- 1Department of Neurology and Rehabilitation Medicine, College of Medicine, University of Cincinnati, Cincinnati, OH, United States
- 2Department of Emergency Medicine, University of Cincinnati, Cincinnati, OH, United States
- 3Department of Rehabilitation, Exercise and Nutrition Sciences, College of Allied Health Sciences, University of Cincinnati, Cincinnati, OH, United States
- 4EDDI Lab—Early Detection of Degenerative Disorders and Innovative Solutions, Department of Environmental Health, University of Cincinnati, Cincinnati, OH, United States
Background: Walking and balance impairment are common sequelae of stroke and significantly impact functional independence, morbidity, and mortality. Adequate postural stability is needed for walking, which requires sufficient integration of sensory information between the visual, somatosensory, and vestibular centers. “Sensory reweighting” describes the normal physiologic response needed to maintain postural stability in the absence of sufficient visual or somatosensory information and is believed to play a critical role in preserving postural stability after stroke. However, the extent to which sensory reweighting successfully maintains postural stability in the chronic stages of stroke and its potential impact on walking function remains understudied.
Methods: In this cross-sectional study, fifty-eight community-dwelling ambulatory chronic stroke survivors underwent baseline postural stability testing during quiet stance using the modified Clinical test of Sensory Interaction in Balance (mCTSIB) and assessment of spatiotemporal gait parameters.
Results: Seventy-six percent (45/58) of participants showed sufficient sensory reweighting with visual and somatosensory deprivation for maintaining postural stability, albeit with greater postural sway velocity indices than normative data. In contrast, survivors with insufficient reweighting demonstrated markedly slower overground walking speeds, greater spatiotemporal asymmetry, and limited acceleration potential.
Conclusion: Adequate sensory system reweighting is essential for chronic stroke survivors’ postural stability and walking independence. Greater emphasis should be placed on rehabilitation strategies incorporating multisensory system integration testing and strengthening as part of walking rehabilitation protocols. Given its potential impact on outcomes, walking rehabilitation trials may benefit from incorporating formal postural stability testing in design and group stratification.
Introduction
Post-stroke walking impairment significantly reduces functional ambulatory independence and is a significant cause of worldwide morbidity and mortality (1, 2). Commonly referred to as the sixth vital sign (3), walking speed is a crucial measure of post-stroke walking impairment, a determinant of functional independence levels, and a predictor of life expectancy (4). In the same vein, stroke survivors often experience balance impairment as a functional sequela and are at an increased risk of falls throughout the continuum of recovery during standing and walking (5, 6). Consequently, stroke survivors with balance impairment often experience low confidence in walking—leading to the loss of life roles, social isolation, dependency, a sedentary lifestyle, and an increased risk of falls, fractures, and secondary medical complications (7). While walking and standing balance have inherent functional and biomechanical differences, one of many key similarities is their dependence on postural stability (8, 9).
Postural stability is the ability to maintain the center of gravity inside one’s base of support through discrete synergies of trunk and leg muscles and is activated both in quiet stance and during motion (10–12). Evidence suggests that the visual, somatosensory (proprioception, joint, cutaneous), and vestibular systems centrally mediate sensorineural integration and processing, and that this sensory integration is critical for maintaining static and dynamic postural stability (8). While the preferred sensory system for maintaining postural stability in non-disabled adults is somatosensory input; the visual and vestibular centers are believed to play complementary roles in resolving conflicting sensory input (13). The subconscious ability to enhance the influence of one type of sensory input to compensate for a decrease or absence of information from another sensory center is termed “sensory reweighting (14, 15).” This phenomenon enables neurologically intact persons to maintain postural stability during visual or somatosensory deprivation. However, after neurologic injury, sensory reweighting is often impaired in the acute stages of stroke and is believed to improve over time—thereby improving motor control and postural stability (16–18). Therefore, it is possible that insufficiencies in sensory reweighting could lead to sustained postural instability, increase the risk of falls, and impact walking performance.
While progress has been made over the past three decades to characterize the inner workings of sensory system adaptations post-stroke (19–23), critical knowledge gaps remain. For example, though stroke survivors are believed to undergo reweighting (14, 24), the extent to which this process sufficiently facilitates postural stability is unknown. In addition, the downstream impact of postural stability on walking capacity measures has been under-reported. Furthermore, the most essential, if any, of the three sensory centers remains unsettled. For example, past work by Bonan and colleagues examined postural stability during quiet stance in 40 chronic stroke survivors and found that stroke survivors relied excessively on visual input to maintain posture (25, 26). In contrast, using a similar study posturography paradigm, Oliveira et al. reported that somatosensation was more critical for postural stability in their cohort (N = 21) (14). Yet, others have reported that visual input and somatosensation play equal roles (5, 27–29). The variations in viewpoints may, in part, result from the vast heterogenicity in baseline comorbidities, stroke lesion size, location, chronicity, the trajectory of recovery, variations in rehabilitation interventions, and social determinants such as access to rehabilitation and community involvement. Nonetheless, further investigations into reweighting and sensorineural integration and its impact on walking function in the chronic stages of stroke recovery are essential to advance the collective understanding of factors influencing balance and walking outcomes.
Hence, the objectives of this study were: (1) to characterize sensory reweighting in cohort of chronic stroke survivors (≥6 months post-stroke) and (2) to determine the relationship between postural stability and walking speed. We hypothesized that chronic stroke survivors would have greater postural instability across all four conditions of the modified Clinical test of Sensory Interaction in Balance (mCTSIB) compared to normative data. Second, participants with the most significant postural instability or the overt loss of balance (non-completion) would demonstrate greater walking impairment (decreased walking speed and acceleration potentials and increased spatiotemporal asymmetry).
Methods
Participants
Fifty-eight community-dwelling, ambulatory chronic stroke survivors (mean age: 56.9 ± 8.79, Female = 29) who had undergone baseline testing in two walking rehabilitation trials (NCT04553198 and NCT04721860) at the University of Cincinnati Neurorecovery Lab from August 2020 to February 2023 were included in this study. According to the Declaration of Helsinki recommendations, all participants provided written informed consent prior to enrollment. Inclusion criteria were: 18–80 years of age, residual walking impairment secondary to ischemic/hemorrhagic stroke(s) and ambulating at least 10 meters without a walker. All participants were able to walk independently without assistance of another person (with or without orthosis) or with an assistive device (cane, quad cane). In addition, all participants were expected to have abstained from formal physiotherapy and botulinum toxin injections at least 2 weeks before the screening visit (30). Exclusion criteria were unstable cardiovascular status precluding participation in a moderate-high intensity exercise, an adverse health condition that might affect walking capacity (other than stroke), severe lower extremity spasticity (modified Ashworth > 2/4), or significant language barrier which may interfere with the ability to follow instructions during testing. The baseline demographics of study participants are highlighted in Table 1. For the purposes of this study, it is worth noting that the current recovery and rehabilitation guidelines identify chronic stroke as individuals who are ≥6 months (without an upper limit), with the idea that most of the recovery will occur between the first 6 months, and to a lesser extent thereafter (31).
