- 1Locomotion Research Laboratory, School of Biological and Health Systems Engineering, Arizona State University, Tempe, AZ, United States
- 2Muhammad Ali Movement Disorders Clinic, Barrow Neurological Institute, St. Joseph’s Hospital and Medical Center, Phoenix, AZ, United States
- 3Gait and Balance Dysfunction Laboratory, College of Health Solutions, Arizona State University, Tempe, AZ, United States
- 4Department of Veteran’s Affairs, Phoenix, AZ, United States
The aim of this study was to investigate to what extent PD affects the ability to walk, respond to balance perturbations in a single training session, and produce acute short-term effects to improve compensatory reactions and control of unperturbed walking stability. Understanding the mechanism of compensation and neuroplasticity to unexpected step perturbation training during walking and static stance can inform treatment of PD by helping to design effective training regimens that remediate fall risk. Current rehabilitation therapies are inadequate at reducing falls in people with Parkinson’s disease (PD). While pharmacologic and surgical treatments have proved largely ineffective in treating postural instability and gait dysfunction in people with PD, studies have demonstrated that therapy specifically focusing on posture, gait, and balance may significantly improve these factors and reduce falls. The primary goal of this study was to assess the effectiveness of a novel and promising intervention therapy (protective step training – i.e., PST) to improve balance and reduce falls in people with PD. A secondary goal was to understand the effects of PST on proactive and reactive feedback responses during stance and gait tasks. Multiple-baseline, repeated measures analyses were performed on the multitude of proactive and reactive performance measures to assess the effects of PST on gait and postural stability parameters. In general, the results indicate that participants with PD were able to use experiences with perturbation training to integrate and adapt feedforward and feedback behaviors to reduce falls. The ability of the participants with PD to adapt to changes in task demands suggests that individuals with PD could benefit from the protective step training to facilitate balance control during rehabilitation.
1. Introduction
Parkinson’s disease (PD) is a neurological disorder characterized by bradykinesia, tremor, rigidity, postural instability and, affects an estimated 1 million individuals in the US. Postural Instability and Gait Dysfunction (PIGD), a subset of PD symptoms describing impaired standing posture and balance, bradykinetic gait features, freezing of gait (FOG), and falls, is a disabling condition that, unlike other cardinal features of PD, is often not adequately treated by dopaminergic medications. Fall incidence rates among the PD population are estimated to range as high as 50–70%, with many individuals suffering recurrent falls, and these falls are a major cause of injury and disability. It is estimated that healthcare expenditures related to these falls exceeded $27 billion in 2013 (1). As the population of older adults (>65 years old) in the US increases over the coming decades, reaching a projected 98 million by 2060 (2), the rates of PD and associated falls are expected to rise dramatically. Although modern medicine and new medical technologies offer enormous potential to improve the diagnosis and treatment of many symptoms, falls still represent a major and largely untreated problem for PD patients. While pharmacologic and surgical treatments have proved largely ineffective in treating PIGD thus far (3–9), studies have demonstrated that therapy specifically focusing on posture, gait, and balance may significantly improve these factors and reduce falls (10–13).
Perturbation-based balance training, or protective step training (PST), defined as balance training using repeated, external perturbations, is one such method of therapy that has demonstrated improvements in balance and fall recovery in multiple populations (14). Several studies have observed decreased fall rate and an increased ability to recover from a fall upon repeated exposure to a perturbation in healthy controls (15, 16). Investigators have reported that adaptations to avoid falling can be modulated via both feedforward (predictive) and feedback (reactive) mechanisms (17). Predictive mechanisms of recovery involve changes to gait parameters such as base of support, trunk angle, and velocity, that may reduce the magnitude of the required balance recovery response upon delivery of a perturbation. Reactive mechanisms of improvement may involve earlier detection of perturbation, likely to require recovery response, and improved motor responses triggering increased relevant muscle action and fewer maladaptive movements following perturbation (17). Studies suggest that reactive balance may not be entirely intact in PD, but that learning is still possible (3–9), making it important to study the effects of PST in this population.
Recent literature for PST in populations with PD is represented in Table 1. Studies indicate that PST may be useful in improving gait and postural control that precipitate future falls (23), however, there is a lack of consistency regarding the specific improvements and whether those improvements can effectively transfer from a rehabilitation setting to activities of daily living. Studies have shown that training is more effective if it is specific to the skill to be improved (23), and while perturbation-training in PD is ongoing, the majority of research is not task-specific and only a few studies have attempted to replicate common causes of falls (e.g., slips and trips) (19–21, 24). To this end, the specificity of PST is in need of further study to determine what types of perturbation are most effective at inducing adaptive response and what intensity, frequency, and duration of perturbation training sessions are required for these results to be retained.
The primary goal of this study was to assess the effectiveness of two kinds of PST (anterior translations of a split-belt forceplate during (1) forward gait and (2) static postural stability tasks) to improve balance and stability in people with PD by better understanding the effects of PST on proactive and reactive feedback responses during stance and gait. We hypothesized that PD patients will be able to learn in an explicit, feedforward manner, adjusting base of support prior throughout walking trials to prepare for unexpected perturbation, but may be unable to improve reactive response variables such as reaction time and strategy. This work may enhance the clinician’s ability to treat balance/gait disturbances leading to falls in people with PD utilizing protective step training.
2. Materials and methods
2.1. Participants
Twelve participants diagnosed with PD by a movement disorders neurologist were recruited for this study (age = 62 ± 7.1; 9 males, 3 females). Participants were included in the study if they were able to ambulate without assistance, had no known neurologic, cardiovascular, or orthopedic deficit that could significantly impact cognition and functional performance (Mini-Mental Status Examination <25), and had a Hoehn & Yahr (H&Y) score between II-III. Subjects were excluded if they exhibited functionally disabling dyskinesia or dystonia, orthostatic hypotension, neurosurgical intervention (deep brain stimulation), and any significant musculoskeletal or metabolic disorders. All subjects were examined during the “on” dopaminergic medication state, having taken their last dose approximately 1–1.5 h prior to testing. Disease severity and clinical scales of symptoms were tested in the “on” state utilizing the H&Y scale (25) and the motor subscale of the International Parkinson and Movement Disorder Society-Sponsored revision of the Unified Parkinson’s Disease Rating Scale (MDS-UPDRS Part III). Subjects in the present study had an average H&Y score of 2.7 and an average disease duration of approximately 3.5 ± 3.1 years. Prior to testing, subjects were randomly assigned to two groups. One group started with postural perturbation (PP) training (during stance), while the other group began with gait perturbation (GP) training before crossing over (during walking). During this onboarding period, self-reported and observed leg dominance in bilateral mobilizing was utilized to determine the dominant leg that will be perturbed in the walking trials. Investigators described a scenario for the participant in which they were asked which leg they would use to kick a ball over the ground. This study (experimental procedures and design) was approved by the Institutional Review Board at Arizona State University and performed according to the declaration of Helsinki. All participants provided written informed consent prior to data collection.
2.2. Study design
Prior to baseline testing, two 3-min walking sessions were given to the participants to familiarize themselves with the treadmill and the laboratory environment. The first session was primarily for familiarizing and adapting to the treadmill. Following this session, the participant was given a rest period and encouraged to ask questions or bring up any concerns with the task. The second session was used to standardize the participant’s preferred walking speed (PWS) that they would be using throughout the trials. In this session, walking speed was increased incrementally until participants indicated that the speed was consistent with their normal walking speed. The walking speed was then increased in 0.1 m/s increments until the participant expressed discomfort or reported the speed to be inconsistent with their normal walking speed. Following the familiarization period, the protocol began with a series of baseline tests: 2-min of overground walking (OG1), Timed Up-and-Go Test (TUG1), Short Physical Performance Battery Test (SPPB1), postural stability in both the eyes-open and eyes-closed conditions (PSEO1, PSEC1), and 2-min of treadmill walking (TW1). Following baseline trials, participants in the first group commenced postural perturbation (PP) training during stance while the second group commenced gait perturbation (GP) training during walking. Both groups were instructed to maintain their balance and avoid a fall when introduced to unexpected perturbations. Upon completion of the respective training paradigms, a ‘washout’ period was introduced in which the groups performed a second 2-min treadmill walking trial (TW2) to observe any after-effects from baseline. Following this period, the two groups crossed over and commenced the alternate perturbation training paradigms. Finally, both groups performed a final 2-min treadmill walking trial (TW3) along with the post-training tests performed during baseline testing: OG2, TUG2, SPPB2, PSEO2, and PSEC2. All perturbations occurred on each participant’s dominant leg. A schematic of the study design is presented in Figure 1. Figure 2 illustrates comparisons made in this study.

Figure 2. Illustration of comparison made between novel recovery from the first perturbation (i.e., GP1 or PP1) vs. trained recovery from the last two perturbations (i.e., GP11,12 or PP11,12).
2.3. A protective step training during stance and gait
Perturbation training protocol was based on previous study on young and older adults without PD (26–29). The protective step training protocol during walking, i.e., gait perturbation (GP) training, consisted of continuously walking on a treadmill with 12 blocks (GP1-12) of unexpected perturbations. Participants began walking unperturbed for a baseline period of approximately 15 s. The subsequent 10 s following the baseline period are designated as the perturbation window: a 10-s window in which the subject is given an unexpected anterior translation of the right treadmill belt (acceleration of 10 m/s2; duration of 0.2 s) at the instant of right heel contact, i.e., the perturbed step. This translation resulted in the displacement of the subject’s COM, in which participants were instructed to restore their balance. Following the perturbed step and the subsequent recovery duration, participants walked unperturbed until they were able to match their preferred walking speed again.
