- 1Department of Neurology, Section of Interventional Neurology, Texas Tech University Health Sciences Center El Paso, El Paso, TX, United States
- 2Ziauddin University, Karachi, Sindh, Pakistan
- 3University of Texas Health Science Center at Houston, Houston, TX, United States
As the majority of large vessel occlusion (LVO) patients are not treated with revascularization therapies or efficiently revascularized, complementary management strategies are needed. In this article we explore the importance of cerebral autoregulation (CA) assessment in the prediction and/or modification of infarct growth and hemorrhagic transformation. In patients with LVO, these are important factors that affect prognosis. A systematic search of the PubMed, EMBASE databases and a targeted Google search was conducted, resulting in the inclusion of 34 relevant articles. There is an agreement that CA is impaired in patients with LVO; several factors have been identified such as time course, revascularization status, laterality, disease subtype and location, some of which may be potentially modifiable and affect outcomes. The personalized CA assessment of these patients suggests potential for better understanding of the inter-individual variability. Further research is needed for the development of more accurate, noninvasive techniques for continuous monitoring and personalized thresholds for CA.
Introduction
Cerebral Autoregulation (CA) is the intrinsic capacity of the vasculature in the brain to maintain constant blood flow despite variations in systemic blood pressure (1). This phenomenon is mediated by myogenic and neurogenic processes (via neurotransmitters) and driven by changes in cerebral metabolism, partial pressure of oxygen (PaO2), or carbon dioxide (PaCO2), temperature, and intracranial pressure among others (See Figure 1). There is impaired CA in all types of acute ischemic stroke (AIS) patients, and it likely leads to worse clinical outcomes. Failure of CA is associated with secondary brain injury that may occur as an extension of the initial ischemic core, “no-reflow phenomenon” (3), cerebral edema, or hemorrhagic transformation.
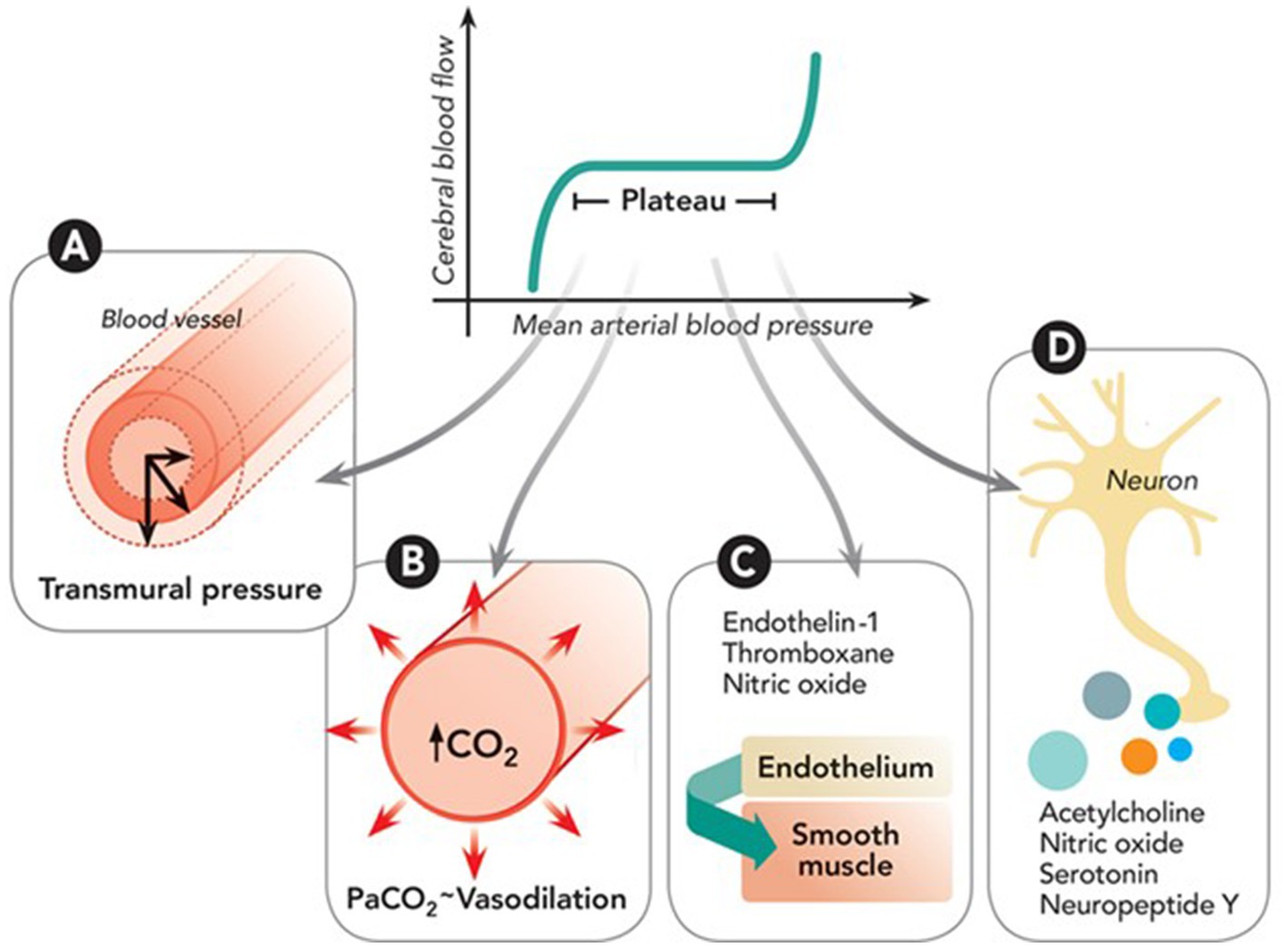
Figure 1. Depiction of cerebral autoregulation processes: (A) Myogenic response adjusts arterial size based on transmural pressure via muscle activity. (B) Metabolic response modulates small artery size based on carbon dioxide (CO₂) levels from oxidative phosphorylation. (C) Endothelial response involves secretion of agents like nitric oxide and endothelin-1 that act on smooth muscle. (D) Neurogenic response involves neuroglial cells regulating vessel diameter through various vasoactive neurotransmitters. Reproduced with permission from the publisher Walters Kluwer Health, Inc., for the original article by Rivera-Lara et al. (2).
Large vessel occlusion (LVO) accounts for approximately 30 to 40% of all acute ischemic stroke (AIS) cases. Currently, only 10–20% of patients with LVO undergo endovascular treatment. Out of those patients that receive endovascular treatment, effective recanalization is achieved in about 70% of the cases, yet only approximately 46% will have an independent outcome at 90 days (4). The importance of optimizing CA in these patients during different phases of AIS including during intensive care has not yet been well established.
Optimization of CA in LVO patients could potentially have a significant impact on their clinical outcome. We hypothesize that patients that are most likely to benefit include those that are awaiting to undergo endovascular treatment, those that were treated but did not have effective recanalization and those patients that lack adequate arterial collaterals. While there are reviews about CA in the general acute ischemic stroke population, there are no specific comprehensive in-depth analyses and/or literature reviews regarding CA in patients with LVO.
Methods
PubMed and EMBASE databases were searched on October 06, 2022, and October 11, 2022, respectively. Stroke, Brain or Cerebrum or Cerebrovascular Circulation, Homeostasis, Procedures or Thrombectomy or Mechanical Thrombolysis or Thrombolytic Therapy or Tissue Plasminogen Activator were selected as relevant Medical Subject Headings (MeSH) and combined using the ‘AND’ clause. All MeSH terms were exploded. All keywords from the PubMed MeSH library were included along with associated MeSH terms using the ‘OR’ clause, except for expired keywords or keywords that had a comma within the term. Additional field-appropriate keywords were added to the final search to expand the query, specifically “large vessel occlusion” and its variants, “acute ischemic stroke” and its variants, “dysautoregulation,” and “cerebral.” The same search was conducted on EMBASE with associated EMTREE terms. All keywords were matched to their synonyms and additional synonyms were included when identified on EMBASE database. The final searches are included as supplemental document (Appendix I). We selected only original research articles written in the English language and excluded non-human subject research, case reports, case series of fewer than five subjects, abstracts, book chapters, studies that did not measure CA, and reviews including commentaries, editorials, short surveys, and letters. Additional targeted Google search was conducted based on topics identified through the primary search from PubMed and EMBASE. Specifically, we identified recent papers studying the effects of perioperative BP management, anesthesia type during endovascular thrombectomy, and PaCO2/ETCO2 levels on CA.
