- 1Department of Exercise Sciences, Faculty of Science, The University of Auckland, Auckland, New Zealand
- 2Department of Neurology, Auckland City Hospital, Auckland, New Zealand
- 3School of Physiology, University of the Witwatersrand, Johannesburg, South Africa
Background: Accelerometers are commonly used for the assessment of PA; however, these devices have not been validated in people with dystonia who experience movement limitations. To properly understand movement behaviors and deliver accurate exercise prescription in this population, the validity of these devices must be tested.
Objective: This study aimed to validate step count and postural transitions detected by the activPAL accelerometer (AP) against direct observation (DO) during two functional assessments: the 30-s sit-to-stand (30STS) and 6-min usual-pace walk tests. Methods: A total of 11 participants with cervical dystonia (CD) (male/female n = 5/6; mean age = 61 years; BMI = 24 kg/m2) performed the 6-min usual pace walking and 30STS while wearing the activPAL. A trained observer counted steps and observed the number of sit-to-stands.
Results: The average step count detected with AP and DO was 651.8 (218–758) and 654.5 (287–798) respectively. The average transitions detected were 11 (4–16) and 12 (4–17) respectively. Both methods showed good agreement and there was a statistically significant and strong correlation between the two methods, i.e., transitions (r = 0.983, p = 0.0001), and step counts (r = 0.9841, p = 0.0001).
Conclusion: There is a good agreement between activPAL and direct observation for step counts and transitions between sitting and standing in people living with CD.
1 Introduction
Dystonia is an idiopathic disorder, the third most common among movement-related disorders (1). Cervical dystonia (CD) is an isolated form of dystonia affecting 10 in 10,000 people (2), comprising 40% of all dystonias (3). CD affects the neck, resulting in twisted, painful, and non-functional positions of the head (4). Physical function may be impaired in CD, due to the motor and non-motor symptoms (5) that disrupt balance in walking, increasing the risk of falls and (6, 7) resulting in avoidance of participation in physical activity (3). Motor impairments are linked to the pathological impairments affecting different brain areas in cervical dystonia. These areas may include the somato-sensory cortex (impairments causing disruption of movement and position sense), basal ganglia (leads to disruption in mechanism controlling the excessive movement), and cerebellum (results in disruption of balance and postural control) (6, 7). Sometimes visual impairment due to the lack of neck stability may also lead to the loss of balance (6).
There are currently no disease-specific physical activity (PA) guidelines for people with dystonia. As per general population guidelines (8), adults with dystonia should take part in at least 150 min of moderate-intensity physical activity per week (9). PA engagement should be encouraged not only to improve overall health but also to minimize secondary complications, including balance and gait deviations (6, 7, 10–12). Accurately measuring and understanding current PA levels in people with dystonia can assist health care professionals and researchers to develop effective health management plans aimed at promoting health and wellbeing through suitable physical activity engagement.
Several established methods are used to estimate levels of physical activity, each with their own benefits and limitations. Doubly-labelled water is considered the gold standard for estimating energy expenditure during activity (13, 14) but is an expensive and time-consuming method unsuitable for use in free-living settings (15, 16). Self-reported physical activity questionnaires are convenient for distribution in large sample settings, however they are associated with over reporting of physical activity and underreporting of sedentary behavior (SB) levels (17, 18). Accelerometers are an increasingly common way of estimating time spent in different activity behaviors in free living settings (16, 19, 20). These small non-invasive devices are also known to avoid self-report bias and are less expensive compared to doubly labelled water (21). Although accelerometers are relatively accurate in estimating activity in healthy adults (22), they have underestimated low-moderate intensity physical activity in people walking at a slower pace (23–27). Accelerometer data are also mostly interpreted by applying pre-determined thresholds to classify activity behavior intensities. These thresholds have been extensively validated in generally healthy populations, however the assumptions may be limited in CD, where ambulation is challenged, thus compromising the ability to make valid clinical recommendations on promoting healthy activity behaviors. The activPAL is commonly used in studies for the measurement of sedentary behavior (SB) and is considered accurate for this purpose (28) but may miscount steps in people living with chronic conditions that can cause them to walk slow (23–27, 29). Therefore, the aim of this study was to test the criterion validity of step counts and postural transitions detected with the activPAL accelerometer against direct observation in people living with cervical dystonia.
2 Methods
2.1 Study design
This was a single-group, single visit cross-sectional observational study where participants performed a series of functional tests in a laboratory setting.
2.2 Participants
Individuals with cervical dystonia over the age of 18 years were invited to take part in the study. Participants were recruited from the Neurology Department of two major hospitals in the North Island of New Zealand. Participants were also recruited via a dystonia-specific social media network in New Zealand. To be included in the study, participants must have been diagnosed with cervical dystonia of idiopathic origin, have had no other neurological or musculoskeletal condition affecting their ability to participate in physical activity (able to walk independently or with an assistive device), and have not received previous deep-brain stimulation procedures. Eligible participants were fully informed about the study and provided written consent prior to participating in the study. The Auckland Health Research Ethics Committee approved the study on 28/04/2020 (Reference Number AH1116).
2.3 Procedures
Participants performed all procedures at the Health and Rehabilitation Clinic at the Department of Exercise Sciences, University of Auckland, Auckland New Zealand.
The activPAL accelerometer (PAL Technologies, Glasgow, Scotland) was used in this study. The activPAL is a small lightweight triaxial inclinometer that uses static and dynamic accelerations and inclination of the thigh to determine the start and end of each period spent sitting/lying, standing, transitions and stepping (24, 30). The device was initialized and taped to the anterior mid-thigh of each participant’s preferred side of the body (right/left). The device was worn throughout a series of assessments and movement tasks.
Participants were asked to walk around a 50 m indoor track for 6 min at their usual walking pace. While the participant was walking, a researcher followed closely (2 m) behind and recorded step count by using a manual clicker (SHARP ELSI MATE EL-120 Tokyo, Japan). A step was considered from the heel strike of one foot to the heel strike of the other.
