- Department of MRI, The First Affiliated Hospital of Harbin Medical University, Harbin, China
Objective: Transient ischemic attack is a significant risk factor for acute cerebral infarction. Previous studies have demonstrated that hypoperfusion in patients with transient ischemic attack was associated with the recurrence of transient ischemic attack, stroke, and persistent worsening of neurological symptoms. Moreover, transient ischemic attack patients classified as high-risk group according to the ABCD2 score have a higher incidence of stroke. Therefore, the objective of this study was to investigate the plaque characteristics of transient ischemic attack patients with concomitant cerebral hypoperfusion using multimodal MRI, as well as hemodynamic changes in the high-risk group with transient ischemic attack patients.
Materials and methods: A total of 151 patients with transient ischemic attack were prospectively recruited for this study. All enrolled patients underwent multimodal MRI, including DWI, TOF-MRA, HR-VWI, and DSC-PWI. Finally, 56 patients met the inclusion criteria. Based on DSC-PWI images, patients were divided into two groups: hypoperfusion (n = 41) and non-hypoperfusion (n = 15). Clinical baseline characteristics and plaque characteristics were analyzed between the two groups. Furthermore, within the hypoperfusion group, patients were further classified into low-risk (n = 11) and high-risk (n = 30) subgroups based on the ABCD2 score. Hemodynamic differences between these subgroups were also analyzed.
Results: Compared with the non-hypoperfusion group, the hypoperfusion group had a significantly higher prevalence of hypertension (68.3% vs. 33.3%, p = 0.019) and hyperhomocysteinemia (65.9% vs. 33.3%, p = 0.029). Moreover, the hypoperfusion group exhibited more significant luminal stenosis degree [41.79
Conclusion: Transient ischemic attack patients with hypoperfusion exhibited a higher prevalence of hypertension and hyperhomocysteinemia, as well as higher luminal stenosis degree, and greater NWI. Furthermore, Transient ischemic attack patients in the high-risk group demonstrated higher MTT.
Introduction
Transient ischemic attack (TIA) is an episode of transient neurological dysfunction without evidence of acute cerebral infarction (1). In Asian populations, intracranial atherosclerotic disease, particularly middle cerebral artery (MCA) atherosclerosis, is the most common cause of acute ischemic stroke and TIA (2, 3). Approximately half of TIA patients experience a stroke within the first 48 h, and the rate of stroke within 90 days can reach 10%–15% (4). Therefore, rapid and effective risk stratification is crucial in identifying TIA patients who may be at risk of ischemic stroke at an early stage. This will enable clinicians to prioritize high-risk patients who require urgent management. In clinical, TIA is a significant risk factor for acute cerebral infarction. Studies have shown that the progressive development of intracranial atherosclerotic plaques leads to the narrowing of the blood vessel lumen, ultimately resulting in a decrease in cerebral blood flow (5). Meanwhile, decreased cerebral perfusion in TIA patients, even in the absence of an acute infarct lesion, may be associated with the recurrence of TIA, stroke, and persistent worsening of neurological symptoms (6). Additionally, the ABCD2 score (named according to five factors: age, blood pressure, clinical characteristics, symptom duration, and diabetes) is a widely used risk prediction tool to determine the risk of early progression to ischemic stroke in TIA patients (1, 7). The ABCD2 score has been found to be positively correlated with the incidence of cerebral infarction within 1 week after TIA, meaning that the higher the score, the higher the incidence of cerebral infarction in the short term (8). Therefore, TIA patients are divided into a low-risk (≤3 points) group and a high-risk (≥4 points) group according to the ABCD2 score (1). Elucidating the mechanisms and predictors of hypoperfusion in TIA patients and understanding the hemodynamics in patients at high risk for TIA is critical.
High-resolution vessel wall imaging (HR-VWI) is widely used to assess the morphology of intracranial atherosclerotic plaques, allowing noninvasive visualization of the wall and lumen of the MCA. It helps explore the distribution characteristics and anatomy of the plaque, aiding in understanding the pathophysiological features of atherosclerosis (9). HR-VWI can also help identify high-risk patients who require intensive treatment by measuring atherosclerotic plaque burden and instability, as well as monitor treatment effects (10). Furthermore, dynamic susceptibility contrast perfusion-weighted imaging (DSC-PWI) is a unique dynamic imaging technique that changes the magnetization rate of tissue and, thus, the strength of the MRI signal over time by intravenous contrast injection to observe the intracranial hemodynamic state (11). Multimodal MRI imaging provides an essential foundation for understanding the pathogenesis and risk stratification of patients with TIA, enabling the development of individualized treatment plans for precision medicine.
The differences in plaque features between ischemic stroke and TIA patients, as well as symptomatic and asymptomatic patients, have been extensively studied (12–14). However, there have been few studies comparing the distinctions in plaque characteristics between hypoperfusion and non-hypoperfusion in TIA patients. It remains unclear whether plaque characteristics differ in TIA patients with and without hypoperfusion. Therefore, the objective of this study was to investigate the plaque characteristics of TIA patients with concomitant cerebral hypoperfusion using multimodal MRI, as well as hemodynamic changes in the high-risk group with TIA patients.
Materials and methods
Study participants and clinical data
The study protocol was approved by the Ethics Committee of The First Hospital of Harbin Medical University All patients provided either written or verbal consent to participate in the study. The study was conducted in accordance with the Declaration of Helsinki (as revised in 2013). Prospective inclusion of patients with atherosclerotic TIA in the MCA, treated at The First Hospital of Harbin Medical University, took place from October 2021 to July 2023 The inclusion criteria were as follows: (1) age 18 years; (2) DWI images were negative; (3) patients had multiple (≥2) TIA symptoms in the past, with each attack lasting no more than 24 h; (4) contralateral limb numbness or weakness at the MCA stenosis in TIA patients; (5) MR examination performed within 7 days after onset; (6) presence of at least one atherosclerotic risk factor, such as hypertension, diabetes mellitus, dyslipidemia, alcohol consumption, or smoking. The exclusion criteria were as follows: (1) acute cerebral infarction confirmed by DWI; (2) contraindications to MRI enhancement; (3) previous history of cerebral infarction, cerebral hemorrhage, brain surgery, and cerebrovascular intervention; (4) combined ipsilateral carotid artery (ICA) stenosis 50%; (5) not due to atherosclerotic diseases, such as dissection, vasculitis, moyamoya disease, or cardiogenic embolism; (6) poor MRI image quality that prevented further analysis.
Clinical baseline characteristics and multimodal MR imaging of the patients were collected for the patients. According to the DSC-PWI images, the patients were divided into two groups: hypoperfusion and non-hypoperfusion. Furthermore, within the hypoperfusion group, segmented into low-(0–3) and high-(4–7) risk groups were performed based on the ABCD2 score (1).