Results
The PubMed and EMBASE search strategy yielded 368 studies after duplicates were removed. Of these, 366 articles were accessible and assessed for eligibility, while two could not be retrieved. Among the accessible articles, 17 met the inclusion and exclusion criteria. In addition, the targeted Google search added 17 more qualifying articles, resulting in a total of 34 articles for our review (Figure 2). We reported the results in a discussion format under different relevant subheadings.
Cerebral autoregulation monitoring
An important obstacle in the bedside monitoring of CA is the lack of a reliable and reproducible method, a gold standard. It is recommended that for appropriate CA assessment, Cerebral blood flow (CBF), systemic blood pressure (SBP) and partial pressure of CO2 (PaCO2) or a surrogate should be recorded, as the latter is a strong factor affecting CBF. Researchers have devised a handful of methods to assess changes in CA; these include static and dynamic methods (1). Cerebral autoregulation can be evaluated by measuring relative CBF changes in response to a steady-state change in the SBP (static method) or during the response to a rapid spontaneous change in SBP (dynamic method). Xenon CT, MRI, and PET scans are examples of static modes of obtaining CA metrics, but they are limited in their utility as they only provide a “snapshot” overview. Therefore, not only are they not optimal for continuous monitoring but also may require pharmacological induction with potential undesired effects.
Dynamic methods are either based on time or frequency analysis and utilize surrogates of CBF such as transcranial Doppler (TCD), laser Doppler flowmetry, diffuse correlation spectroscopy (DCS) and near-infrared spectroscopy (NIRS) (1) to assess the relation between CBF and SBP changes. Various linear and non-linear mathematical techniques are used to analyze this relationship. Examples of techniques utilized in the time domain include cerebrovascular resistance (CVR), cerebrovascular resistance index (CVRi), pulsatility index (PI), critical closing pressure (CrCP), rate of recovery (RoR), and autoregulatory index (ARI). See Table 1 reproduced from van Beek et al. (5).
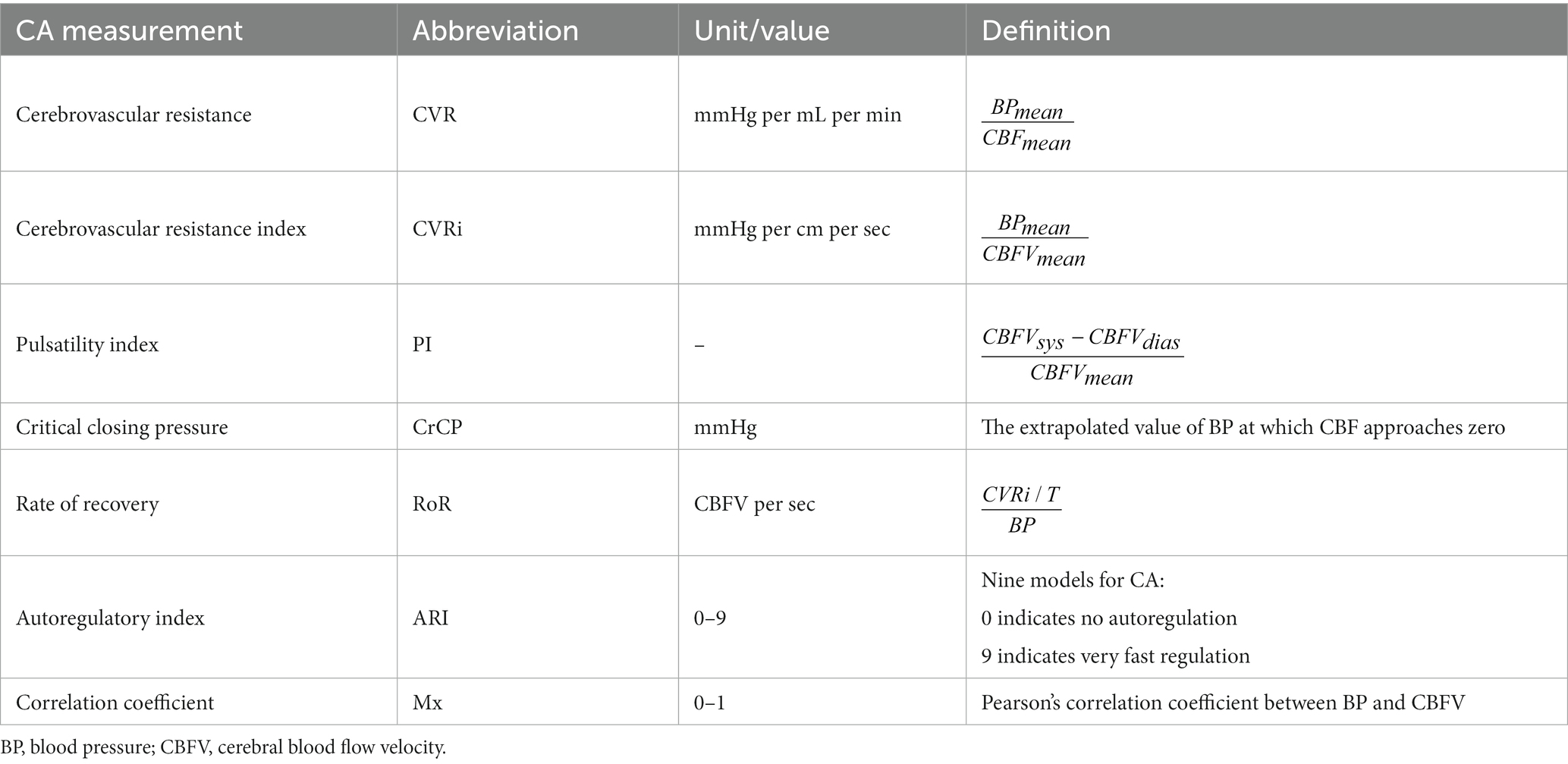
Table 1. Overview of various dynamic quantifications of cerebral autoregulation in the time domain [reproduced with permission from Van Beek et al. (5)].
In the frequency domain analysis, the regulation of CBF in response to changes in SBP can be described using transfer function analysis (TFA) which includes the following parameters:
i. Gain, which is the damping effect between input and output of the transfer function (SBP and CBF, respectively); it depicts the attenuation of cerebral blood flow velocity (CBFV) amplitude for every unit change of SBP.
ii. Phase shift, which is the time delay in transmission of SBP to CBF and is usually measured in degree radians.
iii. Coherence, which is the assessment of linearity of the relation between input and output, i.e., SBP and CBFV fluctuations, respectively. See Figure 3 reproduced from van Beek et al. (5).
