- Department of Radiological Sciences, School of Medicine, University of California, Irvine, Irvine, CA, United States
Neuroimaging is an inevitable component of the assessment of neurological emergencies. Magnetic resonance imaging (MRI) is the preferred imaging modality for detecting neurological pathologies and provides higher sensitivity than other modalities. However, difficulties such as intra-hospital transport, long exam times, and availability in strict access-controlled suites limit its utility in emergency departments and intensive care units (ICUs). The evolution of novel imaging technologies over the past decades has led to the development of portable MRI (pMRI) machines that can be deployed at point-of-care. This article reviews pMRI technologies and their clinical implications in acute neurological conditions. Benefits of pMRI include timely and accurate detection of major acute neurological pathologies such as stroke and intracranial hemorrhage. Additionally, pMRI can be potentially used to monitor the progression of neurological complications by facilitating serial measurements at the bedside.
Introduction
Neurological disorders are the second leading cause of death with 9.0 million deaths per year globally and the leading cause of disability with disability-adjusted life-years (DALYs) of 276 million (1, 2). Almost half of the burden of neurological diseases takes place in low-income and middle-income countries (3). Magnetic resonance imaging (MRI) is the preferred imaging modality for neurological disorders. However, MRI scanners are costly, immobile, and lack timely availability. This potentially delays diagnosis and management. The development of low-cost portable MRI (pMRI) devices has enabled point-of-care (POC) neuroimaging. The pMRI device was first cleared by the U.S. Food and Drug Administration in August 2020 (4). This article will briefly introduce pMRI technology. Then we review the current role of pMRI in acute neurological and critical care settings. Finally, we conclude with a discussion on the current scope and ongoing opportunities.
Neuroimaging modalities
Neuroimaging is a critical step for timely triage, diagnosis, and decision-making for treatment of patients with clinically suspected acute conditions such as stroke (4–6). Non-contrast computed tomography (CT) has historically been a cost-effective imaging modality for the initial evaluation of neurologic patients (7, 8). However, studies have increasingly demonstrated that multimodal MRI has higher sensitivity for detecting many acute neurological conditions. Improved soft tissue contrast, lack of radiation exposure, and detection of small infarcts are the reasons for MRI's superiority over CT (9, 10).
Portable MRI
Conventional MRI with high-strength magnetic field (1.5–3 T) systems are large, immobile, costly, and require special infrastructure, rigid safety precautions, and highly trained technicians (4, 11, 12). Usage of the pMRI with a considerably lower strength (64 mT) allows for 3D, whole brain Diffusion-weighted imaging (DWI), Fluid-attenuated inversion recovery (FLAIR), T2-weighted (T2W), and T1-weighted (T1W) imaging (Figure 1). DWI is recognized as a superior method for detection of acute infarcts by providing the most relevant contrast. FLAIR, T1W, and T2W are highly sensitive in detection of subacute and chronic ischemic brain infarcts and observation of response to treatment (9). Some of the advantages of pMRI include:
