, are significant at a Bonferroni adjusted significance level of α = 0.05/162 = 0.0003.
Discussion
In the present study of a large group of young, non-hospitalized COVID-19 convalescents, the main findings were: (a) That brain injury biomarkers were normalized 6 months after acute infection; (b) That neither brain injury biomarkers, neurocognitive test performance nor clinical neurological finding were associated with PCC or PIFS; and (c) That the burden of subjective neurological and/or neuropsychological symptoms is high in both PCC and PIFS.
In baseline data from our cohort, we observed a slight increase in Nfl and GFAp levels in the sub-acute phase of COVID-19 (11). Our current finding of these brain injury biomarkers returning to normal levels 6-months after mild COVID-19 infection aligns with the findings of others. Kanberg et al. found that Nfl and GFAp serum concentrations were normalized 6 months post-infection in a cohort of mild, moderate and severe COVID-19 cases (43), and Rogatzki et al. found normalization of serum levels of Nfl/GFAp as early as 1 month following mild COVID-19 infection in young adults (35).
Both Nfl and GFAp has previously been suggested as useful biomarkers for identification of patient suffering from neurological sequelae following COVID-19 infection (44). In a study of critically ill COVID-19-patients investigated 3 to 6 months after discharge from the intensive care unit, GFAp and Nfl were found to be associated with neurocognitive dysfunction and neuropsychiatric outcome (45). Contrary, in our cohort of young individuals with mild disease course, there were no association between GFAp/Nfl and neurocognitive symptoms or post-COVID-19 symptomatology. This corroborates with previous reports on milder cases. De Boni et al. reported lower levels of Nfl and GFAp in patients with persistent post-COVID-19 headache compared to patients with severe COVID-19 (46). Lennol et al. evidenced normalization of plasma GFAp and Nfl within 2 months following acute infection in patients with or without symptoms of fatigue, headache and memory loss (47), and Farhadian et al. found no evidence of neuroinflammation or blood–brain barrier dysfunction in a cohort of adults with self-reported PCC (48).
Our results are in contrast to those of Telser et al. (36) who reported higher GFAp levels among participants adhering to PCC criteria compared to those without PCC adherence. However, the study by Telser et al. is limited by the rather small sample size of 146 COVID-19 positive participants and the lack of a COVID-19 negative control group. Additionally, the patients’ COVID-19 status was determined retrospectively based on the presence of antibodies, and differences in time span to the acute infection were not accounted for.
In line with previous reports, the present data confirm a high burden of neurological and/or neuropsychiatric symptoms among patients with PCC and PIFS (49, 50). However, these previous reports do not include measures of neuronal damage nor objective testing of neurocognitive performance. A striking result from the present study is the discrepancy between subjective symptoms and objective findings. This resonates with findings from PIFS in the aftermath of other infections (51, 52).
Various hypotheses have been suggested to explain the pathogenesis of PCC. These include systemic chronic inflammation (53), as well as neuroinflammation and autoimmunity (54). In severe cases of acute COVID-19 there is evidence that the neuroinflammation is linked to cytokine storms, as elevated serum Nfl and GFAp are associated with elevations in pro-inflammatory cytokines (55, 56). Elevated levels of Nfl have even been found to have prognostic value in acute, severe cases of COVID-19 (57). The normalization of brain injury biomarkers 6 months after mild COVID-19 infection found in the current study, suggests that the neurological symptoms associated with PCC do not align with enduring or ongoing CNS injury. This argue against the notion of neuroinflammation as an explanation for the persisting symptoms. In a previous publication (58), we reported a distinct immune signature associated with COVID-19 at 6-month follow-up. However, this did not appear to be connected to PCC symptomatology. In the current study, we found no evidence of ongoing neuroinflammation, and neither brain injury biomarkers nor neurocognitive test results were associated with subjective reported symptomatology. Hence, the findings from the present study adds to a growing body of evidence suggesting that PCC may be associated with functional CNS alterations and have origins more related to a combination of biological, psychological and social factors, rather than being solely biomedical in nature (59).