After the walking test, participants performed a 30-s sit-to-stand test as previously described (30). Participants were asked to stand from sitting on a box and repeat as many times as they could until the end of 30 s. The height of the box was determined from the participants height (box making around 90°o angle at knees), ensuring a comfortable stand from sitting transition. The number of times the participant completed a transition was recorded. Hence, both measures, walking and sit-standing, were validated against direct observation, which is considered the gold standard while determining the counts of specific activity in the current study, step count and transitions (31).
2.3.1 Data downloading and processing
Data was processed using the activPAL commercially available software (PAL software suite v8, PAL technologies Inc., Scotland). The “events extended” and “raw uncompressed acceleration” data files were generated using the default CREA algorithm (v 1.3). The CREA is considered more accurate than the VANE algorithm for transitions (32). There is an updated CREA version known as GHLA which includes calibration of the sensor. This algorithm is mainly for ActivPAL-4 plus and we used PAL-3 in our study. It is not known if this algorithm has any difference when catered to stepping and transitions in adults. Although it has been tested on children with results showing stepping mean absolute percentage of error being quite high for stepping (5–10 min) (33) these results although cannot be generalized on adults but it is suggested to make caution while comparing the results across studies using these algorithms. The start times of each of the activities (6-min walking and 30-s sit-to-stand) were synchronized to the same computer that the activPAL was initialized on, thus providing the same time stamp. The start and stop times in the activPAL datasets were identified and marked. The difference in cumulative step count at the start and end of the six-minute period was taken as the total number of steps taken in the 6 min. The total number of steps obtained from the activPAL for each individual was multiplied by 2 due to the activPAL recording reciprocal limb movements (leg backwards and forwards) as one-step, while direct observation recorded the leg backwards and then forwards as two steps. Walking cadence (number of steps per minute) was calculated by dividing the total number of steps by 6 min.
The start and stop times of the 30-s sit-to-stand in the raw acceleration file were also identified and marked. The raw acceleration values along the x, y, and z-axes were graphed over the time-period and the number of peaks during that period were counted, one transition was considered from sitting to standing (Figure 1).
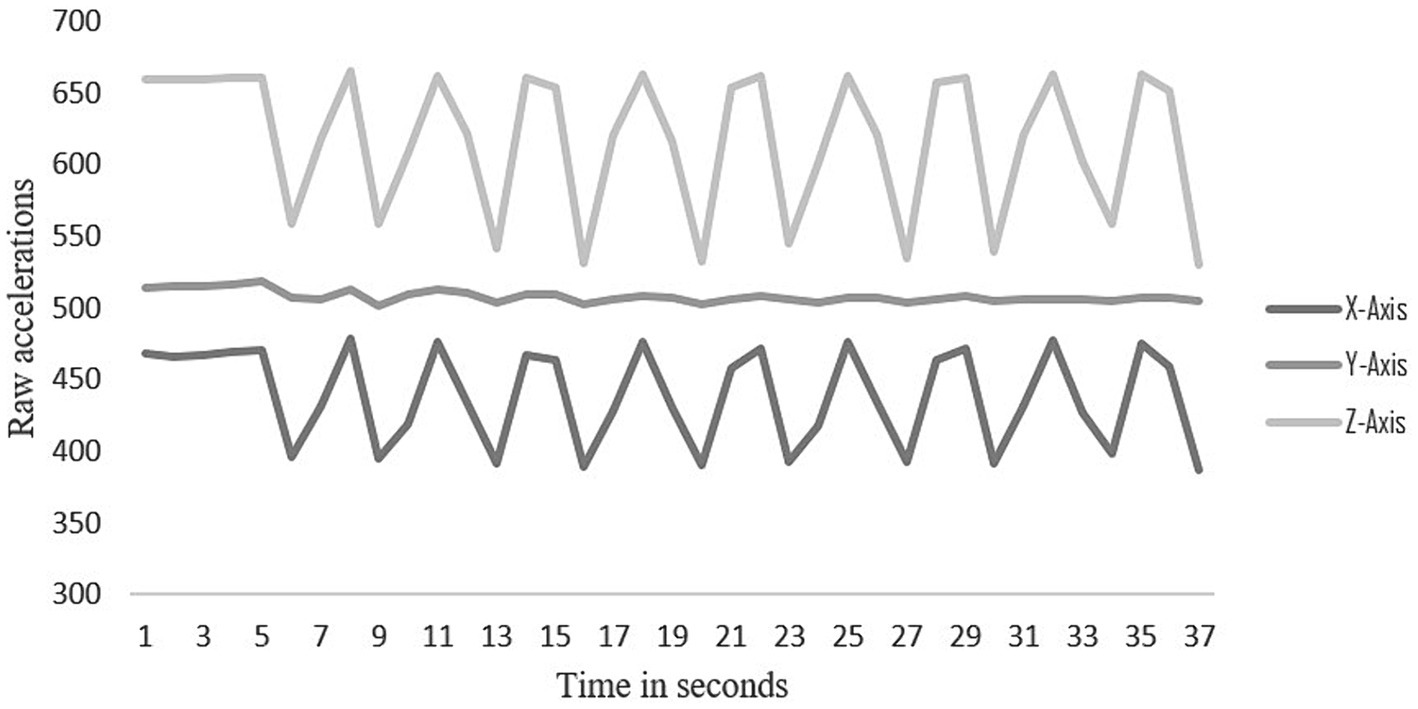
Figure 1. Raw data of one participant showing transitions between sitting and standing on the X, Y and Z axes.
