- 1Health Economics Resource Center (HERC), Ci2i, VA Palo Alto Health Care System, Menlo Park, CA, United States
- 2Center for Healthcare Organization and Implementation Research, VA Boston Healthcare System, Boston, MA, United States
- 3Boston University School of Public Health, Boston, MA, United States
- 4Informatics, Decision-Enhancement, and Analytic Sciences Center of Innovation, VA Salt Lake City Health Care System, Salt Lake City, UT, United States
- 5Division of Epidemiology, Department of Internal Medicine, University of Utah School of Medicine, Salt Lake City, UT, United States
- 6Department of Physical Medicine and Rehabilitation, School of Medicine, Virginia Commonwealth University, Richmond, VA, United States
Background: While emerging evidence supports a link between traumatic brain injury (TBI) and progressive cognitive dysfunction in Veterans, there is insufficient information on the impact of cannabis use disorder (CUD) on long-term cognitive disorders. This study aimed to examine the incidences of cognitive disorders in Veterans with TBI and CUD and to evaluate their relationship.
Methods: This retrospective cohort study used the US Department of Veterans Affairs and Department of Defense administrative data from the Long-term Impact of Military-Relevant Brain Injury Consortium-Chronic Effects of Neurotrauma Consortium Phenotype study. Diagnoses suggesting cognitive disorders after a TBI index date were identified using inpatient and outpatient data from 2003 to 2022. We compared the differential cognitive disorders incidence in Veterans who had the following: (1) no CUD or TBI (control group), (2) CUD only, (3) TBI only, and (4) comorbid CUD+TBI. Kaplan-Meier analyses were used to estimate the overall cognitive disorders incidence in the above study groups. The crude and adjusted Cox proportional hazards models were used to estimate crude and adjusted hazard ratios (HRs) for cognitive disorders.
Results: A total of 1,560,556 Veterans [82.32% male, median (IQR) age at the time of TBI, 34.51 (11.29) years, and 61.35% white] were evaluated. The cognitive disorder incidence rates were estimated as 0.68 (95% CI, 0.62, 0.75) for CUD only and 1.03 (95% CI, 1.00, 1.06) for TBI only per 10,000 person-months of observations, with the highest estimated cognitive disorder incidence observed in participants with both TBI and CUD [1.83 (95% CI, 1.72, 1.95)]. Relative to the control group, the highest hazard of cognitive disorders was observed in Veterans with CUD+TBI [hazard ratio (HR), 3.26; 95% CI, 2.91, 3.65], followed by those with TBI only (2.32; 95 CI%, 2.13, 2.53) and with CUD (1.79; 95 CI%, 1.60, 2.00). Of note, in the CUD only subgroup, we also observed the highest risk of an early onset cognitive disorder other than Alzheimer's disease and Frontotemporal dementia.
Discussion: The results of this analysis suggest that individuals with comorbid TBI and CUD may be at increased risk for early onset cognitive disorders, including dementia.
Introduction
Individuals with traumatic brain injury (TBI), independent of severity, are at increased risk for dementia (1) a neurodegenerative disorder that is characterized by a decline in one or more cognitive domains (2) and profoundly affects mortality, quality of life, caregiver stress, and economic burden (3). The risk of dementia is of particular concern for Veterans with TBI since they frequently present with other associated risk factors for dementia, including post-traumatic stress disorder (PTSD), depression, and sleep impairment that may compound risk and accelerate neurodegenerative processes (4, 5). TBI has been a central focus of morbidity in recent war efforts, as nearly 20% of the more than 2.5 million deployed U.S. military Service Members and Veterans (SMVs) since 2003 sustained at least one TBI (6, 7). Importantly, more than 80% of the TBIs are mild in severity (mTBI) and up to 8% of all Veterans who have sustained TBI are expected to have persistent symptoms related to the event more than 6 months post-injury (8, 9). Difficulty with cognitive, affective, somatosensory, and vestibular symptoms are common post-TBI complaints (10, 11). Post-9/11 combat-deployed service members are at risk of single and repetitive blast and non-blast injuries, in particular mild TBIs (12). Although yet to be fully defined, the mechanisms by which TBI promotes neurodegeneration may be modulated by an array of processes manifesting from insult related neuropathological changes that may be further exacerbated by repetitive injury (13, 14). A history of TBI exposure may also accelerate the time to dementia diagnosis (15), evidenced by a recent study showing an increased risk for early-onset dementia in young post-9/11 Veterans with prior TBI (16).
No study has demonstrated the beneficial effects of smoking marijuana (17). To date, the United States Food and Drug Administration (FDA) has not recommended cannabis for the treatment of any disease or condition (18). Cannabis use disorder (CUD) is defined as problematic marijuana use that causes impairment or distress, without necessarily leading to addiction (19, 20). Zehra et al. (21) suggested that CUD possesses addictive properties akin to other drugs of abuse. Despite the lack of efficacy, cannabis is frequently used to self-treat a wide array of symptoms and conditions, including those associated with persistent post-concussion symptoms (e.g., chronic pain, headache, insomnia, anxiety, irritability, etc.) (22–25). Owing to expanded legalization, lower perceptions of risk, and the absence of established medication regimens (26), the use of cannabis for symptom management following TBI has likely increased (25, 27) in parallel with growing trends in overall use in both the general U.S. population (28) and in Veterans (29–32). Cannabinoids may regulate some of the processes that lead to neurodegeneration (33), and therefore may be useful in the treatment of neurodegenerative dementias such as Alzheimer's disease (AD), in particular for symptoms of agitation (34, 35). However, to date, systematic reviews have noted that available data evaluating cannabinoids for the treatment of dementia progression are insufficient to draw clear conclusions (36, 37). Additionally, studies have shown that cannabis use acutely impairs cognitive functions including attention, concentration, episodic memory, and associative learning in a dose-dependent fashion (38, 39). As observed in some types of dementia, structural changes, such as decreases in regional brain volume in the hippocampus, amygdala, and striatum have also been linked to heavy, chronic cannabis use (40–45). The existence of these non-conclusive and contradictory studies on effectiveness of cannabis on dementia treatment warrant further study. The objective of this study was to examine the association of CUD in the emergence of cognitive disorders in Post-9/11 Veterans diagnosed with TBI.
Methods
Participants and data source
The cohort for this retrospective analysis included participants from the Long-term Impact of Military-Relevant Brain Injury Consortium–Chronic Effects of Neurotrauma Consortium (LIMBIC-CENC) Phenotype study. As described in detail previously (46), this is a large cohort of Post-9/11 active duty and veteran U.S. military persons who received care in the Department of Defense (DoD) for at least 3 years, including those exposed and unexposed to TBI(s). Data for this study included healthcare data during deployment [e.g., DoD Trauma Registry (DoDTR) and Theater Management Data Store (TMDS)], DoD, VA, and Non-VA community inpatient and outpatient data. To ensure accurate TBI status and sufficient data to identify cognitive disorder, we included only those participants who also had 2 years of care in the Veterans Health Administration (VHA) during the study period. The research protocol was reviewed and approved by the University of Utah and Stanford institutional review board (IRB) and was conducted in accordance with all applicable federal regulations.