The small number of COVID-19 negative controls is a limitation to the study. Further, controls were recruited following SARS-CoV-2 testing for either infectious symptoms, or suspected SARS-CoV-2 exposure. Other viral diseases could have caused the symptoms leading to testing. Considering the established role of Epstein–Barr virus (EBV) as a trigger for post-infectious fatigue syndrome (60), individuals with recent EBV infection were not included in the analysis. To further strengthen the quality of the control group, SARS-CoV-2 antibody testing was conducted both at inclusion, and 6 months follow-up. Participant displaying antibodies indicative of prior COVID-19 infection were excluded from the control group.
The external validity of the study is limited by the potential for self-selection bias. It is plausible that our participants exhibited a higher prevalence of symptoms compared to the general population. This selection bias might be even more relevant in the control group. Further, the current study only focused on a cohort of young individuals, mostly infected with the B1.1.7 variant of SARS-CoV-2. Therefore, the generalizability of our findings to other viral strains, and to older age groups, who could exhibit increased vulnerability to both COVID-19 and PCC remains uncertain.
Conclusion
In the current study, we found that brain injury biomarkers were normalized 6 months after acute COVID-19 and that the post-COVID-19 condition, despite high symptom burden, was not associated with brain injury biomarkers, neurocognitive test performance or clinical neurological symptoms. Hence, among adolescents and young adults, neurological symptoms linked to post-COVID-19 condition do not align with continuous CNS damage, thereby challenging the notion of neuroinflammation as an underlying cause of the enduring symptoms.
Data availability statement
The raw data supporting the conclusions of this article will be made available by the authors, without undue reservation.
Ethics statement
The studies involving humans were approved by the Regional Committee for Ethics in Medical Research. The studies were conducted in accordance with the local legislation and institutional requirements. Written informed consent for participation in this study was provided by the participants’ legal guardians/next of kin.
Author contributions
LH: Conceptualization, Data curation, Formal analysis, Investigation, Methodology, Writing – original draft. JS: Conceptualization, Data curation, Formal analysis, Investigation, Methodology, Writing – review & editing. LL: Conceptualization, Data curation, Investigation, Methodology, Writing – review & editing. TS-S: Data curation, Investigation, Methodology, Writing – review & editing. HZ: Writing – review & editing. KB: Data curation, Writing – review & editing. TH: Conceptualization, Writing – review & editing, Data curation. VW: Conceptualization, Funding acquisition, Investigation, Methodology, Project administration, Resources, Supervision, Writing – review & editing.
Funding
The author(s) declare financial support was received for the research, authorship, and/or publication of this article. This work was supported by the Norwegian Research Council (grant #302079).
Acknowledgments
The authors thank Helene Larssen Mathiesen, Jeanette Dulsrud, Ann Cathrin Pettersen, Siri H. Sletner, Lene Andersen, Birute Stankuniene, and Line Sletner at the Department of Paediatrics and Adolescent Medicine, Akershus University Hospital, Truls Leegaard at the Department of Microbiology and Infection Control, Akershus University Hospital, David Hui and the Unit for Research Support at the Department of Internal Medicine, Akershus University Hospital, for invaluable practical assistance; Trygve Tjade and Sigve Fossum Grande at Fürst Medical Laboratory, Oslo, for laboratory analyses, Kirsten Haugland at Akershus University Hospital and Helge Røsjø and Torbjørn Omland at the University of Oslo and Akershus University Hospital for institutional support; and finally, all the study participants.
Conflict of interest
The authors declare that the research was conducted in the absence of any commercial or financial relationships that could be construed as a potential conflict of interest.
The author(s) declared that they were an editorial board member of Frontiers, at the time of submission. This had no impact on the peer review process and the final decision.
Publisher’s note
All claims expressed in this article are solely those of the authors and do not necessarily represent those of their affiliated organizations, or those of the publisher, the editors and the reviewers. Any product that may be evaluated in this article, or claim that may be made by its manufacturer, is not guaranteed or endorsed by the publisher.