2.4 Statistical analysis
Data was analyzed using IBM SPSS V 23 and MS excel. Pearson’s correlation was used to determine the strength of the relationship between the outputs from the activPAL and those obtained through direct observation. The strength of the relationship was considered based on Dancey and Reidy (34) with ±1 representing perfect correlation, ±0.7 to ±0.9 strong, ±0.4 to ±0.6 moderate, ±0.1 to ±0.3 weak, and 0 considered as no relationship between variables. The Bland–Altman method was used to determine the agreement between the outputs obtained from the activPAL and those obtained through direct observation. The mean of the observations and the difference between the observations (activPAL and direct observations) were calculated and plotted. Statistical limits were calculated as mean ± 1.96 SD. In order for the two methods to have excellent agreement, 95% of the data points from the Bland–Altman plot were to lie within the ±1.96 SD (35). However, because of small sample we were able to collect, 90% agreement was acceptable based on Pedersen et al. (36) who considered 80% agreement acceptable.
3 Results
Twelve eligible individuals took part in the study and completed the procedures. Data from one participant for both assessments was not recorded on the activPAL due to an unknown technical error, while the sit-to-stand data from another participant was not synchronized. Therefore, data from 10 participants with valid sit-to-stand data and 11 participants with valid step count data were included in the final analysis. One participant used crutches as their mobility aid while performing the tests. All participants were using BOTOX® as a treatment except for one participant, who stated the treatment was not effective for controlling their symptoms.
Participants (n = 6 female; n = 5 males) were older adults aged 61 ± 13 (mean ± SD) years with age range between 32 and 73 years. Participants had a BMI of 24 ± 3 kg/m2, ranges between 18 and 32 kg/m2 and the overall group had been living with dystonia for an average of 12 ± 7 years, the duration ranged between 3 and 20 years.
The number of steps and transitions obtained by direct observation and with the activPAL during the functional tests are presented in Supplementary Table S1.
Both the number of steps and transitions between sitting and standing showed a strong positive correlation between the direct observation and activPAL using Pearson’s correlations. The strength of relation is presented as scatter dot plots for step counts during 6 min in Figure 2 and Transitions during 30 s in Figure 3 between the direct observation and activPAL (step count: r = 0.941, transitions: r = 0.983; p = 0.0001****).
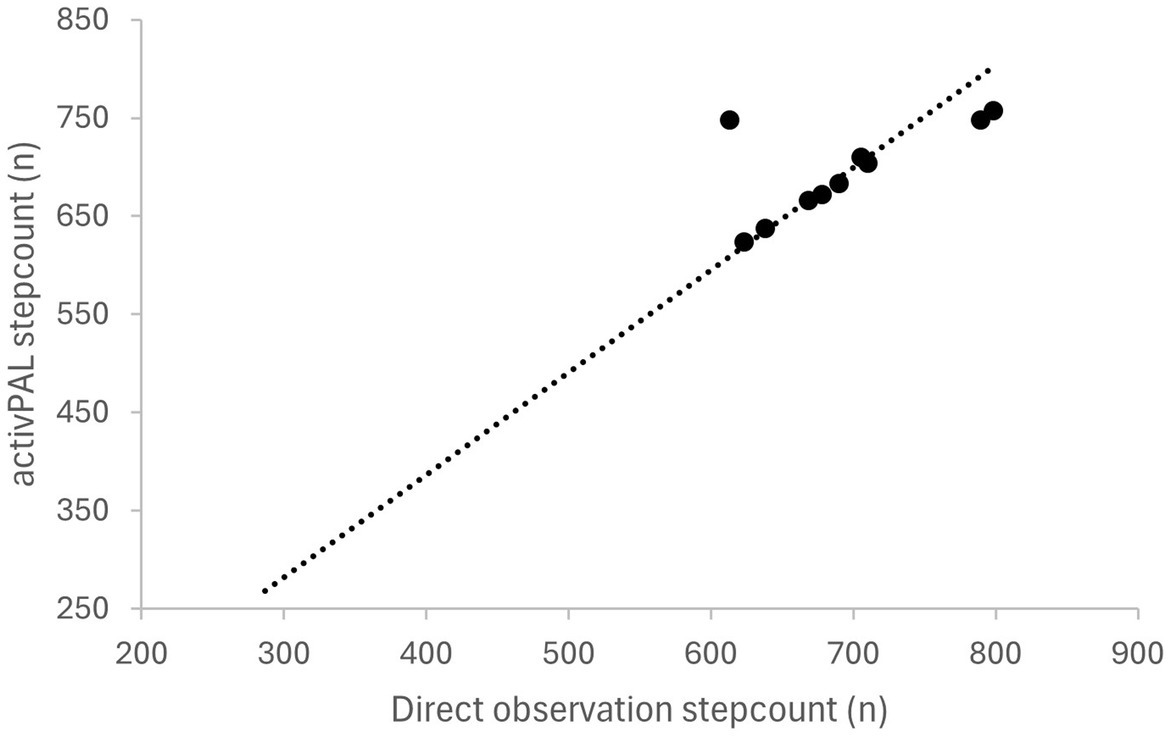
Figure 2. Correlation between step count measured with the activPAL and using direct observation (r = 0.941; p = 0.0001).