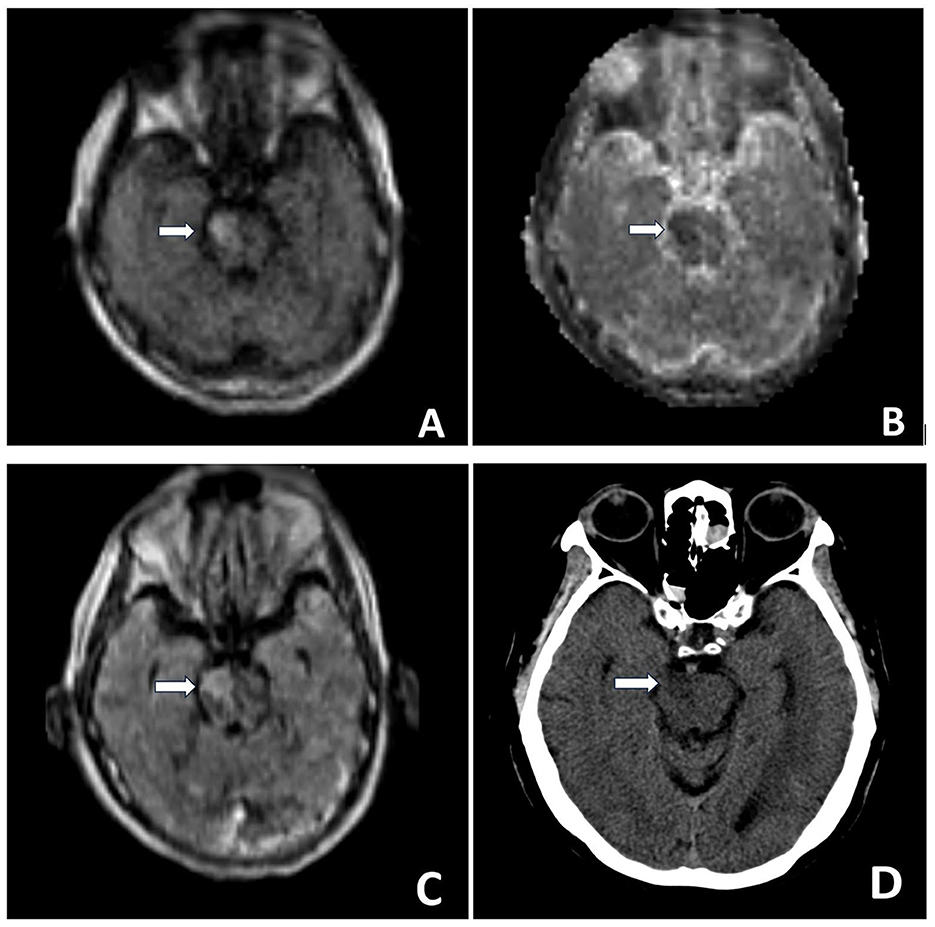
Figure 1. Brain MR POC MRI DWI sequence (A), ADC sequence (B) demonstrate right ventral pontine restricted diffusion. T2/FLAIR sequence (C) demonstrates right ventral pontine hyper-intense signal. Head CT performed the same day (D): Hypo-attenuation at the site of right pontine infarction, but at times difficult to confidently diagnose posterior fossa strokes on CT given artifacts in the area. MRI exam helps verify diagnosis. MR Images were obtained using the Swoop Hyperfine Portable MR Imaging System (64 mT) at the University of California, Irvine Medical Center.
(1) POC neuroimaging in emergency settings (13–19) or for critically ill patients in intensive care units (ICU) (11, 20, 21); (2) Reduced risk of transmission of infections during pandemics like coronavirus disease 2019 (COVID-19) (12, 22, 23); (3) Logistical access for brain MR imaging (24–26) in the ICU or in remote low- and middle-income regions (9, 27–29); (4) Fewer safety concerns regarding intra-hospital transport due to required restrictions for conventional clinical MRI (30–33); (5) Cost-effectiveness (34–37); (6) Reduced hospital length of stay (LOS) (11, 15, 21); (7) No risk of thermal burns and ferrous projectiles; (8) Detection of intracranial pathologies similar to that observed on conventional MRI by offering different pulse sequences (4, 14, 38).
Role of pMRI in acute neurological conditions
Strategies for pMRI imaging in the emergency department
Lang et al. have proposed dividing the usage of pMRI imaging in ED into three groups based on their field strength and portability (39). The first group are “easy-to-site suite” scanners using a standard superconducting solenoid magnet architecture; Examples are High-field (3T) (40, 41) and Mid-field (between 0.5 T and 1.0 T) head-only MRI scanners (42–44). The second group would be truly portable scanners that would operate at a low field (50 mT to 200 mT), need unconventional electromagnetic interference (EMI) mitigation, and must operate with substantially reduced electrical power and without water cooling or cryogenics. The 64 mT Hyperfine Swoop scanner (Guilford, CT, USA), the first FDA-cleared clinical pMRI scanner (12, 45), and the 80 mT “Halbach-bulb” rare-earth magnet configuration (38, 46, 47) are some of the advances in this class. The ultra-low-field shield-free pMRI using a Samarium-cobalt magnet enables the cancellation of EMI and elimination of the traditional radiofrequency shielding cage. This scanner promisingly met the clinical needs to diagnose brain tumors and stroke (48). The third group are “hand-held” level MRI devices, likely with greatly reduced imaging capabilities, but inexpensive and small enough to be considered MR detectors or monitoring devices more than diagnostic imaging devices. An example of this is a 7 kg device “MR Cap” (49). This type of instrument could warn of early changes and impending events by continuously imaging the brain in ED or ICU, particularly in patients that are difficult for clinical evaluation and examination (e.g. sedated patients).
