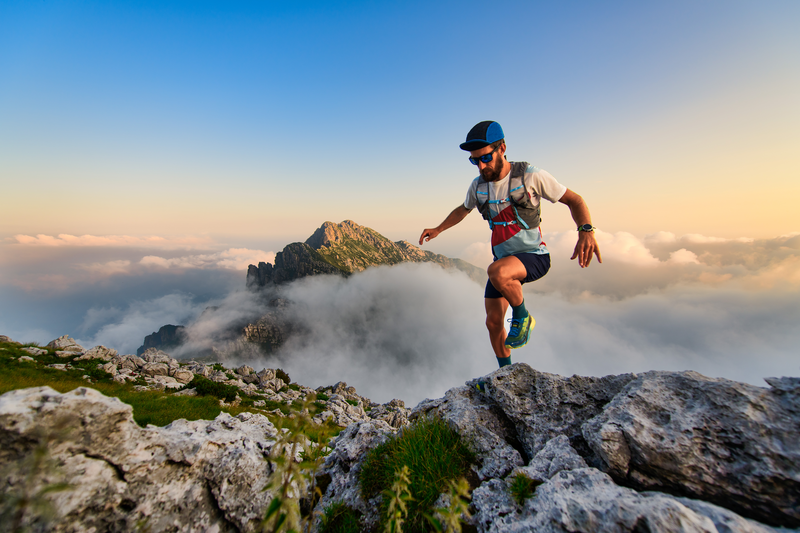
95% of researchers rate our articles as excellent or good
Learn more about the work of our research integrity team to safeguard the quality of each article we publish.
Find out more
ORIGINAL RESEARCH article
Front. Neurol. , 03 May 2024
Sec. Applied Neuroimaging
Volume 15 - 2024 | https://doi.org/10.3389/fneur.2024.1335408
This article is part of the Research Topic Recent insights in Neuroimaging: advancements in therapeutic studies and early diagnosis View all 7 articles
Objectives: Multiple sclerosis (MS) is a demyelinating disorder of the central nervous system. Increasing evidence indicates additional peripheral nerve involvement in early and chronic disease stages. To investigate the evolution of peripheral nerve changes in patients first diagnosed with MS using quantitative MR neurography.
Materials and methods: This prospective study included 19 patients with newly diagnosed MS according to the revised McDonald criteria (16 female, mean 30.2 ± 7.1 years) and 19 age-/sex-matched healthy volunteers. High-resolution 3 T MR neurography of the sciatic nerve using a quantitative T2-relaxometry sequence was performed, which yielded the biomarkers of T2 relaxation time (T2app) and proton spin density (PSD). Follow-up scans of patients were performed after median of 12 months (range 7–16). Correlation analyses considered clinical symptoms, intrathecal immunoglobulin synthesis, nerve conduction study, and lesion load on brain and spine MRI.
Results: Patients showed increased T2app and decreased PSD compared to healthy controls at initial diagnosis and follow-up (p < 0.001 each). Compared to the initial scan, T2app further increased in patients at follow-up (p = 0.003). PSD further declined by at least 10% in 9/19 patients and remained stable in another 9/19 patients. Correlation analyses did not yield significant results.
Conclusion: Peripheral nerve involvement in MS appears at initial diagnosis and continues to evolve within 1 year follow-up with individual dynamics. Quantitative MRN provides non-invasive biomarkers to detect and monitor peripheral nerve changes in MS.
• Growing evidence documents peripheral nerve involvement in multiple sclerosis (MS) already at early disease stages, however, with so far unknown longitudinal development.
• This first longitudinal MR neurography study in multiple sclerosis reveals evolution of quantitative peripheral nerve changes within 1 year follow-up.
• Quantitative MRN provides novel non-invasive biomarkers to detect and monitor peripheral nerve involvement in MS.
Multiple sclerosis (MS) is a demyelinating disease of the central nervous system (CNS). Evidence of spatial and temporal distribution of inflammatory CNS lesions is required according to the revised McDonald criteria of 2017 (1). Recently, there is growing evidence indicating an additional involvement of the peripheral nervous system (PNS) in early and chronic MS, as shown by recent MR studies (2–4), electrophysiology (5–8) as well as neuropathology (9, 10). Understanding the etiology and pathophysiology of these findings is relevant to prevent potentially irreversible peripheral nerve affection.