Outcome measures
Postural Stability
The mCTSIB is an established and reliable instrument for measuring static posture during quiet stance (10, 32, 33). It consists of four conditions designed to measure the relative contribution of each sensory center (visual, somatosensory, and vestibular) in maintaining postural stability during quiet stance in the presence or absence of manipulations to the other sensory systems. The output measure for each mCTSIB condition was the sway velocity index (SVI, degree of sway/s), representing the mean velocity of center of gravity sway during that condition, with higher values representing greater instability.
Task
While on the Biodex Balance Platform™ (Biodex Medical Systems, Shirley NY, United States), with a safety harness and without body weight support or use of balance aids, participants were asked to stand with their hands at their sides, in comfortable double limb stance with shoes approximately shoulder width apart. The test was performed under the four different conditions of the mCTSIB, with each trial lasting 30 s, see Figure 1A. Conditions were: 1 (eyes opened, fixed supporting surface = all sensory modalities are operational); 2 (eyes closed, fixed supporting surface = absence of visual input = somatosensory and vestibular systems unperturbed); 3 (eyes open, foam surface = perturbed somatosensory input = visual and vestibular systems unperturbed); 4 (eyes closed, foam surface = absence of visual input and perturbed somatosensory input = only vestibular function unperturbed; Figure 1B). Participants were allowed to use their prescribed orthosis as a safety measure. Three trials of each condition were performed concurrently and then averaged to determine the mean SVI. In addition, a designation of “non-complete” was given and documented for participants that needed immediate discontinuation or halting of one or more conditions on the mCTSIB due to excessive loss of balance (i.e., falls) or in instances when the side rails were emergently held to avoid falling. Such instances did not generate SVI values and were marked as “non-complete.” Nonetheless, the designation of “non-complete” provided the opportunity to evaluate for potential differences in baseline characteristics and walking capacity between this group vs. stroke survivors who completed all four mCTSIB conditions.
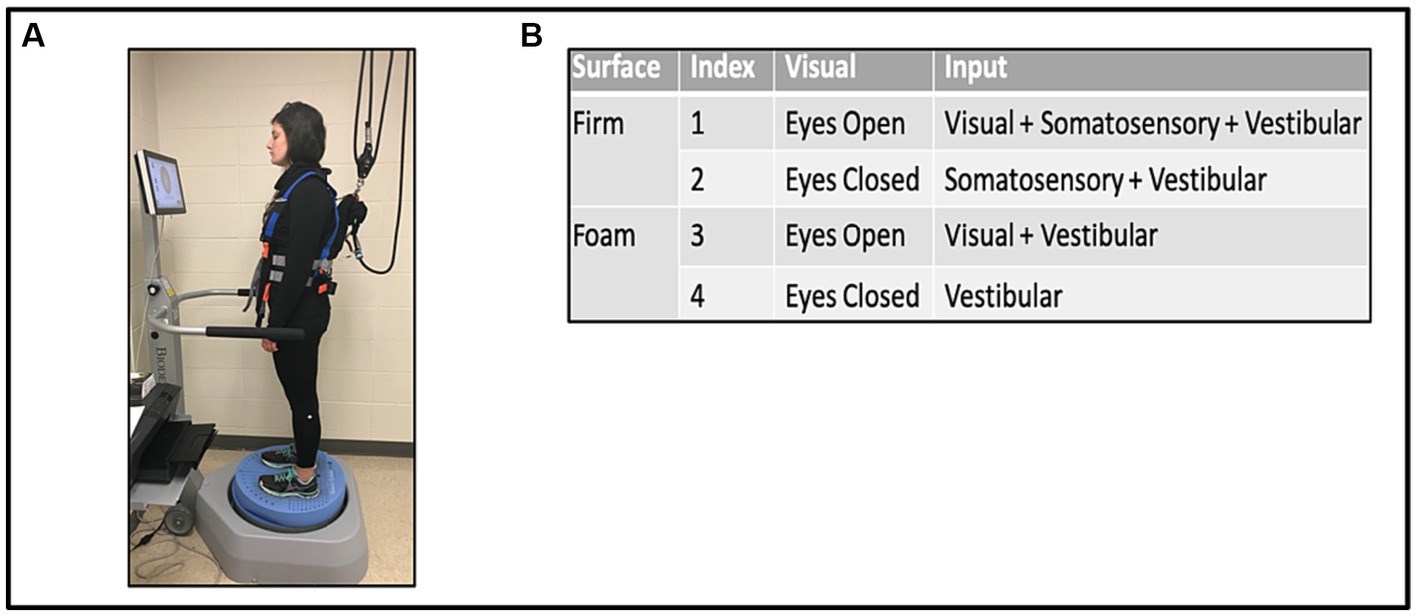
Figure 1. Modified clinical test of sensory interaction in balance (mCTSIB) with the Biodex Balance System™ (A), and the four conditions tested (B).
Walking speed
The 10-meter walk test (10 MWT) is the gold standard measure of post-stroke walking function reflecting overall mobility and health status (34).
Task
A trained physical therapist used a handheld stopwatch to time participants over the 10-meter walkway to obtain the self-selected (SS) and fast-paced (FP) walking speeds. A two-meter space was provided prior to the starting and ending markers to allow for acceleration and deceleration, respectively. The timing started when the participant’s lead leg broke the plane of the starting marker and stopped when both legs crossed the marker at the end of the path. Participants were allowed to use their home assistive device and orthosis. First, participants were asked to walk at their SS speed. Next, they were asked to walk at their FP speed without running. There were two trials for each walking condition, and the respective results were averaged for analysis.
SS walking speed is an universally accepted measure for classifying walking impairment level (household/severe vs. community ambulator/Mild–moderate) (35), while FP walking speed is often associated with a greater risk of imbalance and falls in the elderly (36). We also calculated walking acceleration potential (FP-SS), where a difference of <0.2 m/s has been associated with significant imbalance among chronic stroke survivors (37).