Measures and outcomes
Development of study groups
We used a hierarchical approach to identify TBI by prioritizing data from DoDTR and TMDS (Glasgow Coma Scale score, Abbreviated Injury Severity Score, and ICD-9-CM and ICD-10-CM codes), followed by self-reported data from the comprehensive TBI evaluation (CTBIE) data collected in the process of clinical care loss of consciousness (mild, ≤ 30 min; moderate to severe, >30 min), alteration of consciousness or posttraumatic amnesia (mild <24 h; moderate to severe, ≥24 h 16), and ICD-9/10-CM diagnosis codes from the Armed Forces Health Surveillance Division algorithm (47). The index date for TBI was the first date of diagnosis or the date of the CTBIE assessment; for those with more than one TBI documented we used the date of the most severe TBI. Veterans who did not enroll in VHA and did not complete the initial VA screening for TBI were excluded from the study (Figure 1). For those without TBI we calculated simulated TBI index dates using a Monte Carlo simulation to generate age-correlated index dates (16). To establish comparable analysis time windows across groups, it was necessary to assign a simulated index date to each individual in the non-TBI group. The simulated index dates were drawn at random from the real distribution of injury dates. To further refine this approach, the simulated index dates were only sampled from a subset of those in the TBI group who were of a similar age as the TBI negative individual (within 5 years) (16).
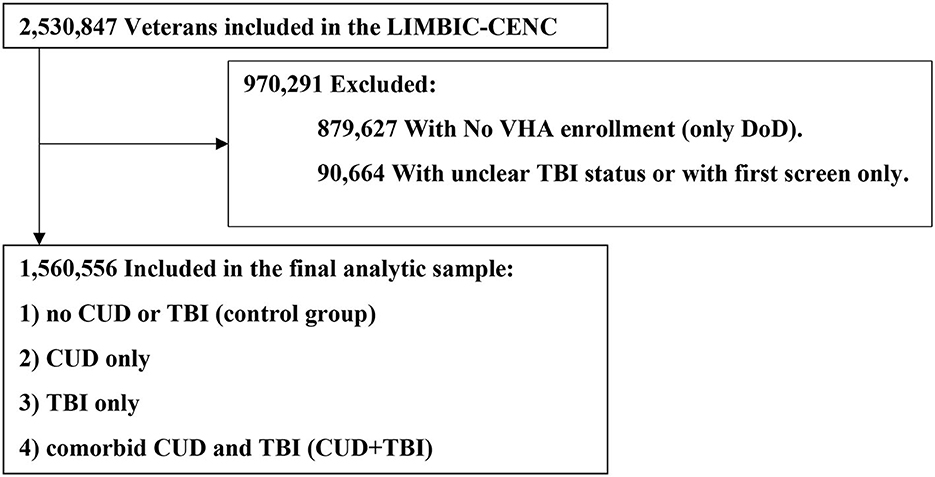
Figure 1. Study flow chart for impact of cannabis on cognitive disorder in Veteran patients with TBI. CUD, Cannabis Use Disorder; DoD, Department of Defense; LIMBIC-CENC, Long-term Impact of Military-Relevant Brain Injury Consortium-Chronic Effects of Neurotrauma Consortium; TBI, Traumatic Brain Injury; VHA, Veterans Health Administration.
Cognitive disorder was indicated by ICD-9/10 diagnosis codes used to identify dementia by VHA Geriatrics and Extended Care (Supplementary Table 3); ascertainment required a single ICD-9/10 dementia diagnosis code after TBI index date through September 11, 2022. Since prior research indicates that these diagnoses are not accurate for individuals under age 65 (48, 49), we classified these diagnoses simply as a “cognitive disorder.” To increase confidence in confirmed dementia cases, we performed a sensitivity analysis on the cohort with at least two ICD-9/10 codes for dementia diagnosis and in the subgroup of 1) early onset of dementia (EOD) (16), consisting of AD (ICD10 = G30.0), and Frontotemporal dementia (FTD) and 2) non-EOD subgroups consisting of early onset cognitive disorder other than AD and FTD.
Primary outcome
The primary outcome, time from TBI index date to cognitive disorder diagnosis, was calculated using the TBI/simulated index date and the first documented diagnosis indicating cognitive disorder. To evaluate the associations among CUD and cognitive disorder emergence following TBI, we categorized the cohort into four groups: (1) Neither CUD nor TBI (control group), (2) TBI only, (3) CUD only, and (4) comorbid CUD and TBI (CUD+TBI). Cannabis CUD was identified using ICD-9 (304.3: Cannabis dependence, and 305.2: Non-dependent cannabis abuse), and ICD-10 codes (F12: Cannabis-related disorders including F12.1 Cannabis abuse, F12.2 Cannabis dependence, and F12.9 Cannabis use, unspecified) for any ICD-9/10 diagnoses after TBI index date.
Covariates
Sociodemographic and military characteristics, including biological sex, age at index date, race/ethnicity, education, marital status, branch, rank, rural/urban location of Veteran residence, and VA service-connected disability group were obtained from the VA DoD Identity Repository (VADIR; FY00-FY19) at the time of military discharge.
Clinical characteristics
We identified comorbid conditions using ICD-9 and 10 codes obtained from the VA Corporate Data Warehouse (CDW) and the DoD and VA Infrastructure for Clinical Intelligence (DaVINCI). Conditions were identified using algorithms provided in Supplementary Table 3 when individuals had one or more ICD-9 and 10 diagnoses between TBI index date to September 11, 2022. We identified the Medication-assisted treatment (MAT) after the TBI index date using the algorithm provided by VA Pharmacy Benefits Management Services (Supplementary Table 3). The U.S. district/region is identified using the VA medical center (stations) that was assigned based on the Veteran home address (Veteran residence).
Statistical analysis
Descriptive analyses of demographic characteristics and risk behaviors from baseline data by CUD and TBI status were conducted. We estimated the cognitive disorder incidence rates (IRs) using Kaplan-Meier methods for the overall cohort and for each of the four groups. Participants were censored at the date of their last health care system encounter or September 11, 2022 (whichever came first). We used Cox proportional hazards models to calculate the crude and adjusted CUD and TBI-specific hazard ratio (HR) for cognitive disorder incidence using CUD+TBI as the main exposure, controlling for sociodemographic and clinical characteristics. To increase confidence in confirmed dementia cases, we performed a sensitivity analysis by re-running the Cox proportional hazards models in the EOD and Non-EOD cohort (Table 2). All analyses were conducted using Stata version 17 (StataCorp LP, College Station, TX).
Results
Sociodemographic and clinical characteristics
A total of 1,560,556 Veterans were included in the analysis and stratified by TBI and CUD status. Table 1 presents some of the key demographic and health characteristics of each of the four groups. A fuller range of these variables may be seen in Supplementary Table 1, which also includes standardized mean differences between the clinical and population differences by TBI and CUD status. The median (IQR) age at the time of TBI was 34.51 (11.29) years. Veterans with CUD+TBI tended to be male, with a high school education or less, were enlisted in the Army, and had higher service-connected disability percentages compared with the other 3 groups (Table 1). The TBI-CUD group also had higher rates of diagnoses for headache, other chronic pain, participation in MAT programs, mental health conditions [i.e., severe mental illness (such as schizophrenia, Bipolar II disorder), depression, PTSD, personality disorder, anxiety, insomnia], and alcohol, opioid, and other substance use disorders compared with the other groups.