High-resolution MR neurography (MRN) is a non-invasive imaging method to detect pathological changes in peripheral nerves at high diagnostic sensitivity (11–13). In addition to conventional fat-saturated T2-weighted sequences, more recent quantitative MRN offers the potential to provide novel biomarkers of nerve tissue integrity. As such, quantitative T2-relaxometry sequences have been successfully applied in peripheral neuropathies of various etiologies (14–18). Based on the density and macromolecular composition, the water content of nerve tissue can be estimated, and thus specific patterns of structural nerve damage identified. Thereby, predominant demyelination as opposed to axonal loss can be differentiated.
MRN studies showed peripheral nerve changes in patients with chronic MS but also already at initial diagnosis (2–4). These findings favor a primary inflammatory co-affection of the PNS over secondary Wallerian degeneration or medication-induced effects. However, so far evidence is based on cross-sectional studies. Hence, the aims of the present longitudinal study are to investigate the evolution of peripheral nerve changes in MS patients at initial diagnosis and at 12 months follow-up in correlation with clinical/neurological features, laboratory parameters as well as lesion load on brain and spine MRI.
This prospective, longitudinal, observational study was performed at a single academic medical center following the STROBE guidelines (19). A consecutive sample of 19 patients with MS according to the revised McDonald criteria of 2017 (1) were enrolled at their first clinical presentation during their in-patient stay from 10/2020-08/2022 (flow chart provided in the Supplementary material). Exclusion criteria were any concurrent differential diagnosis to MS, risk factors for peripheral neuropathy such as vitamin deficiency and endocrinological disorders, prior medication with potentially neurotoxic effects, and general MRI contraindications. In addition, 19 demographically matched healthy volunteers without any history of neurological diseases were recruited by public announcement. The study was approved by the local ethics committee (S-405/2012, updated 2018 and 2023) and performed in accordance with the Declaration of Helsinki 2013. Written informed consent was obtained from all participants.
Detailed medical history and comprehensive neurological examination were documented for all patients at initial presentation. Cerebrospinal fluid (CSF) cell count was determined using a Fuchs-Rosenthal chamber (upper limit of normal: 4 cells/μL) and CSF cytology was performed after May–Grünwald staining of individual cytospins. Concentrations of immunoglobulin (Ig) G and IgM were measured in serum and in CSF by standard nephelometric assays to calculate the CSF-to-serum quotients. Intrathecal IgG and IgM synthesis was quantified with the formula by Reiber (20). If quantitative intrathecal IgG synthesis yielded negative results, presence of oligoclonal bands was assessed with isoelectric focusing followed by immunoblotting and considered positive in cases of patterns 2 or 3 (21). Visual evoked potential analysis for P100 latency and amplitude was performed as part of clinical routine in 16/19 patients. Extended nerve conduction studies (NCS) of the same leg as examined with MRN were performed in 18/19 patients. The NCS protocol included distal motor latencies, compound muscle action potentials, and F-waves of the tibial and peroneal nerves, nerve conduction velocities of the tibial, peroneal, and sural nerves, as well as sensory nerve action potentials of the sural nerve. In all NCS, skin temperature was controlled at a minimum of 32°C. The administered dose of intravenous corticosteroids during stationary therapy at initial diagnosis was documented for all patients. At follow-up, patients were asked to complete a questionnaire about any residual or new clinical symptoms and about previous disease-modifying therapies.
Contrast-enhanced brain MRI including high-resolution T1-, T2-weighted and fluid-attenuated inversion recovery (FLAIR) sequences as well as spine MRI studies with sagittal and axial T1- and T2-weighted sequences as available were used to determine the CNS lesion load at initial presentation and at follow-up, i.e., the number of T2- and contrast-enhancing T1-lesions.