Demographic and clinical characteristics were collected from clinical records, including age, gender, BMI, history of hypertension, diabetes, total cholesterol, triglycerides, LDL, HDL, and hyperhomocysteinemia. Hypertension was defined as self-reported history of hypertension, mean blood pressure ≥ 140/90 mmHg, or taking anti-hypertensive medication (15); diabetes was defined as self-reported use of insulin or oral hypoglycemic drugs, or HbA1c ≥ 6.5% (16); total cholesterol ≥ 5.2 mmol/L, triglycerides ≥ 1.7 mmol/L, LDL ≥ 3.7 mmol/L, HDL ≤ 1.04 mmol/L, or self-reported history of dyslipidemia (17), and hyperhomocysteinemia defined as >15 mol/L (18). History of smoking: ≥1 cigarette per day for six consecutive months; history of alcohol consumption: >2 U for men and >1 U for women on average per day for the past year (19).
MRI data acquisition
A 3.0 T MRI scanner (Achieva; Philips Healthcare, The Netherlands) with a 32-channel phased-array coil was used. Patients were trained to breathe before scanning to reduce the effect of motion artifacts on image quality. The scanning protocol and parameters included diffusion-weighted imaging (DWI), TR/TE 21/3.45 ms, thickness 0.6 mm, Flip angle 20°, FOV 194 mm 194 mm; three-dimensional time-of-flight magnetic resonance angiography (3D-TOF-MRA), TR/TE 1,927/55 ms, thickness 4 mm, Flip angle 90°, FOV 230 mm 230 mm; HR-VWI, and DSC-PWI. HR -VWI including T1-weighted three-dimensional volumetric isotropic turbo spin echo acquisition (T1-3D-VISTA), TR/TE 800/18 ms, thickness 0.6 mm, Flip angle 90°, FOV 180 mm 144 mm; simultaneous non-contrast angiography and intraplaque hemorrhage (SNAP), TR/TE 10/5.8 ms, thickness 0.6 mm, Flip angle 11°, FOV 160 mm 160 mm; T2-weighted three-dimension volumetric isotropic turbo spin echo acquisition (T2-3D-VISTA), TR/TE 2,400/90 ms, thickness 0.6 mm, Flip angle 90°, FOV 250 mm 250 mm; and enhanced T1-3D-VISTA. The contrast agent was Gd-DTPA contrast, using 0.1 mL/kg. DSC-PWI, TR/TE 1,795/40 ms, thickness 0.6 mm, Flip angle 75°, FOV 224 mm 224 mm, was performed by injecting Gd (0.1 mL/kg) via the anterior elbow vein at a rate of 5 mL/s with a high-pressure syringe, followed by flushing 20 mL of saline at the same rate and acquiring planar gradient echoes every 2 s (T2*) images for the 60s.
MRI image analysis
Two radiologists with more than 2 years of diagnostic experience conducted qualitative and quantitative analyses of the images while remaining blind to the clinical data of all recruited patients. In case of any disagreements between the observers, a consensus was reached through a joint reading. All parameter measurements were performed on the Philips Intellispace Portal workstation. The quality of MR images was classified as poor, marginal, good, and excellent according to the signal-to-noise, and images graded as poor were excluded from the study. On HR-VWI images, atherosclerotic plaques exhibited eccentric thickening of the vessel wall (17). According to the symptoms of TIA, the location of diseased blood vessels (left/right MCA) was defined. Plaque was defined as eccentric thickening of MCA wall. A culprit plaque was defined as the layer of the maximum lumen narrowing (MLN) of the MCA (20, 21). The reference lumen was the plaque-free segment proximal or distal to the largest luminal stenosis. The short axial T1-3D-VISTA images were magnified to 200% and the outer wall area (OWA) and inter wall area (IWA) were manually measured at the MLN site and the reference site. Intraplaque hemorrhage (IPH) was defined when the signal intensity (SI) of the culprit plaque was higher than 150% of gray matter (22). All cross-sections with plaque were categorized in accordance with whether the plaque was centered on the superior, inferior, dorsal, or ventral side of the vessel. If a plaque was distributed between two quadrants, the quadrant with the thickest plaque was selected (23). The plaque measurement is as follows: wall area (WA) = OWA-IWA; plaque area (PA) was calculated as WAMLN-WAreference (24); the degree of stenosis = (1 − IWAMLN/IWAreference) 100% (12). The measurement of plaque burden is expressed by the normalized wall index (NWI), which is defined as WAMLN/OWAMLN 100% (25); remodeling index (RI) = OWAMLN/OWAreference (RI > 1.05 was defined as positive remodeling and RI < 0.95 was defined as negative remodeling) (21). Additionally, SI was quantified with manually drawn regions of interest on HR-VWI images. The signal intensity of gray matter (SIgraymatter) was measured by manually drawing a round region of 8–10 mm2 at the adjacent normal gray matter on matched pre and post-contrast T1-weighted images, respectively. The contrast enhancement ratio = [(SIpost-plaque/SIpost-graymatter) − (SIpre-plaque/SIpre-graymatter)]/(SIpre-plaque/SIpre-graymatter) 100% (26).
The DSC-PWI sequence was utilized to generate pseudo-color maps of region cerebral blood flow (rCBF), region cerebral blood volume (rCBV), mean transit time (MTT), and time to peak (TTP). It is challenging to compare hemodynamic parameters due to individual differences. Therefore, to avoid bias in the data, the relative rCBF (rrCBF), relative rCBV (rrCBV), relative MTT (rMTT), and relative TTP (rTTP) were calculated based on the affected/mirror contralateral side. Since there is no universally validated or consistent perfusion value for distinguishing risky from benign tissue. We based on previous research that, in the pseudo-color map, the hypoperfusion group was defined as reduced perfusion on the affected side compared with the contralateral side, with rrCBF < 75%. The non-hypoperfusion group was defined as no abnormal perfusion areas observed on the pseudo-color chart by visual observation (27, 28).
Statistical analysis
The statistical analysis of the data was performed using IBM SPSS 26.0 software. The Kolmogorov–Smirnov test was used to analyze the normality of continuous variables. Variables that followed a normal distribution were expressed as mean standard deviation (SD), and comparisons between groups were conducted using independent Student’s t-test. For variables with skewed distributions, median and interquartile range (IQR) were used, and Mann–Whitney U-test for comparison between groups. Categorical variables were represented as numbers and frequencies (%), and group comparisons were carried out using the chi-square test. In the chi-square test, if the expected count of any cell was less than 5, Pearson’s Chi-square test was applied. If any cell had an expected count was less than 5, a continuity correction test was used. If the minimum expected count was less than 1, Fisher’s exact test was employed The interobserver reproducibility of continuous variables was assessed using intra-group correlation coefficients (ICC) and the agreement was classified as very good (r > 0.75), good (r = 0.4–0.75), or poor (r < 0.4). Cohen’s kappa was utilized to determine the interobserver agreement in identifying plaque distribution. The p < 0.05 difference was statistically significant.