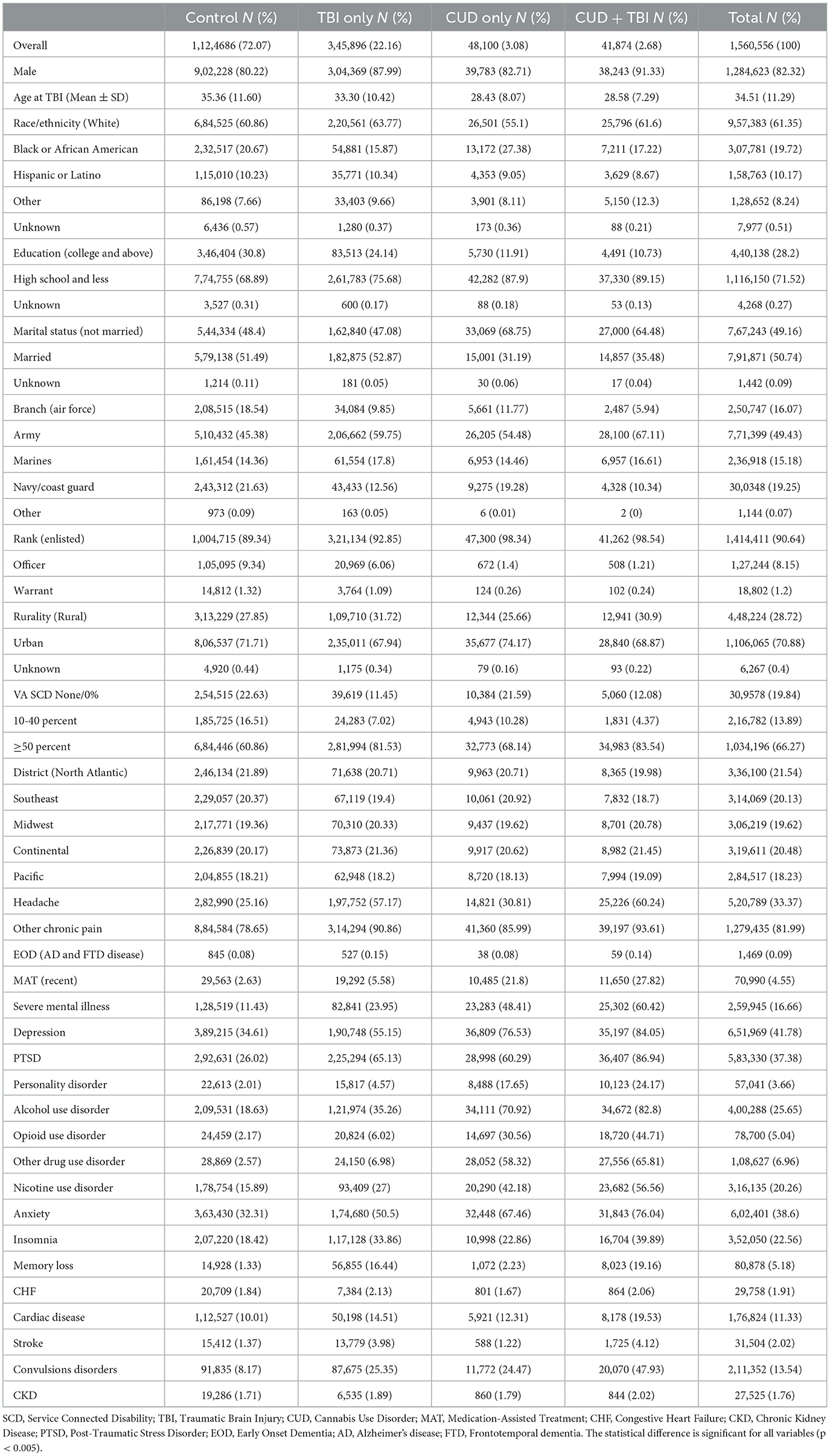
Table 1. Demographic and clinical characteristics of veterans by CUD and TBI status (N = 1,560,556).
Cognitive disorder IR and hazard ratio by TBI and CUD status
The cognitive disorder IR and corresponding HR by TBI and CUD status are shown in Table 2. Overall, we identified 9,844 Veterans with a history of any type of cognitive disorder. The overall cognitive disorder IR was estimated as 0.52 (95% CI: 0.51, 0.53) per 10,000 person months of observations (PMO). After controlling for all demographic and risk factors, the hazard of cognitive disorder was 2.32 (95% CI: 2.13, 2.53), 1.79 (95% CI: 1.60, 2.00), and 3.26 (95% CI: 2.91, 3.65) for Veterans with TBI only, CUD only, and CUD+TBI, respectively, compared to the control group. Figure 2 shows the time from TBI to cognitive disorder by subgroup. Despite a very low incidence of cognitive disorder in our cohort, the risk of cognitive disorder was significantly higher in Veterans with CUD+TBI. The cognitive disorder rate was 0.25%, 0.30%, and 4.4% at 5, 10, and 15 years after TBI, respectively, in Veterans with CUD + TBI. After controlling for all demographic and risk factors, the modifying effect (interaction term) between CUD and TBI on the progression of dementia was <22% the expected rate for the combined risks of TBI and CUD [Supplementary Table 2, HR = 0.78 (95% CI: 0.69, 0.89)] in Veterans diagnosed with TBI.
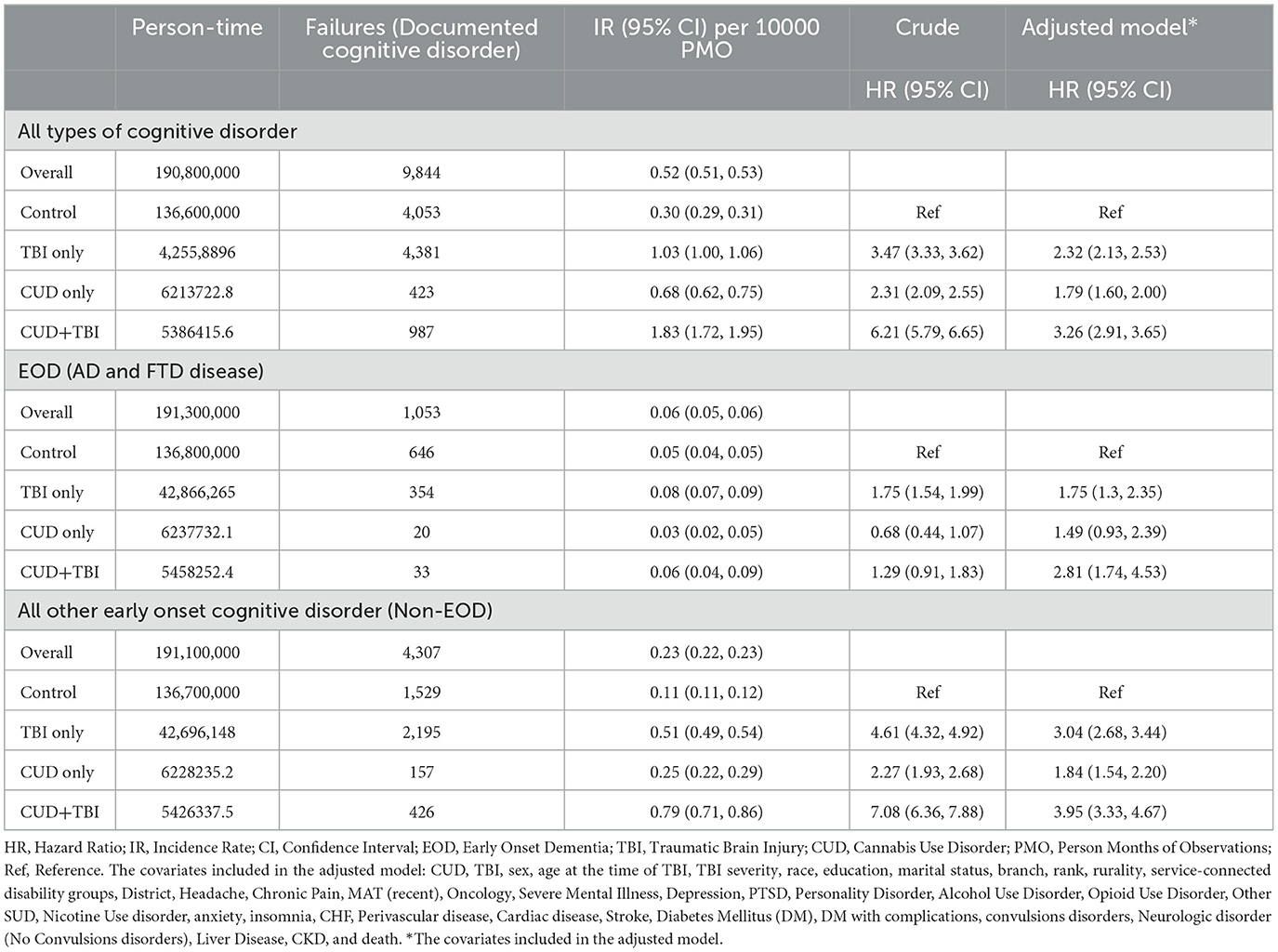
Table 2. Cognitive disorder incidence rate (overall and by TBI and CUD status), and hazard ratio of dementia by CUD and TBI status.