Spatiotemporal symmetry during overground walking
Decreased paretic limb contribution to standing balance control has been associated with greater walking asymmetry and increased likelihood of falls due to the limited capacity of the paretic limb to respond to instability (38). Specific to this study, spatiotemporal testing allowed the opportunity to detect differences in spatial and temporal symmetry in walking performance between participants who were able to complete all four conditions of the mCTSIB (i.e., complete) compared with those who were unsuccessful at completing all four conditions (i.e., “non-complete”)—suggestive of insufficient sensory reweighting. Therefore, to test the relationship between insufficient sensory reweighting and lower limb gait symmetry, the average lower extremity single support time (temporal) and step length (spatial) symmetry were collected at the same time as the SS and FP 10 MWT trials using the ProtoKinetics ZenoTM Walkway Gait Analysis System (ProtoKinetics LLC, Havertown, PA, United States). The following equation was used for measuring spatial and temporal symmetry during the 10 MWT: [1 − | Paretic – Non-paretic | / (Paretic + Non-paretic)] * 100%, where the integer values for the respective measure, based on the limb (i.e., paretic or nonparetic) are inputted. Possible symmetry values range from 0% to 100%, where 100% means perfect symmetry and 0% means complete asymmetry (39).
Statistics
A total of 58 participants were included in the analysis. The Kolmogorov–Smirnov test was used to assess for deviations from normal distribution among the continuous variables. The means and standard deviations are given, and confidence intervals are reported for all measures. Univariate linear regression using walking speed (variable-dependent), SVI (variable-independent), and non-completion (dichotomous-independent) were performed to determine the relationship between performance on each of the four test conditions and SS and FP walking speeds. The normative SVI value from the general population (N = 2,195, Ages: 13–84) provided by Biodex was used for comparison (40). Multiple student t-tests were performed to evaluate differences in SVI between respective conditions on the mCTSIB between our study cohort and normative data, as well as for comparing baseline differences in characteristics between those that were able to complete all four conditions relative to those marked “non-complete” for one or more condition. Chi-square and Fisher’s Exact tests were used only to compare categorical variables between both groups. A significance level was set at p ≤ 0.05 for all measures.
Results
Postural stability during quiet stance
Condition #1: All 58 participants completed the eyes-opened, fixed support surface condition testing the contributions of visual, somatosensory, and vestibular systems combined on postural stability. Chronic stroke survivors experienced greater SVI (Mean ± SD: 1.05 ± 0.73) compared to normative data (0.44 ± 0.48), p < 0.0001 (95% CI: 0.48, 0.74), see Figure 2.
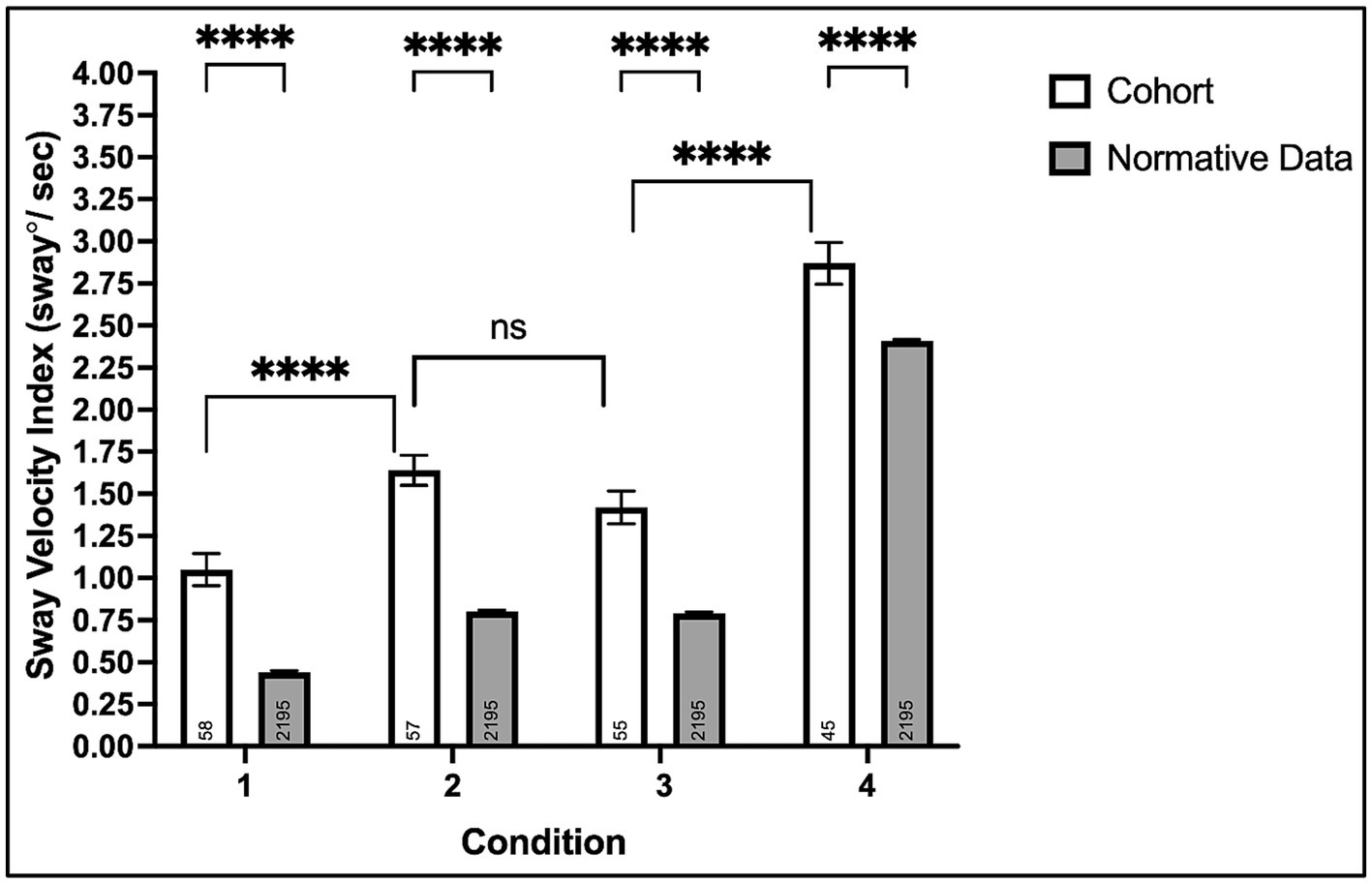
Figure 2. Mean sway velocity index on the modified clinical test of sensory interaction in balance with the Biodex Balance System™. Non-significant (ns, p > 0.05) and ****p < 0.0001.