Stroke
Stroke is the second leading cause of mortality and morbidity worldwide and affects 15 million people each year. Neuroimaging plays a significant role in both the diagnosis and treatment of stroke within the golden time (first 3–4.5 h after the event). The availability of open-bore systems has enabled better temporal access than fixed CT or conventional MRI and resulted in timely therapeutic intervention and reduced door-to-needle time (9). DWI remains the gold standard sequence imaging for acute stroke and is available on low-field and very low-field/ultra-low-field MR scanners (29). In 2020, Cahn et al. suggested the clinical utility of POC MRI for evaluation of stroke patients. Using the 64 mT scanner (50), they examined acute stroke patients and were able to produce 3D clinical quality images by performing DWI sequences and suggested it as a safe and viable tool in complex clinical care environments (17). Zubair et al. (13) and Hovis et al. (15) in 2021 reported using 64 mT pMRI for evaluation of patients with suspected stroke. The implementation of pMRI resulted in faster work-up, decreased hospital stays, and yielded reliable results compared to non-contrast CT scan and conventional MRI. In 2022, Yuen et al. utilized 64 mT pMRI as first-line diagnostic imaging for the detection of ischemic stroke. POC MRI was able to capture lesions as small as 4 mm. Infarcts were detected as hyper-intense regions on T2-W, FLAIR, and DWI sequences with a sensitivity of 98, 100, and 86%, respectively. Stroke volume measurements were consistent between low-field pMRI and conventional high-field MRI studies. Significant correlations were seen between low-field pMRI stroke volumes and stroke severity, and consequently functional outcome at discharge. They validated the clinical use of low-field pMRI as a novel imaging solution for patients with ischemic stroke, especially in resource-limited environments (18).
Mobile imaging of stroke
The mobile stroke unit was first proposed hypothetically by Fassbender et al. for evaluation and diagnosis of stroke considering timely accessibility (51) and was first introduced by Parker et al. in 2015 (52). It is a combination of emergency medical services, telemedicine, and a portable CT scanner (53). Low-field pMRI could potentially provide POC service in this setting. Portable low-field scanners do not require additional shielding and have a similar footprint as portable CT scanners. Therefore, imaging of stroke patients even in remote regions or resource-limited geographies would be possible (9). Deoni et al. assessed the feasibility of a cargo van equipped with 64 mT low-field pMRI. The data collected by the mobile system showed no significant differences regarding geometric distortion, signal-to-noise ratio, or tissue segmentation compared to laboratory settings. Their results illustrated a promising approach that allows for neuroimaging of participants at home, school, etc. (54). However, one significant challenge in mobile unit implementation is motion artifact.
Intracerebral hemorrhage and midline shift
In 2020, Shah et al. analyzed the ICH detection capacity of a bedside POC MRI scanner. Prospectively, using a 64 mT scanner, a pathologic lesion was identified on every exam with 100% sensitivity by T2-W and FLAIR sequences. They suggested that low-field, POC MRI may be used to detect hemorrhagic strokes at the bedside (19). In 2021 Mazurek et al. evaluated the use of low-field MRI (64 mT) for assessing ICH in comparison with conventional 1.5/3 T MRI or non-contrast CT scan. PMRI could detect ICH with 80.4% sensitivity and 96.6% specificity. They found that hematoma volume measurements derived from pMRI exams correlated with impaired cognitive status and worse functional capacity at discharge. They proposed the Low-field pMRI as a useful technology in resource-limited settings (16). With the same methodology, Sheth et al. assessed mass effects and the resulting MLS in brain-injured patients using pMRI. There was significant agreement between pMRI and conventional MRI or CT. Low-field pMRI identified MLS with a sensitivity of 93% and specificity of 96% and could predict poor clinical outcomes at discharge. They regarded bedside pMRI as valuable for detecting mass effects (14).
Critically ill patients
In patients admitted to the ICU, transportation to an MRI suite may place the patient at numerous risks, including compromise of venous or arterial access, endotracheal tube displacement, hypoxia, hypotension, increased intracranial pressure, and cardiac arrest. In patients with contagious infections such as COVID-19 other concerns such as exposure to a larger patient population and hospital staff and the need for decontamination of radiology suites are logistical barriers (12, 22). On the other hand, substantial delays between ordering and obtaining an MRI may further increase patients' hospital length of stay and subsequent total hospitalization costs. Importantly, limited access to MRI hinders timely and targeted therapeutic decision-making in this patient population (4, 11, 21).