Supplementary material
The Supplementary material for this article can be found online at: https://www.frontiersin.org/articles/10.3389/fneur.2024.1345787/full#supplementary-material
References
1. Huang, C , Huang, L , Wang, Y , Li, X , Ren, L , Gu, X, et al. 6-month consequences of COVID-19 in patients discharged from hospital: a cohort study. Lancet. (2021) 397:220–32. doi: 10.1016/S0140-6736(20)32656-8
2. Huang, L , Yao, Q , Gu, X , Wang, Q , Ren, L , Wang, Y, et al. 1-year outcomes in hospital survivors with COVID-19: a longitudinal cohort study. Lancet. (2021) 398:747–58. doi: 10.1016/S0140-6736(21)01755-4
3. Al-Aly, Z , Xie, Y , and Bowe, B . High-dimensional characterization of post-acute sequelae of COVID-19. Nature. (2021) 594:259–64. doi: 10.1038/s41586-021-03553-9
4. Logue, JK , Franko, NM , McCulloch, DJ , McDonald, D , Magedson, A , Wolf, CR, et al. Sequelae in adults at 6 months after COVID-19 infection. JAMA Netw Open. (2021) 4:e210830. doi: 10.1001/jamanetworkopen.2021.0830
5. Haddad, A , Janda, A , Renk, H , Stich, M , Frieh, P , Kaier, K, et al. Long COVID symptoms in exposed and infected children, adolescents and their parents one year after SARS-CoV-2 infection: a prospective observational cohort study. EBioMedicine. (2022) 84:104245. doi: 10.1016/j.ebiom.2022.104245
6. Premraj, L , Kannapadi, NV , Briggs, J , Seal, SM , Battaglini, D , Fanning, J, et al. Mid and long-term neurological and neuropsychiatric manifestations of post-COVID-19 syndrome: a meta-analysis. J Neurol Sci. (2022) 434:120162. doi: 10.1016/j.jns.2022.120162
7. Molteni, E , Sudre, CH , Canas, LS , Bhopal, SS , Hughes, RC , Antonelli, M, et al. Illness duration and symptom profile in symptomatic UK school-aged children tested for SARS-CoV-2. Lancet Child Adolesc Health. (2021) 5:708–18. doi: 10.1016/S2352-4642(21)00198-X
8. Pinzon, RT , Wijaya, VO , Jody, AA , Nunsio, PN , and Buana, RB . Persistent neurological manifestations in long COVID-19 syndrome: a systematic review and meta-analysis. J Infect Public Health. (2022) 15:856–69. doi: 10.1016/j.jiph.2022.06.013
9. Soriano, JB , Murthy, S , Marshall, JC , Relan, P , and Diaz, JVWHO Clinical Case Definition Working Group on Post-COVID-19 Condition. A clinical case definition of post-COVID-19 condition by a Delphi consensus. Lancet Infect Dis. (2022) 22:e102–7. doi: 10.1016/S1473-3099(21)00703-9
10. Wong, TL , and Weitzer, DJ . Long COVID and Myalgic encephalomyelitis/chronic fatigue syndrome (ME/CFS)—a systemic review and comparison of clinical presentation and symptomatology. Medicina. (2021) 57:418. doi: 10.3390/medicina57050418
11. Havdal, LB , Berven, LL , Selvakumar, J , Stiansen-Sonerud, T , Leegaard, TM , Tjade, T, et al. Neurological involvement in COVID-19 among non-hospitalized adolescents and young adults. Front Neurol. (2022) 13:915712. doi: 10.3389/fneur.2022.915712
12. Virhammar, J , Nääs, A , Fällmar, D , Cunningham, JL , Klang, A , Ashton, NJ, et al. Biomarkers for central nervous system injury in cerebrospinal fluid are elevated in COVID-19 and associated with neurological symptoms and disease severity. Eur J Neurol. (2021) 28:3324–31. doi: 10.1111/ene.14703