Results
Demographic and clinical information
The patient selection process is summarized in Figure 1. During the trial period, 151 patients with TIA were prospectively recruited for MRI. Among the recruited patients, 95 patients were excluded due to the following reasons: poor image quality (n = 30); ipsilateral ICA stenosis ≥ 50% (n = 15), moyamoya disease (n = 12), vasculitis (n = 14), cerebral hemorrhage (n = 9), dissection (n = 9), and incomplete of clinical information (n = 6). Ultimately, 56 patients with a mean age of 53.45 ± 11.86 years were included in this study. The hypoperfusion group consisted of 41 patients (mean age 55 years, 21 male), while the non-hypoperfusion group consisted of 15 patients (mean age 50 14 years, 10 male).
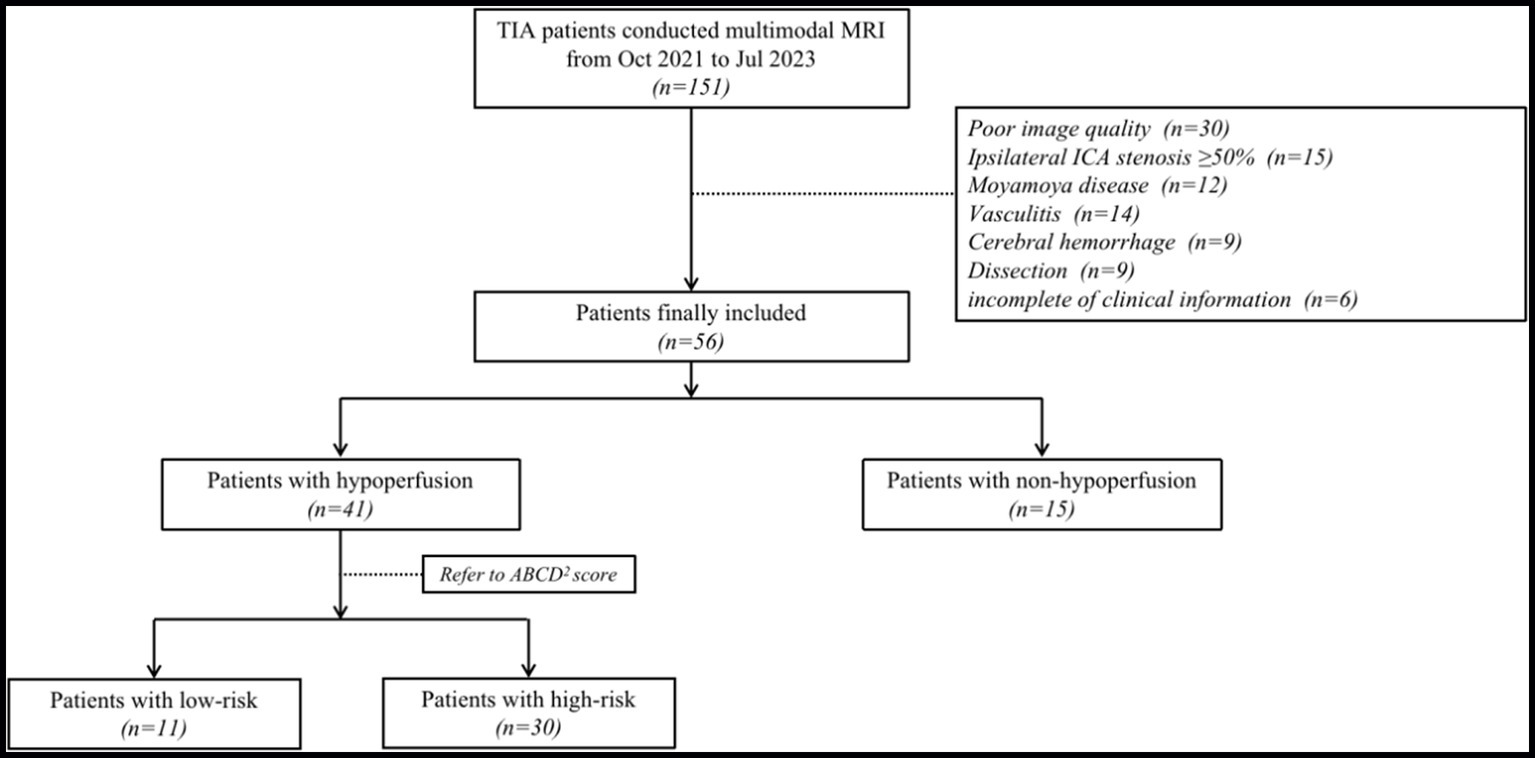
Figure 1. Flow chart showing process of study population selection. TIA, transient ischemic attack; ICA, internal carotid artery.
Among the 56 patients with TIA, the prevalence of hypertension was 58.92%, diabetes mellitus was 32.14%, hyperhomocysteinemia was 57.14%, and dyslipidemia was 37.5%. In addition, 20 patients (35.71%) had a history of smoking, and 13 patients (23.21%) had a history of alcohol consumption. In the hypoperfusion group, the proportion of patients with hypertension (68.3% vs. 33.3%) and hyperhomocysteinemia (65.9% vs. 33.3%) was significantly higher than that in the non-hypoperfusion group, and the difference was statistically significant (p = 0.019, p = 0.029). There were no significant differences between the two groups in terms of gender, age, BMI, diabetes, cholesterol, triglycerides, LDL, HDL, smoking, and alcohol consumption (p > 0.05). The clinical characteristics of TIA patients in the hypoperfusion and non-hypoperfusion groups were compared in Table 1.
Interreader reproducibilities
Good interreader reproducibilities were found: IWAMLN (ICC 0.997, 95% CI 0.994–0.998); IWAreference (ICC 0.91, 95% CI 0.985–0.995); OWAMLN (ICC 0.994, 95% CI 0.990–0.997); OWAreference (ICC 0.988, 95% CI 0.980–0.993); SIpre-plaque (ICC 0.954, 95% CI 0.923–0.973); SIpre-graymatter (ICC 0.878, 95% CI 0.801–0.927); SIpost-plaque (ICC 0.946, 95% CI 0.910–0.968); SIpost-graymatter (ICC 0.880, 95% CI 0.803–0.928); rrCBF (ICC 0.760, 95% CI 0.623–0.852); rrCBV (ICC 0.795, 95% CI 0.674–0.874); rMTT (ICC 0.802, 95% CI 0.684–0.879); rTTP (ICC 0.758, 95% CI 0.618–0.851); plaque distribution k = 0.818; IPH k = 0.707.
Plaque characteristics between hypoperfusion and non-hypoperfusion groups
Measurement of vessel and plaque characteristics in hypoperfusion and non-hypoperfusion is shown in Figures 2, 3, respectively. In the hypoperfusion group, both luminal stenosis (41.79% vs. 17.62%) and NWI (57.1 vs. 40.21) were significantly higher than in the non-hypoperfusion group, and the differences were statistically significant (p = 0.006,0.009). The remaining plaque characteristics did not significantly differ between the two groups (all p > 0.05). The comparison of plaque characteristics between the two groups is presented in Table 2. Figure 4 illustrates the differences in clinical baseline characteristics and plaque characteristics between the two groups.