The protective step training protocol during stance, i.e., postural perturbation (PP) training, evaluated standing balance maintenance given 12 unexpected perturbation blocks (PP1-12). Participants were instructed to stand upright with their arms by their sides and look straight ahead. The training began with participants in quiet stance for approximately 15 s. Following this static period, a simultaneous anterior translation of both treadmill belts was induced with an acceleration of 8 m/s2 and duration of 0.1 s. Akin to the GP training, the resulting platform translation resulted in the displacement of the subject’s COM, in which participants were instructed to restore their balance.
2.4. Experimental setup
GRAIL system (Gait Real-time Analysis Interactive Lab, Motek Medical, Amsterdam, the Netherlands) was utilized to simulate both types of (i.e., during standing and walking) perturbations. GRAIL consisted of a dual-belt instrumented treadmill equipped with dual embedded force plates in a speed-matched virtual environment projected on a semi-cylindrical screen (during the experiment, the virtual environment was turned off and a blank wall was projected to avoid any visual perturbation effects) (Figure 3). Subjects were equipped with standardized footwear to minimize experimental confounds, as well as a full-body harness tethered to an instrumented safety system that supported their full weight. The dual force plates embedded in the treadmill belts were utilized to collect data during postural stability trials. Lower body kinematics was recorded using 12 Vicon cameras (100 Hz; Vicon Bonita, Vicon, United States) with a modified Helen-Hayes marker set, including 25 reflective markers, which were placed in accordance with the lower body Vicon full-body Plug-in-Gait model. Motion capture data was filtered using a fourth-order low-pass Butterworth filter and a cut-off frequency of 6 Hz. Accordingly, force plate data was filtered using a fourth-order-low-pass Butterworth filter with a cut-off frequency of 10 Hz, to eliminate extraneous measurement noise. Nonlinear measures – applied during TW1, TW2, and TW3 – were implemented to estimate the structure of variability, e.g., the scaling behavior (scaling exponent α) of stride intervals and the signal regularity (MSE) of center of mass (COM) velocities – were unfiltered during the analysis. All analysis was performed using custom Matlab routines (The Mathworks, Version 2016a).
2.5. Data analyses
Feedforward responses from gait were analyzed from the 15 s of unperturbed walking prior to each perturbation. Spatiotemporal parameters and gait variability were extracted from the 10 steps prior to the perturbation, while dynamic stability was examined at the final heel contact before the perturbation (perthc). Adaptive behavior was determined by comparing responses from GP11 and GP12, with GP1. Regarding PP training, predictive postural adjustments were assessed from the 15 s of quiet standing prior to the perturbation. The whole-body center-of-mass (COM) dynamics, base of support (BOS), and angular kinematics of the trunk, knee, and ankle in the sagittal plane delineated any changes to feedforward control prior to a perturbation. Adaptive behavior was assessed by comparing responses from the last two perturbation blocks (PP11 and PP12)-Post-test-with the initial block (PP1) – Pre-test. Joint angles were measured from perthc, while joint angle range of motion (ROM) was standardized from the minimum and maximum angles within the dominant leg’s normalized gait cycle just prior to perthc. Angles were calculated by the segmental method for determining 2D joint angles by the means of cardan sequences and a 6 degrees of freedom model. Relative angle was determined between the local coordinate systems of each proximal and distal segment. The angles chosen for sagittal plane analysis were trunk flexion/extension (measured as the angle between a vertical line, perpendicular to the ground, bisecting the sacrum and a line bisecting the thoracic spine), knee flexion/extension (defined by the long axis of the tibia with respect to the long axis of the femur), and ankle plantar and dorsiflexion determined by the shank and foot segments. For knee joint angles, full extension was defined as zero degrees and movement into flexion being positive. Regarding ankle angles, zero was set at 90° to delineate plantarflexion and dorsiflexion. Plantarflexion was set as the negative degrees. In the frontal plane, lateral trunk flexion was measured as the angle between a vertical line bisecting the contralateral ASIS (perpendicular to the ground) and a line from the ASIS to the AC joint marker. Table 2 provides further operational definitions of the feedforward parameters.
Reactive feedback responses from GP training were examined by identifying the contralateral recovery step (rechc) immediately following the perturbation (Figure 4A). Dynamic stability of the first recovery step from perturbation blocks (GP11 and GP12) was compared with the dynamic stability of the initial block (GP1), to evaluate adaptive feedback control. For PP training, reactive modifications from the first recovery step after the perturbation (rechc) was identified as the initial recovery mechanism. The end of the recovery period was identified as the zero-cross point of the COM velocity in the anteroposterior direction (Figure 4B). Table 2 outlines the feedback parameters used to evaluate subject performance.
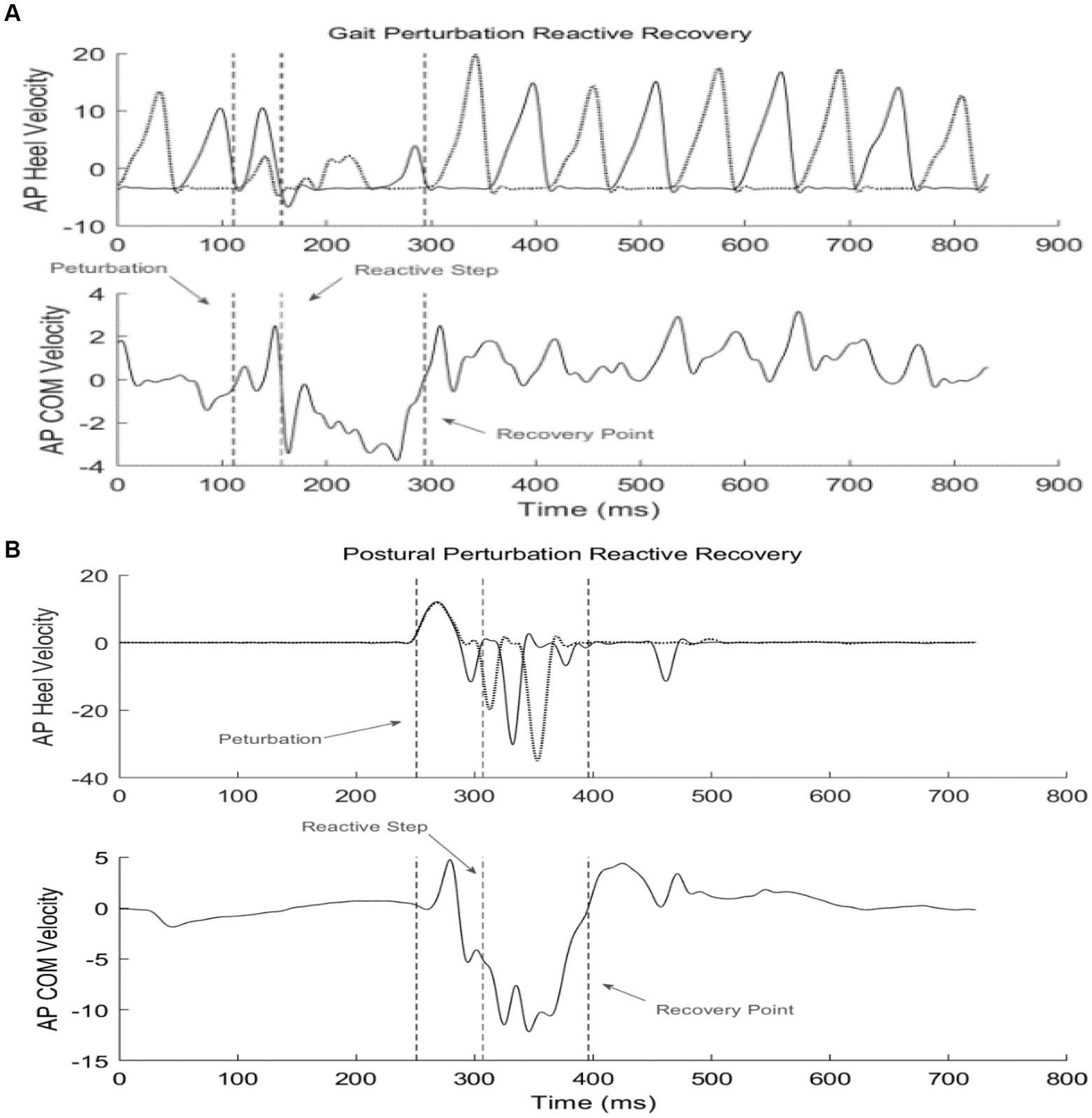
Figure 4. Example of reactive recovery dynamics given a perturbation during stance or gait. The 1st dotted line identifies perturbation onset; the 2nd line identifies the reactive recovery step (rechc); the 3rd line marks the termination of the recovery period. (A) Gait perturbation example; (B) Postural perturbation example.
Dynamic stability was calculated by the margin of stability (MOS), which measures the movement of the COM relative to the base of support (30). Specifically, MOS in the anteroposterior (AP) direction, was determined by the distance between the anterior boundary of the BOS at heel contact (toe marker of the leading foot) and the extrapolated COM (30); MOS in the mediolateral (ML) direction was calculated as the difference between the lateral boundary of the BOS at heel contact (heel marker of the leading foot) and the extrapolated COM. An increased MOS indicates the COM is further within the BOS, while decreased MOS indicates COM is nearer to the limits of the BOS.
Combined after-effects of the perturbation training were evaluated from continuous gait on the treadmill, both before and after testing (TW1 and TW3). Measures of gait variability, complexity, and smoothness were employed to determine the sensitivity of the pre-and post-training effects. Variability was assessed by the RMS of COM accelerations (AP, ML, and V) along with statistical measures of variability from spatiotemporal gait parameters: Standard deviation (SD) and coefficient of variation (CV). CV denotes the variability of a specific gait parameter normalized to its mean value; it is represented as a percentage (CV=SD/mean × 100). Gait complexity was measured by multiscale entropy (MSE). MSE is a regularity measure developed by Costa et al. (31) that quantifies the information content of the gait signal (COM velocities in the AP, ML, and V directions) over a range of physiologically relevant time scales while sample entropy is computed for every consecutive coarse-grained time series. The entropy values are then plotted as a function of the time scales in which the area under the curve reveals the signal’s complexity index (CI). A complex signal is associated with a time evolution with a rich structure on multiple scales. The first 10 s and the last 10 s – initiation and termination of 2-min treadmill walking (TW1-3) – were excluded from the analysis. The local average and the local SD of each time series were computed for each spatiotemporal parameter. Table 2 provides further details.