13. Kanberg, N , Ashton, NJ , Andersson, LM , Yilmaz, A , Lindh, M , Nilsson, S, et al. Neurochemical evidence of astrocytic and neuronal injury commonly found in COVID-19. Neurology. (2020) 95:e1754–9. doi: 10.1212/WNL.0000000000010111
14. Franke, C , Ferse, C , Kreye, J , Reincke, SM , Sanchez-Sendin, E , Rocco, A, et al. High frequency of cerebrospinal fluid autoantibodies in COVID-19 patients with neurological symptoms. Brain Behav Immun. (2021) 93:415–9. doi: 10.1016/j.bbi.2020.12.022
15. Solomon, T . Neurological infection with SARS-CoV-2 — the story so far. Nat Rev Neurol. (2021) 17:65–6. doi: 10.1038/s41582-020-00453-w
16. Hanson, BA , Visvabharathy, L , Ali, ST , Kang, AK , Patel, TR , Clark, JR, et al. Plasma biomarkers of Neuropathogenesis in hospitalized patients with COVID-19 and those with Postacute sequelae of SARS-CoV-2 infection. Neurol Neuroimmunol Neuroinflamm. (2022) 9:e1151. doi: 10.1212/NXI.0000000000001151
17. Pröbstel, AK , and Schirmer, L . SARS-CoV-2-specific neuropathology: fact or fiction? Trends Neurosci. (2021) 44:933–5. doi: 10.1016/j.tins.2021.10.006
18. Van den Bergh, O , Witthöft, M , Petersen, S , and Brown, RJ . Symptoms and the body: taking the inferential leap. Neurosci Biobehav Rev. (2017) 74:185–203. doi: 10.1016/j.neubiorev.2017.01.015
19. Barrett, LF , and Simmons, WK . Interoceptive predictions in the brain. Nat Rev Neurosci. (2015) 16:419–29. doi: 10.1038/nrn3950
20. Selvakumar, J , Havdal, LB , Drevvatne, M , Brodwall, EM , Lund Berven, L , Stiansen-Sonerud, T, et al. Prevalence and characteristics associated with post-COVID-19 condition among nonhospitalized adolescents and young adults. JAMA Netw Open. (2023) 6:e235763. doi: 10.1001/jamanetworkopen.2023.5763
21. Wang, S , Quan, L , Chavarro, JE , Slopen, N , Kubzansky, LD , Koenen, KC, et al. Associations of depression, anxiety, worry, perceived stress, and loneliness prior to infection with risk of post–COVID-19 conditions. JAMA Psychiatry. (2022) 79:1081–91. doi: 10.1001/jamapsychiatry.2022.2640
22. Fyfe, I . Neurofilament light chain — new potential for prediction and prognosis. Nat Rev Neurol. (2019) 15:557–7. doi: 10.1038/s41582-019-0265-2
23. Zetterberg, H . Is there a value of Neurofilament light as a biomarker for neurodegeneration in Parkinson's disease? Mov Disord. (2020) 35:1111–2. doi: 10.1002/mds.28101
24. Abu-Rumeileh, S , Abdelhak, A , Foschi, M , D'Anna, L , Russo, M , Steinacker, P, et al. The multifaceted role of neurofilament light chain protein in non-primary neurological diseases. Brain. (2023) 146:421–37. doi: 10.1093/brain/awac328
25. Hendricks, R , Baker, D , Brumm, J , Davancaze, T , Harp, C , Herman, A, et al. Establishment of neurofilament light chain Simoa assay in cerebrospinal fluid and blood. Bioanalysis. (2019) 11:1405–18. doi: 10.4155/bio-2019-0163
26. Lee, Y , Lee, BH , Yip, W , Chou, P , and Yip, BS . Neurofilament proteins as prognostic biomarkers in neurological disorders. Curr Pharm Des. (2020) 25:4560–9. doi: 10.2174/1381612825666191210154535
27. Cabezas, JA , Bustamante, A , Giannini, N , Pecharroman, E , Katsanos, AH , Tsivgoulis, G, et al. Discriminative value of glial fibrillar acidic protein (GFAP) as a diagnostic tool in acute stroke. Individual patient data meta-analysis. J Investig Med. (2020) 68:1379–85. doi: 10.1136/jim-2020-001432