High-resolution MRN of patients and healthy volunteers was performed in supine position at a 3 Tesla scanner (MAGNETOM Prisma, Siemens Healthineers, Erlangen, Germany) using a 15-channel transmit/receive array knee coil (Siemens Healthineers). For standardization, the left distal thigh at comparable height was examined in all subjects unless patients had symptoms in only the right lower extremity (clinical data is outlined in Table 1). An axial dual-echo turbo-spin-echo 2D sequence was acquired with repetition time: 5,860 ms, echo times: TE1 = 14 ms and TE2 = 86 ms, field-of-view: 140 × 140 mm2, matrix size: 512 × 512, slice thickness: 3.5 mm, interslice gap: 0.385 mm, flip angle: 180°, spectral fat saturation, and an acquisition time of 8:06 min. At follow-up, patients were examined using identical sequence parameters as in their first scan.
Image analysis was performed blinded to clinical data. The tibial part of the sciatic nerve was manually segmented by a neuroradiologist with 5 years of experience in peripheral nerve imaging. Mean values of signal intensity (SI) were extracted within each region of interest at TE1 and TE2 and then used to calculate the quantitative parameters of T2 relaxation time (T2app; ms Eq. 1) and proton spin density (PSD; unitless Eq. 2) for each slice according to:
Non-parametric Mann–Whitney tests were performed to assess group differences in MRN parameters between patients and healthy controls as well as between patient subgroups. Within-subject differences in patients between the first and second MRN examination were investigated using non-parametric Wilcoxon tests. Spearman’s correlation coefficient was calculated in patients to analyze whether the change in MRN parameters between initial and follow-up scans were associated with the number of T2-lesions on brain or spine MRI, intrathecal IgG rate, the dose of corticosteroids received prior to MRN, the latency of follow-up as well as duration of disease-modifying therapy. Significance was set at p ≤ 0.05 except for correlation analyses where the p-value was adapted to p = 0.01 using Bonferroni’s correction for multiple comparisons. Statistical analyses were performed with Prism (Version 9, GraphPad Software, La Jolla, United States).
Demographic details are outlined in Table 2. Patients and healthy controls did not differ in age (mean: 30.2 ± 7.1 years and 28.4 ± 4.1 years, respectively) or sex (male/female: 16/3 and 16/3) at the time of study enrollment. Patients had sensory and/or motor deficits in at least one leg in 7/19 cases (37%). Mean symptom duration prior to the first MRN study was 16 days (SD 16). The median initial EDSS score was 3.0 (range 2.0–4.5). In CSF studies, intrathecal IgG synthesis was present in 13/19 patients (68%) with a mean rate of 18.2% (SD 19.4). All other patients had evidence of CSF-restricted oligoclonal bands. Intrathecal IgM synthesis was detected in 4/19 patients (21%) with a mean fraction of 2.8% (SD 8.0). NCS was unremarkable in 17/19 patients, and absences of F-waves or A-waves detected in the peroneal nerve in two patients, respectively (Table 1). Intravenous corticosteroid therapy was initiated prior to MRN in 18/19 patients (95%) with a mean duration of 2.9 days (SD 1.6) and a mean dose of 2.8 g (SD 1.5). At follow-up 15/19 (79%) patients received disease-modifying therapy, as outlined in Table 1.
Median latency from first MRN to brain MRI was 4 days (range 0–24) and to spine MRI 2 days (range 0–6). All patients had at least two T2/FLAIR lesions in MS-typical locations on brain MRI (mean 20, SD 14), of which 8 studies showed contrast-enhancing lesions (42%). On spine MRI, 11 patients had MS-typical medullary T2 lesions (mean 2, SD 2) with contrast-enhancement in 6 cases (32%). Follow-up brain MRI was available in 15 patients of whom 12 had no new T2/FLAIR lesions, while spine MRI was available in 11 patients without evidence of new lesions in 9 cases (details in Table 1).
Patients showed increased T2app compared to healthy controls at initial diagnosis and at follow-up (mean 67.9 ms ±5.6 and 71.3 ms ±7.3 vs. 59.0 ± 0.9, p < 0.001; Table 3 and Figure 1). In contrast, PSD was decreased in patients at both time points in comparison to healthy volunteers (mean 369.3 ± 48.5 and 306.9 ± 86.5 vs. 468.9 ± 60.5, p < 0.001). Representative examples are depicted in Figure 2. Compared to the initial scan, T2app further increased in patients at follow-up (mean difference 3.4 ms, p = 0.003; Figure 3). PSD further declined by at least 10% in a subgroup of patients (9/19, 47%), remained stable (i.e., within 10% range) in another 9/19 patients, while it increased in a single patient by 16%. Subgroup analyses of patients did not reveal any significant differences nor correlations between MRN parameters and clinical or radiographic features (Table 4).