Low-field pMRI (64 mT) produces less than 5 Gauss beyond the 5-foot (1.52 meter) safety zone of the magnet's center, which is significantly lower than conventional MRI. It can be feasibly employed in the presence of all ICU equipment during scanning without adverse events (26). Additionally, POC MRI may improve the functionality of existing fixed MRI machines by removing complicated and time-sensitive critically ill patients from the scheduled waiting list (11, 20, 21). In 2020–21, Turpin et al. (22) and Mazurek et al. (23) reported their experience of imaging critically ill COVID-19 patients with a self-shielding, 64 mT pMRI. The use of pMRI was safe, and feasible and led to changes in clinical management based on imaging results. Also, the diagnostic MRI quality was graded to be adequate (85%) with FLAIR sequences demonstrating the highest quality, and DWI scoring the lowest (22). Similarly, Sheth et al. assessed the utility of pMRI in complex clinical settings such as neuroscience labs and COVID-19 ICUs and reached similar results (12). However, Kuoy et al. obtained a diagnostic rate of 72% and mentioned that motion artifact, habitus, and lower field-strength may necessitate higher field-strength MRI subsequently. They also found that the turnaround time was reduced for patients in the ICU (5.3 vs. 11.7 h for fixed scanner) but not for patients in the ED (3.4 vs. 3.7 h for fixed scanner), suggesting that ease of transport and proximity to the fixed scanner may affect turnaround times (21). Regarding the time-dependent nature of critically ill patients and particularly those resuscitated from cardiac arrest, Beekman et al. demonstrated that pMRI could successfully identify patients with hypoxic-ischemic brain injury (HIBI). Accurate outcome prediction is required to prevent withdrawal of life-sustaining therapy in comatose survivors with good functional outcomes; this is possible through serial MR imaging and quantitative analyses using FLAIR intensity (11). The same applies to patients on extracorporeal membrane oxygenation (ECMO) as both logistic and diagnostic advantages of POC MRI led to early detection and timely intervention in acute brain injuries and could improve their outcomes (20).
Other applications
The application of pMRI for musculoskeletal, spine, and neck imaging seems promising. Due to common use of metal implants in orthopedic patients, low-field MRI scanners have attracted interest in this field. Some specific designs (e.g. open bores, extremity-specific scanners, and vertical scanners for weight-bearing studies) have facilitated clinical imaging, for example by positioning the limbs in the magnetic field (55). Lee et al. showed an excellent correlation between low- and high-field systems for detection of disk herniation and nerve root compression (56). The application of outpatient neuroimaging by pMRI in neurology and neurosurgery fields has also been studied in recent years. To evaluate the sensitivity of pMRI in detecting multiple sclerosis lesions, Arnold et al. compared 3T MRI with 64 mT pMRI. They showed 94% sensitivity and a strong correlation between the scanners, however, smaller white matter lesions may be missed by pMRI (57). Ventricular volumes in hydrocephalous could be successfully estimated by pMRI compared to conventional MRI and the measurements were strongly correlated. Implanted shunts were similarly affected by magnetic mechanism; However, with reduced interference compared to high-field MRI (58). Interestingly, high-quality cognitive data collection can be achieved through remote neuroimaging and is preferred by patients in a study conducted by Deoni et al. They proposed that pMRI may be an important tool in neuroimaging research (59). Due to the limitation of data regarding pediatric neurodevelopment in lower and middle-income countries, Deoni et al. conducted a study on volumetric measurements and developmental patterns by a 64 mT pMRI in children 6 weeks to 16 years. The authors reported higher success rates with 64 mT pMRI (89%) compared to 3T MRI (75%). Nonetheless, some sequences and analytical software needed to be optimized for pediatric populations. They suggested that implementation of low-field pMRI may provide a more profound understanding of neurodevelopmental patterns among children in low-resource countries that are affected by malnutrition, hydrocephaly, infections, psychosocial challenges, and other environmental factors (60).
Disadvantages
Some studies have reviewed the clinical challenges of pMRI. Examples include lower quality of DWI sequences on portable scanners and the potential risk of overlooking pathologies; Identifying stroke penumbra and vasculature, i.e. the infarct core and the collateral flow; need for skilled personnel for running the scanner, reading the results, and providing therapy on-site (with the ability to administer tPA) (9, 55). Additionally, the dangers of transferring the scanner through hospital floors; the field shield needed to protect nearby pacemakers, defibrillators, implants, foreign metal bodies, etc.; and possible hygiene issues by moving tools that may serve as a source of spreading pathogens are some of the other potential limitations that can be proposed and further studies are needed to address these issues.
Discussion
The studies and technologies described in this review demonstrate that low-field pMRI can be successfully integrated into the clinical workflow to provide timely, accurate, and accessible information for the detection of major acute neurological pathologies such as stroke, hemorrhage, and lesions with mass effect. It is particularly useful in settings where cost, space, or scan-time cause limitations; such as the emergency department, critical care setting, mobile stroke units, and resource-limited environments. By serial measurements, pMRI can potentially enhance insight into the dynamic profile and time course of neurological pathologies and hold promise for preventing the progression of neurological complications. However, further studies aimed at establishing the real added value of POC MRI compared to portable head CT scan and the concordance between POC MRI and conventional MRI in a larger variety of clinical settings, optimizing and improving acquisition and post-processed images, addition of newer sequences, and the ability to perform scans with contrast agents to detect subtle neurological abnormalities are needed (22). Moreover, further work is still needed to fully determine the accuracy of POC MRI, clinical indications and utility, and patient selection.