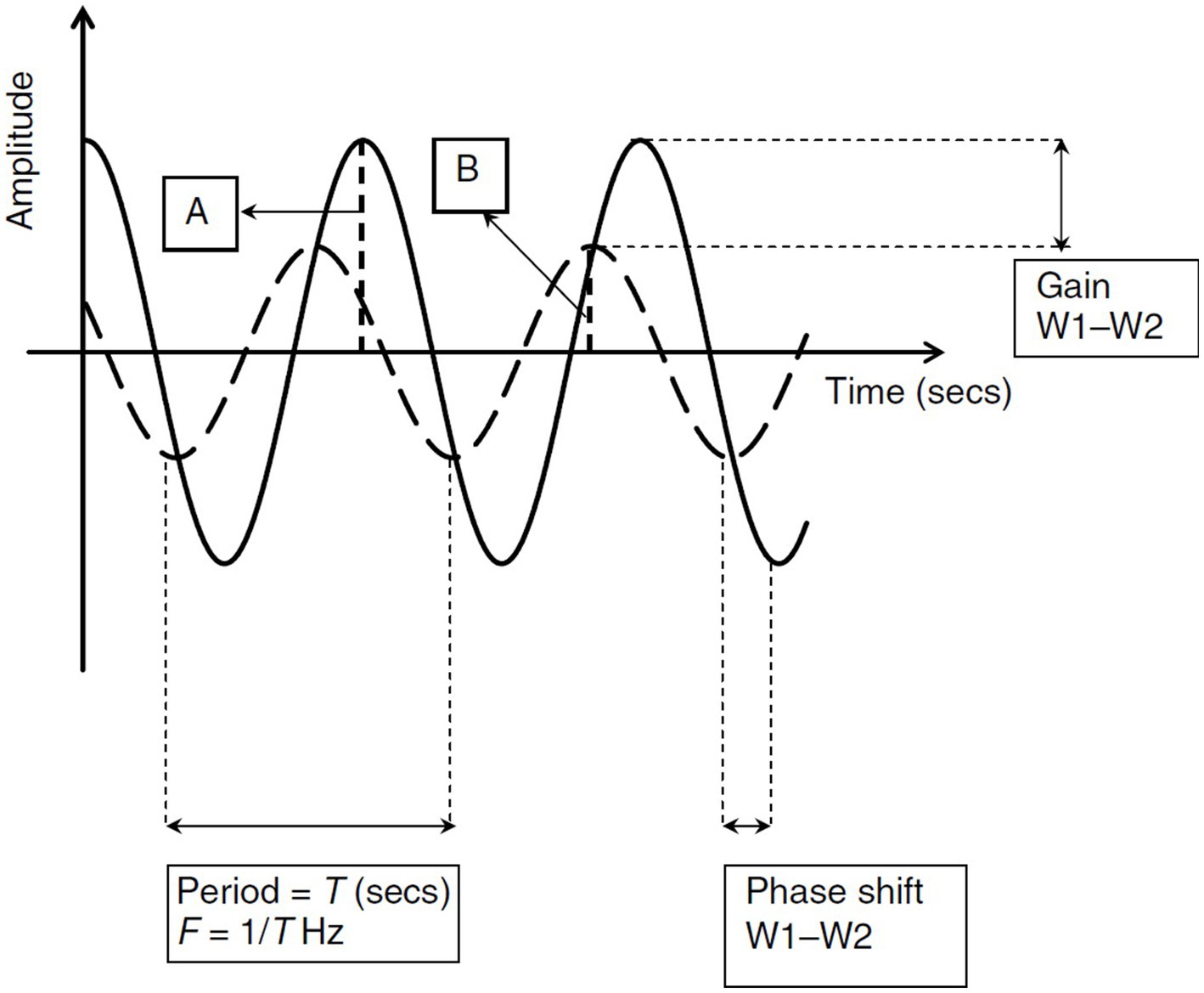
Figure 3. Analysis of Transfer Functions: This figure provides a visual interpretation of transfer function analysis using two sinusoidal waves with identical periods (T). The frequency (F) of these waveforms is determined as 1/T. (A) Illustrates Waveform 1 (W1), shown as a solid line, with its amplitude. (B) Depicts Waveform 2 (W2), indicated by a dashed line, and its amplitude. The concept of gain in this context refers to the reduction effect observed from W1 to W2, while the phase shift indicates the time lag between W1 and W2. In this scenario, W1 serves as the input and W2 as the output in the transfer function. The system’s controller reduces the amplitude of the input, resulting in a diminished amplitude (gain) in W2. The temporal displacement of W2 compared to W1 is depicted through the phase shift. Reproduced with permission from the publisher SAGE Publications for the original article by van Beek et al. (5).
While there is no universally accepted method to monitor CA, there are unique advantages and disadvantages for each modality. For instance; while TCD has good temporal resolution and provides an indicator of macrovascular autoregulation, it requires adequate temporal acoustic windows in the patient, which are lacking in 5 to 37% of the population (6, 7). In addition, the technique is highly operator-dependent. Near infrared spectroscopy (NIRS) is one common alternative to TCD; it utilizes near infrared light to estimate the relative oxyhemoglobin and deoxyhemoglobin concentrations in the cortical surface to provide an indirect marker of CBF (1). It reflects the state of autoregulation at the microvascular level and therefore serves as an indicator for superficial cortical perfusion pertaining to the vascular territory being assessed (1). Therefore, it has good temporal resolution but lacks spatial resolution. Another optical modality which utilizes scatter of red blood cells to provide a direct estimate of CBF is diffuse correlation spectroscopy (DCS) (8). This technology shares the non-invasiveness, deep tissue penetration, excellent time-resolution, and potential for continuous bedside monitoring with NIRS but was designed to overcome some of its limitations by providing a more direct and distinct measure of CBF (9). The combination of the two techniques provides a more comprehensive assessment of cerebrovascular hemodynamics, autoregulation and cerebral metabolic rate of oxygen extraction (CMR02); this combination is referred to as hybrid diffuse optical technologies (10).
The most suitable modality is therefore likely dependent on the specific condition being studied, patient specific factors and presence of other intrinsic limitations. In our opinion, two or more complementary modalities may be necessary to adequately assess CA in LVO cases.
Time course of impaired CA in AIS
Among AIS patients of different subtypes, Salinet et al. (11) demonstrated significantly lower cerebral blood flow velocity (CBFV) in the affected hemisphere within 72 h compared to a historical control, however the sample of patients studied was not limited to LVO patients (11). The CA index was mainly impaired at 2 weeks but returned to baseline at 3 months during the clinical recovery (11). In a more recent study, which included confirmed LVO patients, the CA was assessed at multiple time points (1–5 days) from symptom onset. Utilizing TCD and TFA, it was observed that CA was initially impaired and eventually a trend toward normalization was demonstrated at approximately 3–4 days (12). The degree of impairment and the rate of recovery was variable and contingent on the degree of revascularization.
In summary, impairment of CA has been documented in the acute phase (<48 h), with changes in phase shift in the ipsilateral hemisphere of subjects suffering from LVO. This gradually normalizes over time from the sub-acute (48 h-7 days) to the chronic phase when measured at 3 months (12, 13).
Laterality of impaired CA
Impaired CA is usually documented in the affected hemisphere compared to the contralateral unaffected hemisphere. In a study by Petersen et al. (14) involving LVO patients, the TFA phase shift was lower (correlating with impaired autoregulation) in the affected hemisphere compared with the unaffected hemisphere at 48 h. Interestingly, in another study measuring laser speckle imaging and MAP in patients undergoing decompressive craniectomy for malignant stroke, the degree of CA impairment was significantly greater in critically hypo perfused viable tissue (perfusion 20 to 40%) compared to the non-infarcted (perfusion 100%) or infarcted tissue (perfusion <20%) (15). According to the INFOMATAS project; dynamic CA status should be evaluated in the acute phase (under 48 h) in the affected hemisphere in LVO and /or severe stroke particularly in context of recanalization therapies (16); which should then be correlated with short and long term clinical outcomes – the grade of recommendation was “strong.” The same group of authors recommend assessment of CA in the unaffected hemisphere during the acute phase which can yield “useful” information (moderate grade) (16).
While it is worth mentioning that in LVO stroke patients CA has been noted to be impaired in the affected hemisphere consistently, interestingly the contralateral side may also show CA impairment even after recanalization of the affected side. Several mechanisms have been postulated but the exact mechanism remains unclear (17–19).
Posterior circulation CA
Several processes affecting mainly the posterior fossa have been linked to an inadequate CA; posterior reversible encephalopathy syndrome, migraine, hypertensive crises and even hypertensive intracerebral hemorrhage (20–22). The CA of the posterior circulation stemming from the vertebral arteries has not been well investigated. An earlier study in healthy human subjects suggested that perceived differences between CA in the anterior and posterior circulation were likely a representation of metabolic vasodilation related to visual cortex activation and not necessarily an inherent difference in the autoregulatory characteristics of the posterior circulation. However, the study used TCD and end tidal CO2 and appeared to test neurovascular coupling which is more and more recognized as an independent mechanism from that of CA. Although, the posterior circulation is believed to have a precarious or absent sympathetic innervation compared to the anterior circulation, an alteration in neurovascular coupling may not necessarily mean an alteration in CA (23).
In patients with severe basilar stenosis, it has been observed that when TFA phase-shift was decreased suggesting impaired CA, patients were more likely to suffer AIS with a poor 90-day functional outcome (24). Another interesting observation is that when CA was assessed in patients with pre-eclampsia using TFA phase shift, impairment was noted in the middle cerebral arteries, however given the MRI brain abnormalities noted in the posterior fossa, the authors assumed a worse CA impairment in the posterior circulation (20).
Extracranial atherosclerotic disease (anterior circulation)
Extracranial atherosclerosis such as internal carotid artery (ICA) stenosis has been well studied and been found to cause impaired CA (25–29). This failure in autoregulation is ipsilateral to the stenotic vessel and is not only a result of severe ICA stenosis (70–99%) but it has also been seen with moderate stenosis (50–69%) and can be effectively restored with revascularization (30). Many studies deducing CA in various types of stroke fail to take into account the effects of extracranial stenosis as it is a known vascular risk factor for AIS (31).
Intracranial atherosclerotic disease (ICAD)
The most common cause of AIS in the Asian, Hispanic and African population is ICAD. Although the most common presentation of ICAD is with a transient ischemic stroke or small stroke, occasionally it can present with LVO which is most likely associated with a different autoregulatory profile. While data in the setting of LVO in ICAD patients is scant, studies performed in ICAD patients in the context of secondary prevention suggest impaired CA.