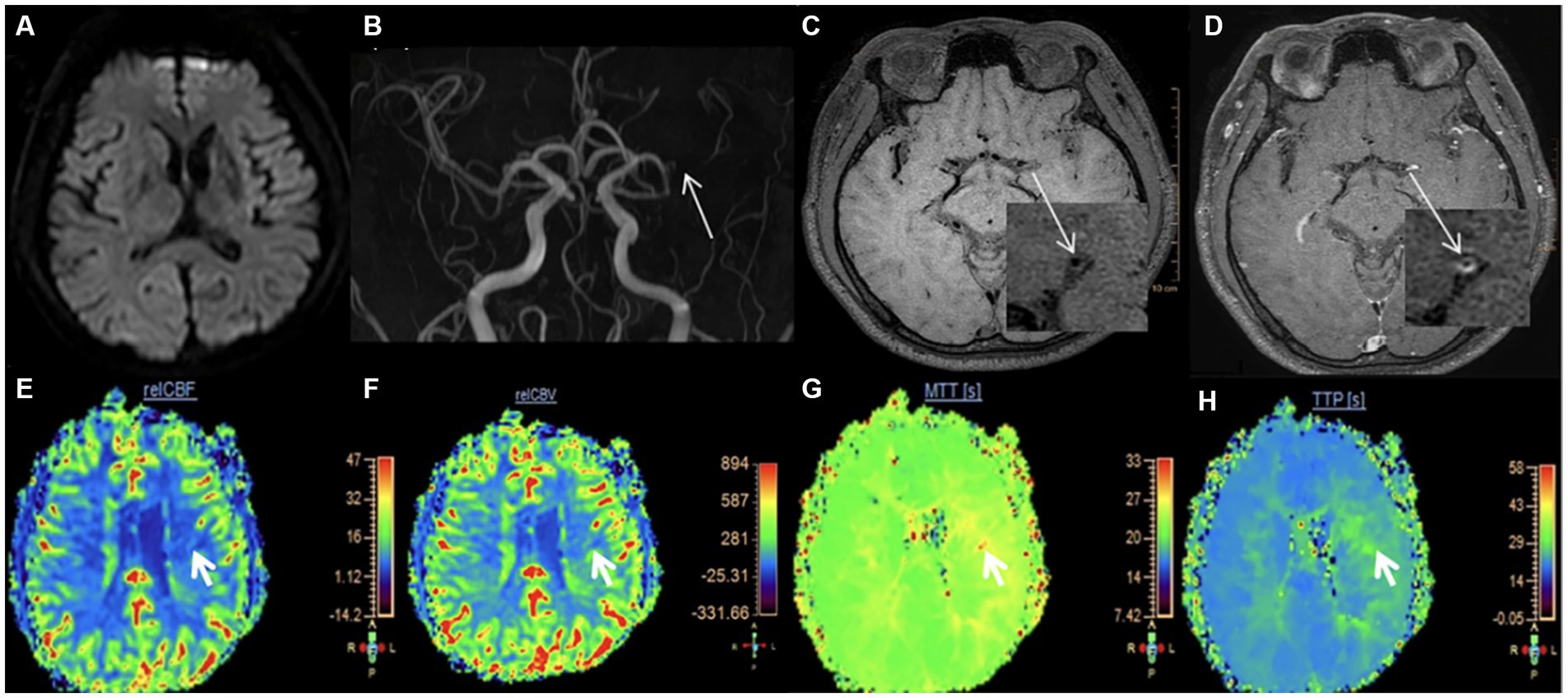
Figure 2. Measurement of vessel and plaque characteristics in hypoperfusion. A 32-year-old male was admitted to our hospital for 4 h due to right limb weakness. (A) DWI is normal; (B) The stenotic site of the left MCA. The white arrow shows the stenotic site of the left MCA; (C) T1-weighted HR-VWI imaging shows the plaque in the left MCA. The white arrow shows the short-axis view; (D) Enhanced T1-weighted HR-VWI imaging showing the measurement at the most narrowed site in left MCA. OWA = 2.04 mm2, IWA = 0.89 mm2. (E–H) The four pseudo-color maps of rCBF, rCBV, MTT, and TTP are shown respectively, with the white arrow indicating areas of hypoperfusion. rCBF = 5.86, rCBV = 156.84, MTT = 26.78 s, TTP = 26.84 s, rrCBF = 71.19%. DWI, diffusion-weighted imaging; MCA, middle cerebral artery; HR-VWI, high-resolution vessel wall imaging; OWA, outer wall area; IWA, inter wall area.
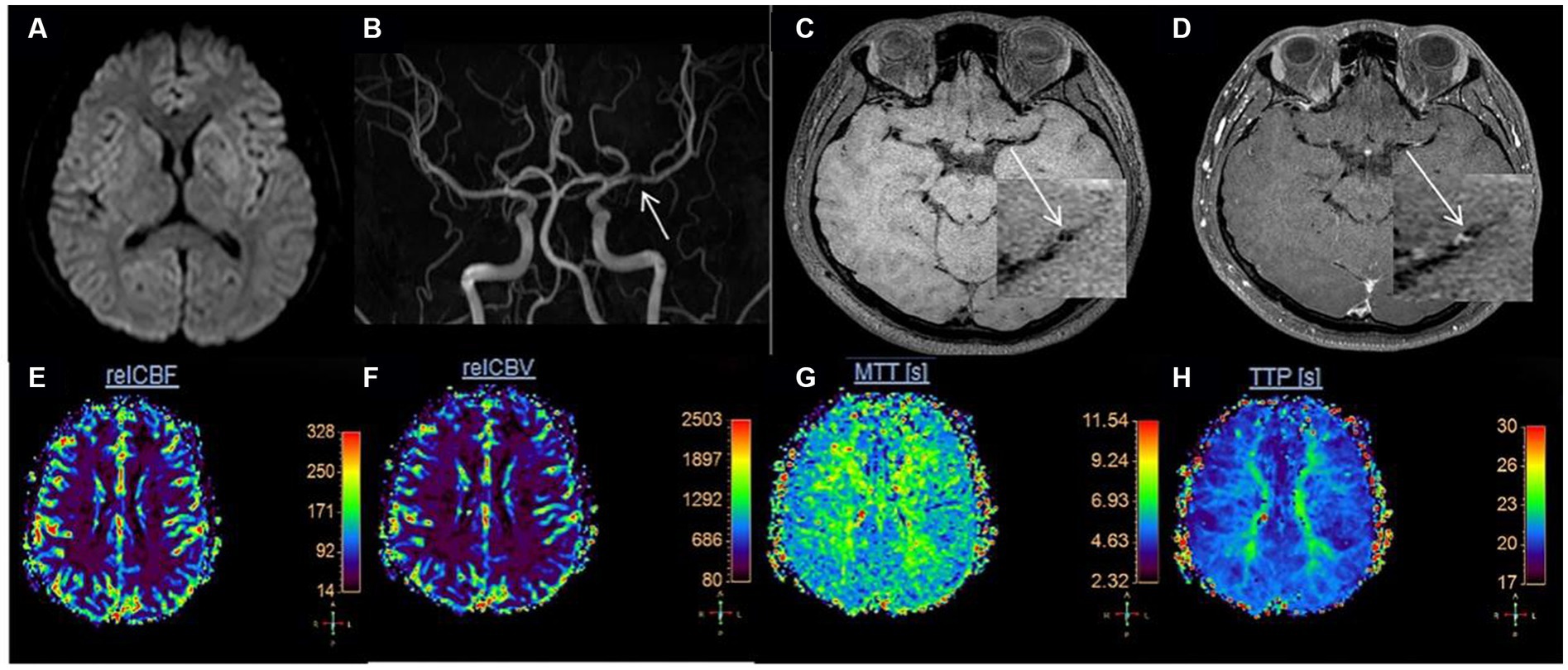
Figure 3. Measurement of vessel and plaque characteristics in non-hypoperfusion. Female, 59 years old, left upper limb weakness for more than 3 h. (A) DWI is normal; (B) The stenotic site of the left MCA. The white arrow shows the stenotic site of the left MCA; (C) T1-weighted HR-VWI imaging shows the plaque in the left MCA. The white arrow shows the short-axis view; (D) Enhanced T1-weighted HR-VWI imaging showing the measurement at the most narrowed site in left MCA. OWA = 5.19 mm2, IWA = 1.33 mm2. (E–H) Pseudo-color maps of rCBF, rCBV, MTT, and TTP were shown respectively, and none of them revealed the region of hypoperfusion. DWI, diffusion-weighted imaging; MCA, middle cerebral artery; HR-VWI, high-resolution vessel wall imaging; OWA, outer wall area; IWA, inter wall area.