28. Laverse, E , Guo, T , Zimmerman, K , Foiani, MS , Velani, B , Morrow, P, et al. Plasma glial fibrillary acidic protein and neurofilament light chain, but not tau, are biomarkers of sports-related mild traumatic brain injury. Brain Commun. (2020) 2:fcaa137. doi: 10.1093/braincomms/fcaa137
29. Watanabe, M , Nakamura, Y , Michalak, Z , Isobe, N , Barro, C , Leppert, D, et al. Serum GFAP and neurofilament light as biomarkers of disease activity and disability in NMOSD. Neurology. (2019) 93:e1299–311. doi: 10.1212/WNL.0000000000008160
30. Abdelhak, A , Foschi, M , Abu-Rumeileh, S , Yue, JK , D’Anna, L , Huss, A, et al. Blood GFAP as an emerging biomarker in brain and spinal cord disorders. Nat Rev Neurol. (2022) 18:158–72. doi: 10.1038/s41582-021-00616-3
31. Sutter, R , Hert, L , de Marchis, GM , Twerenbold, R , Kappos, L , Naegelin, Y, et al. Serum Neurofilament light chain levels in the intensive care unit: comparison between severely ill patients with and without coronavirus disease 2019. Ann Neurol. (2021) 89:610–6. doi: 10.1002/ana.26004
32. Aamodt, AH , Høgestøl, EA , Popperud, TH , Holter, JC , Dyrhol-Riise, AM , Tonby, K, et al. Blood neurofilament light concentration at admittance: a potential prognostic marker in COVID-19. J Neurol. (2021) 268:3574–83. doi: 10.1007/s00415-021-10517-6
33. Sahin, BE , Celikbilek, A , Kocak, Y , Saltoglu, GT , Konar, NM , and Hizmali, L . Plasma biomarkers of brain injury in COVID-19 patients with neurological symptoms. J Neurol Sci. (2022) 439:120324. doi: 10.1016/j.jns.2022.120324
34. Abdelhak, A , Barba, L , Romoli, M , Benkert, P , Conversi, F , D’Anna, L, et al. Prognostic performance of blood neurofilament light chain protein in hospitalized COVID-19 patients without major central nervous system manifestations: an individual participant data meta-analysis. J Neurol. (2023) 270:3315–28. doi: 10.1007/s00415-023-11768-1
35. Rogatzki, MJ , Szeghy, RE , Stute, NL , Province, VM , Augenreich, MA , Stickford, JL, et al. Plasma UCHL1, GFAP, tau, and NfL are not different in young healthy persons with mild COVID-19 symptoms early in the pandemic: a pilot study. Neurotrauma Rep. (2023) 4:330–41. doi: 10.1089/neur.2023.0014
36. Telser, J , Grossmann, K , Weideli, OC , Hillmann, D , Aeschbacher, S , Wohlwend, N, et al. Concentrations of serum brain injury biomarkers following SARS-CoV-2 infection in individuals with and without long-COVID-results from the prospective population-based COVI-GAPP study. Diagnostics. (2023) 13:2167. doi: 10.3390/diagnostics13132167
37. Grizzle, R . Wechsler intelligence scale for children In: Goldstein, S., Naglieri, J.A. (eds) Encyclopedia of Child Behavior and Development. 4th ed Springer, Boston, MA. (2011). 1553–5. https://doi.org/10.1007/978-0-387-79061-9_3066
38. Benedict, RHB , Schretlen, D , Groninger, L , and Brandt, J . Hopkins verbal learning test – revised: normative data and analysis of inter-form and test-retest reliability. Clin Neuropsychol. (2010) 12:43–55. doi: 10.1076/clin.12.1.43.1726
39. Klepstad, P , Loge, JH , Borchgrevink, PC , Mendoza, TR , Cleeland, CS , and Kaasa, S . The Norwegian brief pain inventory questionnaire. J Pain Symptom Manag. (2002) 24:517–25. doi: 10.1016/S0885-3924(02)00526-2