ICAD has three well-described mechanisms of causing AIS: perforator artery atherothrombosis, artery-to-artery embolism and hypoperfusion; the latter mechanism is most likely to respond to endovascular therapies but also more susceptible to harm if there is collateral failure. Corroborating this view, one case control study demonstrated that in those ICAD patients with stroke related to hypoperfusion, ipsilateral CA was closely correlated with collateral status and CBF as assessed by MRI ASL (arterial spin labeling) (32). The same study showed unilateral CA impairment with perforator artery atherothrombosis, on the contrary bilateral impairment was seen with other mechanisms.
In general, studies assessing CA in ICAD are scant (33). Interestingly in asymptomatic middle cerebral artery (MCA) stenosis, CA has been demonstrated to be impaired unilaterally while symptomatic MCA stenosis was found to affect CA bilaterally (34). The degree of stenosis was directly related to CA impairment (35, 36).
Moyamoya disease
Moyamoya disease (MMD) is a rare cerebrovascular entity with progressive stenosis of the distal internal carotid arteries at the level of the ICA terminus and their proximal branches. It is more commonly seen in pediatric patients. While LVO is an uncommon presentation of MMD, there have been reports of patients requiring IV thrombolysis and/or thrombectomy (37). An earlier study demonstrated that dynamic CA was impaired in the early stages of MMD and that autoregulatory parameters are well correlated with angiographic stages of MMD (38). Indirect or direct revascularization procedures are often necessary and have variable success rates. Utilizing NIRS, one study was able to identify the lower limits of autoregulation (usually 55–65 mmHg) and optimum MAP goals (ranging from 70–90 mmHg) for pediatric patients undergoing indirect revascularization (39). Another study utilizing cerebral oximetry index (COx) and coherence from transfer function analysis (TFA) was able to predict postoperative infarction (40).
Modifiable factors affecting CA in AIS
Effect of revascularization status on CA
Intravenous (IV) thrombolytics
Agents such as tissue plasminogen activator (tPA) and Tenecteplase (TNK) have been the mainstay treatments of AIS in the early time window (41). A study by Nogueira et al. (42) using the Autoregulation index (ARI) as a surrogate for CA measured by TCD demonstrated that CA was impaired in subjects who did not respond to IV thrombolysis and had worse outcomes as assessed by NIHSS scores at 24–48 h. This was corroborated by another study which demonstrated increasingly impaired CA especially in the affected hemisphere over the first 5 days after unsuccessful IV thrombolysis (43).
Mechanical thrombectomy (MT)
When used with or without preceding IV thrombolysis, MT is now the standard of care for LVO patients presenting within the first 6 h and in select cases up to 24 h. Despite successful recanalization, some patients do not have favorable outcomes (12, 44). This might be due to reperfusion injury (45) or the ‘no-reflow phenomenon’ (3), among other factors. A multi-center study assessing dynamic CA patients after MT, showed that subjects with complete (Thrombolysis in Cerebral Infarction (TICI) 3) recanalization were associated with a more favorable autoregulation profile compared to incomplete (TICI 2b or worse) recanalization (12).
Effect of blood pressure control
Fixed targets
It has been shown that the severity of CA impairment in AIS correlates with blood pressure variations (14). A target below 180/105 mmHg in the first 24 h, during and post MT has been recommended in the American Heart Association/American Stroke Association (AHA/ASA) guidelines as an extrapolation from IV thrombolysis literature (46, 47). Previously published observational studies had shown a relationship between systemic hypertension, hemorrhagic transformation and poor outcomes post MT (48, 49). The BEST study, a large multicenter prospective study demonstrated in unadjusted analysis that a peak SBP post MT of 158 mmHg was able to discriminate good from poor functional outcomes (with higher SBP portending worse outcomes) (50, 51). The ENCHANTED 2/MT was a multicenter blinded-endpoint randomized trial which enrolled 821 patients into an intensive and a less intensive arm for BP goals post MT. The intensive arm with a BP goal <120 mmHg had a higher percentage of subjects with neurologic deterioration and mRS score(3–5) at 90 days (p < 0.001) than the less intensive treatment cohort with BP targets 140–180 mmHg (50, 52). There was no difference in rates of symptomatic ICH. The recently published BEST 2 study, demonstrated that SBP targets after endovascular therapy of less than 140 mmHg and less than 160 mmHg, did not demonstrate significantly lower infarct volumes. While it did not meet prespecified criteria for futility compared with a target of 180 mmHg or less, there was low probability of lower SBP targets demonstrating benefit in future clinical trials (53). These results highlight the limitations of fixed BP targets.
Personalized targets
Tailored blood pressure goals to the state of CA in an individual patient at a given point in time is an attractive alternative to fixed BP goal in light of the afore described RCT data. Petersen et al. (14) studied one such personalized approach post MT in patients with AIS. The authors studied different thresholds; predetermined or fixed according to the guidelines and thresholds based on perfusion status. For personalized targets, using NIRS and time-correlation analysis they deduced the mean arterial pressure (MAP) at which CA is most preserved (MAP opt). Time spent above the upper limit of autoregulation (ULA) and below the lower limit of autoregulation (LLA) was calculated for both groups; 30% of the subjects had favorable outcomes (mRS 0–2) at 3 months (14). The study showed that more time spent within personalized AL (79%) was associated with a good outcome, less hemorrhagic transformation and infarct growth compared to those with poor outcomes who spent 60% within personalized AL. Every 10% increase in time spent above ULA was associated with a 1.9-fold increase in the odds of shifting toward a worse outcome on the mRS at 90 days (14).
Effect of anesthesia type
General Anesthesia (GA) has traditionally been used for some neurointerventional procedures over conscious sedation (CS) as it lowers the risk of procedural injury, facilitates blood pressure control, protects the airway, and makes the procedure more tolerable for patients. Potential drawbacks include delays to treatment, drops in MAP/CPP and the effect of general anesthetic agents on CA. Earlier retrospective studies and meta-analyses showed that subjects exposed to GA had poorer outcomes compared to CS (54–57). These studies had certain limitations including being retrospective and not taking into account the type of anesthetic agents. The latter factor is particularly important given the variable effects of anesthetic agents on CA; for instance, propofol and sevoflurane have been associated with the least impairment of CA. A combination of remifentanil and propofol induces a dose-dependent metabolism-coupled reduction in CBF with preserved CA making it ideal for cases where systemic hypotension is a concern (58, 59). In the General or Local anesthesia in intra-arterial therapy (GOLIATH) study which was a prospective randomized open label blinded end-point study, 128 subjects were randomized between GA and CS. It was found that while undergoing MT therapy for LVO, the GA group had significantly higher successful reperfusion compared to the CS arm. They also had a shift toward a lower mRS score at 90 days (60). The Sedation vs. Intubation for Endovascular stroke treatment (SIESTA) study showed no difference in NIHSS at 24 h which was the primary outcome, however there was a higher proportion of patients who had attained functional independence at 3 months in the GA arm compared with the CS arm (61). Given the discordance between earlier observational studies and more recent prospective randomized but single center open label studies, further RCT data is needed.
Effect of partial pressure of CO2(PaCO2) and end-tidal CO2
Hypocapnia (low PaCO2) causes vasoconstriction in the cerebral vasculature. Its effect on CA has yet to be completely understood. Minhas et al. studied the effects of end tidal carbon dioxide (ETCO2) on CA, CBF velocity (CBFv), autoregulatory index (ARI) and arterial blood pressure in 45 healthy volunteers and found a logistic curve relationship akin to a “dose response” curve for the effects of PaCO2 on the cerebral vasculature (62). Salinet et al. (11) demonstrated a lower CBFv step response to CO2 in the stroke group compared with controls suggesting a possible modulatory effect of hypocapnia on CA and neurovascular coupling (63). A single institution study in patients undergoing GA for MT demonstrated that end tidal carbon dioxide (ETCO2) at 60 and 90 min was observed to be significantly associated with the outcome; mean ETCO2 was higher in the good clinical outcome group at both 60 and 90 min (defined as 90-day mRS 0–3) (64). Interestingly, the difference in ETCO2 between the good and poor outcome groups was relatively small; 35.2 vs. 32.2 mmHg respectively, at 60 min and 34.9 vs. 31.9 mmHg respectively, at 90 min (64).