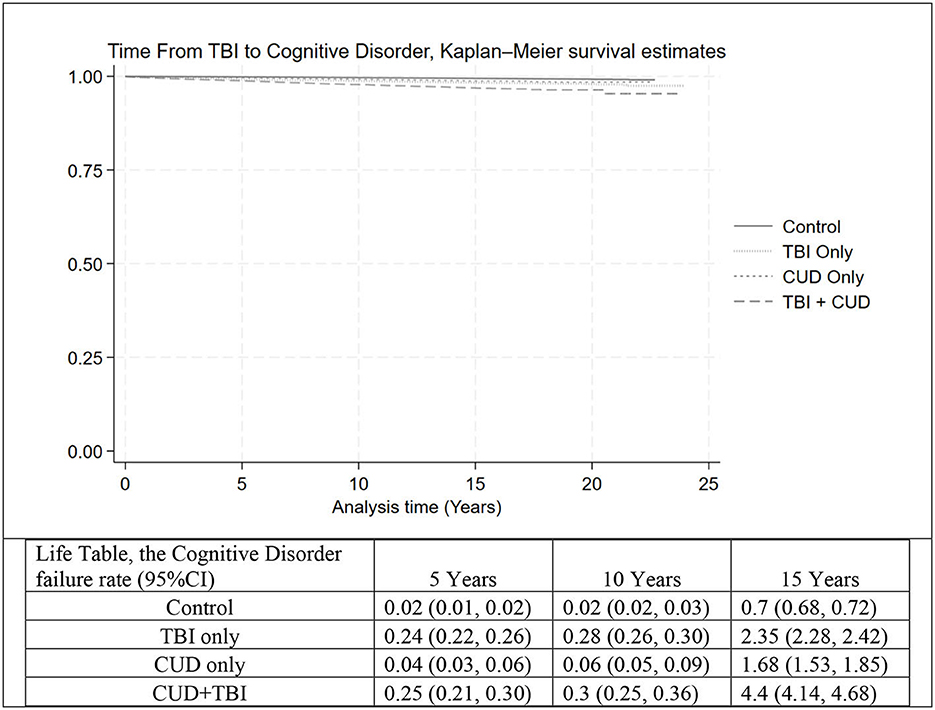
Figure 2. The time from TBI (or TBI index date in the control group) to cognitive disorder, Kaplan–Meier survival estimate and life table, in four groups: Control, TBI only, CUD only, and CUD+TBI. TBI, Traumatic Brain Injury; CUD, Cannabis Use Disorder.
EOD vs. non-EOD
Among the 9,844 Veterans with an assigned diagnosis of cognitive disorder, 5,360 were identified as early onset cognitive disorder with at least 2 documented dementia diagnoses (1,053 and 4,307 Veterans with EOD and non-EOD, respectively, Table 2). The crude and adjusted HRs of the CUD+TBI, CUD only, and TBI only groups, relative to the control groups, were lower in the EOD subgroup relative to the Non-EOD group. We did not observe any significant differences in the hazard of EOD between the CUD only and control groups.
EOD and non-EOD among Veterans with TBI
We examined factors related to EOD and Non-EOD development among Veterans with TBI, after adjusting for select variables: TBI severity, sex, race/ethnicity, and education, as shown in Table 3. More severe categories of TBI were associated with higher risk of dementia development, as observed with penetrating and moderate/severe TBI; and conversely, less severe TBI, specifically mild TBI and post concussive syndrome (which is indicative of mild TBI), were associated with lower risk of EOD and non-EOD development relative to no TBI. Other factors related to increased risk of EOD were being male, older age at time of TBI, and Hispanic or Latino ethnicity (relative to White). Other factors related to Non-EOD development were being male, older age at time of TBI, Black or African American (relative to White), and having up to a high school education (relative to completing college or higher).
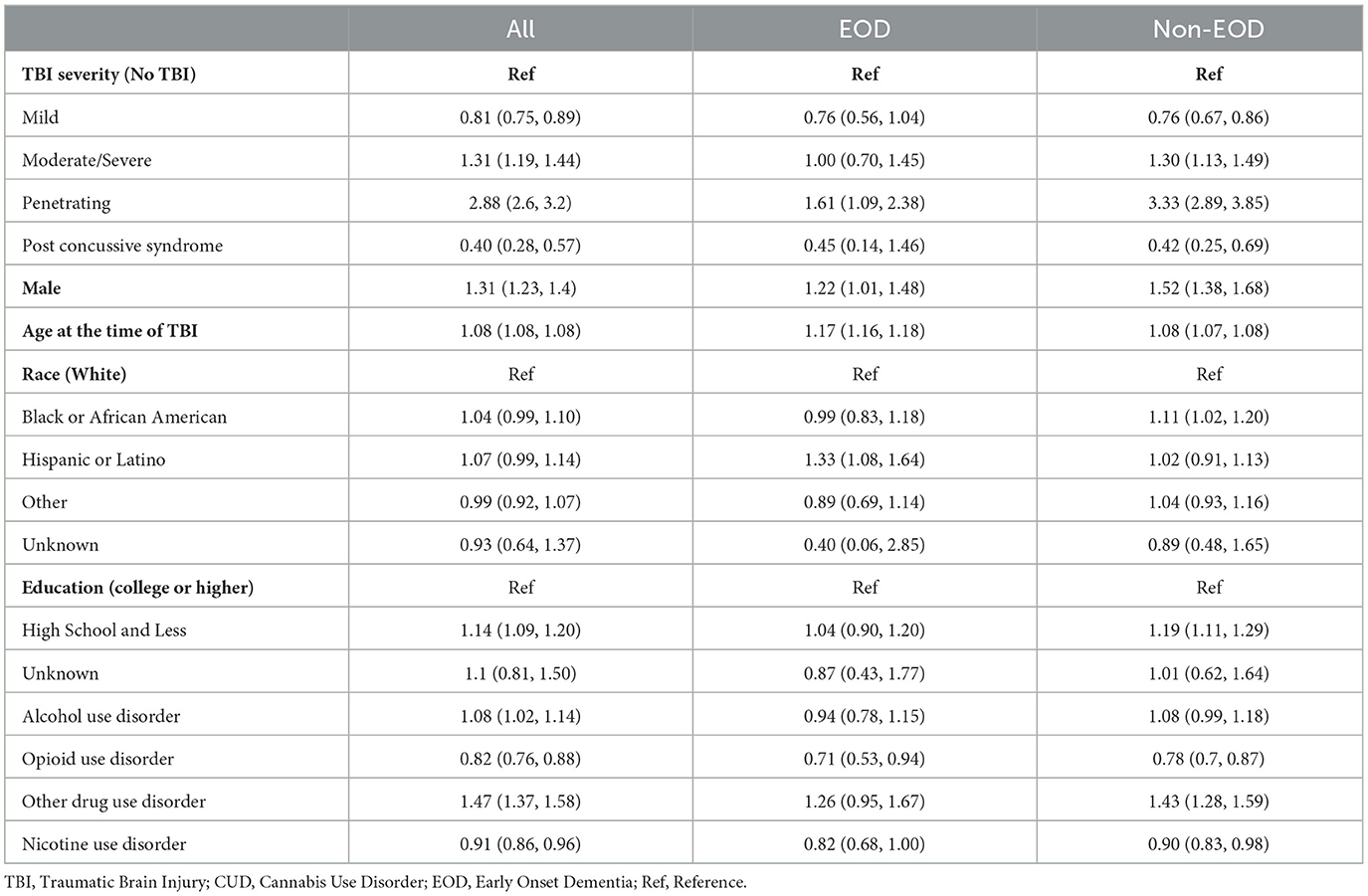
Table 3. The impact of selected demographic and clinical characteristics on cognitive disorder (adjusted cox proportional hazards model) among veterans with TBI (N = 1,554,319).