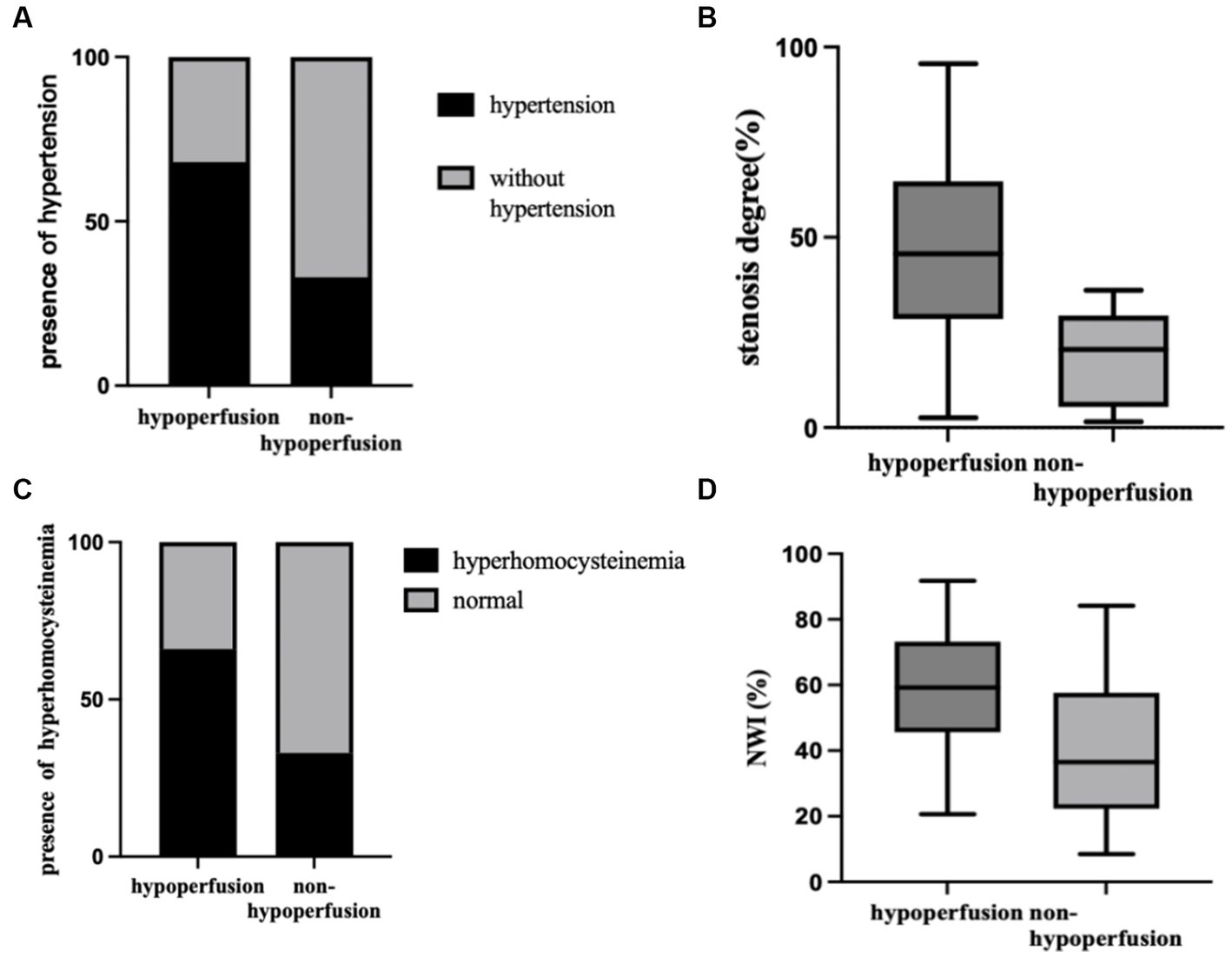
Figure 4. Comparison between hypoperfusion and non-hypoperfusion in prevalence of hypertension (A), hyperhomocysteinemia (B), stenosis degree (C), and NWI (D). All were statistically different. NWI, normalized wall index.
Comparison of hemodynamic between the high-risk and low-risk groups
Patients in the hypoperfusion group were divided into low-risk and high-risk groups according to the ABCD2 score. The rMTT was higher in the high-risk group [108.36(100.67–119.92)] than the low-risk group [117.6(109.31–128.14)], and the difference was statistically significant (p = 0.037). The hemodynamic comparison between the low-risk and high-risk groups is shown in Table 3.
Discussion
TIA serves as a vital warning indicator of impending stroke (29). Intracranial atherosclerotic significantly contributes to both vascular events (30). Prior studies have highlighted the persistent high risk faced by TIA patients in the early stages (31). Multimodal MRI enables the observation of changes in brain structure and function from various perspectives, facilitating the early identification of cerebral ischemic states and etiology. This, in turn, aids in the accurate stratification of risk among patients with cerebrovascular disease and helps identify high-risk groups for personalized and precise treatment to mitigate risk. To the best of our knowledge, this is the first study to use HR-VWI combined with DSC-PWI to assess plaque characteristics in TIA patients with reduced cerebral blood flow and examine hemodynamic changes in high-risk TIA patients.