Condition #2: Fifty-seven of fifty-eight (98%) participants completed the eyes-closed, fixed support surface condition testing somatosensory and vestibular systems’ contributions to postural balance in the absence of visual input. Chronic stroke survivors experienced greater SVI (1.64 ± 0.67) compared to normative data (0.80 ± 0.44), p < 0.0001 (0.72, 0.96).
Condition #3: Fifty-five of fifty-eight (95%) participants completed the eyes-opened foam surface condition, testing visual and vestibular systems’ contributions to postural stability when somatosensory information is perturbed. Chronic stroke survivors experienced greater SVI (1.42 ± 0.72) compared to normative data (0.79 ± 0.43), p < 0.0001 (0.51, 0.75). Although three participants could not complete condition #3 (compared to only one for condition #2), the SVI was comparable between both conditions, p = 0.10 (−0.04, 0.48).
Condition #4: Forty-five of fifty-eight (78%) participants completed the eyes-closed foam surface condition testing the contributions of the vestibular system alone and the relative dependence of vision and somatosensation on postural stability. Chronic stroke survivors experienced greater SVI (2.87 ± 0.83) compared to normative data (2.41 ± 0.38), p < 0.0001 (0.34, 0.58).
Walking speed
All 58 study participants completed the walking speed trials. Of these, 25 (43%) required the use of an assistive device during walking (12 cane, 13 quad cane), including 15 who used an ankle foot orthosis (AFO). The average group SS walking speed was 0.62 ± 0.32 m/s and 0.89 ± 0.50 m/s for FP. The average group acceleration potential (FP-SS Speed) was 0.27 ± 0.21 m/s.
Details demonstrating walking speed differences between Completers and Non-completers on mCTSIB, are in Table 2.
Temporal and spatial symmetry during walking
The average lower extremity temporal symmetry [single support time (s)] during SS and FP walking was 83.7% ± 10.4% and 84.6% ± 11.0%, respectively. The average lower extremity spatial symmetry [step length (cm)] was 89.0% ± 10.5% during SS and 89.0% ± 12.3% during FP walking. Details showing differences in spatiotemporal walking patterns between Completers and Non-completers on mCTSIB, are in Table 2.
Postural stability and walking speed
The relationship between SVI and walking speed was assessed in participants who completed the posturography task: condition #1 (n = 58), #2 (n = 57), #3 (n = 55), #4 (n = 45). There was a weak negative association between SVI in condition #1 and each walking speed: SS [Intercept: 0.75, slope: −0.13 (−0.26, −0.00), p = 0.05, R2 = 0.07] and FP [1.35, −0.33 (−0.66, 0.00), p = 0.05, R2 = 0.07]. Otherwise, there were no associations between SVI in conditions #2–4 and walking speed.
Non-completion of mCTSIB and walking performance
There was no association between non-completion of condition #2 and overground walking speed [SS: 0.93 m/s, 0.08 (−0.03, 0.19), p = 0.14, R2 = 0.04; FP: 0.94 m/s, 0.03 (−0.02, 0.12), p = 0.15, R2 = 0.04]. However, there was a weak association between non-completion of condition #3 and walking speed [SS: 0.81 m/s, 0.21 (0.03, 0.39), p = 0.02, R2 = 0.10; FP: 0.83 m/s, 0.14 (0.02, 0.25), p = 0.02, R2 = 0.09], and a moderate association for non-completion of condition #4 [SS: 0.25, 0.13 (0.59–1.12), p < 0.0001, R2 = 0.43; FP: 0.29, 0.54 (0.37, 0.72), p < 0.0001, R2 = 0.42]. The average speed was faster for participants completing all four mCTSIB conditions [0.73 ± 0.27 m/s (SS) and 1.06 ± 0.43 (FP)] compared to participants unable to complete one or more conditions [0.23 ± 0.12 (SS) and 0.29 ± 0.17 (FP)], <0.0001(0.35, 0.66), <0.0001 (0.52, 1.02). Participants unable to complete one or more conditions of the mCTSIB were at or below the established threshold (<0.4 m/s) for self-selected walking speed necessary for community ambulation (X2 = 49.7, <0.0001) (35), see Figure 3. Furthermore, the acceleration difference between FP and SS walking speeds was significantly lower for non-completers (0.06 ± 0.07 m/s) than completers (0.33 ± 0.20 m/s), <0.0001 (0.1563–0.3837). Non-completers also demonstrated more significant spatiotemporal asymmetry during SS and FP walking, see Table 2.
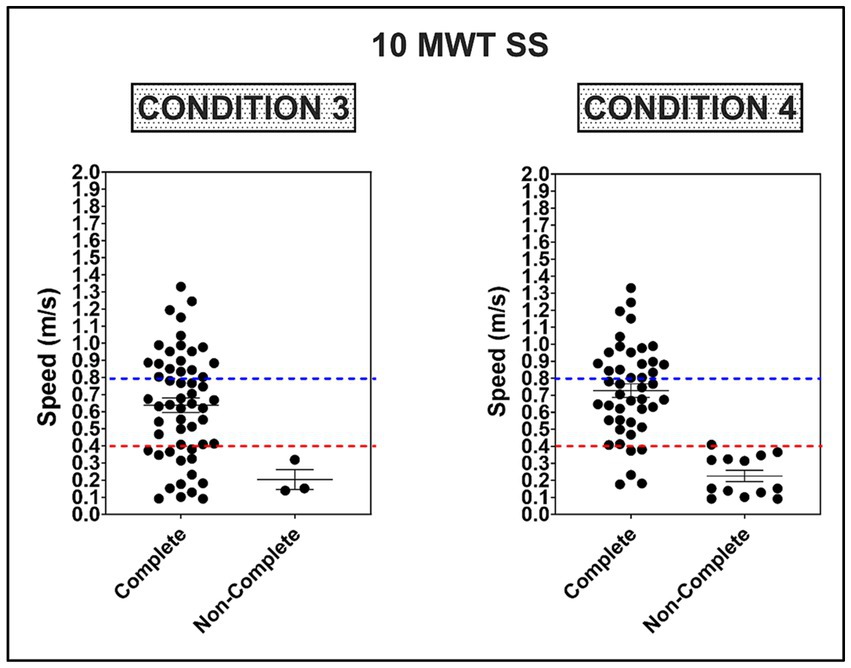
Figure 3. Average self-selected (SS) walking speed on the 10 m walk test (10 MWT) as a function of completing Conditions 3 and 4 on the mCTSIB. Dotted red and blue lines represent the threshold for limited (≥0.4–0.8 m/s) and full community ambulation (≥0.8 m/s), respectively (35).