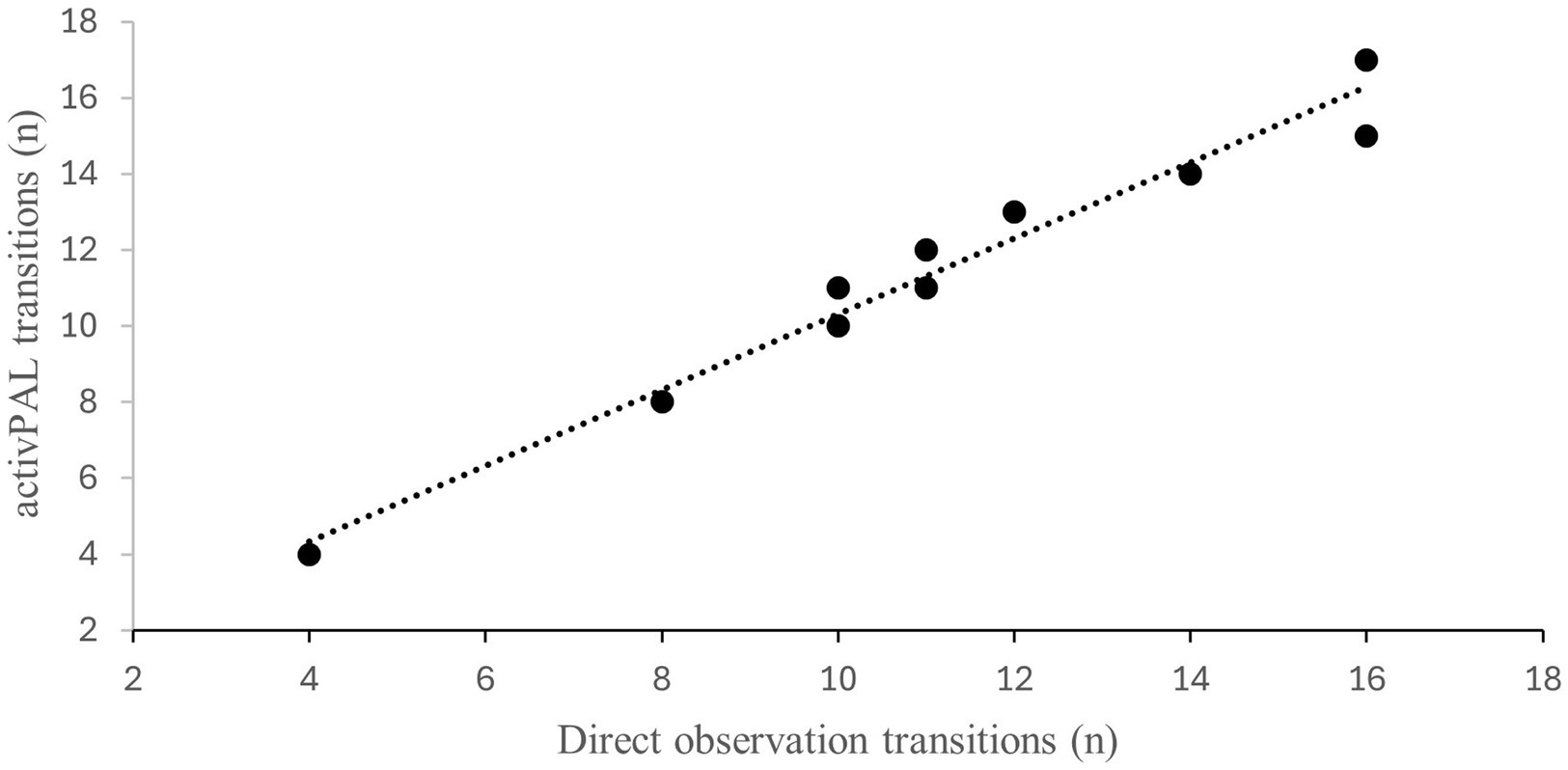
Figure 3. Correlation between transitions measured with the activPAL and using direct observation (r = 0.983; p =0.0001).
Figure 4 shows the Bland–Altman plots for the number of steps taken during the 6 min of walking. There was 91% agreement between the number of steps of walking measured with the activPAL and direct observation. The absolute percentage of error for steps was 0.4%. The limits of agreements in this figure are wide and may be attributed to one of the outlier in the sample. As this outlier has a difference of 135 counts between direct observation and activPAL. The results did not change significantly even after removing the outlier. This outlier was in accordance with the inclusion criteria as well, hence there was no other reason for removing this outlier. Hence this analysis was done by adding the outlier.
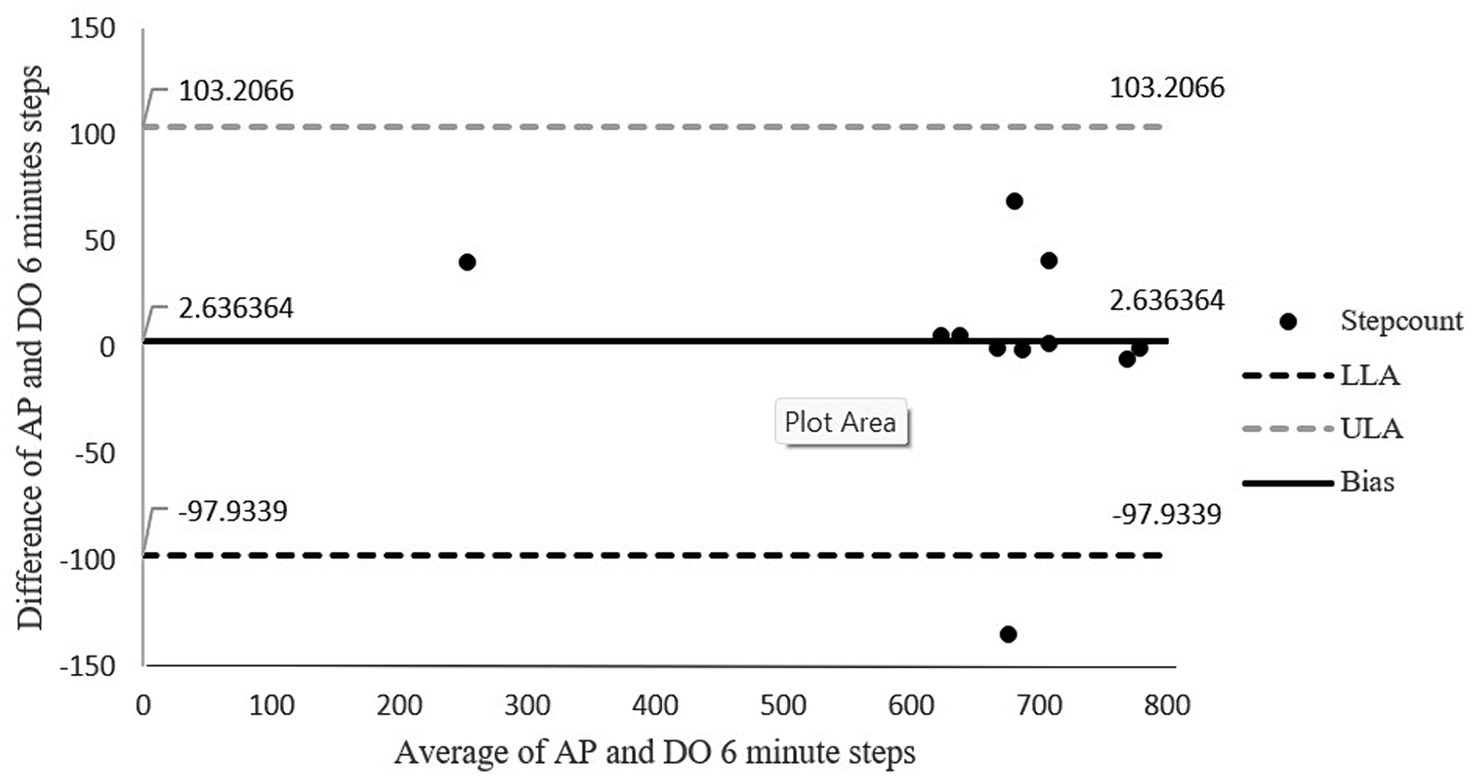
Figure 4. Bland-Altman plot for step count agreement between direct observation and the activPAL. The middle solid line represents the mean of difference (bias) while the outer dotted lines represent the limit of agreements. LLoA: lower limits of agreement; ULoA: upper limit for agreement; Bias: mean of the difference between the two methods.