Discussion
Among a large cohort of Post-9/11 Veterans, incidence rates of cognitive disorder were highest among those with a history of TBI and concomitant CUD followed by those with TBI only, CUD only, and those without a history of TBI or CUD. Veterans with CUD + TBI had a 3.26 times higher hazard for cognitive disorder compared with those in the control group. Prior studies have established the association between TBI and dementia (1, 50) and potential mechanisms linking the two conditions (14, 51, 52). As expected, Veterans with TBI only had a 2.32 times higher hazard for cognitive disorder compared with those in the control group. While we are not able to assess a dose-response association between CUD and cognitive disorder, we found a higher hazard of cognitive disorder in those with CUD only and CUD+TBI, compared with the control group. Depending on the type and severity, TBI may be exhibited by focal brain damage causing 'shearing and stretching' injuries in cerebral brain tissues (53, 54) or diffuse axonal injury that may involve subcortical and deeper white matter tissues such as the brainstem and corpus callosum (55). Conversely, the distribution of cannabinoids in the brain, regardless of the intake route, occurs after modifying the deleterious effects on the blood–brain barrier (56). Although the brain's blood supply originates in the base of the skull (the brainstem, amygdala, and hypothalamus) and terminates in the cortical area, a previous study demonstrated that cannabis users exhibited significantly increased blood volumes in the frontal, temporal, and cerebellar areas (57).
While our finding is consistent with a previous study indicating higher risk of EOD in Veterans with TBI (16), our data also suggests that CUD is an independent risk factor for cognitive disorder only in the non-EOD group. Compared with the control group, the CUD-only group exhibited a 79% higher hazard for cognitive disorders, primarily driven by the non-EOD subgroup (excluding AD and FTD). A previous systematic review of brain imaging studies among adolescent cannabis users revealed functional and structural evidence of lesions in the frontoparietal, frontolimbic, frontostriatal, and cerebellum regions (58). The results are also consistent with previous studies demonstrating that cannabis use is associated with cognitive functional disorder and bilateral hippocampal and amygdala volume reduction in midlife patients with heavy, chronic cannabis use (40, 45). Our findings indicate AD and FTD are less likely to be observed in the CUD patients, which may be explained by intact cortical areas in cannabis users but need further investigation. Since AD is characteristically a disease of older age (59) and those who were older tended to have lower cannabis quantity use and fewer consequences associated with cannabis use (60, 61), other considerations include possible age-related behavioral changes among those with CUD. Additional factors not measured in these analyses, including social, structural, and biological characteristics, may contribute to dementia susceptibility in Veterans with non-EOD and co-occurring CUD.
The hazard ratio for dementia diagnosis across all categories was more pronounced in individuals with non-EOD, compared with EOD, which could be explained by genetic risk factors and the physiopathology of TBI and CUD in the progression of dementia. The genetic risk factors may pave the way to approach the hypothesis behind the dissimilarity in our EOD vs. non-EOD results. Previous studies addressed the potential roles of several missense mutations and known variant genes in the pathogenesis of early-onset AD (62) and decreased levels of dopaminergic neurotransmitters in patients with AD (63). Conversely, distinct genetic factors for CUD might concurrently initiate underlying pathways related to AD (64). Cannabinoids have been shown to increase mesolimbic dopamine transmission in the short term (65). The risk factors underlying CUD development likely involve multiple genes that interact with each other and the environment, ultimately leading to cognitive disorders. TBI is defined as an impact, penetration, or rapid movement of the brain within the skull and the event can be classified as either impact (direct contact of the head with an object) or non-impact (encountering non-impact forces like blast waves or rapid acceleration and deceleration) (66).
Limitations
This study has several limitations. The results were restricted to Veterans and based on characteristics and conditions measured and stored in electronic health records (EHRs). Therefore, they may not represent other patient populations. We attempted to account for the difficulties associated with obtaining chronicity and severity of cannabis use by examining both DoD and VA records and limiting cannabis exposures to ICD codes related to CUD. The EHR system in VHA allowed us to identify a CUD diagnosis after the TBI index date, further strengthening the methodology. However, we note that we were not able to quantify the route of cannabis intake (i.e., inhalation vs. ingestion), which is an area for further exploration. While our approach focused on cognitive disorders due to inaccuracy of dementia codes in younger individuals, further analysis is warranted in other older cohorts, and subsequent analyses as this longitudinal cohort ages.
Conclusions
The results of our study suggest that CUD and TBI are independent risk factors for cognitive disorder and the highest incidence of cognitive disorder is observed in Veterans with comorbid CUD+TBI. TBI and CUD are both independently associated with cognitive impairment. Cognitive impairment is a common post-TBI symptom that may last more than 6 months post-injury (8, 9). Acute inhaled cannabis use is associated with cognitive impairment that may last at least 5 h (38). However, the timing of cognitive disorders is the key point in our study (i.e., time from TBI to ICD codes for dementia diagnosis) and likely indicates permanent cognitive dysfunction after TBI insult. The heterogeneity in impact of CUD on emergence of EOD and Non-EOD subgroup in our cohort, who were relatively young at the time of TBI, may be indicative of the potential harms of cannabis use on long-term cognitive dysfunction. Given that cannabis receptor (CB1R) is enriched in the mesocorticolimbic system (67) and cannabis exposure increases long-term vulnerability to cognitive impairments (68, 69), our results support the long-term harmful effect of cannabis use in patients with cognitive disorder and dementia subtypes that involved brain areas other than frontal and temporal lobes (AD and FTD). Cannabis users showed that the cerebral blood flow reduced in cortical regions and increased in the right precuneus at baseline (70). Also, in the experimental animal's study, noxious effects of chronic cannabis exposure led to higher THC and cannabidiol concentrations in cerebellum and occipital cortex of squirrel monkeys and persisted after discontinuation of the treatment (71). Further studies is needed to evaluate the impact of the chronic cannabis use and structural changes in medial temporal structures and midbrain (40–45). Given the findings of this analysis and the increasing awareness of the potential long-term impacts of combat-related and civilian TBI and the growing rates of CUD, further investigations are warranted.
Data availability statement
The datasets presented in this article are not readily available because VA regulation required the dataset behind the firewall. Requests to access the datasets should be directed to vinci@va.gov.
Ethics statement
The studies involving humans were approved by the University of Utah and Stanford University. The studies were conducted in accordance with the local legislation and institutional requirements. Written informed consent for participation was not required from the participants or the participants' legal guardians/next of kin in accordance with the national legislation and institutional requirements.