2.6. Statistical analyses
For all statistical comparisons, assumptions of ANOVA (e.g., homogeneity of variance and normal distribution) were tested using the normality and Leven’s tests. Correction for multiple follow-up comparisons was done using the Bonferroni correction. All other univariate analyses uses one-way split-plot repeated measures ANOVA with Greenhouse–Geisser correction for sphericity (i.e., between subject effect, training group, is the whole plot effect of a split-plot design). The Subject effect is nested within the Group effect which was specified as random.
The generalizability of two types of training programs (PST during stance and dynamic gait) and Pre-test and Post-test differences on dependent measures in Table 2 were ascertained using a linear mixed effect model on all gait and posture parameters using the above repeated measures ANOVA. The statical analyses were processed using the JMP Pro 16, 2021, SAS Institute.
3. Results
3.1. Effects of PST during stance on feedforward or proactive adaptation and, associated group effect (generalizability of two types of PST-stance and walking)
The results of the univariate repeated measures ANOVAs on all dependent measures associated with feedforward variables in Table 2 indicated a significant differences on only one of the feedforward variables – Knee flexion angle during pre and post-trial period (F1,10 = 5.662, p = 0.0386, effect size 0.259) (Figure 5). No significant differences were observed for the group comparison or generalizability of two types of training (F1,10 = 0.0102, p = 0.92) on the knee flexion angle or all other feedforward dependent measures in Table 2. In general, knee flexion angles were significantly different indicating a proactive response using the knee strategy (i.e., bending the knee to lower the whole body COM) to maintain stability (Figure 6).
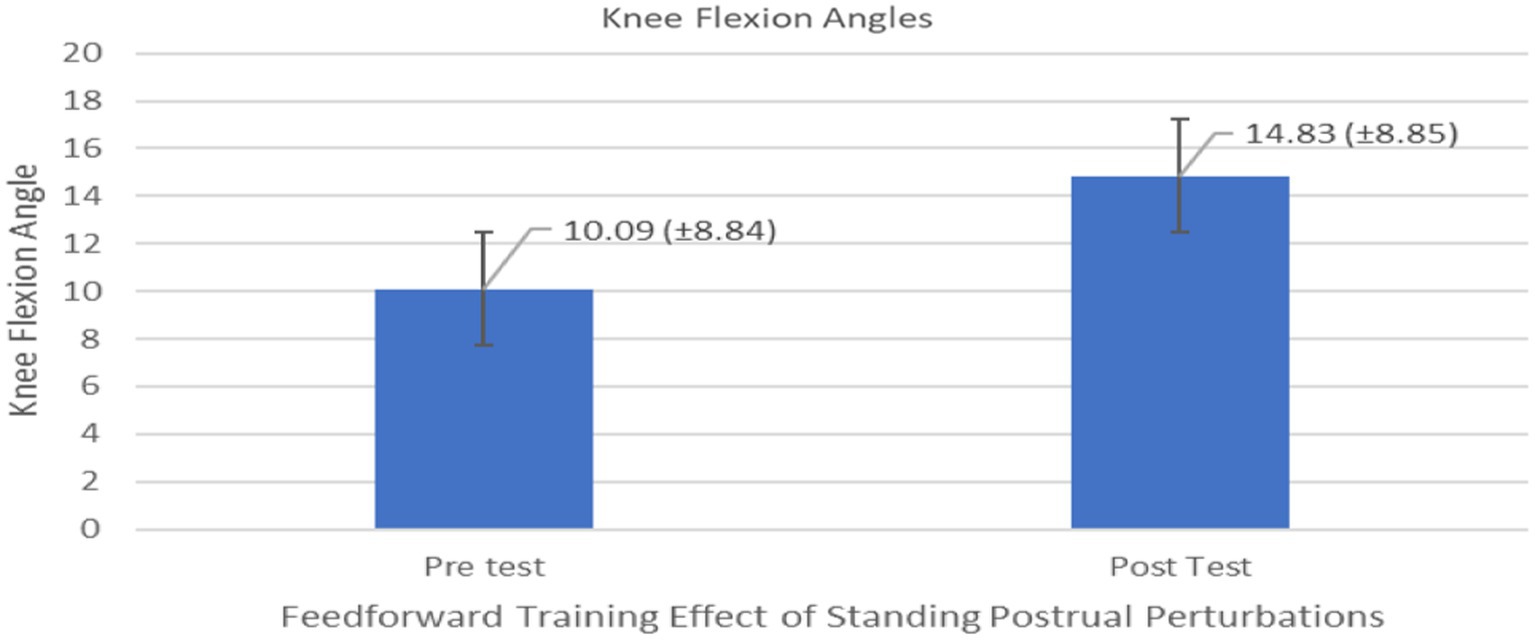
Figure 5. Feedforward Effects of PST during stance on the knee flexion angles with the means and standard deviations shown.
3.2. Effects of PST during stance on feedback or reactive adaptations
Compared to the initial unexpected perturbation block, reaction time (TimeLatency) showed significant improvement (F1,10 = 4.94, p = 0.050, effect size =0.352) (Figure 7). This suggests that reactive adaption utilizing feedforward mechanisms is still active and may be trained and directed toward improving fall safety (Figure 8). No significant group (generalizability of two types of training) effect (F1,10 = 0.012, p = 0.916) was observed for the reactive adaptation variables in Table 2.
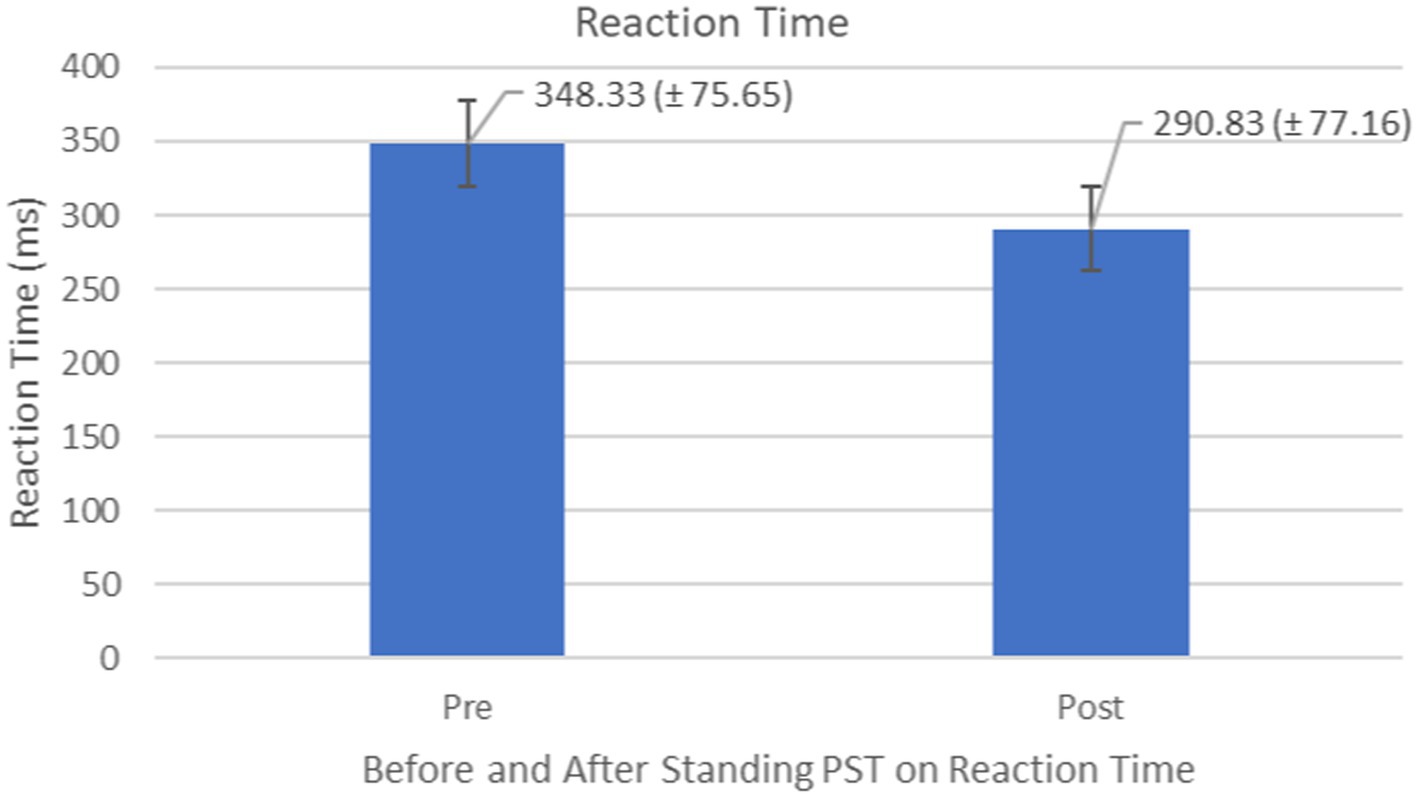
Figure 7. Feedback Effects of PST during stance on reaction time of the stance foot with the mean reaction times and standard deviations shown.