Figure 5 shows the Bland–Altman plot for the number of sit to stand transitions over a 30-s period. The graph shows good agreement of 100% between the two methods in which entire data lies within the limits of agreements. The absolute percentage of error for transitions was = 2.6.
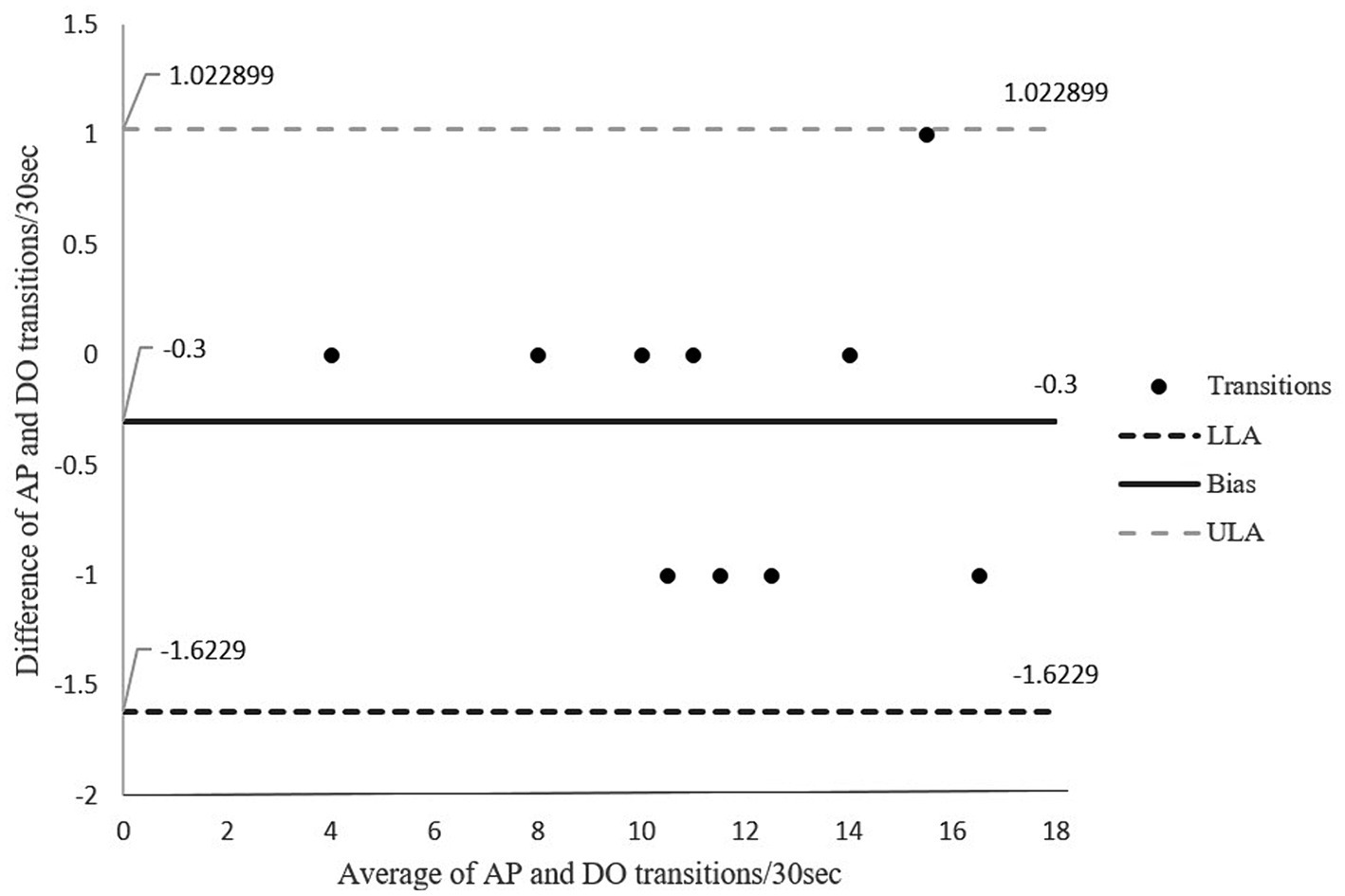
Figure 5. Bland-Altman Plot for agreement in transitions between sitting and standing for direct observation and activPAL transitions. The middle solid line represents the mean of difference (bias), the outer dotted lines represent the limit of agreements. LLoA: lower limits of agreement; ULoA: upper limit for agreement; Bias: mean of the difference between the two methods.