Our results found that patients with hypoperfusion had a significantly higher prevalence of hypertension and hyperhomocysteinemia compared to those without hypoperfusion. These findings align with previous research (32, 33), and Wang et al. concluded that hypertension is an independent risk factor for perfusion abnormalities in TIA patients (34). Hypertension has a substantial impact on cerebral circulatory changes, as repeated mechanical stress, degradation of elastin fibers in the vascular wall, and alteration of vascular endothelial function or morphology caused by hypertension contribute to reduced cerebral perfusion through increased cerebrovascular resistance and impaired self-regulation (35). Another possible explanation is that angiotensin II (Ang II), the primary hormone of the renin-angiotensin system (RAS), plays a vital role in the pathophysiology of hypertension. Ang II affects cerebral blood flow through a cascade of vasoconstriction and endothelial dysfunction (36). In addition, hyperhomocysteinemia is associated with the formation of atherosclerotic plaque, platelet aggregation, and adhesion. It can also disrupt vascular endothelial cells, vascular elastic fibers, and flow regulation, adversely influencing vascular regeneration and circulation. Wang et al. have also discovered that hyperhomocysteinemia expression promotes vascular stenosis (37). Furthermore, this study revealed that patients with more significant stenosis are more likely to develop hypoperfusion. Proximal arterial stenosis can transmit the slowdown effects on blood flow to the downstream areas of the brain parenchyma; reduced cerebral blood flow leads to decreased perfusion (38). As a result, the higher the degree of luminal stenosis, the lower the cerebral blood flow and the higher the risk of ischemic cerebrovascular events. Consequently, TIA with luminal stenosis should be given high prioritized. Studies have demonstrated that the relationship between NWI and luminal stenosis is primarily linear (39). Moreover, Jia et al. have shown that NWI is the most effective index for evaluating the severity of atherosclerosis and more accurately reflects plaque progression (40). Additionally, NWI progression is a significant independent predictor of recurring symptomatic ischemic events. Therefore, according to these data, we can infer that a larger NWI in TIA patients is more likely to lead to adverse cerebrovascular events.
The ABCD2 score performed well in identifying patients at high risk of TIA and was frequently used to predict TIA recurrence (41, 42). DSC-PWI is a functional imaging approach that can sensitively reflect the blood perfusion of brain tissue and can also semi-quantitatively study the changes in blood flow. However, in patients with negative DWI and focal hypoperfusion on DSC-PWI, there was a high risk of stroke recurrence in the abnormal perfusion area after 7 days (43). Abnormal hemodynamics inhibit cerebrovascular self-regulation, decreasing vascular reserve and increasing the risk of stroke (44). Therefore, the brain’s ability to regulate blood flow to meet metabolic demands and compensate for cerebral perfusion is an important protective mechanism against the development of cerebral ischemia. In this study, the patients in the hypoperfusion group were divided into low-risk and high-risk groups based on the ABCD2 score, which helped clarify the hemodynamics of the high-risk group for early detection and timely treatment. This study found that both the high-risk group and the low-risk group experienced perfusion changes, which may be attributed to the decompensation of the blood supply in the cerebrovascular trunk, leading to a reduction in cerebral perfusion pressure through self-regulation and resulting in abnormal in local hemodynamics and a decrease in distal vascular resistance. As a result, collateral circulation compensation becomes the primary mechanism. Furthermore, the high-risk group exhibited more pronounced MTT prolongation compared to the low-risk group. MTT, defined as the time required for the contrast agent to pass through the microcirculation, is highly sensitive to perfusion differences (45). This suggests that the high-risk group had poorer microcirculation status. Therefore, it is further suggested that patients in the high-risk group may require more active and comprehensive interventions to maintain the normal metabolism of brain tissue and reduce the incidence of stroke when compared to patients in the low-risk group.
Limitation
The present study has several limitations. Firstly, the sample size of this study was small, and further studies with large sample sizes are warranted. Secondly, this is a single-center study conducted on an Asian population with a high prevalence of ICAD. Therefore, there may be a selection bias in this sample, and the findings may be less applicable to other populations. Finally, long-term followed-up of patients is necessary to determine the impact on the prevention and recurrence of TIA patients. Further research on race and ethnicity is also necessary.
Conclusion
Multimodal MRI may provide valuable insight into TIA patients. This study suggested that rMTT is higher in high-risk TIA patients and emphasizes the importance of considering hypertension, hyperhomocysteinemia, higher luminal stenosis degree, and greater NWI as high-priority factors. These findings contribute to the early diagnosis and individualized treatment of TIA patients, providing an additional clinical perspective.
Data availability statement
The original contributions presented in the study are included in the article/supplementary material, further inquiries can be directed to the corresponding author.
Ethics statement
The studies involving humans were approved by the First Hospital of Harbin Medical University Ethics Committee. The studies were conducted in accordance with the local legislation and institutional requirements. The participants provided their written informed consent to participate in this study. Written informed consent was obtained from the individual(s) for the publication of any potentially identifiable images or data included in this article.
Author contributions
YS, JS, and WW conceived and designed the research. YS acquired the data. YS, JS, and YC analyzed and interpreted the data. YS and YC performed the statistical analysis. WW handled the supervision. YS drafted the manuscript. All authors contributed to the article and approved the submitted version.
Conflict of interest
The authors declare that the research was conducted in the absence of any commercial or financial relationships that could be construed as a potential conflict of interest.
The reviewer BS declared a shared parent affiliation with the authors to the handling editor at the time of review.
Publisher’s note
All claims expressed in this article are solely those of the authors and do not necessarily represent those of their affiliated organizations, or those of the publisher, the editors and the reviewers. Any product that may be evaluated in this article, or claim that may be made by its manufacturer, is not guaranteed or endorsed by the publisher.