Author contributions
AS: Writing—original draft, Writing—review and editing. DC: Writing—review and editing. EK: Writing—review and editing. VY: Supervision, Writing—review and editing.
Funding
The author(s) declare that no financial support was received for the research, authorship, and/or publication of this article.
Conflict of interest
The authors declare that the research was conducted in the absence of any commercial or financial relationships that could be construed as a potential conflict of interest.
Publisher's note
All claims expressed in this article are solely those of the authors and do not necessarily represent those of their affiliated organizations, or those of the publisher, the editors and the reviewers. Any product that may be evaluated in this article, or claim that may be made by its manufacturer, is not guaranteed or endorsed by the publisher.
References
1. Feigin VL, Abajobir AA, Abate KH, Abd-Allah F, Abdulle AM, Abera SF, et al. Global, regional, and national burden of neurological disorders during 1990–2015: a systematic analysis for the Global Burden of Disease Study 2015. Lancet Neurol. (2017) 16:877–9. doi: 10.1016/S1474-4422(17)30299-5
2. Carroll WM. The global burden of neurological disorders. Lancet Neurol. (2019) 18:418–9. doi: 10.1016/S1474-4422(19)30029-8
3. Organization WH. Neurological Disorders: Public Health Challenges. Geneva: World Health Organization (2006).
4. Prabhat AM, Crawford AL, Mazurek MH, Yuen MM, Chavva IR, Ward A, et al. Methodology for low-field, portable magnetic resonance neuroimaging at the bedside. Front Neurol. (2021) 12:760321. doi: 10.3389/fneur.2021.760321
5. Williamson C, Morgan L, Klein JP. Imaging in neurocritical care practice. Semin Respir Crit Care Med. (2017) 38:840–52. doi: 10.1055/s-0037-1608770
6. Powers WJ, Rabinstein AA, Ackerson T, Adeoye OM, Bambakidis NC, Becker K, et al. Guidelines for the early management of patients with acute ischemic stroke: 2019 update to the 2018 guidelines for the early management of acute ischemic stroke: a guideline for healthcare professionals from the American Heart Association/American Stroke Association. Stroke. (2019) 50:e344–418. doi: 10.1161/STR.0000000000000211
7. Cihangiroglu M, Ramsey RG, Dohrmann GJ. Brain injury: analysis of imaging modalities. Neurol Res. (2002) 24:7–18. doi: 10.1179/016164102101199440
8. Ibrahim R, Samian Sd, Mazli MZ, Amrizal MN, Aljunid SM. Cost of Magnetic Resonance Imaging (MRI) and Computed Tomography (CT) scan in UKMMC. BMC Health Serv Res. (2012) 12:P11. doi: 10.1186/1472-6963-12-S1-P11
9. Bhat SS, Fernandes TT, Poojar P, da Silva Ferreira M, Rao PC, Hanumantharaju MC, et al. Low-field MRI of stroke: challenges and opportunities. J Magn Reson Imaging. (2021) 54:372–90. doi: 10.1002/jmri.27324
10. Vymazal J, Rulseh AM, Keller J, Janouskova L. Comparison of CT and MR imaging in ischemic stroke. Insights Imaging. (2012) 3:619–27. doi: 10.1007/s13244-012-0185-9
11. Beekman R, Crawford A, Mazurek MH, Prabhat AM, Chavva IR, Parasuram N, et al. Bedside monitoring of hypoxic ischemic brain injury using low-field, portable brain magnetic resonance imaging after cardiac arrest. Resuscitation. (2022) 176:150–8. doi: 10.1016/j.resuscitation.2022.05.002
12. Sheth KN, Mazurek MH, Yuen MM, Cahn BA, Shah JT, Ward A, et al. Assessment of brain injury using portable, low-field magnetic resonance imaging at the bedside of critically ill patients. JAMA Neurol. (2020) 78:41–7. doi: 10.1001/jamaneurol.2020.3263
13. Zubair AS, Crawford A, Prabhat AM, Sheth KN. Use of portable imaging modalities in patients with neurologic disorders: a case-based discussion. Cureus. (2021) 13:e15841. doi: 10.7759/cureus.15841