Figure 1. Scatter dot plots of T2 relaxation time (T2app) and proton spin density (PSD) in healthy volunteers and patients with multiple sclerosis at initial scan as well as at follow-up. Lines indicate the median. p-values were obtained using Kruskal–Wallis tests between patients and controls or using Wilcoxon tests between patient groups.
Figure 2. Representative images of the sciatic nerve at distal thigh level in a healthy participant (A) and in patient #14 at initial diagnosis (B) and at follow-up (C). Axial fat-saturated T2-weighted (T2w) images of the nerve as well as parameter maps of T2app and PSD with corresponding calibration bars are shown.
Figure 3. Graph showing the change in T2 relaxation time (T2app) and proton spin density (PSD) within individual patients from initial scan (time 1) to follow-up (time 2). p-values were obtained using Wilcoxon tests.
Table 4. Results of correlation analyses between relative change in T2 relaxation time or PSD and clinical/CNS imaging parameters in patients using Spearman’s correlation coefficient.
Multiple sclerosis is characterized by demyelinating lesions of the CNS. Recently, increasing evidence points towards an additional involvement of peripheral nerves in patients with MS (2–10). In line with previous cross-sectional imaging studies, this first longitudinal investigation provides novel insights into the evolution of PNS changes using quantitative T2 relaxometry. At 1 year follow up, both MRN biomarkers of T2app and PSD indicate ongoing affection of peripheral nerves with individual pace of severity. Lack of association with CNS demyelination or disease-modifying therapy strengthens the hypothesis of an inflammatory etiology of the detected PNS changes.
As shown by three recent imaging studies using quantitative MR-neurography, peripheral nerve changes can be detected in early and chronic stages of MS (2–4). The current investigation confirms these previous findings and complements them by a longitudinal perspective from initial diagnosis up to a median of 1 year follow-up. While the T2 relaxation time showed a rather consistent longitudinal increase in the study population, the other analyzed MRN biomarker of PSD was dominated by two distinct subgroups with either ongoing or stable changes. However, we could not clearly identify any separating factor among the included clinical/neurological parameters, CSF markers, electrophysiological studies, CNS lesion load nor therapy regimen. Yet, some analyses were limited by the small sample size of the sub-populations. Future studies should therefore further explore which factors best predict the appearance and individual course of PNS involvement in order to develop even more tailored treatment regimens of MS patients.
Subtyping of MS requires objective biomarkers with high diagnostic accuracy. Quantitative MRN sequences such as T2-relaxometry could provide important novel biomarkers to detect and monitor peripheral nerve affection in MS. It proved to be highly sensitive in various peripheral neuropathies (11, 12, 14, 22) as well as methodologically reliable and robust (23, 24). T2-relaxometry is a quantitative measure of water content and thereby provides biomarkers of nerve tissue integrity (22, 25, 26). The specific pattern of the derived biomarkers T2app and PSD hints at the underlying pathomechanism, e.g., increased T2app was found in peripheral nerve swelling due to amyloidosis or multifocal motor neuropathy (18, 27, 28) and decreased T2app and PSD was detected in predominant axonal damage due to neurodegenerative disorders (14, 22). In line with our findings, acute CNS lesions in MS patients are characterized by an increase in T2app reflecting the demyelinating process (29–31). This pattern remained at 1 year follow-up in our cohort, but may invert over a longer period of time, as observed by a previous MRN study in chronic MS patients with mean 6.8 years of disease history (2). Such a temporal evolution of T2 relaxometry parameters was also described in central MS lesions (31–33). MRN may thus become a valuable diagnostic tool to detect and monitor PNS affection in MS patients, helping to prevent further, possibly irreversible peripheral nerve damage.