40. Åkerstedt, T , Ingre, M , Kecklund, G , Folkard, S , and Axelsson, J . Accounting for partial sleep deprivation and cumulative sleepiness in the three-process model of alertness regulation. Chronobiol Int. (2009) 25:309–19. doi: 10.1080/07420520802110613
41. Norwegian Institute of Public Health , Norwegian immunisation registry SYSVAK. (n.d.). Available online at: https://www.fhi.no/en/hn/health-registries/norwegian-immunisation-registry-sysvak/ (Accessed ongoing at time of follow-up visits)
42. Fukuda, K , Straus, SE , Hickie, I , Sharpe, MC , Dobbins, JG , and Komaroff, A . The chronic fatigue syndrome: a comprehensive approach to its definition and study. International chronic fatigue syndrome study group. Ann Intern Med. (1994) 121:953–9. doi: 10.7326/0003-4819-121-12-199412150-00009
43. Kanberg, N , Simrén, J , Edén, A , Andersson, LM , Nilsson, S , Ashton, NJ, et al. Neurochemical signs of astrocytic and neuronal injury in acute COVID-19 normalizes during long-term follow-up. EBioMedicine. (2021) 70:103512. doi: 10.1016/j.ebiom.2021.103512
44. Comeau, D , Martin, M , Robichaud, GA , and Chamard-Witkowski, L . Neurological manifestations of post-acute sequelae of COVID-19: which liquid biomarker should we use? Front Neurol. (2023) 14:1233192. doi: 10.3389/fneur.2023.1233192
45. Bark, L , Larsson, IM , Wallin, E , Simrén, J , Zetterberg, H , Lipcsey, M, et al. Central nervous system biomarkers GFAp and NfL associate with post-acute cognitive impairment and fatigue following critical COVID-19. Sci Rep. (2023) 13:13144. doi: 10.1038/s41598-023-39698-y
46. de Boni, L , Odainic, A , Gancarczyk, N , Kaluza, L , Strassburg, CP , Kersting, XAK, et al. No serological evidence for neuronal damage or reactive gliosis in neuro-COVID-19 patients with long-term persistent headache. Neurol Res Pract. (2022) 4:53. doi: 10.1186/s42466-022-00217-5
47. Lennol, MP , Ashton, NJ , Moreno-Pérez, O , García-Ayllón, MS , Ramos-Rincon, JM , Andrés, M, et al. Transient changes in the plasma of astrocytic and neuronal injury biomarkers in COVID-19 patients without neurological syndromes. Int J Mol Sci. (2023) 24:2715. doi: 10.3390/ijms24032715
48. Farhadian, SF , Reisert, HD , McAlpine, L , Chiarella, J , Kosana, P , Yoon, J, et al. Self-reported neuropsychiatric post–COVID-19 condition and CSF markers of Neuroinflammation. JAMA Netw Open. (2023) 6:e2342741–1. doi: 10.1001/jamanetworkopen.2023.42741
49. Søraas, A , Bø, R , Kalleberg, KT , Støer, NC , Ellingjord-Dale, M , and Landrø, NI . Self-reported memory problems 8 months after COVID-19 infection. JAMA Netw Open. (2021) 4:e2118717. doi: 10.1001/jamanetworkopen.2021.18717
50. Brunvoll, SH , Nygaard, AB , Fagerland, MW , Holland, P , Ellingjord-Dale, M , Dahl, JA, et al. Post-acute symptoms 3-15 months after COVID-19 among unvaccinated and vaccinated individuals with a breakthrough infection. Int J Infect Dis. (2023) 126:10–3. doi: 10.1016/j.ijid.2022.11.009
51. Øie, MG , Rødø, ASB , Bølgen, MS , Pedersen, M , Asprusten, TT , and Wyller, VBB . Subjective and objective cognitive function in adolescent with chronic fatigue following Epstein-Barr virus infection. J Psychosom Res. (2022) 163:111063. doi: 10.1016/j.jpsychores.2022.111063