14. Sheth KN, Yuen MM, Mazurek MH, Cahn BA, Prabhat AM, Salehi S, et al. Bedside detection of intracranial midline shift using portable magnetic resonance imaging. Sci Rep. (2022) 12:67. doi: 10.1038/s41598-021-03892-7
15. Hovis G, Langdorf M, Dang E, Chow D. MRI at the bedside: a case report comparing fixed and portable magnetic resonance imaging for suspected stroke. Cureus. (2021) 13:e16904. doi: 10.7759/cureus.16904
16. Mazurek MH, Cahn BA, Yuen MM, Prabhat AM, Chavva IR, Shah JT, et al. Portable, bedside, low-field magnetic resonance imaging for evaluation of intracerebral hemorrhage. Nat Commun. (2021) 12:5119. doi: 10.1038/s41467-021-25441-6
17. Cahn B, Shah J, Dyvorne H, O'Halloran R, Poole M, Yuen M, et al. Deployment of portable, bedside, low-field magnetic resonance imaging for evaluation of stroke patients. Neurology. (2020) 94(Suppl. 15):272. doi: 10.1161/str.51.suppl_1.57
18. Yuen MM, Prabhat AM, Mazurek MH, Chavva IR, Crawford A, Cahn BA, et al. Portable, low-field magnetic resonance imaging enables highly accessible and dynamic bedside evaluation of ischemic stroke. Sci Adv. (2022) 8:eabm3952. doi: 10.1126/sciadv.abm3952
19. Shah J, Cahn B, By S, Welch EB, Sacolick L, Yuen M, et al. Portable, bedside, low-field magnetic resonance imaging in an intensive care setting for intracranial hemorrhage. Neurology. (2020) 94(Suppl. 15):270. doi: 10.1161/str.51.suppl_1.wp413
20. Cho SM, Wilcox C, Keller S, Acton M, Rando H, Etchill E, et al. Assessing the SAfety and FEasibility of bedside portable low-field brain Magnetic Resonance Imaging in patients on ECMO (SAFE-MRI ECMO study): study protocol and first case series experience. Crit Care. (2022) 26:119. doi: 10.1186/s13054-022-03990-6
21. Kuoy E, Glavis-Bloom J, Hovis G, Yep B, Biswas A, Masudathaya L-A, et al. Point-of-care brain mri: preliminary results from a single-center retrospective study. Radiology. (2022) 305:666–71. doi: 10.1148/radiol.211721
22. Turpin J, Unadkat P, Thomas J, Kleiner N, Khazanehdari S, Wanchoo S, et al. Portable magnetic resonance imaging for ICU patients. Crit Care Explor. (2020) 2:e0306. doi: 10.1097/CCE.0000000000000306
23. Mazurek MH, Yuen MM, Cahn BA, Rosen MS, Gobeske KT, Gilmore EJ, et al. Low-field, portable magnetic resonance imaging at the bedside to assess brain injury in patients with severe COVID-19. Neurology. (2021) 96(Suppl. 15):1349.
24. Lother S, Schiff SJ, Neuberger T, Jakob PM, Fidler F. Design of a mobile, homogeneous, and efficient electromagnet with a large field of view for neonatal low-field MRI. Magma. (2016) 29:691–8. doi: 10.1007/s10334-016-0525-8
25. O'Reilly T, Webb A. Deconstructing and reconstructing MRI hardware. J Magn Reson. (2019) 306:134–8. doi: 10.1016/j.jmr.2019.07.014
26. Sien ME, Robinson AL, Hu HH, Nitkin CR, Hall AS, Files MG, et al. Feasibility of and experience using a portable MRI scanner in the neonatal intensive care unit. Arch Dis Child Fetal Neonatal Ed. (2022) 108:45–50. doi: 10.1136/archdischild-2022-324200
27. Shen FX, Wolf SM, Bhavnani S, Deoni S, Elison JT, Fair D, et al. Emerging ethical issues raised by highly portable MRI research in remote and resource-limited international settings. Neuroimage. (2021) 238:118210. doi: 10.1016/j.neuroimage.2021.118210
28. Geethanath S, Vaughan JT Jr. Accessible magnetic resonance imaging: a review. J Magn Reson Imaging. (2019) 49:e65–77. doi: 10.1002/jmri.26638
29. Okorie CK, Ogbole GI, Owolabi MO, Ogun O, Adeyinka A, Ogunniyi A. Role of diffusion-weighted imaging in acute stroke management using low-field magnetic resonance imaging in resource-limited settings. West Afr J Radiol. (2015) 22:61–6. doi: 10.4103/1115-3474.162168