Limitations of this study are the moderate sample size precluding detailed subgroup analyses, particularly regarding factors that predict the evolution of T2app and PSD changes over time. Especially, the influence of different therapy regimens should be further elucidated in subsequent studies as well as the possible influence of corticosteroid treatment in the acute setting. Follow-up brain and spine imaging was not available at the same day as MRN in all cases, which would have improved the correlation between PNS and CNS lesions. The dual-echo relaxometry sequence and mono-exponential fit may lead to minor inaccuracies due to B1 field inhomogeneities (34, 35). Absolute T2-relaxometry values, primarily PSD, cannot be easily generalized as they depend on scanner hardware and sequence parameters. Finally, additional MRN sequences such as diffusion-weighted imaging could complement the perspective on pathophysiological changes in peripheral nerves of MS patients.
In conclusion, this study confirms the occurrence of additional peripheral nerve involvement in patients with early-stage MS. Longitudinal data collected over 1 year of follow-up point at sub-populations more susceptible to PNS affection. The pattern of quantitative MRN parameters suggests predominant demyelination and strengthens the hypothesis of an inflammatory co-affection or rather co-demyelination of the PNS. Quantitative MRN sequences could provide non-invasive biomarkers to detect and monitor peripheral nerve involvement in MS.
The raw data supporting the conclusions of this article will be made available by the authors, without undue reservation.
The studies involving humans were approved by the Ethical Board of the University Hospital Heidelberg (S-405/2012). The studies were conducted in accordance with the local legislation and institutional requirements. The participants provided their written informed consent to participate in this study.
OF: Data curation, Formal analysis, Funding acquisition, Investigation, Project administration, Validation, Visualization, Writing – original draft, Writing – review & editing. JH: Conceptualization, Funding acquisition, Methodology, Project administration, Resources, Supervision, Validation, Writing – review & editing. MW: Investigation, Project administration, Resources, Supervision, Validation, Writing – review & editing. GS: Data curation, Investigation, Project administration, Resources, Validation, Writing – review & editing. BW: Resources, Supervision, Validation, Writing – review & editing. WW: Funding acquisition, Resources, Supervision, Validation, Writing – review & editing. MB: Conceptualization, Funding acquisition, Methodology, Resources, Software, Supervision, Validation, Writing – review & editing. SH: Conceptualization, Funding acquisition, Methodology, Resources, Software, Supervision, Validation, Visualization, Writing – review & editing. LJ: Conceptualization, Formal analysis, Investigation, Methodology, Project administration, Resources, Validation, Writing – original draft, Writing – review & editing.
The authors declare that no financial support was received for the research, authorship, and/or publication of this article.
The author(s) declare financial support was received for the research, authorship, and/or publication of this article. OF was supported by the Rahel Goitein-Straus research grant and the Physician-Scientist fellowship of the Medical Faculty of the University of Heidelberg. SH and GS (SFB 1118), MB (SFB 1158), and BW (FOR 2289) were supported by the German Research Council. MB reports personal fees from Novartis, Seagen, Springer, NeuroScios and Boehringer-Ingelheim, as well as grants from the DFG, European Union and Novartis. JH received a research grant, personal fees, lecture honoraria and financial support for conference attendance from Alnylam Pharmaceuticals, and lecture and advisory honoraria from Horizon Therapeutics. MW is a member of the European Reference Network for Neuromuscular Diseases (ERN EURO-NMD). BW received grants from the German Ministry of Education and Research, Dietmar Hopp Foundation, Klaus Tschira Foundation, grants and personal fees from Merck and Novartis, and personal fees from Alexion, INSTAND, Roche, none related to this work. WW reports to be inventor and patent-holder on “Peptides for use in treating or diagnosing IDH1R132H positive cancers” (EP2800580B1) and “Cancer therapy with an oncolytic virus combined with a checkpoint inhibitor” (US11027013B2). He consulted for Apogenix, Astra Zeneca, Bayer, Enterome, MSD and Roche/Genentech with honoraria paid to the Medical Faculty at the University of Heidelberg.
The remaining authors declare that the research was conducted in the absence of any commercial or financial relationships that could be construed as a potential conflict of interest.
All claims expressed in this article are solely those of the authors and do not necessarily represent those of their affiliated organizations, or those of the publisher, the editors and the reviewers. Any product that may be evaluated in this article, or claim that may be made by its manufacturer, is not guaranteed or endorsed by the publisher.