Author contributions
AE: Conceptualization, Data curation, Formal analysis, Funding acquisition, Investigation, Methodology, Resources, Software, Validation, Visualization, Writing – original draft, Writing – review & editing. CD-G: Conceptualization, Funding acquisition, Investigation, Methodology, Supervision, Writing – review & editing. TP: Investigation, Methodology, Writing – review & editing. MA: Data curation, Software, Writing – review & editing. CG: Project administration, Resources, Writing – review & editing. AD: Investigation, Writing – review & editing. MM: Project administration, Writing – review & editing. EK: Writing – review & editing, Data curation, Methodology, Validation. DC: Conceptualization, Funding acquisition, Investigation, Supervision, Writing – review & editing. MP: Conceptualization, Funding acquisition, Investigation, Methodology, Supervision, Validation, Writing – review & editing.
Funding
The author(s) declare financial support was received for the research, authorship, and/or publication of this article. This work was supported by the Assistant Secretary of Defense for Health Affairs endorsed by the Department of Defense, through the Psychological Health/Traumatic Brain Injury Research Program Long-Term Impact of Military-Relevant Brain Injury Consortium (LIMBIC) Award/W81XWH-18-PH/TBIRP-LIMBIC under awards no. W81XWH1920067 and W81XWH-13-2-0095 and by the U.S. Department of Veterans Affairs awards no. I01 CX002097, I01 CX002096, I01 HX003155, I01 RX003444, I01 RX003443, I01 RX003442, I01 CX001135, I01 CX001246, I01 RX001774, I01 RX 001135, I01 RX 002076, I01 RX 001880, I01 RX 002172, I01 RX 002173, I01 RX 002171, I01 RX 002174, and I01 RX 002170. The U.S. Army Medical Research Acquisition Activity, 839 Chandler Street, Fort Detrick, MD 21702-5014 is the awarding and administering acquisition office. The work is also supported by the Veterans Health Administration (VHA), Office of Research and Development (VA-ORD), Research Supplements to Promote Diversity, Award # SDR 20-030 and Research Career Scientist Award (1 IK6 HX002608 RCS 17-297). Effort for this project was also supported by Health Economics Resource Center (HERC), Ci2i, VA Palo Alto Health Care System, the Informatics, Decision-Enhancement, and Analytic Sciences (IDEAS) Center of Innovation (CIN 13-414) and HSR&D, US Department of Veterans Affairs.
Conflict of interest
The authors declare that the research was conducted in the absence of any commercial or financial relationships that could be construed as a potential conflict of interest.
Publisher's note
All claims expressed in this article are solely those of the authors and do not necessarily represent those of their affiliated organizations, or those of the publisher, the editors and the reviewers. Any product that may be evaluated in this article, or claim that may be made by its manufacturer, is not guaranteed or endorsed by the publisher.
Author disclaimer
Opinions, interpretations, conclusions, and recommendations are those of the author and are not necessarily endorsed by the Department of Defense. Any opinions, findings, conclusions, or recommendations expressed in this publication are those of the author(s) and do not necessarily reflect the views of the U.S. Government, or the U.S. Department of Veterans Affairs, and no official endorsement should be inferred. The views, opinions, and or/findings contained in this abstract are those of the authors and should not be construed as an official Veterans Affairs or Department of Defense position, policy or decision, unless so designated by other official documentation. The content is solely the responsibility of the authors.
Supplementary material
The Supplementary Material for this article can be found online at: https://www.frontiersin.org/articles/10.3389/fneur.2024.1261249/full#supplementary-material
References
1. Barnes DE, Byers AL, Gardner RC, Seal KH, Boscardin WJ, Yaffe K, et al. Association of mild traumatic brain injury with and without loss of consciousness with dementia in US military veterans. JAMA Neurol. (2018) 75:1055–61. doi: 10.1001/jamaneurol.2018.0815
2. Harvey PD. Domains of cognition and their assessment. Dialog Clin Neurosci. (2019) 21:227–37. doi: 10.31887/DCNS.2019.21.3/pharvey
3. Kramarow EA, Tejada-Vera B. Dementia mortality in the United States, 2000-2017. Natl Vital Stat Rep. (2019) 68:1–29. Available online at: https://www.cdc.gov/nchs/products/nvsr.htm
4. Barnes DE, Kaup A, Kirby KA, Byers AL, Diaz-Arrastia R, Yaffe K, et al. Traumatic brain injury and risk of dementia in older veterans. Neurology. (2014) 83:312–9. doi: 10.1212/WNL.0000000000000616
5. Raza Z, Hussain SF, Ftouni S, Spitz G, Caplin N, Foster RG, et al. Dementia in military and veteran populations: a review of risk factors-traumatic brain injury, post-traumatic stress disorder, deployment, and sleep. Mil Med Res. (2021) 8:55. doi: 10.1186/s40779-021-00346-z
6. Warden D. Military TBI during the Iraq and Afghanistan wars. J Head Trauma Rehabil. (2006) 21:398–402. doi: 10.1097/00001199-200609000-00004
7. Trexler LE, Corrigan JD, Dave S, Hammond FM. Recommendations for prescribing opioids for people with traumatic brain injury. Arch Phys Med Rehabil. (2020) 101:2033–40. doi: 10.1016/j.apmr.2020.07.005
8. Ivins BJ. Hospitalization associated with traumatic brain injury in the active duty US Army: 2000-2006. NeuroRehabilitation. (2010) 26:199–212. doi: 10.3233/NRE-2010-0556
9. Meyer KS, Marion DW, Coronel H, Jaffee MS. Combat-related traumatic brain injury and its implications to military healthcare. Psychiatr Clin North Am. (2010) 33:783–96. doi: 10.1016/j.psc.2010.08.007
10. Cicerone KD, Kalmar K. Persistent postconcussion syndrome: The structure of subjective complaints after mild traumatic brain injury. J Head Trauma Rehabil. (1995) 10:1–17. doi: 10.1097/00001199-199510030-00002
11. Meterko M, Baker E, Stolzmann KL, Hendricks AM, Cicerone KD, Lew HL, et al. Psychometric assessment of the neurobehavioral symptom inventory-22: the structure of persistent postconcussive symptoms following deployment-related mild traumatic brain injury among veterans. J Head Trauma Rehabil. (2012) 27:55–62. doi: 10.1097/HTR.0b013e318230fb17
12. Cifu DX. Clinical research findings from the long-term impact of military-relevant brain injury consortium-chronic effects of neurotrauma consortium (LIMBIC-CENC) 2013-2021. Brain Inj. (2022) 36:587–97. doi: 10.1080/02699052.2022.2033843
13. Washington PM, Villapol S, Burns MP. Polypathology and dementia after brain trauma: Does brain injury trigger distinct neurodegenerative diseases, or should they be classified together as traumatic encephalopathy? Exp Neurol 275 Pt 3. (2016) 381–8. doi: 10.1016/j.expneurol.2015.06.015
14. Graham NS, Sharp DJ. Understanding neurodegeneration after traumatic brain injury: from mechanisms to clinical trials in dementia. J Neurol Neurosurg Psychiatry. (2019) 90:1221–33. doi: 10.1136/jnnp-2017-317557
15. Nemetz PN, Leibson C, Naessens JM, Beard M, Kokmen E, Annegers JF, et al. Traumatic brain injury and time to onset of Alzheimer's disease: a population-based study. Am J Epidemiol. (1999) 149:32–40. doi: 10.1093/oxfordjournals.aje.a009724
16. Kennedy E, Panahi S, Stewart IJ, Tate DF, Wilde EA, Kenney K, et al. Traumatic brain injury and early onset dementia in post 9-11 veterans. Brain Inj. (2022) 36:620–7. doi: 10.1080/02699052.2022.2033846
17. Greydanus DE, Hawver EK, Greydanus MM, Merrick J. Marijuana: current concepts(dagger). Front Public Health. (2013) 1:42. doi: 10.3389/fpubh.2013.00042
18. Food and Drug Administration. FDA and Cannabis: Research and Drug Approval Process. Silver Spring, MD: Food and Drug Administration (2023)
19. American Psychiatric Association. Diagnostic and Statistical Manual of Mental Disorders, 5th Edition (DSM-5). Washington, DC: American Psychiatric Association (2013).