Effect of head or body position
The literature differs on the effect of head positioning on AIS outcome and CA. It has been shown that “lying flat” increases CBFV (65–67), CPP (68) and cerebral oxygenation (69) which in theory could improve perfusion in AIS. It has also been shown that there is a reduction in CA when patients with mild AIS go from “lying flat” to “sitting up” compared to controls without any significant changes in CBFV (70). A prospective randomized cluster study (HeadPoST) in patients with acute ischemic stroke did not show any differences in functional outcome between subjects who were “lying flat” or “sitting up” (71, 72). The median NIHSS was 4 in both arms likely suggesting that LVO patients did not constitute a significant proportion of the study population.
Optimization of CA and clinical implications
In the absence of supportive data, guidelines on optimization of CA in clinical management of LVO patients are lacking. It is a common practice to adopt the following pragmatic approach which relies on an understanding of the interaction between the various physiologic factors affecting CA and neurovascular coupling (See Figure 1). This incorporates some evidence discussed in the previous sections, while awaiting prospective randomized clinical trials (See Table 2; Figure 4).
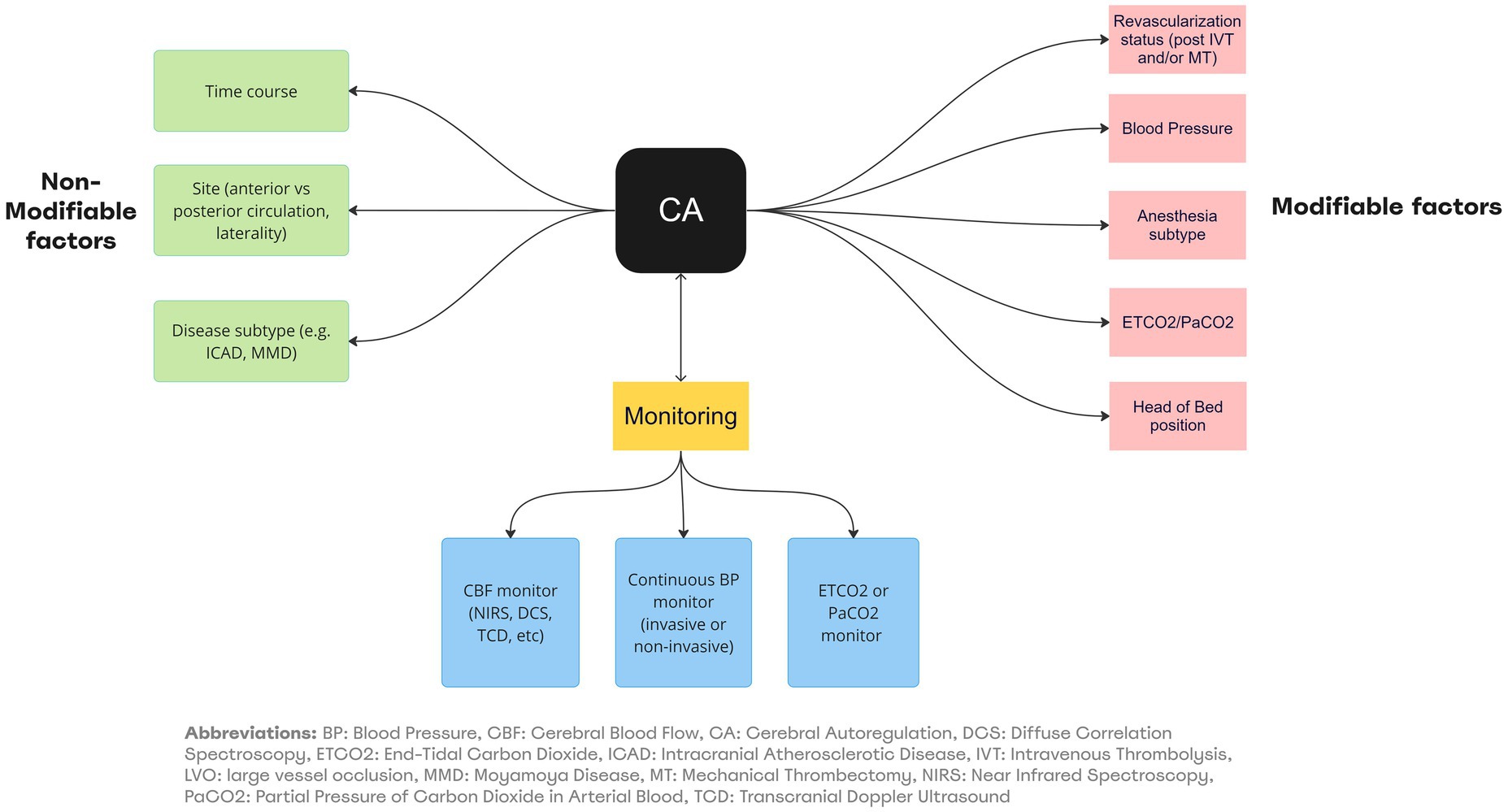
Figure 4. Summary of factors affecting CA/Neurovascular coupling and monitoring in LVO. BP, Blood Pressure; CBF, Cerebral Blood Flow; CA, Cerebral Autoregulation; DCS, Diffuse Correlation Spectroscopy; ETC02, End-Tidal Carbon Dioxide; ICAD, Intracranial Atherosclerotic Disease; IVT, Intravenous Thrombolysis; LVO, large vessel occlusion; MMO, Moyamoyo Disease; MT, Mechanical Thrombectomy; NIRS, Near-Infrared Spectroscopy; PaCO2, Partial Pressure of Carbon Dioxide in Arterial Blood; TCD, Transcranial Doppler Ultrasound.
Patients presenting with LVO in the pre-treatment phase are laid flat unless there is a concern for increased ICP or a high aspiration risk. An arterial line is placed prior to intervention and permissive systolic hypertension considering end-organ injury risk (up to 220 mmHg in non-thrombolysis eligible candidates and up to 180 mmHg in thrombolysis-eligible candidates) prior to and during intervention. Relative drops in systolic and mean arterial pressures prior to recanalization should be avoided. Upon attainment of revascularization, systolic blood pressures are lowered, usually to less than 160 mmHg and in some cases under 140 mmHg based on degree of recanalization and perceived hemorrhagic transformation risk. Tight systolic BP control under 120 mmHg is avoided except in cases with high risk for hyper perfusion syndrome such as in tandem strokes. These blood pressure parameters are observed for a minimum of 24 h and in certain cases for up to 96 h post recanalization. End tidal CO2 is commonly measured during and after treatment and a goal of ~35 mmHg is aimed for. Conscious sedation is performed in appropriate patients; in case general anesthesia is deemed necessary this decision is made early on. A dedicated anesthesiologist ensures maintenance of blood pressure and end tidal CO2 goals within the aforementioned parameters. In addition, either total intravenous anesthesia with propofol and/or remifentanil OR select inhalational gases such as sevoflurane are administered based on their favorable autoregulatory profiles. The ideal scenario will entail identifying optimum mean arterial pressure (MAP opt), limits of autoregulation (LA) and in certain cases optimum cerebral perfusion pressure (CPP opt) for each individual which will allow for real time adjustment of BP, end tidal CO2, ICP and even medication/ anesthestic agents. This precision based medicine strategy has the potential to improve clinical outcomes as suggested by preliminary work (14), but requires validation in larger cohorts of patients.
Prognostication
Infarct growth
Intact CA during AIS has not only been shown to be responsible for a smaller infarct volume but also protects the vulnerable penumbra. Sudden drastic variations in blood pressure especially the diastolic component have been shown to cause penumbral loss (73). With timely revascularization, preserved CA within 6 h (phase >37 radians) is associated with smaller infarct volumes at 24 h (17). Regional assessment of CA using intraoperative laser speckle imaging showed reduced CA in the affected hemisphere with severe impairment of CA in the ischemic yet viable penumbra (15). An important caveat is that despite successful revascularization there can be penumbral tissue loss possibly due to reperfusion injury (74).
Hemorrhagic transformation
Hemorrhagic transformation (HT) is a serious complication of AIS yet its mechanism is still under debate. Various studies have shown that an early (<24 h) impairment of CA (decrease in phase) in AIS is a strong predictor of HT (14, 74). In a study focused on CA in LVO, early impaired CA was a predictor of type 2 parenchymal hematoma (PH2), which is usually associated with worsening in the neurologic exam (12).