The Supplementary material for this article can be found online at: https://www.frontiersin.org/articles/10.3389/fneur.2024.1335408/full#supplementary-material
CNS, Central nervous system; CSF, Cerebrospinal fluid; FLAIR, Fluid-attenuated inversion recovery; Ig, Immunoglobulin; MRN, Magnetic resonance neurography; MS, Multiple sclerosis; PNS, Peripheral nervous system; SI, Signal intensity.
1. Thompson, AJ, Banwell, BL, Barkhof, F, Carroll, WM, Coetzee, T, Comi, G, et al. Diagnosis of multiple sclerosis: 2017 revisions of the McDonald criteria. Lancet Neurol. (2018) 17:162–73. doi: 10.1016/S1474-4422(17)30470-2
2. Jende, JME, Hauck, GH, Diem, R, Weiler, M, Heiland, S, Wildemann, B, et al. Peripheral nerve involvement in multiple sclerosis: demonstration by magnetic resonance neurography. Ann Neurol. (2017) 82:676–85. doi: 10.1002/ana.25068
3. Pietsch, AM, Viehover, A, Diem, R, Weiler, M, Korporal-Kuhnke, M, Wildemann, B, et al. Quantification and proximal-to-distal distribution pattern of tibial nerve lesions in relapsing-remitting multiple sclerosis: assessment by MR neurography. Clin Neuroradiol. (2022) 33:383–92. doi: 10.1007/s00062-022-01219-1
4. Foesleitner, O, Jäger, LB, Schwarz, D, Hayes, J, Sam, G, Wildemann, B, et al. Peripheral nerve involvement at first diagnosis of multiple sclerosis: a prospective MR neurography study. Investig Radiol. (2023) 58:173–9. doi: 10.1097/RLI.0000000000000915
5. Gartzen, K, Katzarava, Z, Diener, HC, and Putzki, N. Peripheral nervous system involvement in multiple sclerosis. Eur J Neurol. (2011) 18:789–91. doi: 10.1111/j.1468-1331.2010.03149.x
6. Misawa, S, Kuwabara, S, Mori, M, Hayakawa, S, Sawai, S, and Hattori, T. Peripheral nerve demyelination in multiple sclerosis. Clin Neurophysiol. (2008) 119:1829–33. doi: 10.1016/j.clinph.2008.04.010
7. Anlar, O, Tombul, T, and Kisli, M. Peripheral sensory and motor abnormalities in patients with multiple sclerosis. Electromyogr Clin Neurophysiol. (2003) 43:349–51.
8. Sarova-Pinhas, I, Achiron, A, Gilad, R, and Lampl, Y. Peripheral neuropathy in multiple sclerosis: a clinical and electrophysiologic study. Acta Neurol Scand. (1995) 91:234–8. doi: 10.1111/j.1600-0404.1995.tb06996.x
9. Di Trapani, G, Carnevale, A, Cioffi, RP, Massaro, AR, and Profice, P. Multiple sclerosis associated with peripheral demyelinating neuropathy. Clin Neuropathol. (1996) 15:135–8.