20. Gordon AJ, Conley JW, Gordon JM. Medical consequences of marijuana use: a review of current literature. Curr Psychiatry Rep. (2013) 15:419. doi: 10.1007/s11920-013-0419-7
21. Zehra A, Burns J, Liu CK, Manza P, Wiers CE, Volkow ND, et al. Cannabis addiction and the brain: a review. J Neuroimmune Pharmacol. (2018) 13:438–52. doi: 10.1007/s11481-018-9782-9
22. Sexton M, Cuttler C, Finnell JS, Mischley LK. A.cross-sectional survey of medical cannabis users: patterns of use and perceived efficacy. Cannabis Cannabinoid Res. (2016) 1:131–8. doi: 10.1089/can.2016.0007
23. Grenier K, Ponnambalam F, Lee D, Lauwers R, Bhalerao S. Cannabis in the treatment of traumatic brain injury: a primer for clinicians. Can J Neurol Sci. (2020) 47:11–7. doi: 10.1017/cjn.2019.298
24. Hergert DC, Robertson-Benta C, Sicard V, Schwotzer D, Hutchison K, Covey DP, et al. Use of medical cannabis to treat traumatic brain injury. J Neurotrauma. (2021) 38:1904–17. doi: 10.1089/neu.2020.7148
25. Utter B, Anderson CA, Filley CM, Kelly JP, Johnston-Brooks C, Arciniegas DB, et al. Cannabis use in a cohort of healthcare-seeking united states military veterans with persisting symptoms after mild traumatic brain injury: preliminary observations. Mil Med. (2022) 188:e2158–e2156. doi: 10.1093/milmed/usac011
26. Carliner H, Brown QL, Sarvet AL, Hasin DS. Cannabis use attitudes, and legal status in the US: A review. Prev Med. (2017) 104:13–23. doi: 10.1016/j.ypmed.2017.07.008
27. Hawley LA, Ketchum JM, Morey C, Collins K, Charlifue S. Cannabis use in individuals with spinal cord injury or moderate to severe traumatic brain injury in Colorado. Arch Phys Med Rehabil. (2018) 99:1584–90. doi: 10.1016/j.apmr.2018.02.003
28. Compton WM, Han B, Jones CM, Blanco C, Hughes A. Marijuana use and use disorders in adults in the USA, 2002-14: analysis of annual cross-sectional surveys. Lancet Psychiatry. (2016) 3:954–64. doi: 10.1016/S2215-0366(16)30208-5
29. Hill ML, Loflin M, Nichter B, Norman SB, Pietrzak RH. Prevalence of cannabis use, disorder, and medical card possession in US military veterans: results from the 2019–2020 national health and resilience in veterans study. Addict Behav. (2021) 120:106963. doi: 10.1016/j.addbeh.2021.106963
30. Browne KC, Stohl M, Bohnert KM, Saxon AJ, Fink DS, Olfson M, et al. Prevalence and correlates of cannabis use and cannabis use disorder among US veterans: results from the national epidemiologic survey on alcohol and related conditions (NESARC-III). Am J Psychiatry. (2022) 179:26–35. doi: 10.1176/appi.ajp.2021.20081202
31. Hasin DS, Saxon AJ, Malte C, Olfson M, Keyes KM, Gradus JL, et al. Trends in cannabis use disorder diagnoses in the US veterans health administration, 2005–2019. Am J Psychiatry. (2022) 179:748–57. doi: 10.1176/appi.ajp.22010034
32. Yockey RA, Hoopsick RA. National trends in past-year Marijuana use among veterans in the United States, 2013-2019. Subst Use Misuse. (2023) 58:822–7. doi: 10.1080/10826084.2023.2191700
33. Eubanks LM, Rogers CJ, Beuscher AET, Koob GF, Olson AJ, Dickerson TJ, et al. A molecular link between the active component of marijuana and Alzheimer's disease pathology. Mol Pharm. (2006) 3:773–7. doi: 10.1021/mp060066m
34. Broers B, Pata Z, Mina A, Wampfler J, Saussure D, Pautex C, et al. Prescription of a THC/CBD-based medication to patients with dementia: a pilot study in Geneva. Med Cannabis Cannabinoids. (2019) 2:56–9. doi: 10.1159/000498924
35. Hillen JB, Soulsby N, Alderman C, Caughey GE. Safety and effectiveness of cannabinoids for the treatment of neuropsychiatric symptoms in dementia: a systematic review. Ther Adv Drug Saf. (2019) 10:2042098619846993. doi: 10.1177/2042098619846993
36. Krishnan S, Cairns R, Howard R. Cannabinoids for the treatment of dementia. Cochr Datab Syst Rev. (2009) 2:1–14 doi: 10.1002/14651858.CD007204.pub2
37. Peprah K, McCormack S. Medical Cannabis for the Treatment of Dementia: A Review of Clinical Effectiveness and Guidelines. Ottawa, ON: Canadian Agency for Drugs and Technologies in Health (2019).
38. McCartney D, Arkell TR, Irwin C, Mcgregor IS. Determining the magnitude and duration of acute Delta(9)-tetrahydrocannabinol (Delta(9)-THC)-induced driving and cognitive impairment: a systematic and meta-analytic review. Neurosci Biobehav Rev. (2021) 126:175–93. doi: 10.1016/j.neubiorev.2021.01.003
39. Ramaekers JG, Mason NL, Kloft L, Theunissen EL. The why behind the high: determinants of neurocognition during acute cannabis exposure. Nat Rev Neurosci. (2021) 22:439–54. doi: 10.1038/s41583-021-00466-4
40. Yucel M, Solowij N, Respondek C, Whittle S, Fornito A, Pantelis C, et al. Regional brain abnormalities associated with long-term heavy cannabis use. Arch Gen Psychiatry. (2008) 65:694–701. doi: 10.1001/archpsyc.65.6.694
41. Ashtari M, Avants B, Cyckowski L, Cervellione KL, Roofeh D, Cook P, et al. Medial temporal structures and memory functions in adolescents with heavy cannabis use. J Psychiatr Res. (2011) 45:1055–66. doi: 10.1016/j.jpsychires.2011.01.004
42. Demirakca T, Sartorius A, Ende G, Meyer N, Welzel H, Skopp G, et al. Diminished gray matter in the hippocampus of cannabis users: possible protective effects of cannabidiol. Drug Alcohol Depend. (2011) 114:242–5. doi: 10.1016/j.drugalcdep.2010.09.020
43. Batalla A, Soriano-Mas C, López-Solà M, Torrens M, Crippa JA, Bhattacharyya S, et al. Modulation of brain structure by catechol-O-methyltransferase Val158Met polymorphism in chronic cannabis users. Addict Biol. (2014) 19:722–32. doi: 10.1111/adb.12027
44. Filbey FM, Aslan S, Calhoun VD, Spence JS, Damaraju E, Caprihan A, et al. Long-term effects of marijuana use on the brain. Proc Natl Acad Sci U S A. (2014) 111:16913–8. doi: 10.1073/pnas.1415297111
45. Meier MH, Caspi AR, Knodt A, Hall W, Ambler A, Harrington H, et al. Long-term cannabis use and cognitive reserves and hippocampal volume in midlife. Am J Psychiatr. (2022) 179:362–74. doi: 10.1176/appi.ajp.2021.21060664
46. Stewart IJ, Amuan ME, Wang CP, Kennedy E, Kenney K, Werner JK, et al. Association between traumatic brain injury and subsequent cardiovascular disease among post-9/11-era veterans. JAMA Neurol. (2022) 79:1122–9. doi: 10.1001/jamaneurol.2022.2682