References
1. Easton, JD, Saver, JL, Albers, GW, Alberts, MJ, Chaturvedi, S, Feldmann, E, et al. Definition and evaluation of transient ischemic attack: a scientific statement for healthcare professionals from the American Heart Association/American Stroke Association stroke council; council on cardiovascular surgery and Anesthesia; council on cardiovascular radiology and intervention; council on cardiovascular nursing; and the interdisciplinary council on peripheral vascular disease. The American Academy of Neurology affirms the value of this statement as an educational tool for neurologists. Stroke. (2009) 40:2276–93. doi: 10.1161/STROKEAHA.108.192218
2. Zhang, DF, Chen, YC, Chen, H, Zhang, WD, Sun, J, Mao, CN, et al. A high-resolution MRI study of relationship between Remodeling patterns and ischemic stroke in patients with atherosclerotic middle cerebral artery stenosis. Front Aging Neurosci. (2017) 9:140. doi: 10.3389/fnagi.2017.00140
3. Yu, YN, Liu, MW, Villablanca, JP, Li, ML, Xu, YY, Gao, S, et al. Middle cerebral artery plaque hyperintensity on T2-weighted Vessel Wall imaging is associated with ischemic stroke. AJNR Am J Neuroradiol. (2019) 40:1886–92. doi: 10.3174/ajnr.A6260
4. Uehara, T, Minematsu, K, Ohara, T, Kimura, K, Okada, Y, Hasegawa, Y, et al. Incidence, predictors, and etiology of subsequent ischemic stroke within one year after transient ischemic attack. Int J Stroke. (2017) 12:84–9. doi: 10.1177/1747493016669884
5. Arteaga, DF, Strother, MK, Faraco, CC, Davis, LT, Scott, AO, and Donahue, MJ. Cerebral blood flow territory instability in patients with atherosclerotic intracranial stenosis. J Magn Reson Imaging. (2019) 50:1441–51. doi: 10.1002/jmri.26737
6. Nam, KW, Kim, CK, Ko, SB, Yoon, BW, Yoo, RE, and Sohn, CH. Regional arterial spin Labeling perfusion defect is associated with early ischemic recurrence in patients with a transient ischemic attack. Stroke. (2020) 51:186–92. doi: 10.1161/STROKEAHA.119.026556
7. Johnston, SC, Rothwell, PM, Nguyen-Huynh, MN, Giles, MF, Elkins, JS, Bernstein, AL, et al. Validation and refinement of scores to predict very early stroke risk after transient ischaemic attack. Lancet. (2007) 369:283–92. doi: 10.1016/S0140-6736(07)60150-0
8. Xi, HY, Si, ZH, Li, JC, Zhu, JG, and Yan, HY. Assessment of cerebral infarction after transient cerebral ischemic attack by ABCD2 score combined with the position of intracranial vascular stenosis. Medicine. (2019) 98:e15081. doi: 10.1097/MD.0000000000015081
9. de Havenon, A, Mossa-Basha, M, Shah, L, Kim, SE, Park, M, Parker, D, et al. High-resolution vessel wall MRI for the evaluation of intracranial atherosclerotic disease. Neuroradiology. (2017) 59:1193–202. doi: 10.1007/s00234-017-1925-9
10. Dieleman, N, Yang, W, Abrigo, JM, Chu, WCW, van der Kolk, AG, Siero, JCW, et al. Magnetic resonance imaging of plaque morphology, burden, and distribution in patients with symptomatic middle cerebral artery stenosis. Stroke. (2016) 47:1797–802. doi: 10.1161/STROKEAHA.116.013007
11. Kim, J, Leira, EC, Callison, RC, Ludwig, B, Moritani, T, Magnotta, VA, et al. Toward fully automated processing of dynamic susceptibility contrast perfusion MRI for acute ischemic cerebral stroke. Comput Methods Prog Biomed. (2010) 98:204–13. doi: 10.1016/j.cmpb.2009.12.005
12. Xiao, J, Padrick, MM, Jiang, T, Xia, S, Wu, F, Guo, Y, et al. Acute ischemic stroke versus transient ischemic attack: differential plaque morphological features in symptomatic intracranial atherosclerotic lesions. Atherosclerosis. (2021) 319:72–8. doi: 10.1016/j.atherosclerosis.2021.01.002
13. Song, JW, Pavlou, A, Xiao, J, Kasner, SE, Fan, Z, and Messé, SR. Vessel Wall magnetic resonance imaging biomarkers of symptomatic intracranial atherosclerosis: a meta-analysis. Stroke. (2021) 52:193–202. doi: 10.1161/STROKEAHA.120.031480
14. Lu, M, Zhang, L, Yuan, F, Peng, P, Zhang, H, Liu, S, et al. Comparison of carotid atherosclerotic plaque characteristics between symptomatic patients with transient ischemic attack and stroke using high-resolution magnetic resonance imaging. BMC Cardiovasc Disord. (2022) 22:190. doi: 10.1186/s12872-022-02624-7
15. Song, X, Zhao, X, Liebeskind, DS, Wang, L, Xu, W, Xu, Y, et al. Incremental value of plaque enhancement in predicting stroke recurrence in symptomatic intracranial atherosclerosis. Neuroradiology. (2020) 62:1123–31. doi: 10.1007/s00234-020-02418-8
16. Li, X, Sun, B, Wang, L, Zhang, J, Zhang, J, Zhao, Z, et al. Association of Type 2 diabetes mellitus and Glycemic control with intracranial plaque characteristics in patients with acute ischemic stroke. J Magn Reson Imaging. (2021) 54:655–66. doi: 10.1002/jmri.27614
17. Yan, X, Tang, M, Gao, J, Wang, L, Li, L, Ma, N, et al. Sex differences in intracranial atherosclerotic plaques among patients with ischemic stroke. Front Cardiovasc Med. (2022) 9:860675. doi: 10.3389/fcvm.2022.860675
18. Ganguly, P, and Alam, SF. Role of homocysteine in the development of cardiovascular disease. Nutr J. (2015) 14:6. doi: 10.1186/1475-2891-14-6
19. Sun, P, Liu, L, Pan, Y, Wang, X, Mi, D, Pu, Y, et al. Intracranial atherosclerosis burden and stroke recurrence for symptomatic intracranial artery stenosis (sICAS). Aging Dis. (2018) 9:1096–102. doi: 10.14336/AD.2018.0301
20. Lu, SS, Ge, S, Su, CQ, Xie, J, Mao, J, Shi, HB, et al. MRI of plaque characteristics and relationship with downstream perfusion and cerebral infarction in patients with symptomatic middle cerebral artery stenosis. J Magn Reson Imaging. (2018) 48:66–73. doi: 10.1002/jmri.25879
21. Qiao, Y, Anwar, Z, Intrapiromkul, J, Liu, L, Zeiler, SR, Leigh, R, et al. Patterns and implications of intracranial arterial Remodeling in stroke patients. Stroke. (2016) 47:434–40. doi: 10.1161/STROKEAHA.115.009955
22. Sui, B, Gao, P, Lin, Y, Jing, L, and Qin, H. Distribution and features of middle cerebral artery atherosclerotic plaques in symptomatic patients: a 3.0T high-resolution MRI study. Neurol Res. (2015) 37:391–6. doi: 10.1179/1743132815Y.0000000023
23. Xu, WH, Li, ML, Gao, S, Ni, J, Zhou, LX, Yao, M, et al. Plaque distribution of stenotic middle cerebral artery and its clinical relevance. Stroke. (2011) 42:2957–9. doi: 10.1161/STROKEAHA.111.618132
24. Li, L, Tang, M, Yan, X, Gao, J, Ma, N, Shi, X, et al. Plaque characteristics in young adults with symptomatic intracranial atherosclerotic stenosis: a preliminary study. Front Neurol. (2022) 13:825503. doi: 10.3389/fneur.2022.825503
25. Cao, X, Yang, Q, Tang, Y, Pan, L, Lai, M, Yu, Z, et al. Normalized wall index, intraplaque hemorrhage and ulceration of carotid plaques correlate with the severity of ischemic stroke. Atherosclerosis. (2020) 315:138–44. doi: 10.1016/j.atherosclerosis.2020.10.896
26. Yang, WJ, Abrigo, J, Soo, YO-Y, Wong, S, Wong, KS, Leung, TW, et al. Regression of plaque enhancement within symptomatic middle cerebral artery atherosclerosis: a high-resolution MRI study. Front Neurol. (2020) 11:755. doi: 10.3389/fneur.2020.00755
27. Doege, CA, Kerskens, CM, Romero, BI, Brunecker, P, Junge-Hülsing, J, von Pannwitz, W, et al. Assessment of diffusion and perfusion deficits in patients with small subcortical ischemia. AJNR Am J Neuroradiol. (2003) 24:1355–63.