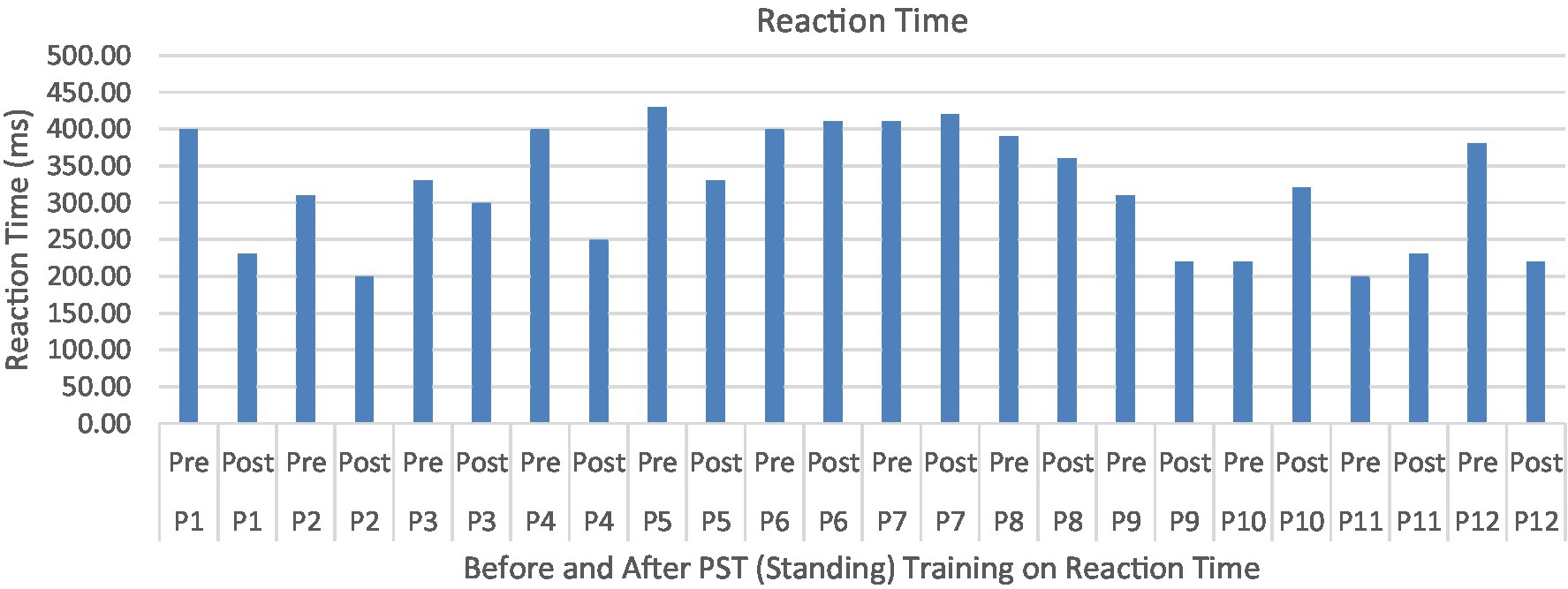
Figure 8. Reaction time or TimeLatency (ms) associated with postural perturbation training during the first and the last perturbations. Participants were able to produce quicker responses to postural perturbations after the PST.
3.3. Effects of PST during walking on feedforward or proactive adaptations and generalizability of training groups
Proactive adaptations during gait were assessed during the 10–15 steps before the initial perturbation block (GP1) and the 10–15 steps preceding the last perturbation block (GP12). Flexion angles of the trunk, hip, and knee of the perturbed limb (pertbhc) were significantly greater from GP1 to GP12, revealing feedforward adaptations in anticipation of the perturbation. These biomechanical modifications are characterized by the varying trunk (F1,10 = 11.311, p = 0.007, effect size = 0.08) (Figures 9–11) and hip flexion angles (F1,10 = 5.709, p = 0.038, effect size = 0.05) (Figures 12, 13) and, adopting vigilant gait marked by higher heel contact velocity (F1,10 = 6.503, p = 0.029, effect size = 0.33) (Figures 14, 15). Group effects (generalizability of training) were not statistically significant for these variables (i.e., trunk and hip flexion angles and heel contact velocity).
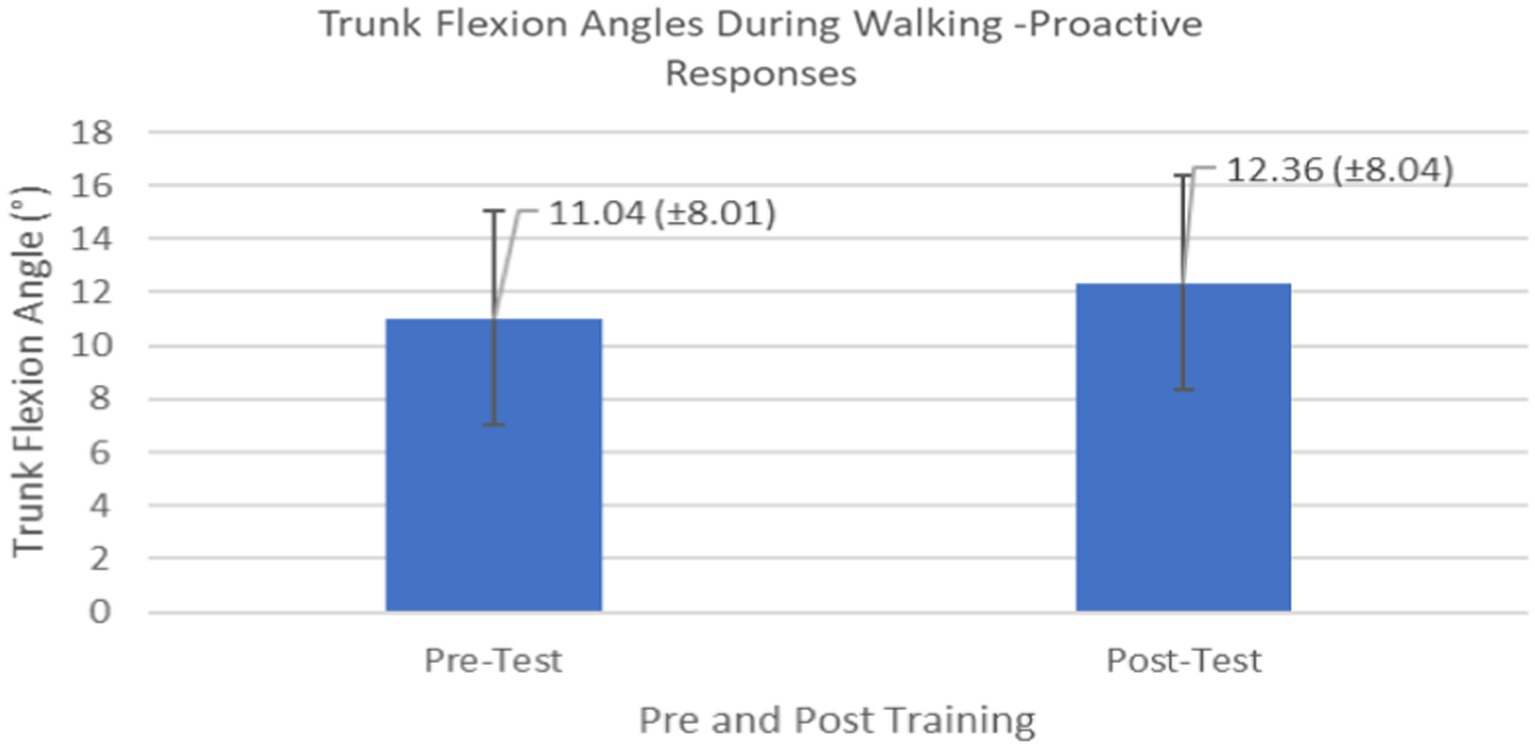
Figure 9. Feedforward Effects of gait perturbation training with the mean reaction times and standard deviations shown.
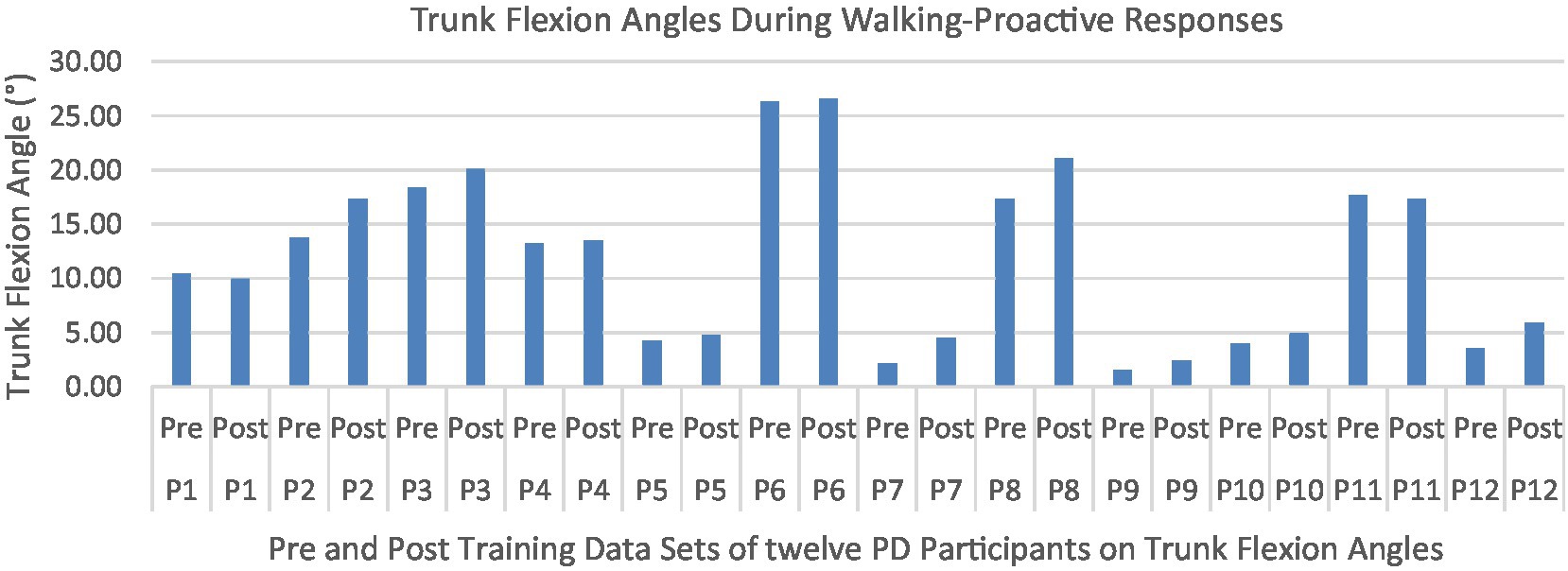
Figure 10. Individual effects of gait perturbation training on feedforward/proactive adaptation of trunk flexion.