47. Military Health System. Armed Forces Health Surveillance Branch. Falls Church, VA: Military Health System (2019).
48. Salem LC, Andersen BB, Nielsen TR, Stokholm J, Jorgensen MB, Waldemar G, et al. Inadequate diagnostic evaluation in young patients registered with a diagnosis of dementia: a nationwide register-based study. Dement Geriatr Cogn Dis Extra. (2014) 4:31–44. doi: 10.1159/000358050
49. Marceaux JC, Soble JR, O'Rourke JJ, Swan AA, Wells M, Amuan M, et al. Validity of early-onset dementia diagnoses in VA electronic medical record administrative data. Clin Neuropsychol. (2020) 34:1175–89. doi: 10.1080/13854046.2019.1679889
50. Tolppanen AM, Taipale H, Hartikainen S. Head or brain injuries and Alzheimer's disease: a nested case-control register study. Alzheimers Dement. (2017) 13:1371–9. doi: 10.1016/j.jalz.2017.04.010
51. Wang HK, Lin SH, Sung PS, Wu MH, Hung KW, Wang LC, et al. Population based study on patients with traumatic brain injury suggests increased risk of dementia. J Neurol Neurosurg Psychiatry. (2012) 83:1080–5. doi: 10.1136/jnnp-2012-302633
52. Mendez MF. What is the relationship of traumatic brain injury to dementia? J Alzheimers Dis. (2017) 57:667–81. doi: 10.3233/JAD-161002
53. Schmidt OI, Infanger M, Heyde CE, Ertel W, Stahel PF. The role of neuroinflammation in traumatic brain injury. Eur J Trauma. (2004) 30:135–49. doi: 10.1007/s00068-004-1394-9
54. Ng SY, Lee AYW. Traumatic brain injuries: pathophysiology and potential therapeutic targets. Front Cell Neurosci. (2019) 13:528. doi: 10.3389/fncel.2019.00528
55. Smith DH, Meaney DF, Shull WH. Diffuse axonal injury in head trauma. J Head Trauma Rehabil. (2003) 18:307–16. doi: 10.1097/00001199-200307000-00003
56. Calapai F, Cardia L, Sorbara EE, Navarra M, Gangemi S, Calapai G, et al. Cannabinoids, blood-brain barrier, and brain disposition. Pharmaceutics. (2020) 12, 265. doi: 10.3390/pharmaceutics12030265
57. Sneider JT, Pope Jr HG, Silveri MM, Simpson NS, Gruber SA, Yurgelun-Todd DA. Altered regional blood volume in chronic cannabis smokers. Exp Clin Psychopharmacol. (2006) 14:422. doi: 10.1037/1064-1297.14.4.422
58. Lichenstein SD, Manco N, Cope LM, Egbo L, Garrison KA, Hardee J, et al. Systematic review of structural and functional neuroimaging studies of cannabis use in adolescence and emerging adulthood: evidence from 90 studies and 9441 participants. Neuropsychopharmacology. (2022) 47:1000–28. doi: 10.1038/s41386-021-01226-9
59. Braak H, Braak E. Frequency of stages of Alzheimer-related lesions in different age categories. Neurobiol Aging. (1997) 18:351–7. doi: 10.1016/S0197-4580(97)00056-0
60. Haug NA, Padula CB, Sottile JE, Vandrey R, Heinz AJ, Bonn-Miller MO, et al. Cannabis use patterns and motives: a comparison of younger, middle-aged, and older medical cannabis dispensary patients. Addict Behav. (2017) 72:14–20. doi: 10.1016/j.addbeh.2017.03.006
61. Zeiger JS, Silvers WS, Fleegler EM, Zeiger RS. Age related differences in cannabis use and subjective effects in a large population-based survey of adult athletes. J Cannabis Res. (2019) 1:7. doi: 10.1186/s42238-019-0006-9
62. Giau VV, Bagyinszky E, Yang YS, Youn YC, An SSA, Kim SY, et al. Genetic analyses of early-onset Alzheimer's disease using next generation sequencing. Sci Rep. (2019) 9:8368. doi: 10.1038/s41598-019-44848-2
63. Pan X, Kaminga AC, Wen SW, Wu X, Acheampong K, Liu A, et al. Dopamine and dopamine receptors in Alzheimer's disease: a systematic review and network meta-analysis. Front Aging Neurosci. (2019) 11:175. doi: 10.3389/fnagi.2019.00175
64. Peng Q, Wilhelmsen KC, Ehlers CL. Pleiotropic loci for cannabis use disorder severity in multi-ancestry high-risk populations. Mol Cell Neurosci. (2023) 125:103852. doi: 10.1016/j.mcn.2023.103852
65. Oleson EB, Cheer JF. A brain on cannabinoids: the role of dopamine release in reward seeking. Cold Spring Harb Perspect Med. (2012) 2:229. doi: 10.1101/cshperspect.a012229
66. Prins M, Greco T, Alexander D, Giza CC. The pathophysiology of traumatic brain injury at a glance. Dis Model Mech. (2013) 6:1307–15. doi: 10.1242/dmm.011585
67. Herkenham M, Lynn AB, Little MD, Johnson MR, Melvin LS, Costa D, et al. Cannabinoid receptor localization in brain. Proc Natl Acad Sci U S A. (1990) 87:1932–6. doi: 10.1073/pnas.87.5.1932
68. Rubino T, Parolaro D. Long lasting consequences of cannabis exposure in adolescence. Mol Cell Endocrinol. (2008) 286:S108–113. doi: 10.1016/j.mce.2008.02.003
69. Krebs MO, Kebir O, Jay TM. Exposure to cannabinoids can lead to persistent cognitive and psychiatric disorders. Eur J Pain. (2019) 23:1225–33. doi: 10.1002/ejp.1377
70. Jacobus J, Goldenberg D, Wierenga CE, Tolentino NJ, Liu TT, Tapert SF, et al. Altered cerebral blood flow and neurocognitive correlates in adolescent cannabis users. Psychopharmacology. (2012) 222:675–84. doi: 10.1007/s00213-012-2674-4
Keywords: traumatic brain injury, cannabis use disorder, dementia, Veterans, Cox proportional hazards model
Citation: Esmaeili A, Dismuke-Greer C, Pogoda TK, Amuan ME, Garcia C, Del Negro A, Myers M, Kennedy E, Cifu D and Pugh MJ (2024) Cannabis use disorder contributes to cognitive dysfunction in Veterans with traumatic brain injury. Front. Neurol. 15:1261249. doi: 10.3389/fneur.2024.1261249
Received: 31 October 2023; Accepted: 03 January 2024;
Published: 16 January 2024.
Edited by:
Gustavo C. Roman, Houston Methodist Research Institute, United StatesReviewed by:
Rebecca J. Henry, University College Cork, IrelandHuajun Liang, University of Maryland, United States
Copyright © 2024 Esmaeili, Dismuke-Greer, Pogoda, Amuan, Garcia, Del Negro, Myers, Kennedy, Cifu and Pugh. This is an open-access article distributed under the terms of the Creative Commons Attribution License (CC BY). The use, distribution or reproduction in other forums is permitted, provided the original author(s) and the copyright owner(s) are credited and that the original publication in this journal is cited, in accordance with accepted academic practice. No use, distribution or reproduction is permitted which does not comply with these terms.
*Correspondence: Aryan Esmaeili, aryan.esmaeili@va.gov