Discussion
This study aimed to test postural stability during quiet stance and determine differences in visual and somatosensory reliance in our cohort of chronic stroke survivors. We also aimed to determine the relationship between postural stability and overground walking performance. Compared to normative data, we found significantly greater postural instability across all conditions in chronic stroke survivors. In addition, we found an increased reliance on visual and somatosensory systems in chronic stroke survivors, based on comparable SVI values in both conditions 2 and 3 of the mCTSIB, and higher SVI values in condition 4, when both are absent or perturbed, see Figure 2. While there was little to no significant association between the degree of postural instability and walking speed, we report that stroke survivors who had difficulty completing one or more conditions of the mCTSIB were more likely to have severe walking impairment, characterized by SS walking speed less than 0.4 m/s (35). Furthermore, non-completers had strikingly greater walking spatiotemporal asymmetries and diminished acceleration potential. To our knowledge, this study is the first to report the association between performance on specific components of quantitative sensorineural integration testing and overground walking outcomes in chronic stroke survivors. Furthermore, this study is the first to report post-stroke sensory reweighting insufficiency (non-completers) on the mCTSIB and its consequential influence on walking capacity.
In this observational study, all participants were able to complete condition #1 of the mCTSIB, suggesting that the cumulative input of the visual, somatosensory, and vestibular inputs was sufficient to prevent falls during quiet stance in the chronic stages of stroke recovery, irrespective of other factors associated with balance such as cognition, sensorimotor impairment, musculoskeletal strength, and coordination. In addition, there was a small negative association between the SVI and self-selected and fast walking speeds, suggesting that individuals with the greatest SVI under condition #1 are more likely to be slower walkers. We found no association between the SVI and walking speed in conditions #2–4. The absence of this relationship is likely due to the variability in sensory system integrity and implicit strategies used to maintain postural control across study participants. Another possible confounder is that participants with the greatest instability (non-completers) did not generate SVI values for inclusion into the correlation analysis, thereby decreasing the sample size and restricting the distribution of SVI values. Moreover, while postural stability during quiet standing and walking has many similarities, one possibility is that additional biomechanical variables not apparent during quiet stance, such as the shifting of the center of mass and motor activation time, become more relevant during walking (41, 42).
Although there have been conflicting reports on sensory system reweighting in the chronic stages of stroke (14, 26), we found that vision and somatosensation were essential for maintaining postural stability in our cohort—consistent with findings from others in the literature, suggesting that both sensory processing centers can play a key role in post-stroke sensory reweighting (28, 29). This conclusion is supported by the presence of greater SVI in condition #4 (compared with SVI in conditions # 1–3) and the increase in the incidence of non-completions in the absence of accurate visual and somatosensory input. Of note, while it is presumed that the greater SVIs and non-completions were a direct result of the disruption of visual and somatosensory input, an alternate perspective is the post-stroke vestibular hypofunction (43, 44). While none of our study participants had evidence of clinical vestibular dysfunction, previous reports have suggested that stroke survivors with lesions involving the vestibular cortex may experience insufficiency (45, 46). Indeed, the factors driving post-stroke sensory reweighting patterns are complex and likely involve several neuropathologic, neuroplastic, and musculoskeletal variables (24). Uncovering the mediators of inter-individual differences in sensory reweighting patterns in post-stroke is underexplored and warrants further investigation in future hypothesis-driven mechanistic studies.
Interestingly, 88% of our study cohort and 77% of non-completers had supratentorial strokes, as opposed to brainstem and cerebellar strokes—regions classically associated with postural control and balance. Recent reports have shed light on the importance of the human vestibular cortex in motor control, postural stability, and walking outcomes (47–51). Specifically, the parieto-insular vestibular cortex (PIVC) of the human parietal operculum has been suggested to play a vital role in postural control, somatosensory awareness, and central to the function of the vestibular cortex (45, 52, 53). However, its role in predicting balance and walking outcomes after stroke remains unknown. Thus, future studies investigating the PIVC and associated networks as a recovery biomarker may help advance our collective understanding and ability to predict balance and walking recovery outcomes, optimize clinical triaging, and better target rehabilitative interventions.
While task completers and non-completers were similar in characteristics such as age, chronicity of stroke, cognition, and stroke location, we found that the inability to complete one or more of the subcomponents of the mCTSIB was associated with slower walking speeds, greater spatiotemporal asymmetry, and diminished acceleration potential. This finding has both quality of life (54, 55) and rehabilitative implications. Notably, a growing body of work suggests that stroke survivors with severe walking impairment have limited responsiveness to walking rehabilitation interventions compared to survivors with SS walking speeds ≥ 0.4 m/s (55–59)—important considerations for clinical trial design and protocol optimization.
Furthermore, this study found that non-completers were more likely to have had a hemorrhagic rather than an ischemic stroke. However, this finding warrants further investigation in a larger prospective study, as the impact that the stroke subtype (ischemic vs. hemorrhagic) on recovery and rehabilitation remains unsettled (60, 61). Moreover, non-completers were more likely to use walking aids for stability during walking and demonstrated more significant spatiotemporal asymmetry. To this end, the degree of motor impairment, due to its influence on the base of support and stepping reaction patterns during stance and walking (62), may help explain the relationship between non-completion as a result of excessive postural instability (in the absence of somatosensory and visual feedback) and severe walking impairment. Nevertheless, 100% of participants were able to maintain postural stability on Condition #1, and 98% for Condition #2, irrespective of motor impairment status.
Lastly, given possible associations between mCTISB completion and other clinical measures (i.e., walking and clinical balance impairment measures), one may infer that such measures can be used in lieu of the mCTISB. However, the mCTSIB provides valuable and objective information on sensorineural integration and reweighting not attainable with other measures. In addition, mCTSIB with posturography provides invaluable continuous data which can be tracked over the continuum of recovery and used to determine the effects of neurorehabilitative interventions on postural stability and walking outcomes.