Conclusion
Cerebral autoregulation is impaired in LVO patients and leads to worse neurologic outcomes likely through secondary brain injury. In the presence of LVO, continuous CA monitoring may soon be a crucial tool for the periprocedural and intensive care management of these patients. A combination of multiple non-invasive modalities will likely provide a more accurate monitoring of both the cerebral macro- and micro-circulation(s) allowing a more comprehensive understanding of the actual state of CA. In unfavorable situations such as unsuccessful revascularization, absence of collateral flow or delayed treatment, tailored monitoring and management of CA may have an impact on the final outcome through a precision medicine based approach. Nonetheless, more research is needed to understand how impaired CA mediates ongoing brain injury. We need to identify associated physiological variables and the development of pharmacologic, neuroprotective or interventional therapies that could mitigate secondary brain injury with the ultimate goal of improving outcomes.
Author contributions
FS: Conceptualization, Data curation, Formal Analysis, Methodology, Resources, Supervision, Writing – original draft, Writing – review & editing. AA: Data curation, Writing – original draft, Writing – review & editing. MI: Data curation, Formal Analysis, Investigation, Methodology, Software, Writing – review & editing. RK: Writing – review & editing. AM: Writing – review & editing. GR: Conceptualization, Data curation, Methodology, Supervision, Writing – original draft, Writing – review & editing.
Funding
The author(s) declare that no financial support was received for the research, authorship, and/or publication of this article.
Conflict of interest
The authors declare that the research was conducted in the absence of any commercial or financial relationships that could be construed as a potential conflict of interest.
The author(s) declared that they were an editorial board member of Frontiers, at the time of submission. This had no impact on the peer review process and the final decision.
Publisher’s note
All claims expressed in this article are solely those of the authors and do not necessarily represent those of their affiliated organizations, or those of the publisher, the editors and the reviewers. Any product that may be evaluated in this article, or claim that may be made by its manufacturer, is not guaranteed or endorsed by the publisher.
Supplementary material
The Supplementary material for this article can be found online at: https://www.frontiersin.org/articles/10.3389/fneur.2023.1287873/full#supplementary-material
References
1. Panerai, RB, Brassard, P, Burma, JS, Castro, P, Claassen, JA, van Lieshout, JJ, et al. Transfer function analysis of dynamic cerebral autoregulation: a CARNet white paper 2022 update. J Cereb Blood Flow Metab. (2023) 43:3–25. doi: 10.1177/0271678X221119760
2. Rivera-Lara, L, Zorrilla-Vaca, A, Geocadin, RG, Healy, RJ, Ziai, W, and Mirskiet, MA. Cerebral autoregulation-oriented therapy at the bedside: a comprehensive review. Anesthesiology, (2017) 126:1187–1199. doi: 10.1097/ALN.0000000000001625
3. Kloner, RA, King, KS, and Harrington, MG. No-reflow phenomenon in the heart and brain. Am J Physiol Heart Circ Physiol. (2018) 315:H550–62. doi: 10.1152/ajpheart.00183.2018
4. Goyal, M, Menon, BK, van Zwam, WH, Dippel, DW, Mitchell, PJ, Demchuk, AM, et al. Endovascular thrombectomy after large-vessel ischaemic stroke: a meta-analysis of individual patient data from five randomised trials. Lancet. (2016) 387:1723–31. doi: 10.1016/S0140-6736(16)00163-X
5. van Beek, AH, Claassen, JA, Rikkert, MGO, and Jansen, RW. Cerebral autoregulation: an overview of current concepts and methodology with special focus on the elderly. J Cereb Blood Flow Metab. (2008) 28:1071–85. doi: 10.1038/jcbfm.2008.13
6. Lin, YP, Fu, MH, and Tan, TY. Factors associated with no or insufficient temporal bone window using transcranial color-coded sonography. J Med Ultras. (2015) 23:129–32. doi: 10.1016/j.jmu.2015.07.002
7. Kwon, JH, Kim, JS, Kang, DW, Bae, KS, and Kwon, SU. The thickness and texture of temporal bone in brain CT predict acoustic window failure of transcranial Doppler. J Neuroimaging. (2006) 16:347–52. doi: 10.1111/j.1552-6569.2006.00064.x
8. Obrig, H, Neufang, M, Wenzel, R, Kohl, M, Steinbrink, J, Einhäupl, K, et al. Spontaneous low frequency oscillations of cerebral hemodynamics and metabolism in human adults. NeuroImage. (2000) 12:623–39. doi: 10.1006/nimg.2000.0657
9. Durduran, T, and Yodh, AG. Diffuse correlation spectroscopy for non-invasive, micro-vascular cerebral blood flow measurement. NeuroImage. (2014) 85:51–63. doi: 10.1016/j.neuroimage.2013.06.017
10. Durduran, T, Kim, MN, Buckey, EM, Zhou, C, Yu, G, Choe, R, et al. Diffuse optical monitoring of cerebral oxygen metabolism at the bed-side in cerebrovascular disorders In:. Frontiers in optics 2008/laser science XXIV/Plasmonics and metamaterials/optical fabrication and testing OSA Technical Digest (CD), paper FTuD2. Optica Publishing Group (2008).
11. Salinet, ASM, Panerai, RB, and Robinson, TG. The longitudinal evolution of cerebral blood flow regulation after acute ischaemic stroke. Cerebrovasc Dis Extra. (2014) 4:186–97. doi: 10.1159/000366017
12. Sheriff, F, Castro, P, Kozberg, M, LaRose, S, Monk, A, Azevedo, E, et al. Dynamic cerebral autoregulation post endovascular thrombectomy in acute ischemic stroke. Brain Sci. (2020) 10:E641. doi: 10.3390/brainsci10090641
13. Kwan, J, Lunt, M, and Jenkinson, D. Assessing dynamic cerebral autoregulation after stroke using a novel technique of combining transcranial Doppler ultrasonography and rhythmic handgrip. Blood Press Monit. (2004) 9:3–8. doi: 10.1097/00126097-200402000-00002
14. Petersen, NH, Silverman, A, Strander, SM, Kodali, S, Wang, A, Sansing, LH, et al. Fixed compared with autoregulation-oriented blood pressure thresholds after mechanical thrombectomy for ischemic stroke. Stroke. (2020) 51:914–21. doi: 10.1161/STROKEAHA.119.026596
15. Hecht, N, Schrammel, M, Neumann, K, Müller, MM, Dreier, JP, Vajkoczy, P, et al. Perfusion-dependent cerebral autoregulation impairment in hemispheric stroke. Ann Neurol. (2021) 89:358–68. doi: 10.1002/ana.25963
16. Nogueira, RC, Aries, M, Minhas, JS, H Petersen, N, Xiong, L, Kainerstorfer, JM, et al. Review of studies on dynamic cerebral autoregulation in the acute phase of stroke and the relationship with clinical outcome. J Cereb Blood Flow Metab. (2022) 42:430–53. doi: 10.1177/0271678X211045222
17. Castro, P, Serrador, JM, Rocha, I, Sorond, F, and Azevedo, E. Efficacy of cerebral autoregulation in early ischemic stroke predicts smaller infarcts and better outcome. Front Neurol. (2017) 8:113. doi: 10.3389/fneur.2017.00113
18. Xiong, L, Tian, G, Lin, W, Wang, W, Wang, L, Leung, T, et al. Is dynamic cerebral autoregulation bilaterally impaired after unilateral acute ischemic stroke? J Stroke Cerebrovasc Dis. (2017) 26:1081–7. doi: 10.1016/j.jstrokecerebrovasdis.2016.12.024
19. Meyer, M, Juenemann, M, Braun, T, Schirotzek, I, Tanislav, C, Engelhard, K, et al. Impaired cerebrovascular autoregulation in large vessel occlusive stroke after successful mechanical Thrombectomy: a prospective cohort study. J Stroke Cerebrovasc Dis. (2020) 29:104596. doi: 10.1016/j.jstrokecerebrovasdis.2019.104596