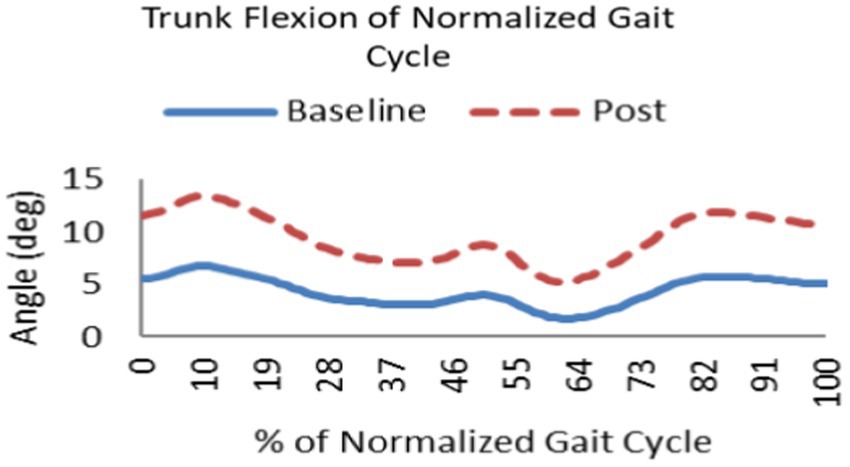
Figure 11. Following repeated gait perturbation training a subject’s trunk flexion is greater throughout the gait cycle exhibiting feedforward/proactive adaptation.
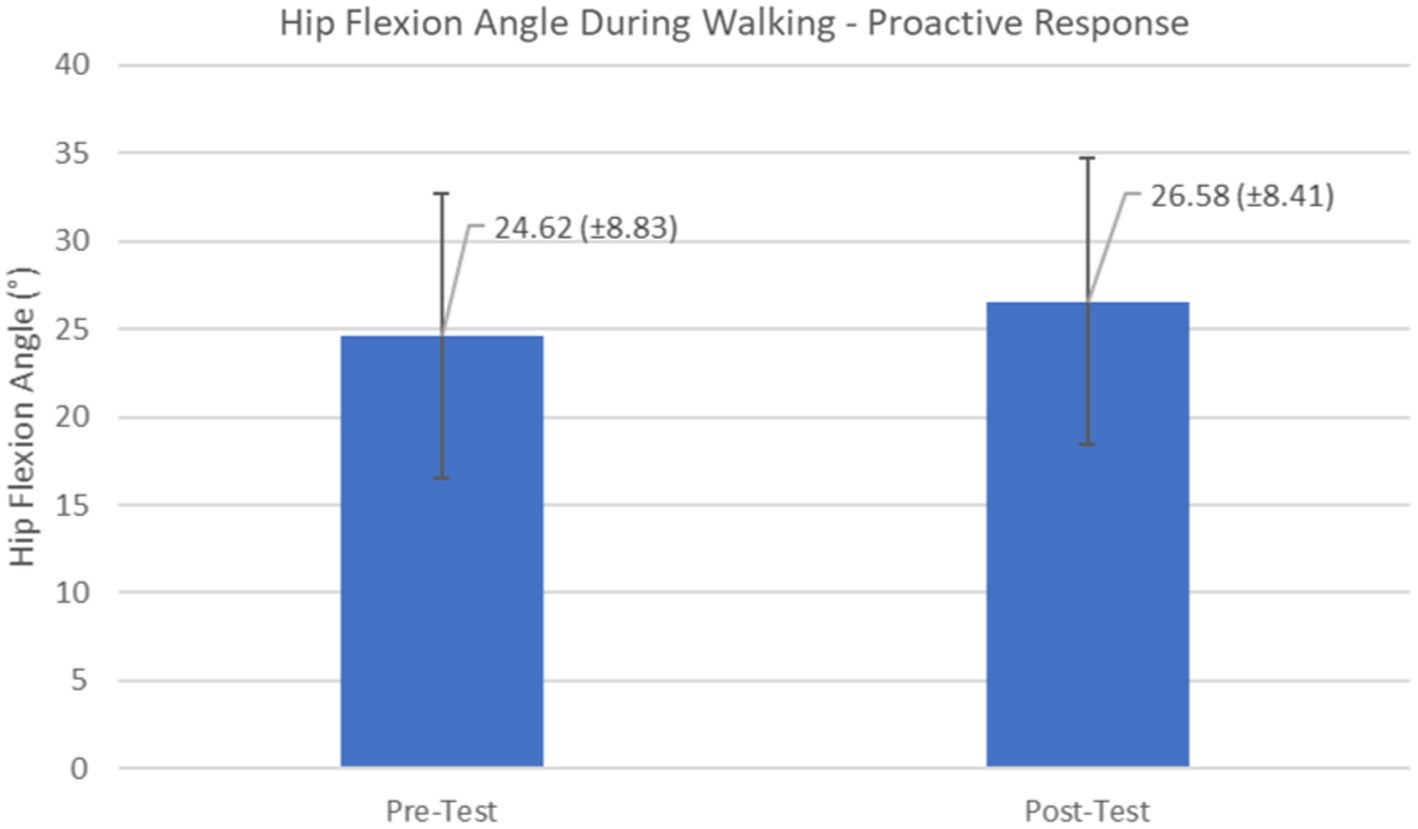
Figure 12. Feedforward Effects of gait perturbation training with the mean hip flexion angles and standard deviations shown.
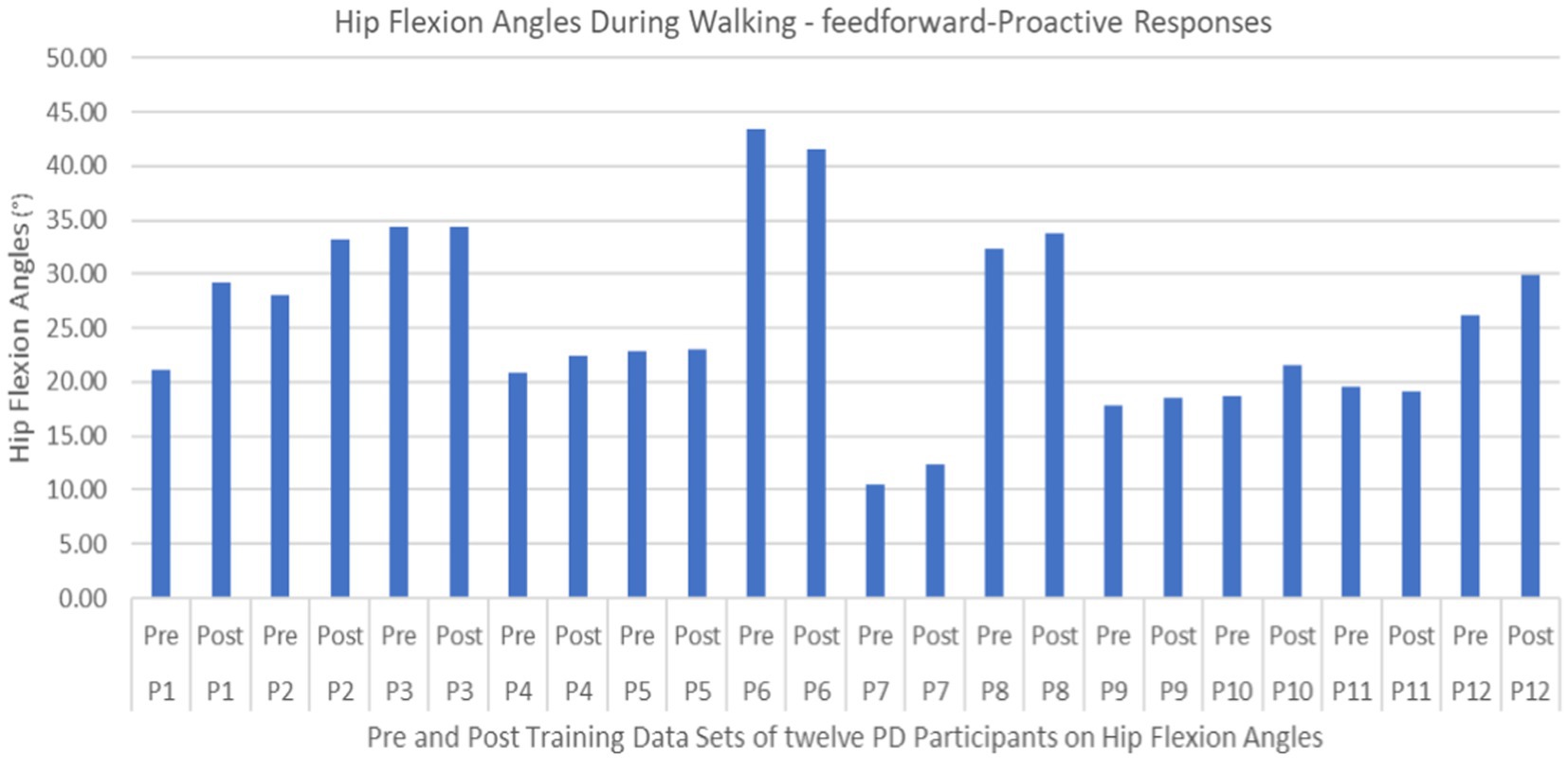
Figure 13. Individual effects of gait perturbation training on feedforward/proactive adaptation of hip flexion.