52. Sandler, C , Cvejic, E , Valencia, BM , Li, H , Hickie, IB , and Lloyd, AR . Predictors of chronic fatigue syndrome and mood disturbance after acute infection. Front Neurol. (2022) 13:935442. doi: 10.3389/fneur.2022.935442
53. Su, Y , Yuan, D , Chen, DG , Ng, RH , Wang, K , Choi, J, et al. Multiple early factors anticipate post-acute COVID-19 sequelae. Cell. (2022) 185:881–895.e20. doi: 10.1016/j.cell.2022.01.014
54. Monje, M , and Iwasaki, A . The neurobiology of long COVID. Neuron. (2022) 110:3484–96. doi: 10.1016/j.neuron.2022.10.006
55. Needham, EJ , Ren, AL , Digby, RJ , Norton, EJ , Ebrahimi, S , Outtrim, JG, et al. Brain injury in COVID-19 is associated with dysregulated innate and adaptive immune responses. Brain. (2022) 145:4097–107. doi: 10.1093/brain/awac321
56. Tsagkaris, C , Bilal, M , Aktar, I , Aboufandi, Y , Tas, A , Aborode, AT, et al. Cytokine storm and neuropathological alterations in patients with neurological manifestations of COVID-19. Curr Alzheimer Res. (2022) 19:641–57. doi: 10.2174/1567205019666220908084559
57. De Lorenzo, R , Loré, NI , Finardi, A , Mandelli, A , Cirillo, DM , Tresoldi, C, et al. Blood neurofilament light chain and total tau levels at admission predict death in COVID-19 patients. J Neurol. (2021) 268:4436–42. doi: 10.1007/s00415-021-10595-6
58. Sommen, SL , Havdal, LB , Selvakumar, J , Einvik, G , and Leegaard, TM . Inflammatory markers and pulmonary function in adolescents and young adults 6 months after mild COVID-19. Front Immunol. (2022) 13:1081718. doi: 10.3389/fimmu.2022.1081718
59. Saunders, C , Sperling, S , and Bendstrup, E . A new paradigm is needed to explain long COVID. Lancet Respir Med. (2023) 11:e12–3. doi: 10.1016/S2213-2600(22)00501-X
Keywords: COVID-19, neurofilament, glial fibrillary acidic protein, post-COVID-19 condition, adolescents, cognitive functions, fatigue
Citation: Havdal LB, Selvakumar J, Lund Berven L, Stiansen-Sonerud T, Zetterberg H, Blennow K, Holmøy T and Wyller VBB (2024) Neurological involvement among non-hospitalized adolescents and young adults 6 months after acute COVID-19. Front. Neurol. 15:1345787. doi: 10.3389/fneur.2024.1345787
Edited by:
Pankaj Seth, National Brain Research Centre (NBRC), IndiaReviewed by:
Penghui Wei, Qilu Hospital of Shandong University (Qingdao), ChinaSamir Abu Rumeileh, Martin Luther University of Halle-Wittenberg, Germany
Jolanta B. Zawilska, Medical University of Lodz, Poland
Copyright © 2024 Havdal, Selvakumar, Lund Berven, Stiansen-Sonerud, Zetterberg, Blennow, Holmøy and Wyller. This is an open-access article distributed under the terms of the Creative Commons Attribution License (CC BY). The use, distribution or reproduction in other forums is permitted, provided the original author(s) and the copyright owner(s) are credited and that the original publication in this journal is cited, in accordance with accepted academic practice. No use, distribution or reproduction is permitted which does not comply with these terms.
*Correspondence: Lise Beier Havdal, bGlzZS5iZWllci5oYXZkYWxAYWh1cy5ubw==
†ORCID: Lise Beier Havdal http://Orcid.org/0000-0001-7429-8119