30. Gimenez FMP, de Camargo WHB, Gomes ACB, Nihei TS, Andrade MWM, Valverde M, et al. Analysis of adverse events during intrahospital transportation of critically ill patients. Crit Care Res Pract. (2017) 2017:6847124. doi: 10.1155/2017/6847124
31. Knight PH, Maheshwari N, Hussain J, Scholl M, Hughes M, Papadimos TJ, et al. Complications during intrahospital transport of critically ill patients: Focus on risk identification and prevention. Int J Crit Illn Inj Sci. (2015) 5:256–64. doi: 10.4103/2229-5151.170840
32. Veiga VC, Postalli NF, Alvarisa TK, Travassos PP, Vale R, Oliveira CZ, et al. Adverse events during intrahospital transport of critically ill patients in a large hospital. Rev Bras Ter Intensiva. (2019) 31:15–20. doi: 10.5935/0103-507X.20190003
33. Picetti E, Antonini MV, Lucchetti MC, Pucciarelli S, Valente A, Rossi I, et al. Intra-hospital transport of brain-injured patients: a prospective, observational study. Neurocrit Care. (2013) 18:298–304. doi: 10.1007/s12028-012-9802-1
34. Sarracanie M, LaPierre CD, Salameh N, Waddington DEJ, Witzel T, Rosen MS. Low-cost high-performance MRI. Sci Rep. (2015) 5:15177. doi: 10.1038/srep15177
35. Wald LL, McDaniel PC, Witzel T, Stockmann JP, Cooley CZ. Low-cost and portable MRI. J Magn Reson Imaging. (2020) 52:686–96. doi: 10.1002/jmri.26942
36. Cooley CZ, Stockmann JP, Witzel T, LaPierre C, Mareyam A, Jia F, et al. Design and implementation of a low-cost, tabletop MRI scanner for education and research prototyping. J Magn Reson. (2020) 310:106625. doi: 10.1016/j.jmr.2019.106625
37. Huang S, Ren ZH, Obruchkov S, Gong J, Dykstra R, Yu W. Portable low-cost MRI system based on permanent magnets/magnet arrays. Invest Magn Reson Imag. (2019) 23:179–201. doi: 10.13104/imri.2019.23.3.179
38. Cooley CZ, McDaniel PC, Stockmann JP, Srinivas SA, Cauley SF, Sliwiak M, et al. A portable scanner for magnetic resonance imaging of the brain. Nat Biomed Eng. (2021) 5:229–39. doi: 10.1038/s41551-020-00641-5
39. Lang M, Rapalino O, Huang S, Lev MH, Conklin J, Wald LL. Emerging techniques and future directions: fast and portable magnetic resonance imaging. Magn Reson Imaging Clin N Am. (2022) 30:565–82. doi: 10.1016/j.mric.2022.05.005
40. Foo T, Vermilyea M, Xu M, Thompson P, Bai Y, Conte G, et al. Dedicated high-performance, lightweight, low-cryogen compact 3.0T MRI system for advanced brain imaging. In: Proceedings of the ISMRM. Singapore (2016).
41. Mohr C. The Siemens Ultra High-field program MAGNETOM Allegra and Trio 3T MR the next dimension in clinical and research MR systems. Siemens Magnetom Flash. (2002) 1:21–2.
42. Campbell-Washburn AE, Ramasawmy R, Restivo MC, Bhattacharya I, Basar B, Herzka DA, et al. Opportunities in interventional and diagnostic imaging by using high-performance low-field-strength MRI. Radiology. (2019) 293:384–93. doi: 10.1148/radiol.2019190452
43. Panther A, Thevathasen G, Connell IR, Yao Y, Wiens CN, Curtis AT, et al., editors. A dedicated head-only MRI scanner for point-of-care imaging. In: Proceedings of the 27th Annual Meeting of ISMRM. Montreal, QC (2019).
44. Stainsby JA, Bindseil GA, Connell IR, Thevathasan G, Curtis AT, Beatty PJ, et al. Imaging at 0.5 T with high-performance system components. In: Proceedings of the 27th Annual Meeting of ISMRM. Montreal, QC (2019).
45. Welch EB, By S, Chen G, Dyvorne H, Hugon C, McNulty C, et al. Use environments and clinical feasibility of portable point-of-care bedside brain MRI. In: Proceedings of the ISMRM. Virtual (2020).
46. McDaniel PC, Cooley CZ, Stockmann JP, Wald LL. Numerically optimized design for a low-cost, lightweight 86 mT whole-brain magnet. In: Proceedings of the ISMRM. Montreal, QC (2019).