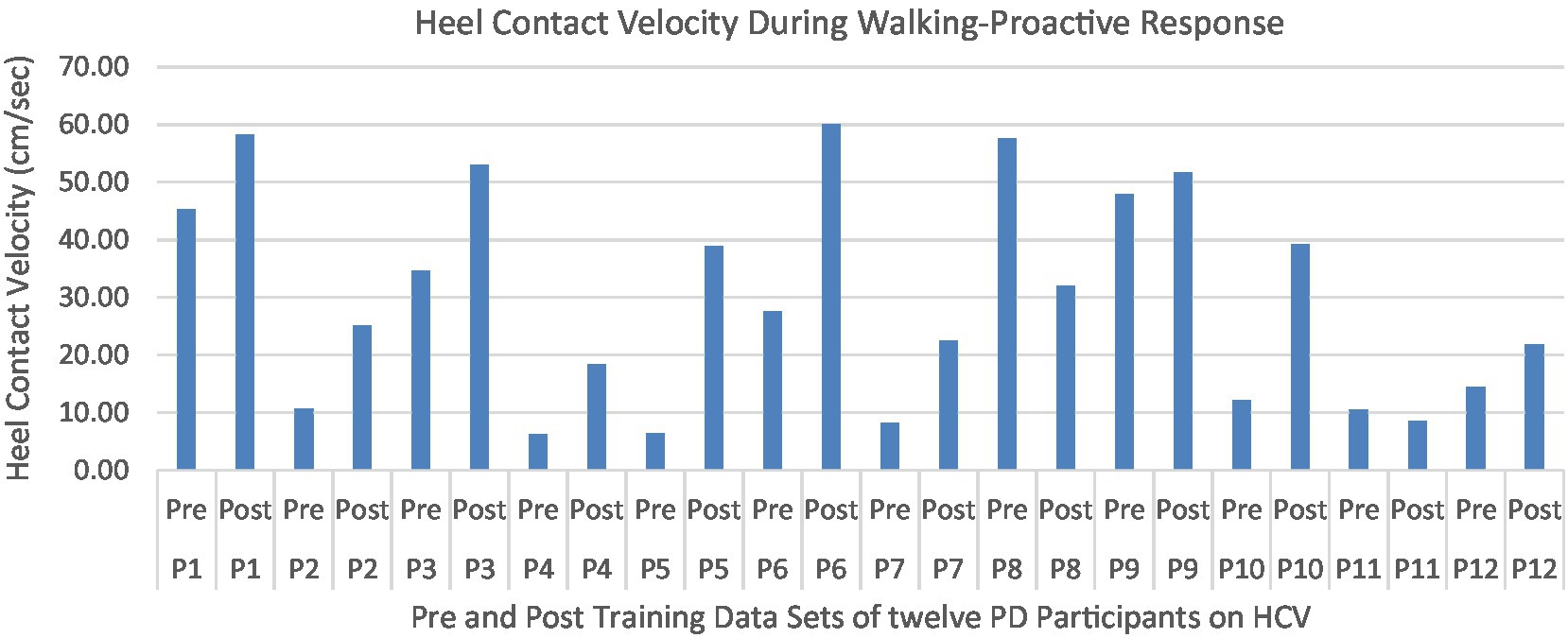
Figure 15. Individual effects of gait perturbation training on feedforward/proactive adaptation of heel contact velocity.
3.4. Effects of PST during walking on feedback or reactive adaptations
No significant differences were observed in any of the reactive parameters associated with recovery from a slip.
4. Discussion
The primary goal of this study was to assess how PD affects the ability to respond to slip specific perturbations and if one session of protective step training can produce short-term adaptations to improve walking and static balance control. A secondary goal was to determine the generalizability of two types of training programs (PST during stance and dynamic gait) and to what extent the perturbation-based balance training are specific and transferable to the nature of the perturbations experienced. At present, little is known regarding the effect of PD on the ability to react and adapt to standing and walking perturbations. For example, previous studies have shown improved adaptive capacity with perturbation responses in the mediolateral direction (21, 32). However, the specific nature of the perturbations in the current study were to simulate realistic slip perturbations without any walking aid, which were performed by anterior translations during standing and walking. We hypothesized that participants with PD would experience difficulty adapting reactive feedback-based strategies, but would adapt to planned feedforward strategies to standing and dynamic walking perturbation programs. The results generally support this hypothesis, showing that participants with PD were able to use experiences with perturbation training to integrate and adapt proactive feedforward strategies. Reactive, feedback strategies were less frequently improved through practice in the current cohort. Notably, there was little generalization between in-place and walking practice. The ability of the participants with PD to adapt to changes in task demands, particularly proactive behavior, suggests that individuals with PD could benefit from a specific training paradigm to facilitate specific balance control during rehabilitation (13, 33).
The results in this study are consistent with previous studies regarding early PD, which reported proactive adjustments during postural stability and locomotor perturbation tasks (6, 7, 20). This was evident during the walking perturbation program when comparing the effects of walking behavior prior to the initial walking perturbation block (GP1) and the final perturbation block (GP12). Particpants demonstrated significant feedforward adaptations in anticipation of the unexpected perturbation by significantly increasing trunk and hip flexions during walking along with higher heel contact velocity to veer away from untimely balance perturbations and adopting a more cautious gait to increase stability. However, this proactive effort may not have been fully realized in the current study as we found no significant differences in any of the reactive measures for these patients.
Predictive control is associated with supraspinal structures (8) involving cognitive processes like attention and memory (9) that may not be impaired in the early stages of the disease (8, 9). Predictive responses are important components for safe locomotion (33, 34) because they reduce the consequences of expected perturbations (12) and ultimately reduce the risk of falls. Thus, the increased risk of falls in early PD patients may be associated with deficits in reactive motor control. Understanding the ability of someone with PD to adapt to changes in task-specific demands will be useful in therapeutic intervention strategies.
Studies have closely linked the striatal system to motor learning, (5, 35, 36), suggesting that individuals with deficiencies in this system, such as those with Parkinson’s disease, would, in addition to the degradation of their movement patterns at baseline, have difficulty acquiring movement schema that would allow them to learn tasks quickly and accurately. However, studies examining the ability of patients with PD to learn and adapt to motor tasks have been relatively inconsistent (10–13, 37–45). While these studies indicate that PD patients are able to learn motor tasks, there is disagreement about the amount and type of improvement. A possible reason for this is that conflicting studies utilized different types of learning (implicit and explicit), considering specific aspects of learning are more severely impacted by PD, especially in the early stages of the disease. It has been reported that PD patients are able to learn specific tasks, however, they may require more practice than healthy controls do. Furthermore, the learned skills are not easily generalizable to other tasks, even if those tasks are similar (5, 40–45). The slower rate of learning and lack of transference may imply that PD patients are still able to learn in an explicit, feedforward manner – they may “pre-program” specific techniques and tasks quite capably - i.e., proactive mechanisms – but they may be unable to easily adjust to changes requiring the use of automatic or reactive mechanisms, making ready adaptation to changing conditions or simultaneous completion of multiple tasks, both of which are often required for balance and gait, quite difficult.
4.1. Predictive motor adaptations of PST
Learning integration for both proactive (feedforward) and reactive (feedback) adaptations were analyzed from the gait and postural PST paradigm, Figure 1. Feedforward responses from gait were extracted from the 15 s of unperturbed walking prior to each perturbation. Trunk flexion and hip flexion of the perturbed limb (pertbhc) were significantly greater compared to baseline, revealing feedforward adaptations in anticipation of the perturbation. Furthermore, heel contact velocity was increased in an effort to regain balance given a perturbation. The gait modification demonstrates the adoption of a more considered and vigilant gait to increase stability.
4.2. Reactive motor adaptations of PST
However, even after a significant effort of the feedforward system, the reactive responses to gait PST were not robustly impacted through a single session of 12 perturbations. Regarding walking slips, recovery step time (Time1stStep) – the time elapsed between perturbation onset and the first recovery step of the contralateral foot – showed no significant difference between baseline and the last perturbation trial. Similarly, the recovery period (TimeRec) – time elapsed from perturbation onset until the zero-cross of the anteroposterior COM velocity – also did not show improvement in their reactive recovery time.
Like gait PST, reactive feedback control for static stance PST was not robustly improved through practice. This is in contrast with some previous results (46, 47). Alternatively, it is also possible that feedforward modifications may not have generated sufficient stability improvements post-perturbation adaptations to the stability margins. Finally, it is possible that 12 perturbations was too little of a dose to produce meaningful improvements as were observed in previous studies.
4.3. Perturbation-specific transference
The secondary goal of this study was to determine the generalizability of PST during stance and PST during dynamic gait, and to what extent the specific type of the perturbations may transfer to perturbations in everyday situations. Dopaminergic treatments has been shown to be ineffective or unsatisfactory at treating postural instability and gait dysfunction in idiopathic PD, however, studies have demonstrated that therapy explicitly focusing on posture, gait, and balance may significantly improve these factors (18, 32). It is hypothesized that therapy specifically modeling situations in which individuals with PD are likely to fall (e.g., slipping due to shuffled steps/reduced executive function) may be more beneficial to prevent future falls than more generalized physical therapy. Further, repeated perturbation training has been shown in previous studies to improve features of postural stability and gait in PD patients. However, most of this preceding work has focused primarily on ascertaining the effects of training during static stance, either through training of center of pressure shifts toward a patient’s limits of stability (teaching the patient to weight-shift and lean safely) (48, 49) or through the training of adaptive responses (either postural adjustments or compensatory stepping) to regain balance following an external perturbation (32, 46, 50–52). These studies suggest that PD patients are able to learn to adapt to perturbations, that these adaptations may persist for several months, and that PST may enhance balance confidence. However, the generalizability of response improvements to other types of perturbation, such as during standing and walking, is uncertain (40–45). Results from the current study suggested that there may be limited generalizability across two types of training programs (PST during stance and dynamic gait). Albeit, healthy older adults were able to genderized their training (slips/trips) (53), in this study with PD patients, the specific training (either postural or gait perturbation training) did not improve balance in the untrained task. Thus, more personalized and specific training program is required to improve balance maintenance in PD patients.