10. Pollock, M, Calder, C, and Allpress, S. Peripheral nerve abnormality in multiple sclerosis. Ann Neurol. (1977) 2:41–8. doi: 10.1002/ana.410020107
11. Bendszus, M, and Stoll, G. Technology insight: visualizing peripheral nerve injury using MRI. Nat Clin Pract Neurol. (2005) 1:45–53. doi: 10.1038/ncpneuro0017
12. Kronlage, M, Knop, KC, Schwarz, D, Godel, T, Heiland, S, Bendszus, M, et al. Amyotrophic lateral sclerosis versus multifocal motor neuropathy: utility of MR neurography. Radiology. (2019) 292:149–56. doi: 10.1148/radiol.2019182538
13. Kollmer, J, Bendszus, M, and Pham, M. MR neurography: diagnostic imaging in the PNS. Clin Neuroradiol. (2015) 25:283–9. doi: 10.1007/s00062-015-0412-0
14. Kollmer, J, Hilgenfeld, T, Ziegler, A, Saffari, A, Sam, G, Hayes, JM, et al. Quantitative MR neurography biomarkers in 5q-linked spinal muscular atrophy. Neurology. (2019) 93:e653–64. doi: 10.1212/WNL.0000000000007945
15. Kollmer, J, Weiler, M, Sam, G, Faber, J, Hayes, JM, Heiland, S, et al. Quantitative magnetic resonance neurographic characterization of peripheral nerve involvement in manifest and pre-ataxic spinocerebellar ataxia type 3. Eur J Neurol. (2022) 29:1782–90. doi: 10.1111/ene.15305
16. Jacobi, H, Weiler, M, Sam, G, Heiland, S, Hayes, JM, Bendszus, M, et al. Peripheral nerve involvement in hereditary spastic paraplegia characterized by quantitative magnetic resonance neurography. Eur J Neurol. (2023) 30:2442–52. doi: 10.1111/ene.15841
17. Kronlage, M, Bäumer, P, Pitarokoili, K, Schwarz, D, Schwehr, V, Godel, T, et al. Large coverage MR neurography in CIDP: diagnostic accuracy and electrophysiological correlation. J Neurol. (2017) 264:1434–43. doi: 10.1007/s00415-017-8543-7
18. Foesleitner, O, Knop, KC, Lindenau, M, Preisner, F, Bäumer, P, Heiland, S, et al. Quantitative MR neurography in multifocal motor neuropathy and amyotrophic lateral sclerosis. Diagnostics. (2023) 13:13. doi: 10.3390/diagnostics13071237
19. von Elm, E, Altman, DG, Egger, M, Pocock, SJ, Gøtzsche, PC, and Vandenbroucke, JP. The strengthening the reporting of observational studies in epidemiology (STROBE) statement: guidelines for reporting observational studies. Lancet. (2007) 370:1453–7. doi: 10.1016/S0140-6736(07)61602-X
20. Reiber, H. Cerebrospinal fluid—physiology, analysis and interpretation of protein patterns for diagnosis of neurological diseases. Mult Scler. (1998) 4:99–107. doi: 10.1177/135245859800400302
21. Freedman, MS, Thompson, EJ, Deisenhammer, F, Giovannoni, G, Grimsley, G, Keir, G, et al. Recommended standard of cerebrospinal fluid analysis in the diagnosis of multiple sclerosis: a consensus statement. Arch Neurol. (2005) 62:865–70. doi: 10.1001/archneur.62.6.865
22. Pham, M, Oikonomou, D, Hornung, B, Weiler, M, Heiland, S, Bäumer, P, et al. Magnetic resonance neurography detects diabetic neuropathy early and with proximal predominance. Ann Neurol. (2015) 78:939–48. doi: 10.1002/ana.24524
23. Preisner, F, Behnisch, R, Foesleitner, O, Schwarz, D, Wehrstein, M, Meredig, H, et al. Reliability and reproducibility of sciatic nerve magnetization transfer imaging and T2 relaxometry. Eur Radiol. (2021) 31:9120–30. doi: 10.1007/s00330-021-08072-9
24. Poncelet, A, Weiler, M, Hegenbart, U, Sam, G, Schönland, S, Purrucker, JC, et al. Dual-echo turbo spin echo and 12-echo multi spin echo sequences as equivalent techniques for obtaining T2-relaxometry data: application in symptomatic and asymptomatic hereditary transthyretin amyloidosis as a surrogate disease. Investig Radiol. (2022) 57:301–7. doi: 10.1097/RLI.0000000000000837
25. Mottershead, JP, Schmierer, K, Clemence, M, Thornton, JS, Scaravilli, F, Barker, GJ, et al. High field MRI correlates of myelin content and axonal density in multiple sclerosis—a post-mortem study of the spinal cord. J Neurol. (2003) 250:1293–301. doi: 10.1007/s00415-003-0192-3