28. Jiang, S, Cui, JY, Yan, YY, Yang, T, Tao, WD, and Wu, B. Association of compromised cerebral perfusion with lenticulostriate artery impairments in the subacute phase of branch atheromatous disease. Ther Adv Neurol Disord. (2022) 15:17562864221109746. doi: 10.1177/17562864221109746
29. Kleindorfer, DO, Towfighi, A, Chaturvedi, S, Cockroft, KM, Gutierrez, J, Lombardi-Hill, D, et al. 2021 guideline for the prevention of stroke in patients with stroke and transient ischemic attack: a guideline from the American Heart Association/American Stroke Association. Stroke. (2021) 52:e364–467. doi: 10.1161/STR.0000000000000375
30. Battistella, V, and Elkind, M. Intracranial atherosclerotic disease. Eur J Neurol. (2014) 21:956–62. doi: 10.1111/ene.12385
31. Lioutas, VA, Ivan, CS, Himali, JJ, Aparicio, HJ, Leveille, T, Romero, JR, et al. Incidence of transient ischemic attack and association with long-term risk of stroke. JAMA. (2021) 325:373–81. doi: 10.1001/jama.2020.25071
32. Grams, RW, Kidwell, CS, Doshi, AH, Drake, K, Becker, J, Coull, BM, et al. Tissue-negative transient ischemic attack: is there a role for perfusion MRI? AJR Am J Roentgenol. (2016) 207:157–62. doi: 10.2214/AJR.15.15447
33. Zhang, WW, Cadilhac, DA, Donnan, GA, O'Callaghan, C, and Dewey, HM. Hypertension and TIA. Int J Stroke. (2009) 4:206–14. doi: 10.1111/j.1747-4949.2009.00277.x
34. Wang, Y, Liang, H, Luo, Y, Zhou, Y, Jin, L, Wang, S, et al. History of hypertension is associated with MR hypoperfusion in Chinese inpatients with DWI-negative TIA. Front Neurol. (2019) 10:867. doi: 10.3389/fneur.2019.00867
35. Claassen, JAHR, Thijssen, DHJ, Panerai, RB, and Faraci, FM. Regulation of cerebral blood flow in humans: physiology and clinical implications of autoregulation. Physiol Rev. (2021) 101:1487–559. doi: 10.1152/physrev.00022.2020
36. Cipolla, MJ, Liebeskind, DS, and Chan, SL. The importance of comorbidities in ischemic stroke: impact of hypertension on the cerebral circulation. J Cereb Blood Flow Metab. (2018) 38:2129–49. doi: 10.1177/0271678X18800589
37. Wang, L, Jia, J, Hong, Z, Zhang, L, and Zhang, J. Effects of chemerin and homocysteine levels and their associations with occurrence and development of ischemic cerebrovascular disease. Lipids Health Dis. (2021) 20:108. doi: 10.1186/s12944-021-01524-7
38. Lin, CJ, Chang, FC, Guo, WY, Hung, SC, Luo, CB, Beilner, J, et al. Changes of time-attenuation curve blood flow parameters in patients with and without carotid stenosis. AJNR Am J Neuroradiol. (2015) 36:1176–81. doi: 10.3174/ajnr.A4239
39. Gutierrez, J, Khasiyev, F, Liu, M, DeRosa, JT, Tom, SE, Rundek, T, et al. Determinants and outcomes of asymptomatic intracranial atherosclerotic stenosis. J Am Coll Cardiol. (2021) 78:562–71. doi: 10.1016/j.jacc.2021.05.041
40. Jia, Q, Liu, H, Li, Y, Wang, X, Jia, J, and Li, Y. Combination of magnetic resonance angiography and computational fluid dynamics may predict the risk of stroke in patients with asymptomatic carotid plaques. Med Sci Monit. (2017) 23:479–88. doi: 10.12659/msm.902995
41. Zheng, J, Wang, Z, Li, N, Zhang, X, and Huo, X. Synthetic role of miR-200b-3p, ABCD2 score, and carotid ultrasound in the prediction of cerebral infarction in patients with transient ischemic attack. Brain Behav. (2022) 12:e2518. doi: 10.1002/brb3.2518
42. Zhao, W, Zhang, J, Liao, J, and Li, X. Evaluation of circulating endothelial progenitor cells and the severity of transient ischemic attack. J Clin Neurosci. (2022) 99:123–9. doi: 10.1016/j.jocn.2022.03.001
43. Tong, T, Yao, Z, and Feng, X. Combined diffusion- and perfusion-weighted imaging: a new way for the assessment of hemispheric transient ischemic attack patients. Int J Dev Neurosci. (2011) 29:63–9. doi: 10.1016/j.ijdevneu.2010.09.002
44. Gupta, A, Chazen, JL, Hartman, M, Delgado, D, Anumula, N, Shao, H, et al. Cerebrovascular reserve and stroke risk in patients with carotid stenosis or occlusion: a systematic review and meta-analysis. Stroke. (2012) 43:2884–91. doi: 10.1161/STROKEAHA.112.663716
Keywords: TIA, multimodal MRI, intracranial atherosclerotic, ABCD2 score, high-resolution MRI
Citation: Sui Y, Sun J, Chen Y and Wang W (2023) Multimodal MRI study of the relationship between plaque characteristics and hypoperfusion in patients with transient ischemic attack. Front. Neurol. 14:1242923. doi: 10.3389/fneur.2023.1242923
Edited by:
Jan Dankbaar, University Medical Center Utrecht, NetherlandsReviewed by:
Bowen Sun, Department of Neurosurgery, First Affiliated Hospital of Harbin Medical University, ChinaQingguang Zhu, Shanghai University of Traditional Chinese Medicine, China
Copyright © 2023 Sui, Sun, Chen and Wang. This is an open-access article distributed under the terms of the Creative Commons Attribution License (CC BY). The use, distribution or reproduction in other forums is permitted, provided the original author(s) and the copyright owner(s) are credited and that the original publication in this journal is cited, in accordance with accepted academic practice. No use, distribution or reproduction is permitted which does not comply with these terms.
*Correspondence: Wei Wang, MjQ0N0BocmJtdS5lZHUuY24=