4 Discussion
Overall, this study showed that the activPAL is a valid instrument to assess movement behavior in patients with CD. Our results showed a strong correlation between the criterion measures of directly observed step count and sit-to-stand transitions and those measured using the activPAL accelerometer. In addition, there was good to very high agreement between the measures of direct observation and the activPAL.
A previous study by Grant et al. (37) has used the activPAL to measure steps on a treadmill at five different speeds and during community walking at three self-selected speeds in healthy adults. The authors found that the activPAL was accurate in classifying the number of steps at all speeds including the lowest treadmill speed of 0.67 m/s where less than 100 steps/min were taken, and at the self-paced slow speed of 1.37 m/s (114 steps/min) (37). Furthermore, the absolute percentage error was <1% and there were narrow limits of agreements (37). The activPAL has been commonly used to measure sedentary behavior (SB) and is considered accurate for this purpose (28) but may miscount steps in people living with chronic conditions that can cause them to walk slow (23–27, 29). People with dystonia may present with balance problems, resulting in a slower walking pace (5). In our study, however there was high correlation and good agreement between direct observation and the activPAL for steps in our study. The usual walking speed of participants in our study was mostly at a cadence of >100 steps/min and may be a reason we demonstrated good agreement between measures. This walking cadence is considered as moderate intensity in adults (38–40). Participants in our study may have had normal function due to the reduced severity of their condition attributed to the fact that almost all participants were using BOTOX® as a treatment. In another study related to slow and fast walking in hospitalized patients, participants performed walking activities while wearing an activPAL and SENS monitor and while being directly observed (36). There was a moderate correlation between steps measured with the activPAL and direct observation at the two different speeds. Similarly, there was a good agreement (80%) between the activPAL and direct observation for steps at both walking speeds and the study reported an acceptable measure of error < 15% (36). Similar to the two aforementioned studies (36, 37), 91% of the data in our study was bound within ±1.96 SD, which is considered good agreement.
We also validated the number of transitions recorded by the activPAL against direct observation. A study that validated the activPAL against video recorded observation in healthy adults (41) determined that both step count and transitions were misclassified by the activPAL and the measurement methods showed poor agreement for the outputs in a lab as well as in free living setting (41). The authors reported the reason for the lack of agreement between methods for step count was that less purposeful and small strides were not detected due to the activPAL being attached to one thigh and the relatively long time taken for the activPAL to detect changes in posture. Similarly, in the study population of CD cohort, due to the lack of postural control at neck it is possible that the ActivPAL may have underreported some of the steps.
The number of transitions performed by participants in our study ranged between 4 and 16. A score of less than 12 is below the expected number of transitions for the age range of 60–64 and is indicative of a higher risk of falls (42). The low number in some participants is perhaps not wholly unexpected considering our participants had cervical dystonia. However, given that all our participants bar one appeared relatively functional based on their mean walking cadence (>100 steps/min), we would have expected participants to achieve a higher number of transitions. However repeated sit-to-stands assesses a different aspect of function to prolonged walking, and participants may have had low muscle endurance rather than slow walking ability. Nevertheless, our results showed a strong relationship and excellent agreement with narrow limits and a small margin of error (2.6%) between the two methods for transitions between sitting and standing.
4.1 Limitations
Although the activPAL was accurate in determining step count and transitions between sitting and standing in a controlled environment, we need to be cautious if extrapolating these data to the free-living setting. In addition, people having severe functional limitations may as well yield different results.
In addition, people having severe functional limitations due to dystonia may yield different results, and therefore a larger study on the timing of treatment and severity of disease impacts on activity behaviors should be conducted. Moreover, our study took place during the COVID-19 pandemic, and the unpredictability of the situation resulted in a small sample of participants. However, it is essential to highlight that cervical dystonia is a rare condition; therefore, we did not expect to gather a large sample size.
5 Conclusion
Based on the results of this study, the activPAL is a valid device to measure step counts and sit-to-stand transitions in individuals living with CD. The study found a good agreement between step counts and transitions measured with direct observation and that measured using activPAL.
Data availability statement
The original contributions presented in the study are included in the article/Supplementary material, further inquiries can be directed to the corresponding authors.
Ethics statement
The studies involving humans were approved by the Auckland Health Research Ethics Committee on 28/04/2020 (Reference Number AH1116). The studies were conducted in accordance with the local legislation and institutional requirements. The participants provided their written informed consent to participate in this study.
Author contributions
IY: Conceptualization, Data curation, Formal analysis, Methodology, Writing – original draft, Writing – review & editing. SG: Conceptualization, Writing – review & editing. MS: Data curation, Writing – review & editing. RM: Conceptualization, Methodology, Supervision, Writing – review & editing.
Funding
The author(s) declare financial support was received for the research, authorship, and/or publication of this article. This study was supported by a Maurice and Phyllis Paykel Project grant (grant number: 3720426).
Conflict of interest
The authors declare that the research was conducted in the absence of any commercial or financial relationships that could be construed as a potential conflict of interest.
Publisher’s note
All claims expressed in this article are solely those of the authors and do not necessarily represent those of their affiliated organizations, or those of the publisher, the editors and the reviewers. Any product that may be evaluated in this article, or claim that may be made by its manufacturer, is not guaranteed or endorsed by the publisher.