26. Tardif, CL, Bedell, BJ, Eskildsen, SF, Collins, DL, and Pike, GB. Quantitative magnetic resonance imaging of cortical multiple sclerosis pathology. Mult Scler Int. (2012) 2012:742018. doi: 10.1155/2012/742018
27. Kollmer, J, Hund, E, Hornung, B, Hegenbart, U, Schönland, SO, Kimmich, C, et al. In vivo detection of nerve injury in familial amyloid polyneuropathy by magnetic resonance neurography. Brain. (2015) 138:549–62. doi: 10.1093/brain/awu344
28. Kollmer, J, Weiler, M, Purrucker, J, Heiland, S, Schönland, SO, Hund, E, et al. MR neurography biomarkers to characterize peripheral neuropathy in AL amyloidosis. Neurology. (2018) 91:e625–34. doi: 10.1212/WNL.0000000000006002
29. Gracien, RM, Reitz, SC, Hof, SM, Fleischer, V, Zimmermann, H, Droby, A, et al. Assessment of cortical damage in early multiple sclerosis with quantitative T2 relaxometry. NMR Biomed. (2016) 29:444–50. doi: 10.1002/nbm.3486
30. Bot, JC, Blezer, EL, Kamphorst, W, Lycklama Nijeholt, GJ, Ader, HJ, Castelijns, JA, et al. The spinal cord in multiple sclerosis: relationship of high-spatial-resolution quantitative MR imaging findings to histopathologic results. Radiology. (2004) 233:531–40. doi: 10.1148/radiol.2332031572
31. MacKay, AL, Vavasour, IM, Rauscher, A, Kolind, SH, Mädler, B, Moore, GRW, et al. MR relaxation in multiple sclerosis. Neuroimaging Clin N Am. (2009) 19:1–26. doi: 10.1016/j.nic.2008.09.007
32. Ormerod, IE, Bronstein, A, Rudge, P, Johnson, G, Macmanus, D, Halliday, AM, et al. Magnetic resonance imaging in clinically isolated lesions of the brain stem. J Neurol Neurosurg Psychiatry. (1986) 49:737–43. doi: 10.1136/jnnp.49.7.737
33. Larsson, HB, Frederiksen, J, Petersen, J, Nordenbo, A, Zeeberg, I, Henriksen, O, et al. Assessment of demyelination, edema, and gliosis by in vivo determination of T1 and T2 in the brain of patients with acute attack of multiple sclerosis. Magn Reson Med. (1989) 11:337–48. doi: 10.1002/mrm.1910110308
34. Milford, D, Rosbach, N, Bendszus, M, and Heiland, S. Mono-exponential fitting in T2-Relaxometry: relevance of offset and first Echo. PLoS One. (2015) 10:e0145255. doi: 10.1371/journal.pone.0145255
Keywords: magnetic resonance imaging, MR neurography, multiple sclerosis, peripheral nervous system, T2 relaxometry
Citation: Foesleitner O, Hayes JC, Weiler M, Sam G, Wildemann B, Wick W, Bendszus M, Heiland S and Jäger LB (2024) Evolution of peripheral nerve changes in early multiple sclerosis—a longitudinal MR neurography study. Front. Neurol. 15:1335408. doi: 10.3389/fneur.2024.1335408
Received: 08 November 2023; Accepted: 12 April 2024;
Published: 03 May 2024.
Edited by:
Kyoko Fujimoto, GE Healthcare, United StatesReviewed by:
Ivan Adamec, University Hospital Centre Zagreb, CroatiaCopyright © 2024 Foesleitner, Hayes, Weiler, Sam, Wildemann, Wick, Bendszus, Heiland and Jäger. This is an open-access article distributed under the terms of the Creative Commons Attribution License (CC BY). The use, distribution or reproduction in other forums is permitted, provided the original author(s) and the copyright owner(s) are credited and that the original publication in this journal is cited, in accordance with accepted academic practice. No use, distribution or reproduction is permitted which does not comply with these terms.
*Correspondence: Olivia Foesleitner, by5mb3NsZWl0bmVyQGdtYWlsLmNvbQ==
Disclaimer: All claims expressed in this article are solely those of the authors and do not necessarily represent those of their affiliated organizations, or those of the publisher, the editors and the reviewers. Any product that may be evaluated in this article or claim that may be made by its manufacturer is not guaranteed or endorsed by the publisher.
Research integrity at Frontiers
Learn more about the work of our research integrity team to safeguard the quality of each article we publish.