5. Limitations
Several limitation to this study should be considered when interpreting the findings. First, this was a preliminary study to elucidate the optimal dose and frequency, as well as the therapeutic index, to determine maximal efficacy for a PST dose–response relationship in PD populations. The present study applies only a single perturbation acceleration of 10 m/s2 in the anterior direction to elicit a slip-specific perturbation effect. We also did not use a younger or age-matched control group to be compared. This perturbation was chosen to represent a worst-case scenario condition for PD and as an incipient marker for a perturbation scenario and population that is not well researched in the literature. Given the preliminary nature of this study, our sample size was relatively small (post effect sizes were from 0.03 to 0.352) and may affect the strength of our conclusions. Given the heterogeneity of PD, further studies involving a larger number and a wider range of PD participants is warranted. Further, the present finding only looked at acute after-effects of compensatory and adaptive behavior modifications, directly after the intervention. The authors did not perform repeated measurements over a period of time to determine the efficacy of the adaptive responses and do not expect the after-effects to imprint over a longitudinal period. Further, observed feedback and feedforward behavior may be dependent on the specific type of the perturbations experienced and may not show transfer to other forms of perturbations. Future studies will investigate the longevity of the acute after-effects produced in the present study. Finally, these effects were associated with PD patients’ average H&Y score of 2.7, and further study linking these two assessments to create a personalized treatment program is highly recommended.
6. Conclusion
PST is an efficient and effective way to discern reactive and proactive responses to therapeutic intervention. It has been suggested that this task-specific training approach may present a paradigm shift in fall prevention. While PD patients are still able to improve performance with practice, particularly in feedforward aspects of postural responses, reactive aspects of postural responses were not uniformly improved through practice. Because of this, patients with PD may require more training to achieve and retain motor learning and may require additional sensory information or motor guidance in order to facilitate this learning. These shortcomings in motor learning in PD could contribute to the degeneration in gait and balance often seen in the disease, as patients are unable to adapt to the gradual sensory and motor degradation. Research has shown that physical and exercise therapy can help PD patients to adapt new feedforward strategies to partially counteract these symptoms. In particular, balance, treadmill, resistance, and repeated perturbation training (PST) therapies have been shown to improve motor patterns in PD. However, much research is still needed to determine which of these therapies best alleviates which symptoms of PIGD, the needed dose and intensity of these therapies, the long-term retention effects, and the benefits of such technologies as augmented feedback, motorized perturbations, virtual reality, and weight-bearing assistance.
Data availability statement
The raw data supporting the conclusions of this article will be made available by the authors, without undue reservation.
Ethics statement
The studies involving humans were approved by Arizona State University Institutional Review Board. The studies were conducted in accordance with the local legislation and institutional requirements. The participants provided their written informed consent to participate in this study.
Author contributions
TL was responsible for compiling the sources used within this study and writing the drafted manuscript. TL, MO, and AL provided additional articles, discussed possible implications, and revised the manuscript. All authors contributed to the article and approved the submitted version.
Funding
The Bob and Renee Parsons Foundation sponsored the Falls Research Program, through which this research was completed, for the duration of the project. This research was supported by the National Science Foundation-Information and Intelligent Systems (IIS) and Smart and Connected Health (1065442, 1547466, and secondary 1065262), and NSF-CNS-1650566.
Acknowledgments
We would like to thank the Bob and Renee Parsons Foundation for sponsoring the Falls Research Program at the Muhammed Ali Movement Disorders Clinic. We would also like to thank the members of the Locomotion Research Laboratory and the Muhammad Ali Movement Disorders Clinic. Also, thanks to the National Science Foundation.
Conflict of interest
The authors declare that the research was conducted in the absence of any commercial or financial relationships that could be construed as a potential conflict of interest.
Publisher’s note
All claims expressed in this article are solely those of the authors and do not necessarily represent those of their affiliated organizations, or those of the publisher, the editors and the reviewers. Any product that may be evaluated in this article, or claim that may be made by its manufacturer, is not guaranteed or endorsed by the publisher.
References
1. Shulman, JM, De Jager, PL, and Feany, MB. Parkinson’s disease: genetics and pathogenesis. Annu Rev Pathol Mech Dis. (2011) 6:193–22. doi: 10.1146/annurev-pathol-011110-130242
2. Roemmich, RT, Nocera, JR, Stegemoller, EL, Hassan, A, Okun, MS, and Hass, CJ. Locomotor adaptation and locomotor adaptive learning in Parkinson’s disease and normal aging. Clin Neurophysiol. (2014) 125:313–9. doi: 10.1016/j.clinph.2013.07.003
3. Wilkinson, L, Khan, Z, and Jahanshahi, M. The role of the basal ganglia and its cortical connections in sequence learning: evidence from implicit and explicit sequence learning in Parkinson’s disease. Neuropsychologia. (2009) 47:2564–73. doi: 10.1016/j.neuropsychologia.2009.05.003
4. Frith, CD, Bloxham, CA, and Carpenter, KN. Impairments in the learning and performance of a new manual skill in patients with Parkinson’s disease. J Neurol Neurosurg Psychiatry. (1986) 49:661–8. doi: 10.1136/jnnp.49.6.661
5. Redgrave, P, Rodriguez, M, Smith, Y, Rodriguez-Oroz, MC, Lehericy, S, Bergman, H, et al. Goal-directed and habitual control in the basal ganglia: implications for Parkinson’s disease. Nat Rev Neurosci. (2010) 11:760–72. doi: 10.1038/nrn2915
6. Pascual-Leone, A, Grafman, J, Clark, K, Stewart, M, Massaquoi, S, Lou, JS, et al. Procedural learning in Parkinson’s disease and cerebellar degeneration. Ann Neurol. (1993) 34:594–602. doi: 10.1002/ana.410340414
7. Muslimović, D, Post, B, Speelman, JD, and Schmand, B. Motor procedural learning in Parkinson’s disease. Brain. (2007) 130:2887–97. doi: 10.1093/brain/awm211
8. Brichta, L, Greengard, P, and Flajolet, M. Advances in the pharmacological treatment of Parkinson’s disease: targeting neurotransmitter systems. Trends Neurosci. (2013) 36:543–54. doi: 10.1016/j.tins.2013.06.003
9. Calabresi, P, Picconi, B, Parnetti, L, and Di Filippo, M. A convergent model for cognitive dysfunctions in Parkinson’s disease: the critical dopamine-acetylcholine synaptic balance. Lancet Neurol. (2006) 5:974–83. doi: 10.1016/S1474-4422(06)70600-7
10. Yitayeh, A, and Teshome, A. The effectiveness of physiotherapy treatment on balance dysfunction and postural instability in persons with Parkinson’s disease: a systematic review and meta-analysis. BMC Sports Sci Med Rehabil. (2016) 8:1–10. doi: 10.1186/s13102-016-0042-0
11. Smania, N, Corato, E, Tinazzi, M, Stanzani, C, Fiaschi, A, Girardi, P, et al. Effect of balance training on postural instability in patients with idiopathic Parkinson's disease. Neurorehabil Neural Repair. (2010) 24:826–34. doi: 10.1177/1545968310376057
12. Pendt, LK, Reuter, I, and Muller, H. Motor skill learning, retention, and control deficits in Parkinsons disease. PLoS One. (2011) 6. doi: 10.1371/journal.pone.0021669
13. Nieuwboer, A, Rochester, L, Müncks, L, and Swinnen, SP. Motor learning in Parkinson’s disease: limitations and potential for rehabilitation. Park Relat Disord. (2009) 15:53–8. doi: 10.1016/S1353-8020(09)70781-3
14. Olson, M, Lockhart, TE, and Lieberman, A. Motor learning deficits in Parkinson’s disease (PD) and their effect on training response in gait and balance: a narrative review. Front Neurol. (2019) 10:62. doi: 10.3389/fneur.2019.00062
15. Gerards, MHG, McCrum, C, Mansfield, A, and Meijer, K. Perturbation-based balance training for falls reduction among older adults: current evidence and implications for clinical practice. Geriatr Gerontol Int. (2017) 17:2294–303. doi: 10.1111/ggi.13082
16. McCrum, C, Gerards, MHG, Karamanidis, K, Zijlstra, W, and Meijer, K. A systematic review of gait perturbation paradigms for improving reactive stepping responses and falls risk among healthy older adults. Euro Rev Aging Phys. (2017) 14:3. doi: 10.1186/s11556-017-0173-7
17. McCrum, C, Bhatt, TS, Gerards, MHG, Karamanidis, K, Rogers, MW, Lord, SR, et al. Perturbation-based balance training: principles, mechanisms and implementation in clinical practice. Front Sports Act Living. (2022) 4:1015394. doi: 10.3389/fspor.2022.1015394
18. Oates, AR, van Ooteghem, K, Frank, JS, Patla, AE, and Horak, FB. Adaptation of gait termination on a slippery surface in Parkinson's disease. Gait Posture. (2013) 37:516–20. doi: 10.1016/j.gaitpost.2012.09.002
19. Steib, S, Klamroth, S, Gaßner, H, Pasluosta, C, Eskofier, B, Winkler, J, et al. Perturbation during treadmill training improves dynamic balance and gait in Parkinson’s disease: a single-blind randomized controlled pilot trial. Neurorehabil Neural Repair. (2017) 31:758–68. doi: 10.1177/1545968317721976
20. Klamroth, S, Steib, S, Gaßner, H, Goßler, J, Winkler, J, Eskofier, B, et al. Immediate effects of perturbation treadmill training on gait and postural control in patients with Parkinson’s disease. Gait Posture. (2016) 50:102–8. doi: 10.1016/j.gaitpost.2016.08.020
21. Martelli, D, Luo, L, Kang, J, Kang, UJ, Fahn, S, and Agrawal, SK. Adaptation of stability during perturbed walking in Parkinson’s disease. Sci Rep. (2017) 7:17875. doi: 10.1038/s41598-017-18075-6
22. Hulzinga, F, Seuthe, J, D'Cruz, N, Ginis, P, Nieuwboer, A, and Schlenstedt, C. Split-Belt treadmill training to improve gait adaptation in Parkinson's disease. Move Disord Off J Move Disord Soc. (2023) 38:92–03. doi: 10.1002/mds.29238
23. Coelho, DB, de Oliveira, CEN, Guimarães, MVC, Ribeiro de Souza, C, Dos Santos, ML, and de Lima-Pardini, AC. A systematic review on the effectiveness of perturbation-based balance training in postural control and gait in Parkinson's disease. Physiotherapy. (2022) 116:58–71. doi: 10.1016/j.physio.2022.02.005
24. Hulzinga, F, de Rond, V, Vandendoorent, B, Gilat, M, Ginis, P, D'Cruz, N, et al. Repeated gait perturbation training in Parkinson's disease and healthy older adults: a systematic review and Meta-analysis. Front Hum Neurosci. (2021) 15:732648. doi: 10.3389/fnhum.2021.732648
25. Goetz, CG, Poewe, W, Rascol, O, Sampaio, C, Stebbins, GT, Counsell, C, et al. Movement Disorder Society task force report on the Hoehn and Yahr staging scale: status and recommendations. Mov Disord. (2004) 19:1020–8. doi: 10.1002/mds.20213
26. Parijat, P, and Lockhart, TE. Effects of moveable platform training in preventing slip-induced falls in older adults. Ann Biomed Eng. (2012) 40:1111–21. doi: 10.1007/s10439-011-0477-0
27. Parijat, P, Lockhart, TE, and Liu, J. Effects of perturbation-based slip training using a virtual reality environment on slip-induced falls. Ann Biomed Eng. (2015) 43:958–67. doi: 10.1007/s10439-014-1128-z
28. Parijat, P, Lockhart, TE, and Liu, J. EMG and kinematic responses to unexpected slips after slip training in virtual reality. IEEE Trans Biomed Eng. (2015) 62:593–9. doi: 10.1109/TBME.2014.2361324
29. Liu, J, Lockhart, TE, Parijat, P, McIntosh, JD, and Chiu, YP. Comparison of slip training in VR environment and on moveable platform. Biomed Sci Instrum. (2015) 51:189–97.