Supplementary material
The Supplementary material for this article can be found online at: https://www.frontiersin.org/articles/10.3389/fneur.2024.1286447/full#supplementary-material
References
1. Albanese, A, Bhatia, K, Bressman, SB, DeLong, MR, Fahn, S, Fung, VSC, et al. Phenomenology and classification of dystonia: a consensus update. Mov Disord. (2013) 28:863–73. doi: 10.1002/mds.25475
2. Steeves, TD, Day, L, Dykeman, J, Jette, N, and Pringsheim, T. The prevalence of primary dystonia: a systematic review and meta-analysis. Mov Disord. (2012) 27:1789–96. doi: 10.1002/mds.25244
3. McCambridge, A, Meiring, RM, and Bradnam, LV. Physical activity, sedentary behavior, and barriers to exercise in people living with dystonia. Front Neurol. (2019) 10:1121. doi: 10.3389/fneur.2019.01121
4. Colosimo, C, Suppa, A, Fabbrini, G, Bologna, M, and Berardelli, A. Craniocervical dystonia: clinical and pathophysiological features. Eur J Neurol. (2010) 17:15–21. doi: 10.1111/j.1468-1331.2010.03045.x
5. Bradnam, LV, Meiring, RM, Boyce, M, and McCambridge, A. Neurorehabilitation in dystonia: a holistic perspective. J Neural Transm. (2021) 128:549–58. doi: 10.1007/s00702-020-02265-0
6. Barr, C, Barnard, R, Edwards, L, Lennon, S, and Bradnam, L. Impairments of balance, stepping reactions and gait in people with cervical dystonia. Gait Posture. (2017) 55:55–61. doi: 10.1016/j.gaitpost.2017.04.004
7. Boyce, MJ, Lam, L, Chang, F, Mahant, N, Fung, VSC, and Bradnam, L. Validation of fear of falling and balance confidence assessment scales in persons with dystonia. J Neurol Phys Ther. (2017) 41:239–44. doi: 10.1097/NPT.0000000000000198
8. Piercy, KL, and Troiano, RP. Physical activity guidelines for Americans from the US Department of Health and Human Services. Circ Cardiovasc Qual Outcomes. (2018) 11:e005263. doi: 10.1161/CIRCOUTCOMES.118.005263
9. Piercy, KL, Troiano, RP, Ballard, RM, Carlson, SA, Fulton, JE, Galuska, DA, et al. The physical activity guidelines for Americans. JAMA. (2018) 320:2020–8. doi: 10.1001/jama.2018.14854
10. Gallanagh, S, Quinn, TJ, Alexander, J, and Walters, MR. Physical activity in the prevention and treatment of stroke. ISRN Neurol. (2011) 2011:953818:–10. doi: 10.5402/2011/953818
11. Reimers, CD. Physical activity and sports as primary prevention of neurological diseases: a narrative review. Deutsche Z Sportmed. (2019) 2019:57–66. doi: 10.5960/dzsm.2019.372
12. Mahalakshmi, B, Maurya, N, Lee, SD, and Bharath Kumar, V. Possible neuroprotective mechanisms of physical exercise in neurodegeneration. Int J Mol Sci. (2020) 21:5895. doi: 10.3390/ijms21165895
13. Westerterp, KR. Doubly labelled water assessment of energy expenditure: principle, practice, and promise. Eur J Appl Physiol. (2017) 117:1277–85. doi: 10.1007/s00421-017-3641-x
14. Conway, JM, Seale, JL, Jacobs Jr, DR, Irwin, ML, and Ainsworth, BE. Comparison of energy expenditure estimates from doubly labeled water, a physical activity questionnaire, and physical activity records. Am J Clin Nutr. (2002) 75:519–25. doi: 10.1093/ajcn/75.3.519
15. De Waele, E, and van Zanten, ARH. Routine use of indirect calorimetry in critically ill patients: pros and cons. Crit Care. (2022) 26:123. doi: 10.1186/s13054-022-04000-5
16. Speakman, JR, Pontzer, H, Rood, J, Sagayama, H, Schoeller, DA, Westerterp, KR, et al. The International Atomic Energy Agency international doubly labelled water database: aims, scope and procedures. Ann Nutr Metab. (2019) 75:114–8. doi: 10.1159/000503668
17. Sylvia, LG, Bernstein, EE, Hubbard, JL, Keating, L, and Anderson, EJ. Practical guide to measuring physical activity. J Acad Nutr Diet. (2014) 114:199–208. doi: 10.1016/j.jand.2013.09.018
18. Adams, SA, Matthews, CE, Ebbeling, CB, Moore, CG, Cunningham, JE, Fulton, J, et al. The effect of social desirability and social approval on self-reports of physical activity. Am J Epidemiol. (2005) 161:389–98. doi: 10.1093/aje/kwi054
19. Fulk, GD, Combs, SA, Danks, KA, Nirider, CD, Raja, B, and Reisman, DS. Accuracy of 2 activity monitors in detecting steps in people with stroke and traumatic brain injury. Phys Ther. (2014) 94:222–9. doi: 10.2522/ptj.20120525
20. Giggins, OM, Clay, I, and Walsh, L. Physical activity monitoring in patients with neurological disorders: a review of novel body-worn devices. Digit Biomark. (2017) 1:14–42. doi: 10.1159/000477384
21. Curtis, RG, Olds, T, Plotnikoff, R, Vandelanotte, C, Edney, S, Ryan, J, et al. Validity and bias on the online active Australia survey: activity level and participant factors associated with self-report bias. BMC Med Res Methodol. (2020) 20:6. doi: 10.1186/s12874-020-0896-4
22. Lyden, K, Keadle, SK, Staudenmayer, J, and Freedson, PS. The activPALTM accurately classifies activity intensity categories in healthy adults. Med Sci Sports Exerc. (2017) 49:1022–8. doi: 10.1249/MSS.0000000000001177
23. Wu, Y, Petterson, JL, Bray, NW, Kimmerly, DS, and O’Brien, MW. Validity of the activPAL monitor to measure stepping activity and activity intensity: a systematic review. Gait Posture. (2022) 97:165–73. doi: 10.1016/j.gaitpost.2022.08.002
24. Coulter, EH, Miller, L, McCorkell, S, McGuire, C, Algie, K, Freeman, J, et al. Validity of the activPAL3 activity monitor in people moderately affected by multiple sclerosis. Med Eng Phys. (2017) 45:78–82. doi: 10.1016/j.medengphy.2017.03.008
25. Larkin, L, Nordgren, B, Purtill, H, Brand, C, Fraser, A, and Kennedy, N. Criterion validity of the activPAL activity monitor for sedentary and physical activity patterns in people who have rheumatoid arthritis. Phys Ther. (2016) 96:1093–101. doi: 10.2522/ptj.20150281
26. Anens, E, Ahlström, I, Emtner, M, Zetterberg, L, Nilsagård, Y, and Hellström, K. Validity and reliability of physical activity measures in multiple sclerosis. Physiother Theory Pract. (2021) 39:137–53. doi: 10.1080/09593985.2021.1996498
27. Negrini, F, Gasperini, G, Guanziroli, E, Vitale, JA, Banfi, G, and Molteni, F. Using an accelerometer-based step counter in post-stroke patients: validation of a low-cost tool. Int J Environ Res Public Health. (2020) 17:3177. doi: 10.3390/ijerph17093177
28. Hendrickx, W, Riveros, C, Askim, T, Bussmann, JBJ, Callisaya, ML, Chastin, SFM, et al. An exploration of sedentary behavior patterns in community-dwelling people with stroke: a cluster-based analysis. J Neurol Phys Ther. (2021) 45:221–7. doi: 10.1097/NPT.0000000000000357
29. Kanoun, N. Validation of the ActivPAL activity monitor as a measure of walking at pre-determined slow walking speeds in a healthy population in a controlled setting. Reinvention. (2009) 2.