Limitations
Since this study was observational and did not adjust for multiple comparisons, future prospective rehabilitation studies with age-matched controls are needed to validate our findings. Furthermore, while there was no reported or clinically evident vestibular dysfunction in our cohort, formal vestibulo-ocular reflex testing was not performed in this study, therefore the influence of subclinical vestibular dysfunction could not be entirely ruled out and limits the interpretation of our study findings beyond what is reported. Additionally, as designed, the study did not include non-ambulatory stroke survivors; therefore, our findings are not generalizable to that population. Moreover, while this study included many of the standard variables associated with post-stroke balance and walking, additional measures such as lower extremity motor impairment levels, lesion size, structural network integrity of critical pathways involved in sensorimotor integration, and the inclusion of ascending and descending electrophysiological measures may better predict the determiners of outcome. In addition, investigations into how center-of-pressure patterns unfold over time (e.g., nonlinear time-series analysis) may help to elucidate adaptability to changing contextual conditions and transfer between different functional tasks.
Conclusion
Findings from this cross-sectional study in ambulatory chronic stroke survivors report insufficiencies in sensory systems processing at this stage of recovery and suggest that further consideration should be given to rehabilitation strategies incorporating multisensory system integration testing and strengthening as part of walking rehabilitation protocols. In addition, future walking rehabilitation trials should consider incorporating sensorineural processing measures to evaluate their prognostic potential.
Data availability statement
The original contributions presented in the study are included in the article/supplementary material, further inquiries can be directed to the corresponding author.
Ethics statement
The studies involving humans were approved by University of Cincinnati College of Medicine Institutional Board Review. The studies were conducted in accordance with the local legislation and institutional requirements. Written informed consent for participation in this study was provided by the participants’ legal guardians/next of kin. Written informed consent was obtained from the individual(s) for the publication of any potentially identifiable images or data included in this article.
Author contributions
OA, AG, CD, HS, PB, EW, ME, and KD: conception and design of the study, acquisition of data, or analysis and interpretation of data. OA, HS, SS, PB, AB, KD, PK, and BK: drafting the article or revising it critically for important intellectual content. All authors contributed to the article and approved the submitted version.
Funding
This work was supported by the University of Cincinnati Gardner Institute and the American Academy of Neurology Career Development Award funded this study.
Acknowledgments
The authors thank the study participants for their time and effort.
Conflict of interest
OA reports grant support from the American Academy of Neurology Institute Career Development Award. PB reports support from the National Institutes of Health under grant R01HD093694. SS reports support from the Foundation for Physical Therapy Research Promotion of Doctoral Studies (PODS) II Scholarship, supported by the Rhomberger Fund.
The remaining authors declare that the research was conducted in the absence of any commercial or financial relationships that could be construed as a potential conflict of interest.
Publisher’s note
All claims expressed in this article are solely those of the authors and do not necessarily represent those of their affiliated organizations, or those of the publisher, the editors and the reviewers. Any product that may be evaluated in this article, or claim that may be made by its manufacturer, is not guaranteed or endorsed by the publisher.
References
2. Rajsic, S, Gothe, H, Borba, HH, Sroczynski, G, Vujicic, J, Toell, T, et al. Economic burden of stroke: a systematic review on post-stroke care. Eur J Health Econ. (2018) 20:107–34. doi: 10.1007/s10198-018-0984-0
3. Middleton, A, Fritz, SL, and Lusardi, M. Walking speed: the functional vital sign. J Aging Phys Act. (2015) 23:314–22. doi: 10.1123/japa.2013-0236
4. Cummings, SR, Studenski, S, and Ferrucci, L. A diagnosis of Dismobility—giving mobility clinical visibility. JAMA. (2014) 311:2061–2. doi: 10.1001/jama.2014.3033
5. Tyson, SF, Hanley, M, Chillala, J, Selley, A, and Tallis, RC. Balance disability after stroke. Phys Ther. (2006) 86:30–8. doi: 10.1093/ptj/86.1.30
6. Harris, JE, Eng, JJ, Marigold, DS, Tokuno, CD, and Louis, CL. Relationship of balance and mobility to fall incidence in people with chronic stroke. Phys Ther. (2005) 85:150–8. doi: 10.1093/ptj/85.2.150
7. Schmid, AA, Puymbroeck, M, Altenburger, PA, Dierks, TA, Miller, KK, Damush, TM, et al. Balance and balance self-efficacy are associated with activity and participation after stroke: a cross-sectional study in people with chronic stroke. Arch Phys Med Rehabil. (2012) 93:1101–7. doi: 10.1016/j.apmr.2012.01.020
8. Takakusaki, K. Neurophysiology of gait: from the spinal cord to the frontal lobe. Mov Disord. (2013) 28:1483–91. doi: 10.1002/mds.25669
9. Saradjian, AH. Sensory modulation of movement, posture and locomotion. Neurophysiol Clin. (2015) 45:255–67. doi: 10.1016/j.neucli.2015.09.004
10. Shumway-Cook, A, and Horak, FB. Assessing the influence of sensory interaction of balance. Suggestion from the field. Phys Ther. (1986) 66:1548–50. doi: 10.1093/ptj/66.10.1548
11. Paillard, T. Relationship between sport expertise and postural skills. Front Psychol. (2019) 10:1428. doi: 10.3389/fpsyg.2019.01428
12. Geurts, ACH, Haart, M, IJW, N, and Duysens, J. A review of standing balance recovery from stroke. Gait Posture. (2005) 22:267–81. doi: 10.1016/j.gaitpost.2004.10.002
13. Wolpert, DM, Diedrichsen, J, and Flanagan, JR. Principles of sensorimotor learning. Nat Rev Neurosci. (2011) 12:739–51. doi: 10.1038/nrn3112
14. Oliveira, CB, Medeiros, ÍR, Greters, MG, Frota, NAF, Tavares Lucato, L, Scaff, M, et al. Abnormal sensory integration affects balance control in hemiparetic patients within the first year after stroke. Clinics. (2011) 66:2043–8. doi: 10.1590/S1807-59322011001200008