30. Hof, AL, Gazendam, MGJ, and Sinke, WE. The condition for dynamic stability. J Biomech. (2005) 38:1–8. doi: 10.1016/j.jbiomech.2004.03.025
31. Costa, M, Goldberger, AL, and Peng, CK. Multiscale entropy analysis of complex physiologic time series. Phys Rev Lett. (2002) 89:6–9. doi: 10.1103/PhysRevLett.89.068102
32. Ribeiro de Souza, C, Ávila de Oliveira, J, Takazono, PS, da Silva Rezende, L, Silva-Batista, C, Coelho, DB, et al. Perturbation-based balance training leads to improved reactive postural responses in individuals with Parkinson's disease and freezing of gait. Eur J Neurosci. (2023) 57:2174–86. doi: 10.1111/ejn.16039
33. Hausdorff, JM, Gruendlinger, L, Scollins, L, O’Herron, S, and Tarsy, D. Deep brain stimulation effects on gait variability in Parkinson s disease. Mov Disord. (2009) 24:1688–92. doi: 10.1002/mds.22554
34. Pötter-Nerger, M, and Volkmann, J. Deep brain stimulation for gait and postural symptoms in Parkinson’s disease. Mov Disord. (2013) 28:1609–15. doi: 10.1002/mds.25677
35. Hikosaka, O. Role of basal ganglia in control of innate movements, learned behavior and cognition—a hypothesis. Adv Behav Biol Basal Ganglia. (1994) 4:589–96. doi: 10.1007/978-1-4613-0485-2_61
36. Hikosaka, O. Basal ganglia - possible role in motor coordination and learning. Curr Opin Neurobiol. (1991) 1:638–43. doi: 10.1016/S0959-4388(05)80042-X
37. Shen, X, and Mak, MKY. Technology-assisted balance and gait training reduces falls in patients with Parkinson’s disease: a randomized controlled trial with 12-month follow-up. Neurorehabil Neural Repair. (2015) 29:103–11. doi: 10.1177/1545968314537559
38. Protas, EJ, Mitchell, K, Williams, A, Qureshy, H, Caroline, K, and Lai, EC. Gait and step training to reduce falls in Parkinson’s disease. Neuro Rehab. (2005) 20:183–90.
39. Jöbges, M, Heuschkel, G, Pretzel, C, Illhardt, C, Renner, C, and Hummelsheim, H. Repetitive training of compensatory steps: a therapeutic approach for postural instability in Parkinson’s disease. J Neurol Neurosurg Psychiatry. (2004) 75:1682–7. doi: 10.1136/jnnp.2003.016550
40. Agostino, R, Sanes, JN, and Hallett, M. Motor skill learning in Parkinson’s disease. J Neurol Sci. (1996) 139:218–26. doi: 10.1016/0022-510X(96)00060-3
41. Soliveri, P, Brown, RG, Jahanshahi, M, and Marsden, CD. Effect of practice on performance of a skilled motor task in patients with Parkinson’s disease. J Neurol Neurosurg Psychiatry. (1992) 55:454–60. doi: 10.1136/jnnp.55.6.454
42. Worringham, CJ, and Stelmach, GE. Practice effects on the Preprogramming of discrete movements in Parkinsons disease. J Neurol Neurosurg Psychiatry. (1990) 53:702–4. doi: 10.1136/jnnp.53.8.702
43. Behrman, AL, Cauraugh, JH, and Light, KE. Practice as an intervention to improve speeded motor performance and motor learning in Parkinson’s disease. J Neurol Sci. (2000) 174:127–36. doi: 10.1016/S0022-510X(00)00267-7
44. Jessop, RT, Horowicz, C, and Dibble, LE. Motor learning and Parkinson disease: refinement of movement velocity and endpoint excursion in a limits of stability balance task. Neurorehabil Neural Repair. (2006) 20:459–67. doi: 10.1177/1545968306287107
45. Swinnen, SP, Steyvers, M, Van Den Bergh, L, and Stelmach, GE. Motor learning and Parkinson’s disease: refinement of within-limb and between-limb coordination as a result of practice. Behav Brain Res. (2000) 111:45–59. doi: 10.1016/S0166-4328(00)00144-3
46. Peterson, DS, Dijkstra, BW, and Horak, FB. Postural motor learning in people with Parkinson's disease. J Neurol. (2016) 263:1518–29. doi: 10.1007/s00415-016-8158-4
47. Monaghan, AS, Finley, JM, Mehta, SH, and Peterson, DS. Assessing the impact of dual-task reactive step practice in people with Parkinson's disease: a feasibility study. Hum Mov Sci. (2021) 80:102876. doi: 10.1016/j.humov.2021.102876
48. Jankovic, J. Parkinson’s disease: clinical features and diagnosis. J Neurol Neurosurg Psychiatry. (2008) 79:368–76. doi: 10.1136/jnnp.2007.131045
49. Sveinbjornsdottir, S. The clinical symptoms of Parkinson’s disease. J Neurochem. (2016) 139:318–24. doi: 10.1111/jnc.13691
50. Barajas, JS, and Peterson, DS. First-trial protective step performance before and after short-term perturbation practice in people with Parkinson's disease. J Neurol. (2018) 265:1138–44. doi: 10.1007/s00415-018-8821-z
51. Smulders, K, Esselink, RA, de Swart, BJ, Geurts, AC, Bloem, BR, and Weerdesteyn, V. Postural inflexibility in PD: does it affect compensatory stepping? Gait Posture. (2014) 39:700–6. doi: 10.1016/j.gaitpost.2013.10.003
52. de Kam, D, Nonnekes, J, Oude Nijhuis, LB, Geurts, ACH, Bloem, BR, and Weerdesteyn, V. Dopaminergic medication does not improve stepping responses following backward and forward balance perturbations in patients with Parkinson's disease. J Neurol. (2014) 261:2330–7. doi: 10.1007/s00415-014-7496-3
Keywords: Parkinson’s disease, accidental falls, protective step training, motor learning, gait and balance, motor adaptability, feedforward and feedback, physical therapy
Citation: Lockhart T, Frames C, Olson M, Moon SH, Peterson D and Lieberman A (2023) Effects of protective step training on proactive and reactive motor adaptations in Parkinson’s disease patients. Front. Neurol. 14:1211441. doi: 10.3389/fneur.2023.1211441
Edited by:
Emily Keshner, Temple University, United StatesReviewed by:
Keith M. McGregor, University of Alabama at Birmingham, United StatesJacky Ganguly, Institute of Neurosciences, Kolkata (I-NK), India
Philipp Wanner, Heidelberg University, Germany
Copyright © 2023 Lockhart, Frames, Olson, Moon, Peterson and Lieberman. This is an open-access article distributed under the terms of the Creative Commons Attribution License (CC BY). The use, distribution or reproduction in other forums is permitted, provided the original author(s) and the copyright owner(s) are credited and that the original publication in this journal is cited, in accordance with accepted academic practice. No use, distribution or reproduction is permitted which does not comply with these terms.
*Correspondence: Thurmon Lockhart, VGh1cm1vbi5Mb2NraGFydEBhc3UuZWR1