30. Taraldsen, K, Askim, T, Sletvold, O, Einarsen, EK, Grüner Bjåstad, K, Indredavik, B, et al. Evaluation of a body-worn sensor system to measure physical activity in older people with impaired function. Phys Ther. (2011) 91:277–85. doi: 10.2522/ptj.20100159
31. McNamara, E, Hudson, Z, and Taylor, SJC. Measuring activity levels of young people: the validity of pedometers. Br Med Bull. (2010) 95:121–37. doi: 10.1093/bmb/ldq016
32. Montoye, AHK, Vondrasek, JD, Neph, SE, Basu, N, Paul, L, Bachmair, EM, et al. Comparison of the activPAL CREA and VANE algorithms for characterization of posture and activity in free-living adults. J Measur Phys Behav. (2022) 5:49–57. doi: 10.1123/jmpb.2021-0053
33. Buchan, DS, and Ugbolue, UC. Comparing the activPAL CREA and GHLA algorithms for the classification of postures and activity in free-living children. Int J Environ Res Public Health. (2022) 19:15962. doi: 10.3390/ijerph192315962
34. Akoglu, H. User’s guide to correlation coefficients. Turk J Emerg Med. (2018) 18:91–3. doi: 10.1016/j.tjem.2018.08.001
35. Giavarina, D. Understanding bland Altman analysis. Biochem Med. (2015) 25:141–51. doi: 10.11613/BM.2015.015
36. Pedersen, BS, Kristensen, MT, Josefsen, CO, Lykkegaard, KL, Jønsson, LR, and Pedersen, MM. Validation of two activity monitors in slow and fast walking hospitalized patients. Rehabil Res Pract. (2022) 2022:9230081. doi: 10.1155/2022/9230081
37. Grant, PM, Dall, PM, Mitchell, SL, and Granat, MH. Activity-monitor accuracy in measuring step number and cadence in community-dwelling older adults. J Aging Phys Act. (2008) 16:201–14. doi: 10.1123/japa.16.2.201
38. Tudor-Locke, C, Aguiar, EJ, Han, H, Ducharme, SW, Schuna, JM Jr, Barreira, TV, et al. Walking cadence (steps/min) and intensity in 21–40 year olds: CADENCE-adults. Int J Behav Nutr Phys Act. (2019) 16:8. doi: 10.1186/s12966-019-0769-6
39. Tudor-Locke, C, Ducharme, SW, Aguiar, EJ, Schuna, JM, Barreira, TV, Moore, CC, et al. Walking cadence (steps/min) and intensity in 41 to 60-year-old adults: the CADENCE-adults study. Int J Behav Nutr Phys Act. (2020) 17:137. doi: 10.1186/s12966-020-01045-z
40. Tudor-Locke, C, Mora-Gonzalez, J, Ducharme, SW, Aguiar, EJ, Schuna, JM Jr, Barreira, TV, et al. Walking cadence (steps/min) and intensity in 61-85-year-old adults: the CADENCE-adults study. Int J Behav Nutr Phys Acti. (2021) 18:129–9. doi: 10.1186/s12966-021-01199-4
41. Bourke, AK, Ihlen, EA, and Helbostad, JL. Validation of the activPAL3 in free-living and laboratory scenarios for the measurement of physical activity, stepping, and transitions in older adults. J Meas Phys Behav. (2019) 2:58–65. doi: 10.1123/jmpb.2018-0056
Keywords: activPAL, accelerometer, agreement, cervical dystonia, physical activity, sedentary behaviour
Citation: Yaqoob I, Gusso S, Simpson M and Meiring RM (2024) Agreement between the activPAL accelerometer and direct observation during a series of gait and sit-to-stand tasks in people living with cervical dystonia. Front. Neurol. 15:1286447. doi: 10.3389/fneur.2024.1286447
Edited by:
Danúbia Da Cunha De Sá Caputo, Rio de Janeiro State University, BrazilReviewed by:
Raymond Lotilla Rosales, University of Santo Tomas, PhilippinesAdérito Ricardo Duarte Seixas, Escola Superior de Saúde Fernando Pessoa, Portugal
Copyright © 2024 Yaqoob, Gusso, Simpson and Meiring. This is an open-access article distributed under the terms of the Creative Commons Attribution License (CC BY). The use, distribution or reproduction in other forums is permitted, provided the original author(s) and the copyright owner(s) are credited and that the original publication in this journal is cited, in accordance with accepted academic practice. No use, distribution or reproduction is permitted which does not comply with these terms.
*Correspondence: Rebecca M. Meiring, rebecca.meiring@auckland.ac.nz