15. Barrett, MM, Doheny, EP, Setti, A, Maguinness, C, Foran, TG, Kenny, RA, et al. Reduced vision selectively impairs spatial updating in fall-prone older adults. Multisens Res. (2013) 26:69–94. doi: 10.1163/22134808-00002412
16. Woollacott, M, and Shumway-Cook, A. Attention and the control of posture and gait: a review of an emerging area of research. Gait Posture. (2002) 16:1–14. doi: 10.1016/S0966-6362(01)00156-4
17. Abbruzzese, G, and Berardelli, A. Sensorimotor integration in movement disorders. Mov Disord. (2003) 18:231–40. doi: 10.1002/mds.10327
18. Helbostad, JL, Askim, T, and Moe-Nilssen, R. Short-term repeatability of body sway during quiet standing in people with hemiparesis and in frail older adults. Arch Phys Med Rehabil. (2004) 85:993–9. doi: 10.1016/j.apmr.2003.07.020
19. Celnik, P, Hummel, F, Harris-Love, M, Wolk, R, and Cohen, LG. Somatosensory stimulation enhances the effects of training functional hand tasks in patients with chronic stroke. Arch Phys Med Rehabil. (2007) 88:1369–76. doi: 10.1016/j.apmr.2007.08.001
20. Conforto, BA, Ferreiro, NK, Tomasi, C, dos Santos, R, Moreira, VL, Marie, SK, et al. Effects of somatosensory stimulation on motor function after subacute stroke. Neurorehabil Neural Repair. (2009) 24:263–72. doi: 10.1177/1545968309349946
21. Smania, N, Picelli, A, Gandolfi, M, Fiaschi, A, and Tinazzi, M. Rehabilitation of sensorimotor integration deficits in balance impairment of patients with stroke hemiparesis: a before/after pilot study. Neurol Sci. (2008) 29:313. doi: 10.1007/s10072-008-0988-0
22. Bolognini, N, Russo, C, and Edwards, DJ. The sensory side of post-stroke motor rehabilitation. Restor Neurol Neurosci. (2016) 34:1–16. doi: 10.3233/RNN-150606
23. Brown, KE, Neva, JL, Feldman, SJ, Staines, WR, and Boyd, LA. Sensorimotor integration in chronic stroke: baseline differences and response to sensory training. Restor Neurol Neurosci. (2018) 36:245–59. doi: 10.3233/RNN-170790
24. Bonan, IV, Marquer, A, Eskiizmirliler, S, Yelnik, AP, and Vidal, P. Sensory reweighting in controls and stroke patients. Clin Neurophysiol. (2013) 124:713–22. doi: 10.1016/j.clinph.2012.09.019
25. Bonan, IV, Yelnik, AP, Colle, FM, Michaud, C, Normand, E, Panigot, B, et al. Reliance on visual information after stroke. Part II: effectiveness of a balance rehabilitation program with visual cue deprivation after stroke: a randomized controlled trial. Arch Phys Med Rehabil. (2004) 85:274–8. doi: 10.1016/j.apmr.2003.06.016
26. Bonan, IV, Colle, FM, Guichard, JP, Vicaut, E, Eisenfisz, M, Tran Ba Huy, P, et al. Reliance on visual information after stroke. Part I: balance on dynamic posturography. Arch Phys Med Rehabil. (2004) 85:268–73. doi: 10.1016/j.apmr.2003.06.017
27. Keenan, MA, Perry, J, and Jordan, C. Factors affecting balance and ambulation following stroke. Clin Orthop. (1984) 182:165–71. doi: 10.1097/00003086-198401000-00021
28. Lee, HH, and Jung, SH. Prediction of post-stroke falls by quantitative assessment of balance. Ann Rehabil Med. (2017) 41:339–46. doi: 10.5535/arm.2017.41.3.339
29. Niam, S, Cheung, W, Sullivan, PE, Kent, S, and Gu, X. Balance and physical impairments after stroke. Arch Phys Med Rehabil. (1999) 80:1227–33. doi: 10.1016/S0003-9993(99)90020-5
30. Jacinto, J, Varriale, P, Pain, E, Lysandropoulos, A, and Esquenazi, A. Patient perspectives on the therapeutic profile of botulinum neurotoxin type a in spasticity. Front Neurol. (2020) 11:388. doi: 10.3389/fneur.2020.00388
31. Bernhardt, J, Hayward, KS, Kwakkel, G, Ward, NS, Wolf, SL, Borschmann, K, et al. Agreed definitions and a shared vision for new standards in stroke recovery research: the stroke recovery and rehabilitation roundtable taskforce. Int J Stroke. (2017) 12:444–50. doi: 10.1177/1747493017711816
32. Antoniadou, E, Kalivioti, X, Stolakis, K, Koloniari, A, Megas, P, Tyllianakis, M, et al. Reliability and validity of the mCTSIB dynamic platform test to assess balance in a population of older women living in the community. J Musculoskelet Neuronal Interact. (2020) 20:185–93.
33. Peller, A, Garib, R, Garbe, E, Komforti, D, Joffe, C, Magras, A, et al. Validity and reliability of the NIH toolbox® standing balance test as compared to the biodex balance system SD. Physiother Theory Pract. (2023) 39:827–33. doi: 10.1080/09593985.2022.2027584
34. Schmid, A, Duncan, PW, Studenski, S, Lai, SM, Richards, L, Perera, S, et al. Improvements in speed-based gait classifications are meaningful. Stroke. (2007) 38:2096–100. doi: 10.1161/STROKEAHA.106.475921
35. Perry, J, Garrett, M, Gronley, JK, and Mulroy, SJ. Classification of walking handicap in the stroke population. Stroke. (1995) 26:982–9. doi: 10.1161/01.STR.26.6.982
36. Callisaya, ML, Blizzard, L, McGinley, JL, and Srikanth, VK. Risk of falls in older people during fast-walking – the TASCOG study. Gait Posture. (2012) 36:510–5. doi: 10.1016/j.gaitpost.2012.05.003
37. Middleton, A, Braun, CH, Lewek, MD, and Fritz, SL. Balance impairment limits ability to increase walking speed in individuals with chronic stroke. Disabil Rehabil. (2016) 39:1–6. doi: 10.3109/09638288.2016.1152603
38. Mansfield, A, Wong, JS, McIlroy, WE, Biasin, L, Brunton, K, Bayley, M, et al. Do measures of reactive balance control predict falls in people with stroke returning to the community? Physiotherapy. (2015) 101:373–80. doi: 10.1016/j.physio.2015.01.009
39. Boyne, P, Doren, S, Scholl, V, Staggs, E, Whitesel, D, Carl, D, et al. Preliminary outcomes of combined treadmill and overground high-intensity interval training in ambulatory chronic stroke. Front Neurol. (2022) 13:812875. doi: 10.3389/fneur.2022.812875
40. Overman, D. Biodex balance system SD and BioSway devices receive enhancements. Rehab Manag. (2019).
41. MacKinnon, CD. Sensorimotor anatomy of gait, balance, and falls. Handb Clin Neurol. (2018) 159:3–26. doi: 10.1016/b978-0-444-63916-5.00001-x
42. Mansfield, A, Inness, EL, and Mcilroy, WE. Chapter 13 stroke. Handb Clin Neurol. (2018) 159:205–28. doi: 10.1016/b978-0-444-63916-5.00013-6
43. Hall, CD, Herdman, SJ, Whitney, SL, Anson, ER, Carender, WJ, Hoppes, CW, et al. Vestibular rehabilitation for peripheral vestibular hypofunction: an updated clinical practice guideline from the academy of neurologic physical therapy of the american physical therapy association. J Neurol Phys Ther. (2022) 46:118–77. doi: 10.1097/NPT.0000000000000382
44. Dougherty, JM, Carney, M, Hohman, MH, and Emmady, PD. Vestibular dysfunction In: StatPearls. Treasure Island. FL: StatPearls Publishing (2023).