20. Oehm, E, Hetzel, A, Els, T, Berlis, A, Keck, C, Will, HG, et al. Cerebral hemodynamics and autoregulation in reversible posterior leukoencephalopathy syndrome caused by pre−/eclampsia. Cerebrovasc Dis. (2006) 22:204–8. doi: 10.1159/000093810
21. Kruit, MC, Launer, LJ, Ferrari, MD, and van Buchem, MA. Infarcts in the posterior circulation territory in migraine. The population-based MRI CAMERA study. Brain. (2005) 128:2068–77. doi: 10.1093/brain/awh542
22. Dunatov, S, Antoncic, I, and Bralic, M. Hemodynamic changes in the posterior cerebral circulation triggered by insufficient sympathetic innervation – cause of primary intracerebral hemorrhage? Med Hypotheses. (2011) 76:668–9. doi: 10.1016/j.mehy.2011.01.027
23. Nakagawa, K, Serrador, JM, LaRose, SL, Moslehi, F, Lipsitz, LA, and Sorond, FA. Autoregulation in the posterior circulation is altered by the metabolic state of the visual cortex. Stroke. (2009) 40:2062–7. doi: 10.1161/STROKEAHA.108.545285
24. Gong, X, Liu, J, Dong, P, Zhang, P, Li, N, Zhao, X, et al. Assessment of dynamic cerebral autoregulation in patients with basilar artery stenosis. PLoS One. (2013) 8:e77802. doi: 10.1371/journal.pone.0077802
25. Reinhard, M, Müller, T, Guschlbauer, B, Timmer, J, and Hetzel, A. Dynamic cerebral autoregulation and collateral flow patterns in patients with severe carotid stenosis or occlusion. Ultrasound Med Biol. (2003) 29:1105–13. doi: 10.1016/S0301-5629(03)00954-2
26. White, RP, and Markus, HS. Impaired dynamic cerebral autoregulation in carotid artery stenosis. Stroke. (1997) 28:1340–4. doi: 10.1161/01.STR.28.7.1340
27. Reinhard, M, Roth, M, Müller, T, Czosnyka, M, Timmer, J, and Hetzel, A. Cerebral autoregulation in carotid artery occlusive disease assessed from spontaneous blood pressure fluctuations by the correlation coefficient index. Stroke. (2003) 34:2138–44. doi: 10.1161/01.STR.0000087788.65566.AC
28. Reinhard, M, Roth, M, Müller, T, Guschlbauer, B, Timmer, J, Czosnyka, M, et al. Effect of carotid endarterectomy or stenting on impairment of dynamic cerebral autoregulation. Stroke. (2004) 35:1381–7. doi: 10.1161/01.STR.0000127533.46914.31
29. Hu, HH, Kuo, TBJ, Wong, WJ, Luk, YO, Chern, CM, Hsu, LC, et al. Transfer function analysis of cerebral hemodynamics in patients with carotid stenosis. J Cereb Blood Flow Metab. (1999) 19:460–5. doi: 10.1097/00004647-199904000-00012
30. Tang, SC, Huang, YW, Shieh, JS, Huang, SJ, Yip, PK, and Jeng, JS. Dynamic cerebral autoregulation in carotid stenosis before and after carotid stenting. J Vasc Surg. (2008) 48:88–92. doi: 10.1016/j.jvs.2008.02.025
31. Reinhard, M, Gerds, TA, Grabiak, D, Zimmermann, PR, Roth, M, Guschlbauer, B, et al. Cerebral dysautoregulation and the risk of ischemic events in occlusive carotid artery disease. J Neurol. (2008) 255:1182–9. doi: 10.1007/s00415-008-0865-z
32. Tian, G, Ji, Z, Lin, Z, Pan, S, and Yin, J. Cerebral autoregulation is heterogeneous in different stroke mechanism of ischemic stroke caused by intracranial atherosclerotic stenosis. Brain Behav. (2020) 11:e01907. doi: 10.1002/brb3.1907
33. Wong, LKS. Global burden of intracranial atherosclerosis. Int J Stroke. (2006) 1:158–9. doi: 10.1111/j.1747-4949.2006.00045.x
34. Wang, S, Guo, ZN, Xing, Y, Ma, H, Jin, H, Liu, J, et al. Dynamic cerebral autoregulation in asymptomatic patients with unilateral middle cerebral artery stenosis. Medicine. (2015) 94:e2234. doi: 10.1097/MD.0000000000002234
35. Chen, J, Liu, J, Xu, WH, Xu, R, Hou, B, Cui, L-Y, et al. Impaired dynamic cerebral autoregulation and cerebrovascular reactivity in middle cerebral artery stenosis. PLoS One. (2014) 9:e88232. doi: 10.1371/journal.pone.0088232
36. Gong, XP, Li, Y, Jiang, WJ, and Wang, Y. Impaired dynamic cerebral autoregulation in middle cerebral artery stenosis. Neurol Res. (2006) 28:76–81. doi: 10.1179/016164106X91915
37. Argetsinger, DS, Miller, JW, and Fletcher, JJ. Intravenous thrombolysis, mechanical embolectomy, and intracranial stenting for hyperacute ischemic stroke in a patient with moyamoya disease. J Clin Neurosci. (2016) 29:173–5. doi: 10.1016/j.jocn.2016.01.016
38. Chen, J, Liu, J, Duan, L, Xu, R, Han, YQ, Xu, WH, et al. Impaired dynamic cerebral autoregulation in Moyamoya disease. CNS Neurosci Ther. (2013) 19:638–40. doi: 10.1111/cns.12130
39. Lee, JK, Williams, M, Jennings, JM, Jamrogowicz, JL, Larson, AC, Jordan, LC, et al. Cerebrovascular autoregulation in pediatric moyamoya disease. Pediatr Anesth. (2013) 23:547–56. doi: 10.1111/pan.12140
40. Kim, J, Ha, EJ, Kim, HS, Park, EY, Lee, HC, Choo, YH, et al. Real-time evaluation of cerebral autoregulation based on near-infrared spectroscopy to predict clinical outcome after bypass surgery in Moyamoya disease. Biomed Res Int. (2022) 2022:e3091660:1–9. doi: 10.1155/2022/3091660
41. National Institute of Neurological Disorders and Stroke rt-PA Stroke Study Group. Tissue plasminogen activator for acute ischemic stroke. N Engl J Med. (1995) 333:1581–8. doi: 10.1056/NEJM199512143332401
42. Nogueira, RC, Lam, MY, Llwyd, O, Salinet, ASM, Bor-Seng-Shu, E, Panerai, RB, et al. Cerebral autoregulation and response to intravenous thrombolysis for acute ischemic stroke. Sci Rep. (2020) 10:10554. doi: 10.1038/s41598-020-67404-9
43. Reinhard, M, Wihler, C, Roth, M, Harloff, A, Niesen, WD, Timmer, J, et al. Cerebral autoregulation dynamics in acute ischemic stroke after rtPA thrombolysis. CED. (2008) 26:147–55. doi: 10.1159/000139662
44. Nogueira, RG, Jadhav, AP, Haussen, DC, Bonafe, A, Budzik, RF, Bhuva, P, et al. Thrombectomy 6 to 24 hours after stroke with a mismatch between deficit and infarct. N Engl J Med. (2018) 378:11–21. doi: 10.1056/NEJMoa1706442
45. Tian, G, Ji, Z, Huang, K, Lin, Z, Pan, S, and Wu, Y. Dynamic cerebral autoregulation is an independent outcome predictor of acute ischemic stroke after endovascular therapy. BMC Neurol. (2020) 20:189. doi: 10.1186/s12883-020-01737-w
46. Powers, WJ, Rabinstein, AA, Ackerson, T, Adeoye, OM, Bambakidis, NC, Becker, K, et al. Guidelines for the early management of patients with acute ischemic stroke: 2019 update to the 2018 guidelines for the early management of acute ischemic stroke: a guideline for healthcare professionals from the American Heart Association/American Stroke Association. Stroke. (2019) 50:e344–418. doi: 10.1161/STR.0000000000000211
47. Mistry, EA, Mistry, AM, Nakawah, MO, Khattar, NK, Fortuny, EM, Cruz, AS, et al. Systolic blood pressure within 24 hours after thrombectomy for acute ischemic stroke correlates with outcome. J Am Heart Assoc. (2017) 6:e006167. doi: 10.1161/JAHA.117.006167
48. Anadani, M, Orabi, MY, Alawieh, A, Goyal, N, Alexandrov, AV, Petersen, N, et al. Blood pressure and outcome after mechanical thrombectomy with successful revascularization. Stroke. (2019) 50:2448–54. doi: 10.1161/STROKEAHA.118.024687
49. Peng, TJ, Ortega-Gutiérrez, S, de Havenon, A, and Petersen, NH. Blood pressure management after endovascular thrombectomy. Front Neurol. (2021) 12:723461. doi: 10.3389/fneur.2021.723461
50. Yang, P, Song, L, Zhang, Y, Zhang, X, Chen, X, Li, Y, et al. Intensive blood pressure control after endovascular thrombectomy for acute ischaemic stroke (ENCHANTED2/MT): a multicentre, open-label, blinded-endpoint, randomised controlled trial. Lancet. (2022) 400:1585–96. doi: 10.1016/S0140-6736(22)01882-7