47. Cooley CZ, Haskell MW, Cauley SF, Sappo C, Lapierre CD, Ha CG, et al. Design of sparse Halbach magnet arrays for portable MRI using a genetic algorithm. IEEE Trans Magn. (2018) 54:2751001. doi: 10.1109/TMAG.2017.2751001
48. Liu Y, Leong ATL, Zhao Y, Xiao L, Mak HKF, Tsang ACO, et al. A low-cost and shielding-free ultra-low-field brain MRI scanner. Nat Commun. (2021) 12:7238. doi: 10.1038/s41467-021-27317-1
49. McDaniel PC, Cooley CZ, Stockmann JP, Wald LL. The MR Cap: A single-sided MRI system designed for potential point-of-care limited field-of-view brain imaging. Magn Reson Med. (2019) 82:1946–60. doi: 10.1002/mrm.27861
50. O'Halloran R. SL, Inventors, InventorHyperfine Research Inc, Assignee. Low-Field Diffusion Weighted Imaging. US (2020). Available online at: https://hyperfine.io/
51. Fassbender K, Walter S, Liu Y, Muehlhauser F, Ragoschke A, Kuehl S, et al. “Mobile Stroke Unit” for hyperacute stroke treatment. Stroke. (2003) 34:e44. doi: 10.1161/01.STR.0000075573.22885.3B
52. Parker SA, Bowry R, Wu T-C, Noser EA, Jackson K, Richardson L, et al. Establishing the first mobile stroke unit in the United States. Stroke. (2015) 46:1384–91. doi: 10.1161/STROKEAHA.114.007993
53. Bowry R, Grotta JC, editors. Bringing Emergency Neurology to Ambulances: Mobile Stroke Unit. Seminars in Respiratory and Critical Care Medicine. New York, NY: Thieme Medical Publishers (2017).
54. Deoni SCL, Medeiros P, Deoni AT, Burton P, Beauchemin J, D'Sa V, et al. Development of a mobile low-field MRI scanner. Sci Rep. (2022) 12:5690. doi: 10.1038/s41598-022-09760-2
55. Arnold TC, Freeman CW, Litt B, Stein JM. Low-field MRI: clinical promise and challenges. Journal of Magnetic Resonance Imaging. (2023) 57:25–44. doi: 10.1002/jmri.28408
56. Lee RKL, Griffith JF, Lau YYO, Leung JHY, Ng AWH, Hung EHY, et al. Diagnostic capability of low- versus high-field magnetic resonance imaging for lumbar degenerative disease. Spine. (2015) 40:382–91. doi: 10.1097/BRS.0000000000000774
57. Arnold TC, Tu D, Okar SV, Nair G, By S, Kawatra KD, et al. Sensitivity of portable low-field magnetic resonance imaging for multiple sclerosis lesions. NeuroImage Clin. (2022) 35:103101. doi: 10.1016/j.nicl.2022.103101
58. Arnold TC, By S, Welch EB, et al. Monitoring hydrocephalus patients using portable, low-field MRI. In: Radiological Society of North America Scientific Assembly Annual Meeting. Chicago, IL: Chicago (2021).
59. Deoni SCL, Burton P, Beauchemin J, Cano-Lorente R, De Both MD, Johnson M, et al. Neuroimaging and verbal memory assessment in healthy aging adults using a portable low-field MRI scanner and a web-based platform: results from a proof-of-concept population-based cross-section study. Brain Struct Funct. (2023) 228:493–509. doi: 10.1007/s00429-022-02595-7
Keywords: MRI, portable MRI, neuroimaging, stroke, ICH, point-of-care (POC)
Citation: Shoghli A, Chow D, Kuoy E and Yaghmai V (2023) Current role of portable MRI in diagnosis of acute neurological conditions. Front. Neurol. 14:1255858. doi: 10.3389/fneur.2023.1255858
Received: 10 July 2023; Accepted: 06 September 2023;
Published: 29 September 2023.
Edited by:
Francesco Santini, University of Basel, SwitzerlandReviewed by:
Thomas Arnold, Subtle Medical, Inc., United StatesThomas Lindner, University of Hamburg, Germany
Copyright © 2023 Shoghli, Chow, Kuoy and Yaghmai. This is an open-access article distributed under the terms of the Creative Commons Attribution License (CC BY). The use, distribution or reproduction in other forums is permitted, provided the original author(s) and the copyright owner(s) are credited and that the original publication in this journal is cited, in accordance with accepted academic practice. No use, distribution or reproduction is permitted which does not comply with these terms.
*Correspondence: Vahid Yaghmai, dnlhZ2htYWkmI3gwMDA0MDtocy51Y2kuZWR1