45. Yeo, S, Jang, S, and Kwon, J. Central vestibular disorder due to ischemic injury on the parieto-insular vestibular cortex in patients with middle cerebral artery territory infarction. Medicine. (2017) 96:e9349. doi: 10.1097/MD.0000000000009349
46. Eguchi, S, Hirose, G, and Miaki, M. Vestibular symptoms in acute hemispheric strokes. J Neurol. (2019) 266:1852–8. doi: 10.1007/s00415-019-09342-9
47. Ferrè, E, Bottini, G, and Haggard, P. Vestibular inputs modulate somatosensory cortical processing. Brain Struct Funct. (2012) 217:859–64. doi: 10.1007/s00429-012-0404-7
48. Lopez, C, Blanke, O, and Mast, FW. The human vestibular cortex revealed by coordinate-based activation likelihood estimation meta-analysis. Neuroscience. (2012) 212:159–79. doi: 10.1016/j.neuroscience.2012.03.028
49. Ferrè, ER, Day, BL, Bottini, G, and Haggard, P. How the vestibular system interacts with somatosensory perception: a sham-controlled study with galvanic vestibular stimulation. Neurosci Lett. (2013) 550:35–40. doi: 10.1016/j.neulet.2013.06.046
50. Ibitoye, RT, Mallas, E, Bourke, NJ, Kaski, D, Bronstein, AM, and Sharp, DJ. The human vestibular cortex: functional anatomy of OP2, its connectivity and the effect of vestibular disease. Cereb Cortex. (2022) 33:567–82. doi: 10.1093/cercor/bhac085
51. Ingemanson, ML, Rowe, JR, Chan, V, Wolbrecht, ET, Reinkensmeyer, DJ, and Cramer, SC. Somatosensory system integrity explains differences in treatment response after stroke. Neurology. (2019) 92:e1098–108. doi: 10.1212/WNL.0000000000007041
52. Park, S, Yeo, S, Jang, S, Cho, I, and Oh, S. Associations between injury of the parieto-insular vestibular cortex and changes in motor function according to the recovery process: use of diffusion tensor imaging. Front Neurol. (2021) 12:740711. doi: 10.3389/fneur.2021.740711
53. Brandt, T, and Dieterich, M. The vestibular cortex: its locations, functions, and disorders. Ann N Y Acad Sci. (1999) 871:293–312. doi: 10.1111/j.1749-6632.1999.tb09193.x
54. Langhorne, P, Stott, DJ, Robertson, L, MacDonald, J, Jones, L, McAlpine, C, et al. Medical complications after stroke. Stroke. (2000) 31:1223–9. doi: 10.1161/01.STR.31.6.1223
55. Duncan, PW, Sullivan, KJ, Behrman, AL, Azen, SP, Wu, SS, Nadeau, SE, et al. Body-weight–supported treadmill rehabilitation after stroke. N Engl J Med. (2011) 364:2026–36. doi: 10.1056/NEJMoa1010790
56. Boyne, P, Billinger, SA, Reisman, DS, Awosika, OO, Buckley, S, Burson, J, et al. Optimal intensity and duration of walking rehabilitation in patients with chronic stroke. JAMA Neurol. (2023) 80:342–51. doi: 10.1001/jamaneurol.2023.0033
57. Awosika, OO, Chan, D, Sucharew, HJ, Boyne, P, Bhattacharya, A, Dunning, K, et al. Backward locomotor treadmill training differentially improves walking performance across stroke walking impairment levels. Brain Sci. (2022) 12:133. doi: 10.3390/brainsci12020133
58. Plummer, P, Behrman, AL, Duncan, PW, Spigel, P, Saracino, D, Martin, J, et al. Effects of stroke severity and training duration on locomotor recovery after stroke: a pilot study. Neurorehabil Neural Repair. (2007) 21:137–51. doi: 10.1177/1545968306295559
59. Dean, CM, Ada, L, and Lindley, RI. Treadmill training provides greater benefit to the subgroup of community-dwelling people after stroke who walk faster than 0.4m/s: a randomised trial. J Physiother. (2014) 60:97–101. doi: 10.1016/j.jphys.2014.03.004
60. Oosterveer, DM, Wermer, MJH, Volker, G, and Vlieland, TPMV. Are there differences in long-term functioning and recovery between hemorrhagic and ischemic stroke patients receiving rehabilitation? J Stroke Cerebrovasc Dis. (2022) 31:106294. doi: 10.1016/j.jstrokecerebrovasdis.2021.106294
61. Kitago, T, and Ratan, RR. Rehabilitation following hemorrhagic stroke: building the case for stroke-subtype specific recovery therapies. F1000Res. (2017) 6:2044. doi: 10.12688/f1000research.11913.1
Keywords: walking, stroke, balance, sensorineural integration, sensory reweighting, gait, outcome measures, stroke recovery
Citation: Awosika OO, Garver A, Drury C, Sucharew HJ, Boyne P, Schwab SM, Wasik E, Earnest M, Dunning K, Bhattacharya A, Khatri P and Kissela BM (2023) Insufficiencies in sensory systems reweighting is associated with walking impairment severity in chronic stroke: an observational cohort study. Front. Neurol. 14:1244657. doi: 10.3389/fneur.2023.1244657
Edited by:
Jana Kimijanová, Slovak Academy of Sciences (SAS), SlovakiaReviewed by:
Barbora Kolářová, University Hospital Olomouc, CzechiaSujin Hwang, Baekseok University, Republic of Korea
Copyright © 2023 Awosika, Garver, Drury, Sucharew, Boyne, Schwab, Wasik, Earnest, Dunning, Bhattacharya, Khatri and Kissela. This is an open-access article distributed under the terms of the Creative Commons Attribution License (CC BY). The use, distribution or reproduction in other forums is permitted, provided the original author(s) and the copyright owner(s) are credited and that the original publication in this journal is cited, in accordance with accepted academic practice. No use, distribution or reproduction is permitted which does not comply with these terms.
*Correspondence: Oluwole O. Awosika, oluwole.awosika@uc.edu