51. Mistry, EA, Sucharew, H, Mistry, AM, Mehta, T, Arora, N, Starosciak, AK, et al. Blood pressure after endovascular therapy for ischemic stroke (BEST): a multicenter prospective cohort study. Stroke (2019) 50:3449–455. doi: 10.1161/STROKEAHA.119.026889
52. Song, L, Yang, P, Zhang, Y, Zhang, X, Chen, X, Li, Y, et al. The second randomized controlled ENhanced control of hypertension ANd Thrombectomy strokE stuDy (ENCHANTED2): protocol and progress. Int J Stroke. (2022):17474930221120345. doi: 10.1177/17474930221120345
53. Mistry, EA, Hart, KW, Davis, LT, Gao, Y, Prestigiacomo, CJ, Mittal, S, et al. Blood pressure management after endovascular therapy for acute ischemic stroke: the BEST-II randomized clinical trial. JAMA. (2023) 330:821–31. doi: 10.1001/jama.2023.14330
54. Abou-Chebl, A, Lin, R, Hussain, MS, Jovin, TG, Levy, EI, Liebeskind, DS, et al. Conscious sedation versus general anesthesia during endovascular therapy for acute anterior circulation stroke: preliminary results from a retrospective, multicenter study. Stroke. (2010) 41:1175–9. doi: 10.1161/STROKEAHA.109.574129
55. Jumaa, MA, Zhang, F, Ruiz-Ares, G, Gelzinis, T, Malik, AM, Aleu, A, et al. Comparison of safety and clinical and radiographic outcomes in endovascular acute stroke therapy for proximal middle cerebral artery occlusion with intubation and general anesthesia versus the nonintubated state. Stroke. (2010) 41:1180–4. doi: 10.1161/STROKEAHA.109.574194
56. Wagner, B, Lorscheider, J, Wiencierz, A, Blackham, K, Psychogios, M, Bolliger, D, et al. Endovascular treatment for acute ischemic stroke with or without general anesthesia: a matched comparison. Stroke. (2022) 53:1520–9. doi: 10.1161/STROKEAHA.121.034934
57. Brinjikji, W, Murad, MH, Rabinstein, AA, Cloft, HJ, Lanzino, G, and Kallmes, DF. Conscious sedation versus general anesthesia during endovascular acute ischemic stroke treatment: a systematic review and meta-analysis. Am J Neuroradiol. (2015) 36:525–9. doi: 10.3174/ajnr.A4159
58. Messick, JM, Newberg, LA, Nugent, M, and Faust, RJ. Principles of neuroanesthesia for the nonneurosurgical patient with CNS pathophysiology. Anesth Analg. (1985) 64:143???174. doi: 10.1213/00000539-198502000-00008
59. Leslie-Mazwi, TM, Sims, JR, Hirsch, JA, and Nogueira, RG. Periprocedural blood pressure management in neurointerventional surgery. J NeuroIntervent Surg. (2011) 3:66–73. doi: 10.1136/jnis.2009.001206
60. Simonsen, CZ, Yoo, AJ, Sørensen, LH, Juul, N, Johnsen, SP, Andersen, G, et al. Effect of general anesthesia and conscious sedation during endovascular therapy on infarct growth and clinical outcomes in acute ischemic stroke: a randomized clinical trial. JAMA Neurol. (2018) 75:470–7. doi: 10.1001/jamaneurol.2017.4474
61. Schönenberger, S, Uhlmann, L, Hacke, W, Schieber, S, Mundiyanapurath, S, Purrucker, JC, et al. Effect of conscious sedation vs general anesthesia on early neurological improvement among patients with ischemic stroke undergoing endovascular thrombectomy: a randomized clinical trial. JAMA. (2016) 316:1986–96. doi: 10.1001/jama.2016.16623
62. Minhas, JS, Panerai, RB, and Robinson, TG. Modelling the cerebral haemodynamic response in the physiological range of PaCO2. Physiol Meas. (2018) 39:065001. doi: 10.1088/1361-6579/aac76b
63. Salinet, ASM, Robinson, TG, and Panerai, RB. Effects of cerebral ischemia on human neurovascular coupling, CO2 reactivity, and dynamic cerebral autoregulation. J Appl Physiol. (2015) 118:170–7. doi: 10.1152/japplphysiol.00620.2014
64. Takahashi, CE, Brambrink, AM, Aziz, MF, Macri, E, Raines, J, Multani-Kohol, A, et al. Association of intraprocedural blood pressure and end tidal carbon dioxide with outcome after acute stroke intervention. Neurocrit Care. (2014) 20:202–8. doi: 10.1007/s12028-013-9921-3
65. Favilla, CG, Mesquita, RC, Mullen, M, Durduran, T, Lu, X, Kim, MN, et al. Optical bedside monitoring of cerebral blood flow in acute ischemic stroke patients during head-of-bed manipulation. Stroke. (2014) 45:1269–74. doi: 10.1161/STROKEAHA.113.004116
66. Wojner-Alexander, AW, Garami, Z, Chernyshev, OY, and Alexandrov, AV. Heads down: flat positioning improves blood flow velocity in acute ischemic stroke. Neurology. (2005) 64:1354–7. doi: 10.1212/01.WNL.0000158284.41705.A5
67. Olavarría, VV, Arima, H, Anderson, CS, Brunser, AM, Muñoz-Venturelli, P, Heritier, S, et al. Head position and cerebral blood flow velocity in acute ischemic stroke: a systematic review and meta-analysis. Cerebrovasc Dis. (2014) 37:401–8. doi: 10.1159/000362533
68. Schwarz, S, Georgiadis, D, Aschoff, A, and Schwab, S. Effects of body position on intracranial pressure and cerebral perfusion in patients with large hemispheric stroke. Stroke. (2002) 33:497–501. doi: 10.1161/hs0202.102376
69. Hargroves, D, Tallis, R, Pomeroy, V, and Bhalla, A. The influence of positioning upon cerebral oxygenation after acute stroke: a pilot study. Age Ageing. (2008) 37:581–5. doi: 10.1093/ageing/afn143
70. Lam, MY, Haunton, VJ, Nath, M, Panerai, RB, and Robinson, TG. The effect of head positioning on cerebral hemodynamics: experiences in mild ischemic stroke. J Neurol Sci. (2020) 419:117201. doi: 10.1016/j.jns.2020.117201
71. Anderson, CS, Arima, H, Lavados, P, Billot, L, Hackett, ML, Olavarría, VV, et al. Cluster-randomized, crossover trial of head positioning in acute stroke. N Engl J Med. (2017) 376:2437–47. doi: 10.1056/NEJMoa1615715
72. Muñoz-Venturelli, P, Arima, H, Lavados, P, Brunser, A, Peng, B, Cui, L, et al. Head position in stroke trial (HeadPoST) – sitting-up vs lying-flat positioning of patients with acute stroke: study protocol for a cluster randomised controlled trial. Trials. (2015) 16:256. doi: 10.1186/s13063-015-0767-1
73. Jeong, HG, Kim, BJ, Kim, H, Jung, C, Han, MK, Liebeskind, DS, et al. Blood pressure drop and penumbral tissue loss in nonrecanalized emergent large vessel occlusion. Stroke. (2019) 50:2677–84. doi: 10.1161/STROKEAHA.119.025426
Glossary
Keywords: cerebral autoregulation, large vessel occlusion, acute ischemic stroke, mechanical thrombectomy, cerebral blood flow
Citation: Sheriff FG, Ahmad A, Inam ME, Khatri R, Maud A and Rodriguez GJ (2023) A systematic review on the assessment of cerebral autoregulation in patients with Large Vessel Occlusion. Front. Neurol. 14:1287873. doi: 10.3389/fneur.2023.1287873
Edited by:
Farhan Siddiq, University of Missouri System, United StatesReviewed by:
Ji Man Hong, Ajou University, Republic of KoreaTakeshi Yoshimoto, National Cerebral and Cardiovascular Center, Japan
Copyright © 2023 Sheriff, Ahmad, Inam, Khatri, Maud and Rodriguez. This is an open-access article distributed under the terms of the Creative Commons Attribution License (CC BY). The use, distribution or reproduction in other forums is permitted, provided the original author(s) and the copyright owner(s) are credited and that the original publication in this journal is cited, in accordance with accepted academic practice. No use, distribution or reproduction is permitted which does not comply with these terms.
*Correspondence: Gustavo J. Rodriguez, Z3VzdGF2by5qLnJvZHJpZ3VlekB0dHVoc2MuZWR1
†ORCID: Hu Liu orcid.org/0000-0002-6779-9377